Abstract
This paper describes a method to study the diversity of young kelp sporophytes that are recruited from the bank of microscopic stages. Small samples of rocky substratum (0.5 cm2) were collected from the low intertidal zone, which was dominated by the kelp Laminaria digitata. Samples were cultivated in the laboratory under conditions permitting gametogenesis. Sporophyte recruits in the cultures were isolated and identified at the species level using the barcoding mitochondrial marker rpl31–rns. Sixty per cent of the collected samples had at least one to a maximum of 30 kelp recruits, belonging to five different species (L. digitata, L. hyperborea, L. ochroleuca, Saccharina latissima and Sacchorhiza polyschides). As the examination of freshly collected rocky samples under a stereo microscope did not reveal any kelp sporophytes, the recruitment in these samples after culture probably occurred from the bank of microscopic forms present on the substratum. Despite the dominance of L. digitata in the field, the young sporophytes obtained after culturing were mainly S. polyschides. This study illustrates the suitability of culturing in combination with molecular identification of young sporophytes to address several key aspects of kelp ecology related to the existence of a bank of microscopic stages in the field.
Introduction
In the last decade, DNA barcoding has provided rapid and accurate species identification of unidentified and/or microscopic organisms (Pawlowski et al., Citation2012). On the other hand, culturing natural substrata is a long-standing technique used to study the resting stages of organisms. The seedling emergence method is one of the best known examples of this, where the soil seed bank is estimated through the germination of soil samples (Roberts, Citation1981). This technique has mainly been employed in terrestrial environments, whereas there are far fewer studies that have investigated seed-bank dynamics in aquatic habitats or wetlands (reviewed by McFarland & Shafer, Citation2011).
In the marine environment, the existence of a seed bank analogue formed by microscopic life-stages has been described for a variety of seaweeds (e.g. Chapman, Citation1986; Hoffmann & Santelices, Citation1991). Potentially, therefore, methods of rocky substratum culturing could be employed to study the ecological role of seed bank analogues in seaweeds, just as has been done in land-plant ecology. However, surprisingly few studies have used this methodology to investigate the biodiversity of microscopic stages of seaweeds on rocky shores (e.g. Müller & Ramírez, Citation1994; Santelices et al., Citation1995). One reason may be the inherent difficulty of identifying early-settlement stages based on morphological criteria. However, molecular systematics can provide efficient tools for identification of small specimens of seaweeds (e.g. Clayden & Saunders, Citation2010; Manghisi et al., Citation2010), including microscopic stages of kelps (Lane & Saunders, Citation2005; Fox & Swanson, Citation2007). Nevertheless, to our knowledge, none of these studies has combined rocky substratum culturing with subsequent molecular identification of recruits.
The lack of seaweed studies using a combination of rocky substratum culturing and barcoding might be due to several factors. First, the establishment of optimal culture conditions for successful germination of heterogeneous macroalgal recruits is challenging. Moreover, early post-settlement stages of macroalgae are sensitive to a variety of grazers and competitors (see Vadas et al., Citation1992 and references therein). It is therefore essential to sample pieces of rocky substratum small enough to prevent their negative impact. Finally, culturing natural substrata might seem outdated when compared with the fast-emerging field of DNA metabarcoding using the next-generation sequencing technologies (Taberlet et al., Citation2012). However, the design of metabarcode markers suitable for DNA amplification from environmental samples remains problematic (Taberlet et al., Citation2012), whereas classical barcode markers for a variety of seaweeds, as well as a comprehensive library of sequences, are available and efficient in species identification (e.g. Engel et al., Citation2008; Saunders & McDevit, Citation2012). In addition, substratum culturing and, to a lesser extent, Sanger-based barcoding, are economically accessible for the majority of laboratories.
In this study, we developed methods for combining substratum culturing with molecular identification in order to investigate the diversity of the kelp bank of microscopic stages (gametophytes or embryonic sporophytes). Toward this goal, we sampled natural rocky substrata from plots located in the low intertidal zone dominated by the kelp Laminaria digitata. We subsequently cultured these sampled substrata in the laboratory and determined the identity of each young sporophyte that recruits from the bank of microscopic forms to the species-level using a barcoding approach.
Materials and methods
The study was conducted in the low intertidal zone of the Perharidy peninsula (48.73058° N, 4.00366° W) to the west of Roscoff, on the northwest coast of Brittany, France. The study area covered approximately 1200 m2 (46 m long by 26 m wide) and was dominated by Laminaria digitata (about 30 sporophytes m−2) even though a visual survey revealed the presence of three other kelp species at low density (less than one sporophyte m−2): L. hyperborea, Saccorhiza polyschides and Saccharina latissima. In order to identify the kelps constituting the bank of microscopic stages present, we haphazardly sampled on 21 March 2011 one piece of substratum (about 0.5 cm2) from each of 30 plots regularly placed 4.5 m apart from each other, using a hammer and chisel. Sampled substrata were placed in separate plastic bags with seawater and brought back to the laboratory, where we examined them under the stereo microscope for the presence of small kelp sporophytes. The substrata were cultivated separately in 30 ml Petri dishes in half-strength Provasoli-enriched autoclaved seawater (Starr & Zeikus, Citation1993) at 10–15°C and natural daylight (north-facing roof window). The medium was not changed during incubation.
After 50 days of culture, substratum samples were checked carefully under the stereo microscope and the number of kelp recruits per dish was recorded. With the help of dissecting forceps, each recruit was detached from its original substratum and transferred to a Petri dish with new culture medium. When they reached appropriate size for DNA extraction (1–20 mm length), all the recruits were harvested and preserved (−20°C) for molecular analysis.
Total DNA was extracted using ChelexTM 100 resin (Biorad, Hercules, California, USA) according to the method described by Goff & Moon (Citation1993). In order to determine the taxonomic identity of kelp recruits, we amplified c. 300 bp of the mitochondrial intergenic spacer rpl31–rns using the primers described by Engel et al. (Citation2008). PCR reactions were performed in a total volume of 20 μl containing 0.5 µM of each primer, 150 µM of each dNTP, 2 mM of MgCl2, 1 × GoTaq® Flexibuffer (Promega, Madison, Wisconsin, USA), 0.35 units of GoTaq® FlexiDNA polymerase (Promega) and 4 μl of 1 : 50 diluted template DNA. The PCR reaction was carried out in a PTC-100 thermocycler (Bio-Rad Laboratories, Hercules, California, USA). Cycling conditions consisted of an initial denaturing step of 5 min at 95°C, followed by 5 cycles of a touchdown PCR (95°C for 30 s, 60°C for 45 s and 72°C for 45 s; 1°C decrease of the annealing temperature every cycle), 30 additional cycles (95°C for 30 s, 55°C for 45 s, and 72°C for 45 s) and a final elongation step of 10 min at 72°C. The presence of PCR-amplified products was checked using agarose gels. PCR products were purified and sequenced with both forward and reverse primers at Genoscope facilities (Evry, France). Chromatograms were edited using ChromasPro (www.technelysium.com.au) and only traces with high quality values and no ambiguities were used. To identify species, sequences were compared with those deposited in Genbank using the basic local alignment search tool (BLAST) from NCBI (Altschul et al., Citation1990).
Results
There were no kelp sporophytes or gametophytes visible on the 30 substratum samples immediately after their collection. However, after 50 days of culture, there were 108 thalli resembling kelp recruits in 20 of the 30 samples. These were isolated, grown further and identified with molecular tools, resulting in a total of 86 kelp recruits distributed among 18 substratum samples. The remaining 22 putative kelp recruits were either kelp species for which DNA extraction or PCR did not work or were not kelps.
The number of recruits per sample was highly variable, ranging from zero to 30 young kelps grouped on the same 0.5 cm2 size bedrock sample (mean ± SE: 2.87 ± 1.07). While eight out of the 18 samples containing kelp recruits had a single individual, four showed a number of recruits varying from two to five, and five had a number of recruits between six and nine. One sample had 30 recruits ().
Fig. 1. Distribution of the numbers of recruits observed on samples of natural rock substrata (N = 30).
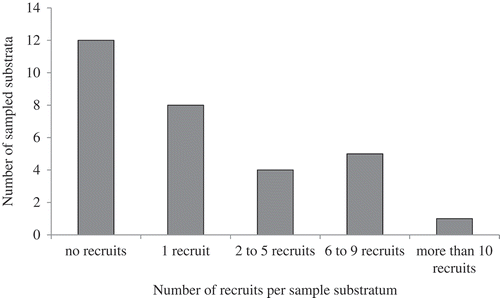
The kelp recruits belonged to five different species: Saccorhiza polyschides, Saccharina latissima, Laminaria digitata, L. hyperborea and L. ochroleuca (). They corresponded to eight distinct haplotypes (). Saccorhiza polyschides was the dominant species in our samples (67 individuals, 77.9%) and exhibited three haplotypes, while L. digitata represented only 9.3% of the recruits (8 individuals) and had a single haplotype. Five S. latissima and five L. hyperborea recruits (5.8%) were identified. A single haplotype was found for L. hyperborea, whereas two were found for S. latissima. One recruit was identified as L. ochroleuca despite the fact we did not detect this species in the close surrounding intertidal area. In the 10 samples exhibiting more than one recruit, six contained a single species while the remaining four displayed at least two different species. Five exhibited one haplotype while the other five had several haplotypes for at least one species ().
Fig. 2. Species composition of the 86 kelp recruits observed over the 30 sampled substrata; Ld = Laminaria digitata, Lh = Laminaria hyperborea, Lo = Laminaria ochroleuca, Sl = Saccharina latissima, Sp = Saccorhiza polyschides.
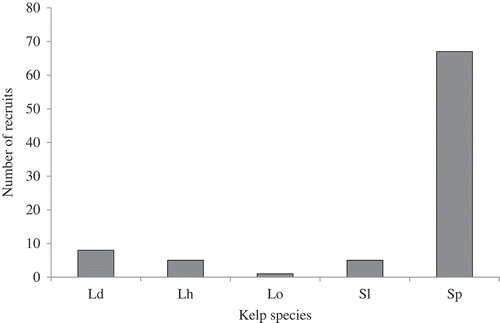
Table 1. Number and haplotype identity for the 18 samples (out of 30) exhibiting recruits. Samples are numbered S1 to S30. GenBank accession numbers are KC337288 for HSp1, KC337289 for HSp2, KC337290 for HSp3, KC337283 for HLd1, KC337286 for HSl1, KC337287 for HSl2, KC337284 for HLh1 and KC337285 for HLo1.
Discussion
In this study, we successfully cultured and identified kelp recruits from natural substratum samples of very small size (0.5 cm2). Our method combining substratum culturing and molecular identification of recruits revealed that some of these samples exhibited both different kelp species and different haplotypes within species, illustrating the fact that 0.5 cm2 substratum samples can harbour a considerable diversity at both intra- and interspecific levels. As the examination of freshly collected rocky samples under a stereo microscope did not reveal any kelp sporophytes, the recruitment in these samples after 50 days of culture probably occurred from microscopic forms of the kelp (i.e. from gametophytes or embryonic sporophytes) and thus corresponds mainly to the bank of microscopic forms which was present on the substratum. These findings are consistent with the growing number of field studies suggesting that kelp microscopic stages are able to resist harsh conditions and may play a crucial role in the recovery of kelp beds (Ladah et al., Citation1999; Barradas et al., Citation2011; Engelen et al., Citation2011; Pereira et al., Citation2011). Recruitment from such stages was particularly obvious in the study of Ladah et al. (Citation1999), who described that after the ENSO events of 1997/1998, macroscopic sporophytes of the giant kelp Macrocystis pyrifera completely disappeared from an area located near the southern distributional limit of the species and remained absent for at least seven months. Nevertheless, macroscopic sporophytes appeared de novo less than one year after their disappearance, though the nearest spore source was more than 100 km away. Ladah et al. (Citation1999) suggested that microscopic stages survived the stressful conditions of ENSO and were the source of the recruitment, supporting the idea that a bank of microscopic forms can survive stressful conditions. However, in all these studies, as in ours, the life stages of the microscopic forms (i.e. gametophytes or embryonic sporophytes) remain uncertain.
The microscopic forms we cultured did reflect the complement of kelp species present in the study site but not their relative abundance. Indeed, although L. digitata was by far the most abundant kelp species in the field, the young sporophytes we obtained after culturing were mainly individuals of S. polyschides. Two different hypotheses might explain this result. First, the bank of microscopic forms might consist mainly of germinations from recently emitted spores, thus inducing a bias in this case towards species reproducing in spring: March is the peak reproductive season for S. polyschides (Norton & Burrows, Citation1969), whereas it is at the end of the reproductive period for S. latissima (Parke, Citation1948) and L. hyperborea (Birkett et al., Citation1998) and prior to reproduction in L. digitata (Birkett et al., Citation1998). Thus, according to this first hypothesis, the dominance of S. polyschides in the bank of microscopic stages present in March is due to a more intense rain of propagules. Alternatively, the dominance of S. polyschides in the recruits might result from differences in competitive abilities among the different kelp species present in the bank of microscopic forms. Indeed, S. polyschides is an annual species and exhibits a high competitive ability (Engelen et al., Citation2011; Pereira et al., Citation2011), whereas L. digitata is perennial. Thus, even though the bank of microscopic forms sampled in the field was composed mainly of L. digitata individuals, S. polyschides might have outcompeted the other kelp species present during germination and/or growth in culture. The culture of substratum revealed the presence of one young sporophyte of L. ochroleuca whereas we did not observe any adult sporophyte in the study site. This suggests that, despite the apparent absence of adult sporophytes, L. ochroleuca has not completely disappeared from the area but instead survives as microscopic forms in a seed-bank analogue. This bank was subsequently able to participate in sporophyte recruitment in culture conditions and this phenomenon might also happen in the field, producing new macroscopic stages under favourable conditions. This finding is consistent with the fact that the proportions of young sporophytes of L. hyperborea and S. latissima in culture (relative to L. digitata) are more than 15 times higher than the proportion of adult sporophytes observed in the study site, suggesting that the sporophytes of these species are not all able to survive at this intertidal level and under these hydrodynamic conditions. These results indicate that microscopic stages may be more tolerant of unfavourable conditions (light, temperature, nutrient and hydrodynamics) than macroscopic stages.
Gametophytes have usually been considered as the resistant phase in kelps. To our knowledge there has been no study investigating the longevity of these stages in the field, although it has been suggested they may only survive for weeks to months (Deysher & Dean, Citation1984). However, in laboratory cultures, gametophytes can survive for decades. For instance, the oldest gametophyte strains of L. digitata available in the Culture Collection of Algae and Protozoa (www.ccap.ac.uk) were isolated in 1974. Furthermore, a laboratory study has shown that kelp gametophytes can survive 16 months in darkness and subsequently regenerate within 1–2 months of post-culture (tom Dieck, Citation1993). These findings suggest that kelp gametophytes have the capacity to survive stressful conditions in the field and to regenerate when conditions improve. Thus, in our experiment, the bank of microscopic stages might consist of gametophytes.
Even though we checked substratum samples immediately after collection, we did not observe any gametophytes or sporophytes. There are several possible explanations. First, gametophytes and/or sporophytes were present but too small to be detected by observations under a stereo microscope. Second, gametophytes were present on the substratum but gametogenesis occurred quickly after sampling, triggering their disappearance (Destombe & Oppliger, Citation2011). Finally, gametophytes were not attached directly to the substratum but hidden as endophytes within microscopic fragments of red algae. Endophytic kelp gametophytes have been reported within various red algae in several studies (Kain, Citation1979; Garbary et al., Citation1999; Hubbard et al., Citation2004). As kelp gametophytes are sensitive to grazing and sedimentation (Vadas et al., Citation1992), it has been suggested that endophytism could provide physical protection (Garbary et al., Citation1999). Further investigations, in particular involving direct detection of gametophytes in the field as has been done for other brown macroalgal species (e.g. Desmarestia ligulata: Edwards, Citation2000), are necessary to discriminate among these hypotheses.
Another feature that emerged from this experiment was considerable disparity in the number of recruits per sample, some pieces of substratum (20 of the 30 samples) yielding zero or one recruits while others produced several to many individuals from an equivalent small area (0.5 cm2). This could be because, although the areas we cultivated were very small, we may have randomly sampled different microhabitats resulting in a highly variable density of microscopic stages. For instance, sampling substrata exhibiting microscale roughness might result in higher numbers of recruits because of spores sedimenting into crevices and being trapped by them. Moreover, such microscopic crevices could protect microscopic gametophytes from grazing. Alternatively, the variation in recruits per sample may have been because of aggregated settlement of kelp spores. Aggregation of individuals is a pattern commonly observed in marine invertebrates (Toonen & Pawlik, Citation2001) and has been reported in all seaweed lineages (Dayton et al., Citation1984; Callow et al., Citation1997; Santelices et al., Citation2008). Because it ensures proximity of reproductive adults, and subsequently of dioecious gametophytes, gregariousness has been described as enhancing reproductive success (Maggs & Callow, Citation2003). In addition, it has been shown to help retain moisture and reduce heat stress during low tides among organisms living in the group (Scrosati & DeWreede, Citation1998). In the particular case of kelps, Reed et al. (Citation1991) showed that a minimum spore density was necessary for sporophyte production in Pterygophora californica and Macrocystis pyrifera, and more recently, Muth (Citation2012) demonstrated that sporophyte production significantly increased as spores became more aggregated in the species M. pyrifera. However, our study was not designed to discriminate between these two hypotheses; thus, it would be interesting to carry out a separate study to specifically address spore settlement.
In conclusion, this paper describes an efficient method to study the species composition of the bank of microscopic forms of kelps and consequently to infer the first steps of recruitment in the field. Other methods have been developed to study the factors influencing algal recruitment by placing artificial substrata in the field and subsequently removing them again for microscopical observations (e.g. Neushul et al., Citation1976; Kennelly, Citation1983). Although these approaches allow the in situ establishment of macroalgal communities to be followed, our method has the advantage of using natural substrata. Furthermore, the method permits substratum-linked factors influencing recruitment to be considered, while also allowing an assessment of the role of microscopic resting stages in recruitment in a climax community. Finally, as an alternative to our approach, DNA metabarcoding of bulk rocky substrata could have been used, without culture and isolation of individual specimens (Taberlet et al., Citation2012). However, these techniques are both currently more expensive and more sensitive to contamination (reviewed by Yoccoz, Citation2012) than the method we present here. Thus, our work demonstrates that, at a time where next-generation sequencing is becoming widespread in modern ecology (Tautz et al., Citation2010), there are still fundamental questions that can be addressed by simple means.
Acknowledgements
This project was supported by the network ‘Bibliothèque du Vivant’ funded by the CNRS, the Muséum National d’Histoire Naturelle, the INRA and the CEA (Centre National de Séquençage). Additional support came from the Interreg programme France (Channel)–England (project Marinexus) and the British Phycological Society (BPS Small grants scheme 2011). L.C. and M.R. thank a postdoctoral fellowship from the Spanish Government (Secretaría de Estado de Universidades e Investigación, Ministerio de Educación) and a PhD fellowship from the French Government (Ministère de l’Enseignement Supérieur et de la Recherche), respectively. We give our deep thanks to the ‘Service Mer et Observation’ of the Station biologique de Roscoff and all the volunteers for their help in the field, as well as S.A. Krueger-Hadfield, L. Le Gall and G.A. Pearson for proofreading. We gratefully acknowledge two anonymous reviewers for their fruitful comments on the manuscript.
References
- Altschul, S.F., Gish, W., Miller, W., Myers, E.W. & Lipman, D.J. (1990). Basic local alignment search tool. Journal of Molecular Biology, 215: 403–410.
- Barradas, A., Alberto, F., Engelen, A.H. & Serrão, E.A. (2011). Fast sporophyte replacement after removal suggests banks of latent microscopic stages of Laminaria ochroleuca (Phaeophyceae) in tide pools in northern Portugal. Cahiers de Biologie Marine, 52: 435–439.
- Birkett, D.A., Maggs, C.A., Dring, M.J., Boaden, P.J.S. & Seed, R. (1998). Infralittoral reef biotopes with kelp species (volume VII), an overview of dynamic and sensitivity characteristics for conservation management of marine SACs. Scottish Association of Marine Sciences (UK Marine SACs Project).
- Callow, M.E., Callow, J.A., Pickett-Heaps, J.D. & Wetherbee, R. (1997). Primary adhesion of Enteromorpha (Chlorophyta, Ulvales) propagules: quantitative settlement studies and video microscopy. Journal of Phycology, 33: 938–947.
- Chapman, A.R.O. (1986). Population and community ecology of seaweeds. In Advances in marine biology (Blaxter J.H.S. & Southwood, A.J., editors), 1–161. Academic Press, London.
- Clayden, S.L. & Saunders, G.W. (2010). Recognition of Rubrointrusa membranacea gen. et comb. nov., Rhodonematella subimmersa gen. et comb. nov. (with a reinterpretation of the life history) and the Meiodiscaceae fam. nov. within the Palmariales (Rhodophyta). Phycologia, 49: 283–300.
- Dayton, P.K., Currie, V., Gerrodette, T., Keller, B.D., Rosenthal, R. & Tresca, D.V. (1984). Patch dynamics and stability of some California kelp communities. Ecological Monographs, 54: 253–289.
- Destombe, C. & Oppliger, L.V. (2011). Male gametophyte fragmentation in Laminaria digitata: a life history strategy to enhance reproductive success. Cahiers de Biologie Marine, 52: 385–394.
- Deysher, L.E. & Dean, T.A. (1984). Critical irradiance levels and the interactive effects of quantum irradiance and dose on gametogenesis in the giant kelp Macrocystis pyrifera. Journal of Phycology, 20: 520–524.
- Edwards, M.S. (2000). The role of alternate life-history stages of a marine macroalga: a seed bank analogue? Ecology, 81: 2404–2415.
- Engel, C.R., Billard, E., Voisin, M. & Viard, F. (2008). Conservation and polymorphism of mitochondrial intergenic sequences in brown algae (Phaeophyceae). European Journal of Phycology, 43: 195–205.
- Engelen, A.H., Lévêque, L., Destombe, C. & Valero, M. (2011). Spatial and temporal patterns of recovery of low intertidal Laminaria digitata after experimental spring and autumn removal. Cahiers de Biologie Marine, 52: 441–453.
- Fox, C.H. & Swanson, A.K. (2007). Nested PCR detection of microscopic life-stages of laminarian macroalgae and comparison with adult forms along intertidal height gradients. Marine Ecology Progress Series, 332: 1–10.
- Garbary, D.J., Kim, K.Y., Klinger, T. & Duggins, D. (1999). Red algae as hosts for endophytic kelp gametophytes. Marine Biology, 135: 35–40.
- Goff, L.J. & Moon, D.A. (1993). PCR amplification of nuclear and plastid genes from algal herbarium specimens and algal spores. Journal of Phycology, 29: 381–384.
- Hoffmann, A.J. & Santelices, B. (1991). Banks of algal microscopic forms: hypotheses on their functioning and comparisons with seed banks. Marine Ecology Progress Series, 79: 185–194.
- Hubbard, C.B., Garbary, D.J., Kim, K.Y. & Chiasson, D.M. (2004). Host specificity and growth of kelp gametophytes symbiotic with filamentous red algae (Ceramiales, Rhodophyta). Helgoland Marine Research, 58: 18–25.
- Kain, J.M. (1979). A view of the genus Laminaria. Oceanography and Marine Biology: an Annual Review, 17: 101–161.
- Kennelly, S.J. (1983). An experimental approach to the study of factors affecting algal colonization in a sublittoral kelp forest. Journal of Experimental Marine Biology and Ecology, 68: 257–276.
- Ladah, L.B., Zertuche-González, J.A. & Hernández-Carmona, G. (1999). Giant kelp (Macrocystis pyrifera, Phaeophyceae) recruitment near its southern limit in Baja California after mass disappearance during ENSO 1997–1998. Journal of Phycology, 35: 1106–1112.
- Lane, C.E. & Saunders, G.W. (2005). Molecular investigation reveals epi/endophytic extrageneric kelp (Laminariales, Phaeophyceae) gametophytes colonizing Lessoniopsis littoralis thalli. Botanica Marina, 48: 426–436.
- Maggs, C.A. & Callow, M.E. (2003). Algal spores, version 1.0. In Encyclopedia of life sciences. Nature Publishing Group, London.
- Manghisi, A., Morabito, M., Bertuccio, C., Le Gall, L., Couloux, A., Cruaud, C. & Genovese, G. (2010). Is routine DNA barcoding an efficient tool to reveal introductions of alien macroalgae? A case study of Agardhiella subulata (Solieriaceae, Rhodophyta) in Cape Peloro lagoon (Sicily, Italy). Cryptogamie: Algologie, 31: 423–433.
- McFarland, D.G. & Shafer, D.J. (2011). Protocol considerations for aquatic plant seed bank assessment. Journal of Aquatic Plant Management, 49: 9–19.
- Müller, D.G. & Ramírez, M.E. (1994). Filamentous brown algae from the Juan Fernández Archipelago (Chile): contribution of laboratory culture techniques to a phytogeographic survey. Botanica Marina, 37: 205–212.
- Muth, A.F. (2012). Effects of zoospore aggregation and substrate rugosity on kelp recruitment success. Journal of Phycology, 48: 1374–1379.
- Neushul, M., Foster, M.S., Coon, D.A., Woessner, J.W. & Harger, B.W.W. (1976). An in situ study of recruitment, growth and survival of subtidal marine algae: techniques and preliminary results. Journal of Phycology, 12: 397–408.
- Norton, T. & Burrows, E. (1969). Studies on marine algae of the British Isles. 7. Saccorhiza polyschides (Lightf.) Batt. British Phycological Journal 4: 19–53.
- Parke, M. (1948). Studies on British Laminariaceae. I. Growth in Laminaria saccharina (L.) Lamour. Journal of the Marine Biological Association of the United Kingdom, 27: 651–709.
- Pawlowski, J., Audic, S., Adl, S., Bass, D., Belbahri, L., Berney, C., Bowser, S.S., Cepicka, I., Decelle, J., Dunthorn, M., Fiore-Donno, A.M., Gile, G.H., Holzmann, M., Jahn, R., Jirků, M., Keeling, P.J., Kostka, M., Kudryavtsev, A., Lara, E., Lukeš, J., Mann, D.G., Mitchell, E.A.D., Nitsche, F., Romeralo, M., Saunders, G.W., Simpson, A.G.B., Smirnov, A.V., Spouge, J.L., Stern, R.F., Stoeck, T., Zimmermann, J., Schindel, D. & De Vargas, C. (2012). CBOL protist working group: barcoding eukaryotic richness beyond the animal, plant, and fungal kingdoms. PLoS Biology, 10: e1001419.
- Pereira, T.R., Engelen, A.H., Pearson, G.A., Serrão, E.A., Destombe, C. & Valero, M. (2011). Temperature effects on the microscopic haploid stage development of Laminaria ochroleuca and Saccorhiza polyschides, kelps with contrasting life histories. Cahiers de Biologie Marine, 52: 395–403.
- Reed, D.C., Neushul, M. & Ebeling, A.W. (1991). Role of settlement density on gametophyte growth and reproduction in the kelps Pterygophora californica and Macrocystis pyrifera (Phaeophyceae). Journal of Phycology, 27: 361–366.
- Roberts, H.A. (1981). Seed banks in soils. Advances in Applied Biology, 6: 1–55.
- Santelices, B., Hoffmann, A.J., Aedo, D., Bobadilla, M. & Otaiza, R. (1995). A bank of microscopic forms on disturbed boulders and stones in tide pools. Marine Ecology Progress Series, 129: 215–228.
- Santelices, B., Correa, J.A., Meneses, I., Aedo, D. & Varela, D. (2008). Sporeling coalescence and intraclonal variation in Gracilaria chilensis (Gracilariales, Rhodophyta). Journal of Phycology, 32: 313–322.
- Saunders, G.W. & Mcdevit, D.C. (2012). Methods for DNA barcoding photosynthetic protists emphasizing the macroalgae and diatoms. Methods in Molecular Biology, 858: 207–222.
- Scrosati, R. & Dewreede, R.E. (1998). The impact of frond crowding on frond bleaching in the clonal intertidal alga Mazzaella cornucopiae (Rhodophyta, Gigartinaceae) from British Columbia, Canada. Journal of Phycology, 34: 228–232.
- Starr, R.C. & Zeikus, J.A. (1993). UTEX – The culture collection of algae at the University of Texas at Austin 1993 list of cultures. Journal of Phycology, 29: 1–106.
- Taberlet, P., Coissac, E., Pompanon, F., Brochmann, C. & Willersev, E. (2012). Towards next-generation biodiversity assessment using DNA metabarcoding. Molecular Ecology, 21: 2045–2050.
- Tautz, D., Ellegren, H. & Weigel, D. (2010). Next generation molecular ecology. Molecular Ecology, 19: 1–3.
- Tom Dieck, I. (1993). Temperature tolerance and survival in darkness of kelp gametophytes (Laminariales, Phaeophyta): ecological and biogeographical implications. Marine Ecology Progress Series, 100: 253–253.
- Toonen, R.J. & Pawlik, J.R. (2001). Foundations of gregariousness: a dispersal polymorphism among the planktonic larvae of a marine invertebrate. Evolution, 55: 2439–2454.
- Vadas, R.L., Sr, Johnson, S. & Norton, T.A. (1992). Recruitment and mortality of early post-settlement stages of benthic algae. British Phycological Journal, 27: 331–351.
- Yoccoz, N.G. (2012). The future of environmental DNA in ecology. Molecular Ecology, 21: 2031–2038.