Abstract
Polyphasic analysis was carried out of natural samples and strains isolated from Phormidium mats, collected from different Spanish rivers with distinct water quality. 16S rRNA gene analysis separated five main clusters in a phylogenetic tree corresponding to five main, previously observed morphotypes. Two isolated strains and three natural samples had a relatively homogeneous morphology, which could correspond to Phormidium autumnale, and their sequences grouped in a well-supported cluster (morphotype–genotype I). However, ultrastructural differences were found among representatives from this cluster, and three clades could be separated in a further phylogenetic analysis. The samples belonging to the first clade were characteristic of hypertrophic waters, while representatives of the second grew in mesotrophic conditions, and those of the third in eutrophic waters. These results suggested that our representatives of morphotype–genotype I belong to three different species. According to our results and other published data, complementary information was obtained on the basis of morphological, phylogenetic and ecological criteria. Morphotype–genotype II, corresponding to Phormidium terebriforme, is a potential indicator of polluted waters. Oscillatoria tenuis, corresponding to morphotype–genotype III, is characteristic of eutrophic conditions. Phormidium mats identified as Phormidium corium (morphotype–genotype IV) were found to be characteristic of waters with low nutrient levels. Finally, morphotype–genotype V, identified as Phormidium aerugineo-caeruleum was dominant in oligo- to mesotrophic conditions.
Introduction
Cyanobacteria are widespread and abundant microorganisms found in many aquatic and terrestrial ecosystems (Whitton & Potts, Citation2000). Members of the order Oscillatoriales, especially the genus Phormidium, are widely distributed and comprise diverse morphotypes, which dominate in various freshwater microbial mats and soils (Komárek & Anagnostidis, Citation2005).
Understanding the role of a particular cyanobacterium in nature requires it to be correctly identified, but identification of field populations within the cyanobacteria is often difficult. The bacteriological approach to cyanobacterial classification, based on the study of axenic strains in culture, is insufficient for use in ecological research, because the number of strains studied is too restricted (Garcia-Pichel et al., Citation1996). Surveys of 16S rRNA variation from environmental samples suggest that culturable bacteria account for less than 0.1% of the species in a given environment (Cohan, Citation2011). On the other hand, identification based on the traditional botanical approach sometimes makes characterization extremely difficult because of the high phenotypic variability among similar morphotypes observed in different environmental conditions, or differences within the same morphospecies, including differences in biochemical and physiological properties (Komárek & Anagnostidis, Citation2005). The application of molecular methods in the identification and phylogeny of cyanobacteria has increased dramatically in recent years, with general agreement about the need for ‘polyphasic’ approaches that combine characterizations of genotypes and their phenotypic expressions (Komárek & Anagnostidis, Citation2005).
Phormidium is a genus widely recognized by many cyanobacteriologists, and also by those who are not specialists in cyanobacteria but who work in the related fields of environmental sciences or physiology. The earliest taxonomic classification of filamentous cyanobacteria is Gomont's Monographie des Oscillatoriées (Gomont, Citation1892), followed by Geitler (Citation1932), Desikachary (Citation1959) and more recently Whitton (Citation2011). Komárek & Anagnostidis (Citation2005) promoted a revised version of the genus. On the other hand, Rippka et al. (Citation1979), in a classification system for cyanobacteria based on axenic cultures, included Phormidium within the ‘LPP group’ (Lyngbya–Phormidium–Plectonema complex) of genera. Their system was adopted with minor modifications in the first and second editions of Bergey's Manual of Systematic Bacteriology (Castenholz & Waterbury, Citation1989; Castenholz et al., Citation2001).
There have recently been many studies of the Phormidium-group based on phenotypic and genotypic analyses (mostly using the 16S rRNA gene) (Casamatta et al., Citation2005; Marquardt & Palinska, Citation2007; Comte et al., Citation2007; Palinska & Marquardt, Citation2008; Heath et al., Citation2010; Strunecký et al., Citation2010; Palinska et al., Citation2011; Hašler et al., Citation2012; Sciuto et al., Citation2012). These studies have revealed considerable diversity and heterogeneity, and demonstrated that the Phormidium group is not phylogenetically coherent, thereby highlighting the need for a comprehensive revision.
In previous studies concerning the diversity of benthic cyanobacterial communities in Spanish rivers we have described differences in the distribution of the cyanobacterial communities along rivers or changes in enzymatic activities in relation to changes in nutrient content (Douterelo et al., Citation2004; Perona & Mateo, Citation2006; Mateo et al., Citation2010). Relationships between cyanobacteria and the physical and chemical characteristics of waters are important for determining the ecological characteristics of these organisms. Recently, we found macroscopic microbial mats built by Phormidium-like cyanobacteria, in different rivers with diverse water quality (from oligotrophic to hypertrophic conditions), which led us to hypothesize that they belonged to different taxa. Thus, the aim of the present study was to establish whether there is a relationship between the trophic status of waters and the occurrence of different filamentous cyanobacteria. The study focuses on field Phormidium mats and isolates from four siliceous rivers (Guadarrama, Guadalix, Manzanares and Mediano) in the central region of Madrid (Spain), by means of a polyphasic approach, including morphological, phylogenetic and ecological analyses.
Materials and methods
Sampling sites and sampling procedure
Benthic cyanobacterial mats were collected from various locations along Spanish rivers with differences in water quality, which we have described previously (Douterelo et al., Citation2004; Serrano et al., Citation2004; Rodríguez et al., Citation2007; Carmona et al. Citation2011). The sampling sites along the Guadarrama (40º 47′ N, 4º 02′ W), Guadalix (upstream, 40º 50′ N, 3º 45′ W, and downstream, 40º 42′ N, 3º 35′ W) Manzanares (40º 46′ N, 3º 55′ W) and Mediano (40º 47′ N, 3º 50′ W) rivers are located in central Spain, near Madrid, in the Tajo Basin (). The geomorphology of the Tajo Basin encompasses large granite-dominated highlands where our sampling sites are located. They are dominated by uplifted blocks of siliceous materials from the Tertiary period. There is a typical continental Mediterranean climate in the high mountains, characterized by cold winters and high summer temperatures; at least 80% of the rain falls during the three winter months. The geomorphological conditions and climate of the area have led to the formation of pine and oak forests.
Table 1. Physicochemical characteristics of water at sampling sites in the rivers Guadarrama, Guadalix, Manzanares and Mediano. Data are given as mean ± SD (range)
Samples of water were taken in one-litre wide-mouthed polyethylene jars and kept cool in the dark. Nutrient chemical measurements and hardness were made in the field within one hour of collection using a DRELL 2010 portable laboratory (Hach, Loveland, Colorado). Water sampling was done early in the morning, and each sample was analysed in triplicate. Cyanobacterial mats were found predominantly on rocky substrata but were collected from fine sandy substrata in the Guadarrama river. Samples for strain isolation were collected in sterile plastic bottles and kept at 4ºC. Aliquots for DNA extraction (see below) were frozen and aliquots for microscopical examination were fixed with formaldehyde at a final concentration of 4%. The dates of sampling were 7 July 2009 (Guadarrama), 28 July 2009 (Guadalix) and 29 September 2009 (Manzanares and Mediano).
Water analysis
Dissolved oxygen, temperature, pH and conductivity were measured in situ at every sampling site. The following electrodes were used and set up on the river bank: for conductivity, Crison CDTM-523 (Crison, Barcelona, Spain); temperature and dissolved oxygen, WTW OXI196 (Wissenschaftliche Technische Werkstätten, Weilheim, Germany) and pH, WTW pH-96. Hardness was estimated titrimetrically and nutrient concentrations (NH4 +-N, NO3 −-N, NO2 −-N, PO4 3–-P) were determined using colorimetric methods, adapted from the Standard Methods for the Examination of Water and Wastewater (APHA, Citation1992) as previously described (Perona et al., Citation1999). Ammonium-N was measured by the Nessler method (estimated detection limit 0.05 mg l−1, precision ± 0.015 mg l−1), reading the absorbance at 425 nm. When values were close to the detection limit, the salicylate method was used (detection limit 0.01 mg l−1), reading absorbance at 655 nm. NO3 −-N was analysed using a modification of the cadmium reduction protocol, using gentisic acid instead of 1-naphthylamine (estimated detection limit 0.5 mg l−1), reading absorbance at 500 nm. Samples below that detection limit were measured by a low-range method, which is an expanded modification of the former one, using a chromotropic acid indicator (estimated detection limit 0.05 mg l−1, precision ± 0.01 mg l−1) and reading at the same wavelength. NO2 −-N was determined colorimetrically using chromotropic and sulphanilic acids as indicators (estimated detection limit 0.01 mg l−1, precision ± 0.01 mg l−1) and reading absorbance at 507 nm. PO4 3–-P was measured by a modification of the molybdenum blue procedure (estimated detection limit 0.01 mg l−1, precision ± 0.01 mg l−1) reading absorbance at 890 nm. Hardness was estimated with a Cal Ver 2 calcium indicator (detection limit 10 mg l−1), titrating with 0.08M EDTA in alkaline medium (Hach, Loveland, Colorado). Every analysis was performed three times. The standard deviations of the pseudoreplicates remained within the typical precision range for each method.
Cyanobacterial isolation and morphological characterization
Each cyanobacterial strain was isolated from a single trichome grown on agar plates with BG11 medium (Rippka et al., Citation1979) in light : dark periods of 16 : 8 h at a temperature of 18ºC. The intensity of light during the light period was 20 µmol photon m−2 s−1. After a few days of cultivation, visible trichomes were observed and separately transferred to sterile liquid BG11 medium in tubes. Five strains were successfully isolated from the Guadarrama river following the sampling days mentioned above, and one further isolate (strain BGU3) was isolated on September 2005. All strains are deposited in the Universidad Autónoma de Madrid, Spain, Culture Collection (UAM).
Identification and morphological characterization of the cyanobacteria were performed under a dissecting microscope (Leica, Leica Microsystems, Wetzlar, Germany) and with an Olympus BH2-RFCA photomicroscope (Olympus, Tokyo, Japan) equipped with phase-contrast and video camera systems (Leica DC Camera). The strains were identified according to Geitler (Citation1932), Komárek & Anagnostidis (Citation2005) and Whitton (Citation2011). All important key features that are generally taken into consideration to identify natural samples and isolates, such as cell width, cell length, attenuation of apical cells, and sheath morphology, were studied in samples of 25–50 cells.
For ultrastructural studies by transmission electron microscopy (TEM), samples were washed three times in 0.1 M sodium phosphate buffer pH 7.2 (the same buffer being used for the whole procedure), transferred into 2% bacteriological agar in buffer, and fixed in 3.1% glutaraldehyde in buffer for 3 h at 4ºC. Post-fixation was done on 1–2 mm agar blocks with osmium tetroxide in buffer for 2 h at 4ºC. Samples were then dehydrated in ethanol and embedded in Spurr's resin (Spurr, Citation1969), sectioned in a Reichert–Jung Ultracut E ultramicrotome, stained with lead citrate (Reynolds, Citation1963) and viewed under a Jeol 1010 (Jeol, Tokyo, Japan) transmission electron microscope.
Isolation of genomic DNA and amplification of the 16S rRNA gene
Total genomic DNA from isolated cultures or frozen field samples of cyanobacteria was obtained using a modification of previously described DNA extraction procedures (Rodríguez et al., Citation2007), in which a step was added at the beginning of the protocol: 1.5-ml aliquots of cell suspension of each culture were homogenized and exposed to three freeze–thaw cycles, alternating immersion in liquid nitrogen and heating to 60ºC. Cyanobacterial cells were then lysed by a hot extraction procedure performed with 4% sodium dodecyl sulfate, 250 mM NaCl, 100 mM EDTA and 350 mM guanidine isothiocyanate at 68°C, after vortex mixing for 1 min and sonication for 2 min. After incubation, the suspension was centrifuged for 15 min at 13 000 × g, and 600 µl of the supernatant phase was mixed with 75 µl of 5 M potassium acetate, 250 µl of 40% polyethylene glycol 8000, and 10 µg of glycogen. Crude DNA extracts were partially cleaned using hexadecyltrimethylammonium bromide (CTAB), chloroform extraction, isopropanol precipitation and filtration with Microcon 100 microconcentrators (Amicon, Beverly, MA).
The 16S rRNA gene was amplified using the forward primer pA (Edwards et al., Citation1989) and the reverse primer PLG2.1 (Urbach et al., Citation1992) for isolates, and the cyanobacteria-specific 23S30R (Lepère et al., Citation2000) for mat samples, which produced amplifications from 1200 to 1500 bp. Thermocycling was performed using a Perkin–Elmer GeneAmp 2400 PCR system under conditions previously described by Mateo et al. (Citation2011) and following the PCR conditions of Gkelis et al. (Citation2005). For sequencing field samples (cyanobacterial mats) a Qiagen PCR Cloningplus kit (Qiagen, Hilden, Germany) was used. Recombinant clones, carrying the correct-sized insert, were sequenced in both strands. The products were purified using the Real Clean Spin kit (REAL, Durviz S, L., Valencia, Spain) and commercially sequenced in both directions. The nucleotide sequences obtained in this work have been deposited in the GenBank database (accession numbers: JN382218–JN382243) and the alignment is included here as a Supplementary file.
Phylogenetic analyses
For the phylogenetic analyses, 16S rRNA gene sequences were aligned using the CLUSTAL W program (Thompson et al., Citation1994) and pairwise similarities were calculated using the web-based tool EzTaxon (http://www.eztaxon.org). The alignment was checked and corrected manually with BIOEDIT (version 7.0.5.3) (Hall, Citation1999).
Sequences of 1235–1400 bp were determined (on both strands) for all samples analysed except one (752 bp). Sequences were subjected to a BLAST search (http://www.ncbi.nlm.nih.gov/blast) and representative sequences assigned to oscillatorian cyanobacteria were added to the alignment. The MALLARD program (Ashelford et al., Citation2006) was used to identify anomalous 16S rRNA gene sequences within the multiple sequence alignment. A first phylogenetic tree was constructed with taxa chosen to represent clades identified in previous analyses (Casamatta et al., Citation2005; Comte et al., Citation2007; Marquardt & Palinska, Citation2007; Palinska & Marquardt, Citation2008; Siegesmund et al., Citation2008; Strunecký et al., Citation2010; Dvořák et al., Citation2012; Hašler et al., Citation2012; Sciuto et al., Citation2012). As the majority of the sequences found in GenBank were partial sequences (about 900–1000 bp), a second phylogenetic tree was constructed selecting complete or nearly complete sequences of the 16S rRNA genes (corresponding to Escherichia coli positions 41–1567, or at least about 1300 bp). The phylogenetic trees were computed using maximum likelihood (ML) neighbour-joining (NJ) and maximum parsimony (MP) analyses, conducted in MEGA 5.0. (Tamura et al., Citation2011). For ML, the general time-reversible model (GTR) was found to fit the data best, assuming a discrete gamma distribution with four categories of site-to-site variability of change with the nearest-neighbour-interchange algorithm; we assessed robustness by 100 bootstrap samples. Distances for the NJ tree were estimated by the algorithm of Tajima & Nei (Citation1984); nucleotide positions containing gaps and missing data were initially retained for all such sites in the analyses, and then excluded as necessary in the pairwise distance estimation (pairwise deletion option). The maximum parsimony method was used with the close-neighbour-interchange search algorithm with random tree addition; missing information and alignment gap sites were treated as missing data in the calculation of tree length. Bootstrap analysis of 1000 replicates was performed for the NJ and MP trees (Felsenstein, Citation1985).
Statistical analysis
Multivariate analyses are useful ordination methods for describing ecosystem gradients, and are widely applied to ecological studies in rivers. For this reason, principal component analysis (PCA) of the physicochemical variables measured was carried out, using SPSS Statistics v.17.0 (IBM, Armonk, New York, U.S.A.). The relation between species distribution and the physicochemical variables was assessed using a canonical correspondence analysis (CCA), followed by Monte Carlo test (999 permutation, α = 0.05) to verify the statistical significance of these relationships. The analysis was carried out using CANOCO software for Windows, version 4.5 (Ter Braak & Šmilauer, Citation2002). Environmental variables included in the analysis were: water temperature, pH, dissolved inorganic nitrogen (DIN), soluble reactive phosphorus (SRP = PO4 3–-P), conductivity, hardness and dissolved oxygen (DO). DIN was calculated as the sum of the three inorganic nitrogen forms measured in water (NH4 +-N, NO3 −-N, NO2 −-N).
Results
Environmental characteristics
The physicochemical variables (range, mean and SD) measured at sampling locations from each river are shown in Table 1. In order to detect general trends in the variation of the physicochemical characteristics of the waters, a principal component analysis (PCA) was carried out (), allowing the variation to be summarized and presented in a more interpretable form. The first two principal components explain 96.5% of the variance. Component I (73.3% of the variance) is correlated to a group of variables that jointly reflect the degree of pollution by sewage, comprising the ion content of waters (including nutrients), temperature, and dissolved oxygen, representing mainly the degree of eutrophication. Component II (23.2% of the variance) is mainly characterized by the pH. Nutrient levels were higher in the Guadarrama river, followed by Guadalix downstream, Manzanares, Guadalix upstream, and the Mediano stream, where the best water quality was found. The greatest amounts of PO4 3–-P were detected at the Guadarrama river sampling site, and can probably be attributed to agricultural and domestic waste disposal. Also, an increase in reduced (NH4 +-N) and oxidized nitrogen compounds (NO2 −-N, NO3 −-N) was detected, indicating the potential continuous presence of waste in this area. On the basis of these results, the trophic status of the five sampling sites was established () according to phosphorus classification limits (Kelly & Whitton, Citation1998). The Guadarrama river at the sampling site (downstream) was classified as hypertrophic; the Guadalix was eutrophic downstream, while upstream it was oligo-mesotrophic; the Manzanares was mesotrophic at the sampling location; and the Mediano stream was oligotrophic.
Table 2. Principal component analysis of physical and chemical properties of water. Non-significant coefficients (r < 0.3) have been omitted. SRP, soluble reactive phosphorus; DIN, dissolved inorganic nitrogen
Table 3. Morphotypes observed in the seven natural samples (mats) collected at different rivers in the central region of Spain, and in the six strains isolated from these mats. Measurements are given as mean ± SD (range) for n = 30
Morphology and ultrastructure
Seven environmental samples of benthic Phormidium mats from the four Spanish rivers, and six isolated strains from these mats, were evaluated microscopically to characterize them morphologically (Table 3; –18). These observations confirmed that the mat-forming species belonged to the order Oscillatoriales and that they mostly corresponded to the genus Phormidium, although a representative of Oscillatoria (in which sheaths were never observed) was found in one of the Phormidium-dominated mats. Morphological characteristics (cell dimensions, apical cell profiles, presence of calyptra, etc.) are presented in Table 3. Five different morphotypes were identified.
Figs 1–18. Photomicrographs of different morphotypes found in environmental samples and isolated strains. 1–6. Morphotype–genotype I. 1. Phormidium filaments in mat from Manzanares river. 2. Phormidium filaments in mat from Guadalix river downstream. 3. Phormidium filaments in mat from Guadarrama river. 4. Phormidium filaments of strain 2TGU3 isolated from Guadarrama mat (UAM 396). 5, 6. Phormidium filaments of strain BGU3 isolated from benthic biofilm of Guadarrama river (5, morphology of first filaments isolated, 6, present morphology) (UAM 361). 7–9. Morphotype–genotype II. 7. Phormidium terebriforme filaments in mat from Guadarrama river. 8. Strain Phormidium terebriforme 3TGU3 isolated from Guadarrama mat (UAM 408). 9. Strain Phormidium terebriforme 5TGU3 isolated from Guadarrama mat (UAM 409). 10–12. Morphotype–genotype III. 10. Oscillatoria tenuis filaments in mat from Guadarrama river. 11. Strain Oscillatoria tenuis 1TGU3 isolated from Guadarrama mat (UAM 410). 12. Strain Oscillatoria tenuis 4TGU3 isolated from Guadarrama mat (UAM 411). 13–15. Morphotype–genotype IV. 13. Phormidium corium filaments in mat from Mediano stream. 14, 15. Phormidium corium filaments in mat from Guadalix river upstream. 16–18. Morphotype–genotype V. 16, 17. Phormidium aerugineo-caeruleum filaments in mat from Mediano stream. 18. Phormidium aerugineo-caeruleum filaments in mat from Manzanares river. The arrows indicate intensely pigmented cells. Scale bar = 10 µm.
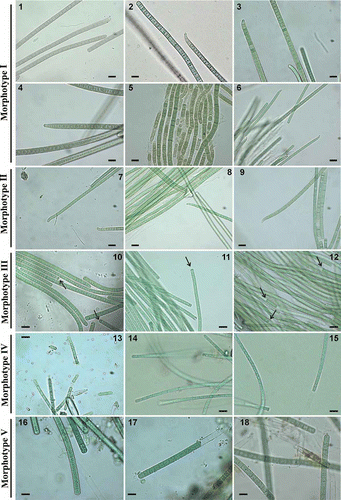
The most abundant morphotype observed (morphotype I, –6) corresponds to the descriptions of Phormidium autumnale. This morphotype was found in Phormidium mats from the Manzanares river (Fig. 1), the Guadalix downstream () and the Guadarrama (), as well as in strain 2TGU3 (), which was isolated from a mat in the Guadarrama, and in strain BGU3 (, ), isolated four years earlier from a benthic biofilm at the same location in the same river. The organisms were morphologically similar, with trichomes 4.0 to 8.0 µm wide, unconstricted at the cross-walls, attenuated and frequently curved at the tips, and ending with conical apical cells with calyptra. Their ultrastructure is shown in Figs 19–24. Isolated strains 2TGU3 and BGU3 showed a fascicular thylakoid pattern (sensu Casamatta et al., Citation2005) in which the thylakoids were arranged in fascicles running parallel to the longitudinal axis of the cell (Figs 19, 21) and mostly radial in cross-section (Figs 20, 22). The sections of mats from the population of the downstream Guadalix (Figs 23, 24) showed less regular, somewhat fragmented thylakoid patterns. In addition, those trichomes from the Guadalix were always narrower (5.8–6.6 µm) than those of the Guadarrama samples (7.0–8.0 µm) (Table 3), while the sheath was thinner than in the Guadarrama samples (Figs 19–24).
Figs 19–24. Transmission electron micrographs of isolated strains and environmental samples. 19, 20. Strain Phormidium sp. BGU3, in longitudinal sections (Fig. 19) and in cross-section (Fig. 20). 21, 22. Strain Phormidium sp. 2TGU3, in longitudinal sections (Fig. 21) and in cross-section (Fig. 22). Both strains show a fascicular pattern with thylakoids running parallel to the longitudinal axis of the filament. 23, 24. Phormidium sp. in mat from Guadalix river downstream, with thylakoids irregularly stacked within the cells: longitudinal sections (Fig. 23 and cross-section (Fig. 24). Scale bars = 0.5 μm (Figs 19, 20, 23, 24) and 1 μm (Figs 21, 22).
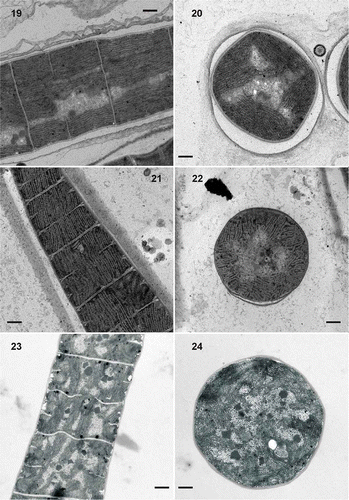
A different morphotype (Morphotype II, Table 3, Figs 7–9), identified as Phormidium terebriforme (=Oscillatoria terebriformis) was also found in the Phormidium mat from the Guadarrama (Fig. 7), and two isolates were obtained from this mat, strains 3TGU3 (Fig. 8) and 5TGU3 (Fig. 9). Filaments in natural samples and strains were morphologically identical, with gradually attenuated apical cells that were hooked and without terminal calyptra. The blue-green trichomes were not constricted at the cross-walls, although sometimes some slightly constricted trichomes could be observed. In addition, necridic cells were observed. Cells were 3.3–5 µm wide, 1.5–3.8 µm long.
Morphotype III, assigned to Oscillatoria tenuis, was observed in two isolates from the Guadarrama river (strains 1TGU3 and 4TGU3) and some trichomes from an environmental mat from this river (Table 3, Figs 10–12). This morphotype showed straight blue-green trichomes with cells 3.5–6.2 µm wide and 1.3–2.8 µm long, narrowed cross-walls, the presence of necridic cells, and intensely pigmented hemispherical terminal cells (see arrows in Figs 10–12).
Morphotype IV, identified as Phormidium corium was found in the Phormidium mats from the Mediano stream (epilithic) and the Guadalix river, upstream (Table 3, Figs 13–15). It had straight trichomes with unconstricted cross-walls, thin sheaths, with cells 2.8–4.2 µm wide, and 3.0–7.0 µm long. Terminal cells were acute-conical or rounded.
Environmental samples from the Mediano stream (epiphytic) and the Manzanares river had a similar morphology (Morphotype V, Table 3, Figs 16–18), with blue-green filaments with sheaths 0.5 µm thick, and cells 6.0–7.6 µm wide, unconstricted cross-walls, and granular cell contents. The apical cells were slightly conical or rounded. These cyanobacteria were identified as belonging to Phormidium aerugineo-caeruleum (=Lyngbya aerugineo-coerulea).
Phylogenetic analysis
The 16S rRNA gene was used as a molecular marker to distinguish between related cyanobacterial isolates and to compare them with environmental samples. Phylogenetic analyses were made to determine the relationships of the 16S rRNA gene sequences from six isolated strains and 19 clones from field samples to other Oscillatoriales sequences obtained from GenBank, such as representatives of Phormidium, Oscillatoria, Microcoleus, Leptolyngbya and Pseudanabaena. Trees were constructed using the NJ, MP and ML methods, but since similar topologies were obtained by the three methods, only the ML tree is presented, with an indication of the bootstrap values for all three approaches (Fig. 25). The resulting phylogenetic tree revealed five principal clusters corresponding to the five previously described morphotypes. Clade I included nine sequences from this study as well as 29 representatives from GenBank, mainly Phormidium autumnale and Microcoleus vaginatus strains. The sequence similarity within clade I ranged from 95.9% to 100%.
Fig. 25. Maximum likelihood tree based on analysis of the 16S rRNA gene (925 bp), showing the position of the sequences obtained from the present study (in bold). Numbers near nodes indicate bootstrap values greater than or equal to 60% for ML, NJ, and MP analyses.
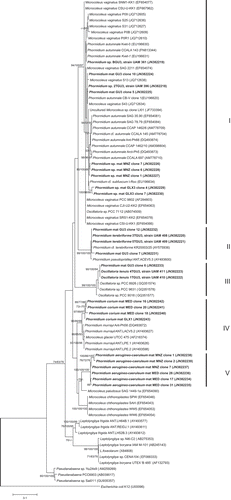
Morphotypes in clade II were isolates 3TGU3 and 5TGU3 from a Guadarrama mat, identified as Phormidium terebriforme, and clones from the Phormidium mat from the Guadarrama river; they showed 99.9% pairwise similarity and grouped with P. cf. terebriformis and P. pseudopriestleyi from the database with high bootstrap support (100/99/99).
Strains 1TGU3 and 4TGU3 (100% similarity), assigned to Oscillatoria tenuis, were included in clade III with other Oscillatoria sp. sequences from the database, supported by high bootstrap values (100/99/98). They exhibited 98.9–99.3% sequence similarity.
Clade IV contained sequences from Phormidium mats identified as P. corium from the Mediano stream (epilithic) and the Guadalix river upstream, with 99.3% similarity. Both environmental samples grouped with P. murrayi and Microcoleus glaciei (previously classified as Phormidium murrayi), exhibiting 97–98.2% sequence similarity.
Environmental samples of Phormidium from the Mediano stream (epiphytic sample) and the Manzanares river (three clones from each sample) identified as P. aerugineo-caeruleum were grouped in clade V, with 98.8–99.7% sequence similarity. The nearest strain in the sister branch was Microcoleus paludosus, which exhibited only 93.8% sequence similarity.
In order to investigate the phylogenetic relationships within clade I in more detail, nearly complete 16S rDNA sequences were selected to construct a new tree (Fig. 26). In this tree we were able to separate our sequences into subclades Ia, Ib and Ic. Subclade Ia was represented by the isolated strains from Guadarrama mat, 2TGU3 and BGU3, and two clones obtained from natural samples from Guadarrama river, as well as representatives of Microcoleus vaginatus, Phormidium autumnale and Oscillatoria sp. The second subclade, Ib, contained the three clones from the Phormidium mat from Manzanares river. Subclades Ia and Ib included sequences with an 11-bp insert within the 16S rRNA gene (between E. coli positions 463 and 468), which has previously been described in some Microcoleus from desert soils (Garcia-Pichel et al., Citation2001). Sequence similarities between our representatives of subclades Ia and Ib ranged from 97.3% to 98.7%. Subclade Ic included two sequences from the environmental cyanobacterial mat of Phormidium from Guadalix river downstream, as well as other sequences from the database mainly belonging to P. autumnale. Sequences similarities between representatives of these clusters and clusters Ia and Ib were 96–98.1%, and 97.1–98.1%, respectively.
Fig. 26. Maximum likelihood tree constructed including only complete or nearly complete 16S rRNA gene sequences (E. coli positions 41–1567, or at least about 1300 bp) of representatives of clade I in previous tree (Fig. 25), showing the position of the sequences obtained from the present study (in bold). Numbers near nodes indicate bootstrap values greater than or equal to 60% for ML, NJ, and MP analyses.
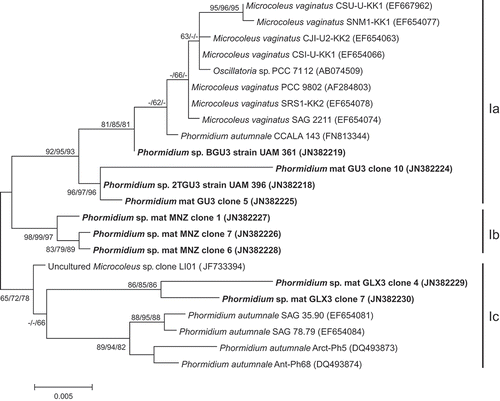
Ecological characteristics
There were differences in water quality between the sites from which the samples were collected, and differences in the mats were related to these characteristics. In order to study the relationships between the differential distribution of cyanobacteria and environmental variables, Canonical Correspondence Analysis (CCA) was carried out (Fig. 27). CCA revealed that the first two axes accounted for 40% of the variance (P = 0.006). The first axis was mainly defined by temperature, hardness, conductivity, SRP and DIN (intraset correlation coefficients: 1.0, 0.95, 0.89 and 0.72). The second axis was mainly correlated with DO and pH (intraset correlation coefficients: 1.0 and 0.64) and negatively correlated with DIN and SRP (intraset correlation coefficients: −0.96, −0.95). These results indicated a clear pollution gradient. Ordination of samples by CCA according to environmental parameters revealed a species distribution along axis (Fig. 27). CCA allowed elucidating the relationships between species (points in Fig. 27) and environmental parameters (arrows in Fig. 27). Therefore, the spread of data indicates a general gradient from high water quality in the upper part of the graph towards eutrophic or hypertrophic conditions in the lower part of the figure. Cyanobacteria of clade Ia (Phormidium mat from Guadarrama river: Phormidium sp. GU3) were located in the lower part of the graph, with higher nitrogen and phosphate values (hypertrophic conditions) while cyanobacteria of clades Ib and Ic were located in the middle (mesotrophic conditions) and low parts (eutrophic conditions), respectively. Cyanobacteria identified as P. terebriforme (clade II), O. tenuis (clade III) and Phormidium sp. (clade Ia) were also predominant at the Guadarrama sampling site with high nutrient content, and were plotted in the lower part of the graph. However, morphotypes–genotypes corresponding to clade IV identified as Phormidium corium, collected in the Guadalix river and Mediano stream, were placed at the upper part, corresponding to low levels of nutrients. Phormidium aerugineo-caeruleum, corresponding to clade V, ranged from oligotrophic to mesotrophic conditions.
Discussion
Relationships between phenotypic, genotypic and ecological characteristics
Special interest has been focused on the diversity of cyanobacteria in an ecological context, reconciling molecular and phenotypic characterizations (Golubic, Citation2010). Results from this study allowed us to distinguish distinct genotypes corresponding to different morphotypes, which in turn corresponded to different ecological characteristics. Thus, a clear relationship was found between the cyanobacterial species in the mats we analysed and the trophic status of the waters where they were collected. From the CCA results, a gradient was distinguishable that coincides with the trophic status of the running waters, assuming that N and P are the dominant nutrients controlling the trophic states of streams and rivers (Kelly & Whitton, Citation1998; Biggs, Citation2000). The spread of the data suggests a general progression from oligo- or mesotrophic water bodies (clear water, low soluble nutrient concentrations, and low algal growth productivity) to eutrophic or hypertrophic ones (increased soluble nutrient concentrations) and benthic algal proliferations. The distribution of the samples was mainly related to water quality. The rivers with the best water quality had the lowest temperatures, conductivities and low nutrient concentrations, while the eutrophic and hypertrophic sampling sites had higher values for these parameters. Cyanobacteria are organisms that are present in a variety of aquatic ecosystems, and their broad ecological distribution reflects a wide variety of species with different characteristics (Tandeau de Marsac & Houmard, Citation1993). In this cosmopolitan distribution, some cyanobacteria have certain ecological requirements that determine the presence, absence or abundance, which may reflect the characteristics of the water.
Morphotype–genotype I: the Phormidium autumnale/Microcoleus vaginatus complex
Clade I, in which our sequences corresponding to morphotype I were located, also included sequences from the database mainly belonging to Phormidium autumnale and Microcoleus vaginatus. These results are similar to those found in other studies (Comte et al., Citation2007; Marquardt & Palinska, Citation2007; Palinska & Marquardt, Citation2008; Siegesmund et al., Citation2008; Heath et al., Citation2010; Strunecký et al., Citation2010; Hašler et al., Citation2012) based on analyses from various geographical regions.
Phormidium autumnale is a taxon considered by many authors to be a very problematic entity and is sometimes referred to as the ‘P. autumnale group’ (Comte et al., Citation2007). Palinska & Marquardt (Citation2008) described this species as a frequent and truly ecologically amorphous taxon, which also has an unusually broad morphological species description. Strunecký et al. (Citation2010) discussed the species P. autumnale sensu lato, presenting a unique and enormous morphological diversity, in which several species (morphospecies and ecospecies) have been described based on a number of diagnostic features. They also discussed typical P. autumnale (P. autumnale sensu stricto), which occurs in the benthos of mesotrophic flowing waters in Central Europe. A list of morphospecies was reviewed by Komárek & Anagnostidis (Citation2005), who especially focused on the morphological ‘Phormidium group VII’ containing P. autumnale. Strunecký et al. (Citation2010) also pointed out that, in some collections, many strains are designated as ‘P. autumnale’, but these strains represent a wide spectrum of various ecotypes and are not all part of the morphological range of P. autumnale. Their morphological variability is very pronounced, depending on the conditions at different localities, and the morphological changes occurring in strains that have been cultured for a long time complicate the classification and understanding of the diversity of this group (Palinska & Marquardt, Citation2008; Strunecký et al., Citation2010). In fact, one of our cultures (strain BGU3), had clearly changed its morphology and dimensions after four years of culture (Figs 5, 6; Table 3), similar to the results of Strunecký et al. (Citation2010), who found that four cultivated strains had become distorted over several years of cultivation.
Genetic diversity in the P. autumnale cluster has also been described previously. Comte et al. (Citation2007), in a phenotypic analysis combined with some genetic analysis (two genotypic methods used in two polar strains), concluded that their strains belonged to the P. autumnale group but not to the same species. Palinska & Marquardt (Citation2008) concluded that their two morphologically similar P. autumnale groups, which shared <97% sequence homology, might be two different species of the same genus. Similar results were found by Heath et al. (Citation2010).
Strunecký et al. (Citation2010) pointed out that because the majority of cyanobacterial genotypes are both ecologically and morphologically restricted, an elucidation of the diversity of this cosmopolitan and widely distributed P. autumnale-complex was expected to be obtained using molecular markers such as 16S rDNA. Hašler et al. (Citation2012) analysed the 16S rRNA gene in 20 epipelic filamentous cyanobacteria and confirmed the relationship between P. autumnale and M. vaginatus previously recorded (Siegesmund et al., Citation2008), stating that the majority of authors consider the P. autumnale–M. vaginatus complex to be polyphyletic, although a high degree of morphological and genetic similarity between the two taxa was found. Palinska & Marquardt (Citation2008) also found very strong morphological similarities between P. autumnale and M. vaginatus. The trichome morphology in both species is almost identical (García-Pichel et al., 2001; Boyer et al., Citation2002; Komárek & Anagnostidis, Citation2005). The genus Microcoleus is characterized by the formation of large bundles of tightly interwoven trichomes, which are enclosed in a common gelatinous sheath (Geitler, Citation1932; Komárek & Anagnostidis, Citation2005). However, under laboratory culture conditions sheath formation may be lost, as has been shown in M. chthonoplastes (Garcia-Pichel et al., Citation1996) and M. vaginatus PCC 9802 (Garcia-Pichel, personal communication). The presence of an 11-bp insert in the variable region 6 (V6) of the 16S rRNA gene, which was described in strains of M. vaginatus (Baker et al., Citation2001; Garcia-Pichel et al., Citation2001), has also been found in strains of Phormidium from Antarctica (Nadeau et al., Citation2001; Casamatta et al., Citation2005, CitationTaton et al., 2006a; Strunecký et al., Citation2010) and European fresh waters (Rudi et al., Citation1998; Lokmer, Citation2007). Strunecký et al. (Citation2010) proposed recognition of a ‘Microcoleus cluster’ and pointed out that Phormidium representatives of this cluster, according to morphological features and the structure of trichomes, were more similar to Microcoleus than to other Phormidium species, especially with respect to the 11-bp insert, and suggested that they probably comprise a single generic unit. CitationHašler et al. (2012) proposed the distinction of M. vaginatus from P. autumnale on the basis of the presence of the 11 bp insert. In our study, when an in-depth phylogenetic analysis was carried out including only nearly complete sequences (Peplies et al., Citation2008; Tindall et al., Citation2010), our samples of the P. autumnale/M. vaginatus complex were clearly separated into three groups (Ia, Ib and Ic). The insert appears in two of these, not only in clade Ia but also in clade Ib, and the sequence similarity between them ranged between 97.3% and 98.7%. A threshold of 97.5% 16S rRNA gene sequence similarity has been suggested for separating prokaryotic species (Stackebrandt & Goebel, Citation1994) because, when two strains have genetic identity below this percentage, they consistently have DNA–DNA hybridization values below 70%, which has been used as a criterion for recognizing bacterial species (Wayne et al., Citation1987). The 16S rDNA threshold was subsequently raised to 98.7–99.0% by Stackebrandt & Ebers (Citation2006). In our case, whether the lower or higher threshold is used, the genetic divergences between subclades Ia, Ib and Ic indicate that these three comprise three different species.
From the morphological point of view, although representatives of morphotype I shared basic morphological characteristics, which are in accordance with classical descriptions of P. autumnale, ultrastructural differences were found between them. The most variable characteristic was the arrangement of the thylakoids. According to Komárek & Anagnostidis (Citation2005), Phormidium is characterized by radially oriented thylakoids, although this feature has not yet been demonstrated for most of the many species described from nature and culture studies (Marquardt & Palinska, Citation2007). In this study we were able to differentiate two types of arrangement, whose distribution was congruent with the 16S rRNA sequences. Our isolated strains of clade Ia, as well as other representatives of this clade (from other ultrastructural studies by Lokmer, Citation2007, and Palinska & Marquardt, Citation2008), showed the thylakoids to be mainly parallel to the longitudinal axis. By contrast, the Phormidium mat from Guadalix river, as well as other representatives of cluster Ic (studied by Marquardt & Palinska, Citation2007, and Palinska & Marquardt, Citation2008), had thylakoids with an irregular orientation, forming small clusters. The consistency between thylakoid arrangement and the results of the genetic analysis agrees with previous studies (Casamatta et al., Citation2005; Lokmer, Citation2007).
Cohan (Citation2011) has proposed the Ecotypic Species Concept, defining species as ‘ecotypes’ on the basis of their ecological niche, in which speciation occurs when a mutation or recombination event places a bacterium into a new ecological niche and creates a new ecotype. Following this concept, our representatives of clades Ia, Ib and Ic can be assigned to three ecotypes, and therefore again to three different species, in accordance with the molecular threshold approach (see above) and phenotypic differences. Thus, for example, our samples of clade Ia were characteristic of polluted waters such as the hypertrophic waters of the Guadarrama river, while those of clade Ib grew in mesotrophic conditions, and those of clade Ic in eutrophic conditions. Although specific ecological data are not generally available for most of the sequences obtained from GenBank, as previously pointed out by Dvořák et al. (2012) for the sequences retrieved from the GenBank in their study, another representative of clade Ia isolated from similar conditions was described as P. autumnale CCALA 143, which was collected from the wastewater of an oxidation pond (Palinska & Marquardt, Citation2008). Microcoleus vaginatus, which has been described as characteristic of hot deserts (Garcia-Pichel et al. Citation2001), is able to survive in habitats under environmental stresses, e.g. tolerance to desiccation and high levels of ultraviolet radiation, or nutrient limitation or excess. These results lead us to hypothesize that clade Ia may be characterized by the capacity to survive in extreme environments, as previously proposed for other strains (Crispim & Gaylarde, 2003; Casamatta et al., Citation2005). Casamatta et al. (Citation2005) found a close genetic relationship between Antarctic and desert Microcoleus strains, pointing out that this relationship was not incongruous since the main environmental stresses in both ecosystems were long periods of desiccation and high UV light levels.
Regarding clades Ib and Ic, although no other sequences were included in clade Ib, apart from our sequences of Phormidium mats from the mesotrophic Manzanares river, there are some indications, as stated above, that typical P. autumnale occurs in the benthos of flowing mesotrophic waters (Strunecký et al. Citation2010), which could correspond to our Phormidium mats. There are also some records about the possibility of pollution in the brook from which one strain of clade Ic (P. autumnale SAG 35.90) was isolated (Palinska & Marquardt, Citation2008), which would therefore agree with our findings, since the Guadalix river where the Phormidium mats of this cluster were found was eutrophic.
Morphotype–genotype II: Phormidium terebriforme
Morphotype–genotype II, assigned to Phormidium terebriforme, was observed in the mat from the Guadarrama and two isolated strains from this mat. It showed high similarity (99%) to sequences from previous studies corresponding to P. cf. terebriformis and P. pseudopriestleyi (Ballot et al., Citation2004; Taton et al., Citation2006b). This morphotype differed from the typical P. autumnale group mainly by its narrower trichomes, hooked terminal cells, and the absence of calyptra, and it was found in the polluted Guadarrama waters. Previous studies have described P. terebriforme as being a potential indicator of polluted waters (Kolkwitz & Marsson, Citation1908; Fjerdingstad, Citation1964; Sládecěk, Citation1973; Rott et al., Citation1997).
Morphotype–genotype III: Oscillatoria tenuis
Oscillatoria tenuis, which, like morphotype–genotype II, was found in the mat from the Guadarrama and two isolates from this mat, differs from the others by the presence of straight trichomes, with clear, narrowed cross-walls, the presence of necridic cells, and remarkable intensely pigmented hemispherical terminal cells. In the phylogenetic analysis, the two strains were grouped with 100% support with three Oscillatoria from databases. Oscillatoria tenuis has been included in the list of characteristic species of eutrophic waters in a new index based on benthic algae to assess the trophic status of Nordic rivers (Schneider & Lindstrøm, Citation2011), clearly in accord with the high levels of nutrients found in the Guadarrama location where it was found.
Morphotype–genotype IV: Phormidium corium
Morphotype–genotype IV, identified as Phormidium corium, was found in the cyanobacterial mats from Mediano stream and Guadalix river upstream, both with low levels of nutrients. The morphological characterization of this morphotype has been controversial, regarding the characteristics of thallus in environmental samples. Cantonati (Citation2008) found P. corium in the phytobenthos of streams in a National Park of northern Italy, where it had a membranaceous or leather-like thallus, while other researchers (Pfister, Citation1992; Pfister & Pipp, Citation2009; Gesierich & Kofler, Citation2010) described P. corium as an endolithic taxon appearing as light-blue spots on the lower sides of the stones. Backhaus (Citation2010) compared an endolithic species of Phormidium inhabiting the flowing waters of the upper Danube river-system with the microscopically similar P. corium, reaching the conclusion that the Phormidium found was a different species. He suggested that it is implausible that the cosmopolitan P. corium develops a totally different lifestyle as an endolith in the Black Forest streams, in contrast to its usual behaviour as a surface-living form. In our study, P. corium formed leathery-looking mats on the stones and was never found as endolithic spots. In 16S rDNA analyses, both environmental samples grouped with Phormidium murrayi and Microcoleus glaciei (formerly P. murrayi) in the phylogenetic tree, but in different branches. Previous published studies found a similarly separated Phormidium murrayi clade in phylogenetic analyses, principally from Antarctic habitats (Casamatta et al., Citation2005; Taton et al., Citation2006b; Comte et al., Citation2007; Strunecký et al., Citation2011) but also from benthic freshwater mats in New Zealand (Heath et al., Citation2010); the clade was also found in a revision of the genus Microcoleus (Siegesmund et al., Citation2008). Casamatta et al. (Citation2005) reassigned P. murrayi to M. glaciei on the basis of phylogenetic and microscopical analyses and Comte et al. (Citation2007) confirmed the separation of P. murrayi from typical Phormidium species (e.g. the P. autumnale group) and advocated a revision of Phormidium and Microcoleus taxa. Recently, Strunecký et al. (Citation2011) proposed the new genus Wilmottia, with the type species Wilmottia murrayi. Considering that this cyanobacterium was originally described as Lyngbya murrayi, and has subsequently been designated as Phormidium murrayi (Anagnostidis & Komárek, Citation1988), Microcoleus glaciei (Casamatta et al., Citation2005) and Wilmottia murrayi (Strunecký et al., Citation2011), this is clearly a problematic taxon in need of further research. We identified our morphotypes as P. corium on the basis of their separation from ‘P. murrayi’ on different branches, and the 97.0–98.2% similarities of our sequences to representatives of ‘P. murrayi’, below the threshold for species separation of ≥ 98.7–99% (Stackebrandt & Ebers, Citation2006). Published studies of the ecological characteristics of P. corium describe its occurrence in oligo- to mesotrophic conditions (Rott et al., Citation1997; Gutowski et al., Citation2004; Cantonati, Citation2008; Gesierich & Kofler, Citation2010), consistent with the characteristics of the waters from which our samples were collected.
Morphotype–genotype V: Phormidium aerugineo-caeruleum
Phormidium aerugineo-caeruleum (formerly Lyngbya aerugineo-coerulea) from the Mediano stream and the Manzanares river (morphotype–genotype V) had long trichomes with a prominent sheath and granular cell contents. In the phylogenetic tree it grouped with Microcoleus paludosus, but they shared <95% sequence homology. Since this is below the 95% threshold, this implies that they belong to different genera (Ludwig et al., Citation1998; Tindall et al., Citation2010) and this conclusion is corroborated by the morphological differences between them. The rivers where they were found were oligo- to mesotrophic, consistent with the previous occurrence of this cyanobacterium in clean waters (Charlton & Hickman, Citation1984; Dell'Uomo, Citation1991; Branco & Pereira, Citation2002; Serrano et al., Citation2004; Perona & Mateo, Citation2006).
Taxonomic implications
Results from this study indicate that some strains and environmental samples of Phormidium-like cyanobacteria represent distinct generic entities (e.g. clades IV and V), while in some clades (e.g. morphotype–genotype I), subgroups represent distinct species, for which we could propose new epithets. The taxonomy of cyanobacteria is in discussion and debate, and a comprehensive revision is urgently needed. Any treatise on cyanobacterial diversity is hampered by the poor state of cyanobacterial taxonomy and nomenclature. Different synonyms tend to be used by different authors for what may be the same morphotype, while on the other hand, what may be referred to in different publications as the same genus and species may encompass a number of different organisms (Oren, Citation2012). During the past 20 years, the great development of molecular biology has allowed an increasing number of polyphasic studies combining phenotypic and genotypic characterizations. Initially these were based on sequences of one particular gene, principally that coding for the small subunit of the ribosome, but studies increasingly combine information from several genes (Whitton & Potts, Citation2012). More detailed genomic analysis has been claimed to provide a much fuller assessment of species relationships, which is necessary before further drastic taxonomic revisions are undertaken. As pointed out by Whitton & Potts (Citation2012), nomenclatural changes have been introduced too rapidly and this will inevitably lead to further revisions in a few years’ time, so that proposals to revise generic limits should be deferred until data for a sufficient number of strains are available. We feel, as previously suggested by Marquardt & Palinska (Citation2007), that more phenotypic and molecular data from additional organisms and multiple genes are needed to confirm and refine the systematic relationships. In addition, ecological characteristics of cyanobacterial populations should also be important traits in taxonomic studies. Studies on the diversity of cyanobacteria in an ecological context contribute to knowledge concerning the identity of the taxa and their phyletic relationships. Accurate identification of cyanobacteria requires information on their ecological characteristics, which can be used as important diagnostic indicators. A starting point would then be the detection of ‘ecotypes’, where genotypic and phenotypic clusters correspond, but for that we need the characterization of ecological niches and their boundaries. In our study we have found correlations between natural phenotypes and ecotypes and their genetic background by matching molecular, phenotypic and ecological traits. Unfortunately, despite the roles of cyanobacteria as environmentally important entities, the ecological range is unknown for many species, as is the effect of variation in environmental factors, such as increases in nutrient loading. These data are essential for interpreting biotic interactions and the structure and composition of cyanobacterial communities in different habitats, as well as predicting possible changes caused by climate change and other anthropogenic pressures.
Supplementary material
Download (126.3 KB)Acknowledgements
This work was supported by grants from the Ministerio de Educación y Ciencia, Spain (CGL2008-02397/BOS), from the Comunidad Autónoma de Madrid, Spain (S2009/AMB-1511) and AECID (A/016417/08). We thank Stjepko Golubic for stimulating discussions and valuable suggestions, which improved the manuscript.
References
- Anagnostidis , K. and Komárek , J. 1988 . Modern approach to the classification system of cyanophytes. 3. Oscillatoriales . Algological Studies , 50–53 : 327 – 472 .
- APHA . 1992 . Standard methods for the examination of waters and wastewater , 18th , Washington, DC : American Public Health Asssociation .
- Ashelford , K.E. , Chuzhanova , N.A. , Fry , J.C. , Jones , A.J. and Weightman , A.J. 2006 . New screening software shows that most recent large 16S rRNA gene clone libraries contain chimeras . Applied and Environmental Microbiology , 72 : 5734 – 5741 .
- Backhaus , D. 2010 . Phormidium ingrediens sp. nova (Cyanobacteria, Oscillatoriales), a limestone-boring euendolithic inhabitant of flowing waters Vol. 68 , 15 – 25 . Carolinea
- Baker , P.D. , Steffensen , D.A. , Humpage , A.R. , Nicholson , B.C. , Falconer , I.R. , Lanthois , B. , Fergusson , K.M. and Saint , C.P. 2001 . Preliminary evidence of toxicity associated with the benthic cyanobacterium . Phormidium in South Australia. Environmental Toxicology , 16 : 506 – 511 .
- Ballot , A. , Dadheech , P.K. and Krienitz , L. 2004 . Phylogenetic relationship of . Arthrospira, Phormidium and Spirulina strains. Algological Studies , 113 : 37 – 56 .
- Biggs , B.J.F. 2000 . Eutrophication of streams and rivers: dissolved nutrient-chlorophyll relationships for benthic algae . Journal of the North American Benthological Society , 19 : 17 – 31 .
- Boyer , S.L. , Johansen , J.R. , Flechtner , V.R. and Howard , G.L. 2002 . Phylogeny and genetic variance in terrestrial . Microcoleus (Cyanophyceae) species based on sequence analysis of the 16S rRNA gene and associated 16S–23S ITS region. Journal of Phycology , 38 : 1222 – 1235 .
- Branco , L.H.Z. and Pereira , J.L. 2002 . Evaluation of seasonal dynamics and bioindication potential of macroalgal communities in a polluted tropical stream . Archiv für Hydrobiologie , 155 : 147 – 161 .
- Cantonati , M. 2008 . Cyanoprokaryotes and algae other than diatoms in springs and streams of the Dolomiti Bellunesi National Park (Northern Italy . Algological Studies , 126 : 113 – 136 .
- Carmona , J. , Perona , E. , Sánchez-Díaz , E. and Loza , V. 2011 . Morphological and ecological characterization of Batrachospermales (Rhodophyta) in the Jarama Basin, Iberian Peninsula . Limnetica , 30 : 117 – 128 .
- Casamatta , D.A. , Johansen , J.R. , Vis , M.L. and Broadwater , S.T. 2005 . Molecular and morphological characterization of ten polar and near-polar strains within the . Oscillatoriales (cyanobacteria). Journal of Phycology , 41 : 421 – 438 .
- Castenholz , R.W. and Waterbury , J.B. 1989 . “ Group I. Cyanobacteria ” . In Bergey's manual of systematic bacteriology , Edited by: Holt , J.G. vol. 3 , 1710 – 1727 . Baltimore, MD : Williams & Wilkins .
- Castenholz , R.W. , Rippka , R. , Herdman , M. and Wilmotte , A. 2001 . “ Subsection III. (Formerly Oscillatoriales Elenkin 1934) ” . In Bergey's manual of systematic bacteriology , 2nd , Edited by: Boone , D.R. , Castenholz , R.W. and Garrity , G.M. 539 – 562 . New York : Springer .
- Charlton , S.E.D. and Hickman , M. 1984 . Seasonal physical, chemical and algal changes in five rivers flowing through the oil sands region of Alberta, Canada . Internationale Revue der gesamten Hydrobiologie und Hydrographie , 69 : 297 – 332 .
- Cohan , F.M. 2011 . “ Are species cohesive? A view from bacteriology ” . In Population genetics of bacteria: a tribute to Thomas S. Whittam , Edited by: Walk , S.T. and Feng , P.C.H. 43 – 65 . Washington, DC : ASM Press .
- Comte , K. , Sabacká , M. , Carré-Mlouka , A. , Elster , J. and Komárek , J. 2007 . Relationships between the Arctic and Antarctic cyanobacteria; three . Phormidium-like strains evaluated by a polyphasic approach. FEMS Microbiology Ecology , 59 : 366 – 376 .
- Crispim , C.A. and Gaylarde , C.C. 2005 . Cyanobacteria and biodeterioration of cultural heritage: a review . Microbial Ecology , 49 : 1 – 9 .
- Dell'Uomo , A. 1991 . “ Use of benthic macroalgae for monitoring rivers in Italy ” . In Use of algae for monitoring rivers , Edited by: Whitton , B.A. , Rott , E. and Friedrich , G. 129 – 138 . Universität Innsbruck, Innsbruck : Institut für Botanik .
- Desikachary , T.V. 1959 . Cyanophyta , New Delhi : Indian Council of Agricultural Research .
- Douterelo , I. , Perona , E. and Mateo , P. 2004 . Use of cyanobacteria to assess water quality in running waters . Environmental Pollution , 127 : 377 – 384 .
- Dvořák , P. , Hašler , P. and Poulíčkova , A. 2012 . Phylogeography of the Microcoleus vaginatus (Cyanobacteria) from three continents. A spatial and temporal characterization. PLoS ONE, 7: e40153
- Edwards , U. , Rogall , T. , Blöcker , H. , Emde , M. and Böttger , E.C. 1989 . Isolation and direct complete nucleotide determination of entire genes. Characterisation of a gene coding for 16S ribosomal RNA . Nucleic Acids Research , 17 : 7843 – 7853 .
- Felsenstein , J. 1985 . Confidence limits on phylogenies: an approach using the bootstrap . Evolution , 39 : 783 – 791 .
- Fjerdingstad , E. 1964 . Pollution of streams estimated by benthal phytomicro-organisms I. A saprobic system based on communities of organisms and ecological factors . Internationale Revue der gesamten Hydrobiologie und Hydrographie , 49 : 63 – 131 .
- Garcia-Pichel , F. , Prufert-Bebout , L. and Muyzer , G. 1996 . Phenotypic and phylogenetic analyses show . Microcoleus chthonoplastes to be a cosmopolitan cyanobacterium. Applied and Environmental Microbiology , 62 : 3284 – 3291 .
- Garcia-Pichel , F. , Lopez-Cortes , A. and Nübel , U. 2001 . Phylogenetic and morphological diversity of cyanobacteria in soil desert crusts from the Colorado Plateau . Applied and Environmental Microbiology , 67 : 1902 – 1910 .
- Geitler , L. 1932 . Cyanophyceae. In Dr L. Rabenhorst’s Kryptogamen–Flora von Deutschland, Österreich und der Schweiz , vol. 14 , Leipzig : Akademische Verlagsgesellschaft .
- Gesierich , D. and Kofler , W. 2010 . Are algal communities from near-natural rheocrene springs in the Eastern Alps (Vorarlberg, Austria) useful ecological indicators? . Algological Studies , 133 : 1 – 28 .
- Gkelis , S. , Rajaniemi , E. , Vardaka , M. , Moustaka-Gouni , M. , Lanaras , T. and Sivonen , K. 2005 . Van Goor) Meffert (Cyanobacteria) strains from lake Kastoria, Greece from a separate phylogenetic group. Microbial Ecology . Limnothrix redekei , 49 : 176 – 182 .
- Golubic , S. 2010 . Encounters with greater bacteria . Periodicum Biologorum , 112 : 227 – 238 .
- Gomont , M. 1892 . Monographie des Oscillariées (Nostocaceae homocystées . Annales des Sciences Naturelles, Série Botanique , 15 : 265 – 368 .
- Gutowski , A. , Foerster , J. and Schaumburg , J. 2004 . The use of benthic algae, excluding diatoms and Charales, for the assessment of the ecological status of running fresh waters: a case history from Germany . Oceanological and Hydrobiological Studies , 33 : 3 – 15 .
- Hall , T.A. 1999 . Bioedit: a user-friendly biological sequence alignment editor and analysis program for Windows 95/98/NT . Nucleic Acids Symposium Series , 41 : 95 – 98 .
- Hašler , P. , Dvořák , P. , Johansen , J.R. , Kitner , M. , Ondřej , V. and Poulíčkova , A. 2012 . Morphological and molecular study of epipelic filamentous genera . Phormidium, Microcoleus and Geitlerinema (Oscillatoriales, Cyanophyta/Cyanobacteria). Fottea , 12 : 341 – 356 .
- Heath , M.W. , Wood , S.A. and Ryan , K.G. 2010 . Polyphasic assessment of fresh-water benthic mat-forming cyanobacteria isolated from New Zealand . FEMS Microbiology Ecology , 73 : 95 – 109 .
- Kelly , M.G. and Whitton , B.A. 1998 . Biological monitoring of eutrophication in rivers . Hydrobiologia , 384 : 55 – 67 .
- Kolkwitz , R. and Marsson , M. 1908 . Ökologie der pflanzliche Saprobien . Berichte der Deutschen Botanischen Gesellschaft , 26 : 505 – 519 .
- Komárek , J. and Anagnostidis , K. 2005 . “ Cyanoprokaryota. 2. Teil: Oscillatoriales ” . In Süsswasserflora von Mitteleuropa , Edited by: Büdel , B. , Krienitz , L. , Gärtner , G. and Schagerl , M. vol. 19/2 , Heidelberg : Elsevier Spektrum .
- Lepère , C. , Wilmotte. , A. and Meyer , B. 2000 . Molecular diversity of Microcystis strains (Cyanophyceae, Chroococcales) based on 16S rDNA sequences . Systematics and Geography of Plants , 70 : 275 – 283 .
- Lokmer , A. 2007 . Polyphasic approach to the taxonomy of the selected oscillatorian strains (Cyanobacteria) , České Budějovice : MSc. Thesis. Faculty of Biological Sciences. University of South Bohemia .
- Ludwig , W. , Strunk , O. , Klugbauer , S. , Klugbauer , N. , Weizenegger , M. , Neumaier , J. , Bachleither , M. and Schleifer , K.H. 1998 . Bacterial phylogeny based on comparative sequence analysis . Electrophoresis , 19 : 554 – 568 .
- Marquardt , J. and Palinska , K.A. 2007 . Genotypic and phenotypic diversity of cyanobacteria assigned to the genus Phormidium (Oscillatoriales) from different habitats and geographical sites . Archives of Microbiology , 187 : 397 – 413 .
- Mateo , P. , Berrendero , E. , Perona , E. , Loza , V. and Whitton , B.A. 2010 . Phosphatase activities of cyanobacteria as indicators of nutrient status in a Pyrenees river . Hydrobiologia , 652 : 255 – 268 .
- Mateo , P. , Perona , E. , Berrendero , E. , Leganés , F. , Martín , M. and Golubić , S. 2011 . Life cycle as a stable trait in the evaluation of diversity of Nostoc from biofilms in rivers . FEMS Microbiology Ecology , 76 : 185 – 198 .
- Nadeau , T.L. , Milbrandt , E.C. and Castenholz , R.W. 2001 . Evolutionary relationships of cultivated Antarctic oscillatorians (Cyanobacteria . Journal of Phycology , 37 : 650 – 654 .
- Oren , A. 2012 . “ Salts and brines ” . In The ecology of cyanobacteria II. Their diversity in space and time , Edited by: Whitton , B.A. 401 – 426 . Dordrecht : Springer .
- Palinska , K.A. and Marquardt , J. 2008 . Genotypic and phenotypic analysis of strains assigned to the widespread cyanobacterial morphospecies Phormidium autumnale (Oscillatoriales) . Archives of Microbiology , 189 : 325 – 335 .
- Palinska , K.A. , Deventer , B. , Hariri , K. and Lotocka , M. 2011 . A taxonomic study on Phormidium-group (cyanobacteria) based on morphology, pigments, RAPD molecular markers and RFLP analysis of the 16S rRNA gene fragment . Fottea , 11 : 41 – 55 .
- Peplies , J. , Kottmann , R. , Ludwig , W. and Glöckner , F.O. 2008 . A standard operating procedure for phylogenetic inference (SOPPI) using (rRNA) marker genes . Systematic and Applied Microbiology , 31 : 251 – 257 .
- Perona , E. and Mateo , P. 2006 . Benthic cyanobacterial assemblages as indicators of nutrient enrichment regimes in a Spanish river . Acta Hydrochimica et Hydrobiologica , 34 : 67 – 72 .
- Perona , E. , Bonilla , I. and Mateo , P. 1999 . Spatial and temporal changes in water quality in a Spanish river . Science of the Total Environment , 241 : 75 – 90 .
- Pfister , P. 1992 . Artenspektrum des Algenaufwuchses in 2 Tiroler Bergbachen – Teil 1: Cyanophyceae, Chrysophyceae, Chlorophyceae, Rhodophyceae . Algological Studies , 65 : 43 – 61 .
- Pfister, P. & Pipp, E. (2009). Leitfaden zur Erhebung der biologischen Qualitätselemente. Teil A3 Phytobenthos. BMLF, Wien. http://wisa.lebensministerium.at/ (http://wisa.lebensministerium.at/)
- Reynolds , S. 1963 . The use of lead citrate at high pH as an electron-opaque stain in electron microscopy . Journal of Cell Biology , 17 : 200 – 11 .
- Rippka , R. , Deruelles , J. , Waterbury , J.B. , Herdman , M. and Stanier , R.Y. 1979 . Generic assignments, strain histories, and properties of pure cultures of cyanobacteria . Journal of General Microbiology , 111 : 1 – 61 .
- Rodríguez , V. , Aguirre de Cárcer , D. , Loza , V. , Perona , E. and Mateo , P. 2007 . A molecular fingerprint technique to detect pollution-related changes in river cyanobacterial diversity . Journal of Environmental Quality , 36 : 464 – 468 .
- Rott , E. , Hofmann , G. , Pall , K. , Pfister , P. and Pipp , E. 1997 . Indikationslisten für Aufwuchsalgen in Österreichischen Fliessgewässern. Teil 1: Saprobielle Indikation , Austria : Bundesministerium für Land- und Forstwirtschaft .
- Rudi , K. , Skulberg , O.M. and Jakobsen , K.S. 1998 . Evolution of cyanobacteria by exchange of genetic material among phyletically related strains . Journal of Bacteriology , 180 : 3453 – 3461 .
- Sciuto , K. , Andreoli , C. , Rascio , N. , La Rocca , N. and Moro , I. 2012 . Polyphasic approach and typification of selected Phormidium strains (Cyanobacteria) . Cladistics , 28 : 357 – 374 .
- Schneider , S.C. and Lindstrøm , E.A. 2011 . The periphyton index of trophic status PIT: a new eutrophication metric based on non-diatomaceous benthic algae in Nordic rivers . Hydrobiologia , 665 : 143 – 155 .
- Serrano , A. , Mateo , P. and Perona , E. 2004 . Estructura y composición de cianobacterias bentónicas de un arroyo de montaña mediterráneo, el arroyo Mediano (Madrid . Limnetica , 23 : 85 – 96 .
- Siegesmund , M.A. , Johansen , J.R. , Karsten , U. and Friedl , T. 2008 . Coleofasciculus gen. nov. (Cyanobacteria): morphological and molecular criteria for revision of the genus Microcoleus Gomont . Journal of Phycology , 44 : 1572 – 1585 .
- Sládecěk , V. 1973 . System of water quality from the biological point of view . Archiv für Hydrobiologie , 7 : 1 – 218 .
- Spurr , A.S. 1969 . A low-viscosity epoxy resin embedding medium for electron microscopy . Journal of Ultrastructure Research , 26 : 31 – 43 .
- Stackebrandt , E. and Ebers , J. 2006 . Microbiology today. Taxonomic parameters revisited: tarnished gold standards . Microbiology Today , 33 : 152 – 155 .
- Stackebrandt , E. and Goebel , B.M. 1994 . Taxonomic note: a place for DNA–DNA reassociation and 16S rRNA sequence analysis in the present species definition in bacteriology . International Journal of Systematic Bacteriology , 44 : 846 – 849 .
- Strunecký , O. , Elster , J. and Komárek , J. 2010 . Phylogenetic relationships between geographically separate Phormidium cyanobacteria: is there a link between north and south polar regions? . Polar Biology , 33 : 1419 – 1428 .
- Strunecký , O. , Elster , J. and Komárek , J. 2011 . Taxonomic revision of the freshwater cyanobacterium . “Phormidium” murrayi = Wilmottia murrayi. Fottea , 11 : 57 – 71 .
- Tajima , F. and Nei , M. 1984 . Estimation of evolutionary distance between nucleotide sequences . Molecular Biology and Evolution , 1 : 269 – 285 .
- Tamura , K. , Peterson , D. , Peterson , N. , Stecher , G. , Nei , M. and Kumar , S. 2011 . MEGA5: Molecular evolutionary genetics analysis using maximum likelihood, evolutionary distance, and maximum parsimony methods . Molecular Biology and Evolution , 28 : 2731 – 2739 .
- Tandeau de Marsac , N. and Houmard , J. 1993 . Adaptation of cyanobacteria to environmental stimuli: new steps towards molecular mechanisms . FEMS Microbiology Reviews , 104 : 119 – 190 .
- Taton , A. , Grubisic , S. , Balthasart , P. , Hodgson , D.A. , Laybourn-Parry , J. and Wilmotte , A. 2006a . Biogeographical distribution and ecological ranges of benthic cyanobacteria in East Antarctic lakes . FEMS Microbiology Ecology , 57 : 272 – 289 .
- Taton , A. , Grubisic , S. , Ertz , D. , Hodgson , D.A. , Piccardi , R. , Biondi , N. , Tredici , M.R. , Mainini , M. , Losi , D. , Marinelli , F. and Wilmotte , A. 2006b . Polyphasic study of Antarctic cyanobacterial strains . Journal of Phycology , 42 : 1257 – 1270 .
- Ter Braak , C.J.F. and milauer , P. 2002 . CANOCO reference manual and Cano-Draw for Windows user´s guide: software for canonical community ordination (version 4.5) , Ithaca, NY : Microcomputer Power .
- Thompson , J.D. , Higgins , D.G. and Gibson , T.J. 1994 . CLUSTAL W: Improving the sensitivity of progressive multiple sequence alignment through sequence weighting positions-specific gap penalties and weight matrix choice . Nucleic Acids Research , 22 : 4673 – 4680 .
- Tindall , B.J. , Rosselló-Móra , R. , Busse , H.J. , Ludwig , W. and Kämpfer , P. 2010 . Notes on the characterization of prokaryote strains for taxonomic purposes . International Journal of Systematic and Evolutionary Microbiology , 60 : 249 – 266 .
- Urbach , E. , Robertson , D. and Chisholm , S.W. 1992 . Multiple evolutionary origins of prochlorophytes within the cyanobacterial radiation . Nature , 355 : 267 – 269 .
- Wayne , L.G. , Brenner , D.J. , Colwell , R.R. , Grimont , P.A.D. , Kandler , O. , Krichevsky , M.I. , Moore , L.H. , Moore , W.E.C. , Murray , R.G.E. , Stackebrandt , E. , Starr , M.P. and Truper , H.G. 1987 . International Committee on Systematic Bacteriology. Report of the ad hoc committee on reconciliation of approaches to bacterial systematics . International Journal of Systematic Bacteriology , 37 : 463 – 464 .
- Whitton , B.A. 2011 . “ Phylum Cyanobacteria ” . In The freshwater algal flora of the British Isles. An identification guide to freshwater and terrestrial algae , 2nd , Edited by: John , D.M. , Whitton , B.A. and Brook , A.J. 31 – 158 . Cambridge : Cambridge University Press .
- Whitton , B.A. and Potts , M. 2000 . The ecology of cyanobacteria, their diversity in time and space , Berlin : Springer .
- Whitton , B.A. and Potts , M. 2012 . “ Introduction to the Cyanobacteria ” . In The ecology of cyanobacteria II. Their diversity in space and time , Edited by: Whitton , B.A. 1 – 13 . Dordrecht : Springer .
Supplementary information
The following supplementary material is available for this article, accessible via the Supplementary Content tab on the article's online page at http://dx.doi.org/10.1080/09670262.2013.799715
Supplementary file. Alignment of 16S rDNA sequences