ABSTRACT
Populations of the benthic diazotrophic cyanobacterium Hydrocoleum Kützing (1843) are widely distributed in coral reefs and lagoons of tropical oceans, where they contribute to benthic nitrogen fixation. We compared the morphological, molecular and physiological features of 31 freshwater and marine Hydrocoleum populations from geographically different localities and five specimens from herbaria. Our aims were to compare the features of Hydrocoleum with the morphologically and phylogenetically related genera Trichodesmium Ehrenberg ex Gomont 1892 and Blennothrix Kützing 1843, and to provide the basis for the validity of the genus Hydrocoleum. The Hydrocoleum populations were distributed over a wide range of sizes, showing no consistent inter-population grouping and leaving no gaps between groups that were traditionally attributed to different species. Transmission electron microscopy showed regular cell division rhythms in all Hydrocoleum populations with each cell division completed before the next one was initiated, similar to that described for Trichodesmium. In contrast, Blennothrix populations exhibited multiple initiation of cross-wall formation. Most Hydrocoleum populations fixed nitrogen at consistently higher nightly rates. The phylogenetic analysis of 16S rRNA and nifH gene sequences placed all morphologically distinct Hydrocoleum and Trichodesmium populations within a tight cluster. Neither of the analysed genes brought any further resolution of phenotypically and ecologically distinguished forms. However, freshwater morphospecies of Blennothrix together with the type species Blennothrix vermicularis Kützing were separated from the Trichodesmium/Hydrocoleum cluster, which further supported the genotypic distinction between the compared taxa, in addition to the fact that they also differ in morphology and ecology. We concluded that the genus Hydrocoleum possesses unique features that warrant its maintenance in the botanical classification system and that its introduction in bacteriological manuals as a valid cyanobacterial genus is acceptable.
INTRODUCTION
Populations of Hydrocoleum are common and persistent inhabitants of the benthos of shallow lagoons and coral reefs, where they form distinct colonies and coherent mats (Abed et al., Citation2003b, Citation2006; Palinska et al., Citation2012; Charpy et al., Citation2010, Citation2012a, Citation2012b). They occur on hard substrata such as dead corals and coral rubble, sandy sediments and as epiphytes overgrowing sea grasses and algae. Under prevailing oligotrophic conditions, benthic cyanobacteria in reefs are subordinate to the growth of eukaryotic algae and healthy corals. However, the release of nutrients following storms and man-made environmental impacts often result in formation of massive blooms dominated by particular cyanobacterial taxa (Szmant, Citation2001; Paul et al., Citation2005; Laurent et al., Citation2012).
Figs 1–4. Field populations of: Fig. 1. Hydrocoleum coccineum Mayotte-4, reef Cou 4-2, 2 m depth; Fig. 2. Field population of H. glutinosum Mayotte-2, attached to Acropora (reef CB2), depth 7 m; Fig. 3. H. majus Mayotte-5; reef Cou 4-2, 2 m depth; Fig. 4. H. glutinosum PP1. Noumea lagoon, New Caledonia.
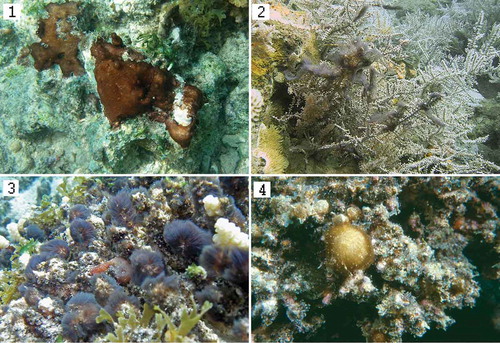
Figs 5–15. Photomicrographs of: Figs 5–14: Hydrocoleum populations representing six morphospecies (note distinctions in size, trichome morphology and pigmentation) and Fig. 15. Blennothrix ganeshii Mex3. Fig. 5. H. coccineum TK21; Fig. 6. H. lyngbyaceum T3; Fig. 7. H. coccineum M4; Fig. 8. H. glutinosum M21; Fig. 9. H. glutinosum M34; Fig. 10. H. cantharidosmum GC; Fig. 11. H. majus RU2007; Fig. 12. H. majus M5; Fig. 13. Exsiccate specimens from the NHN: H. lyngbyaceum (L0055299); Fig. 14. Exsiccate specimens from the Herbarium NHN: H. brebissonii (L0571047); Fig. 15. Filaments of Blennothrix ganeshii Mex3. Scale bar for Figs 5–12 is in Fig. 5.
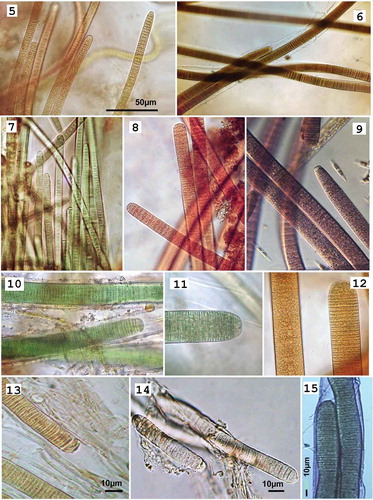
In New Caledonia, massive populations of Hydrocoleum lyngbyaceum Kützing ex Gomont, Citation1892 and H. glutinosum Gomont ex Gomont, Citation1892 have been identified as the cause of fish poisoning (Laurent et al., Citation2012; Golubic et al., Citation2009; Méjean et al., Citation2009). Detailed morphological and molecular (16S rRNA, nifH and phycocyanin and phycoerythrin intergenic spacers genes) characterization of natural populations of Hydrocoleum from tropical lagoons of Tikehau Atoll, French Polynesia and around New Caledonia demonstrated their potential to fix nitrogen and showed a phylogenetic proximity to the planktonic Trichodesmium (≥ 98% 16S rRNA sequence similarity) (Abed et al., Citation2006). It was suggested (Abed et al., Citation2006) that the benthic Hydrocoleum and the planktonic Trichodesmium have a common evolutionary origin, but maintain separate ecological niches, which they support with distinct cellular arrangements and structures. Both Hydrocoleum and Trichodesmium fix dinitrogen in the ocean and are major contributors to the nitrogen budget of tropical oceans, but they show differences in timing of nitrogenase activity.
The genus Hydrocoleum was described in the mid-19th century by Kützing (Citation1843) and endorsed later by Gomont’s determination manual (Citation1892), which is acknowledged as the botanical taxonomic ‘later starting point’ source for oscillatoriacean cyanobacteria, because the earlier descriptions were often poorly documented. Gomont (Citation1892) described and illustrated several marine and freshwater species of Hydrocoleum. Kützing (Citation1843) also described a marine cyanobacterium under the name Blennothrix vermicularis, which was later revised by Gomont as a variety of Hydrocoleum glutinosum β vermiculare. Both Hydrocoleum and Blennothrix are retained in the new botanical revision of cyanobacterial systematics (Komárek & Anagnostidis, Citation2005), albeit in different families with the species revised and distributed between them. Here, we test whether the reclassification of Hydrocoleum taxa under the name Blennothrix is supported by ultrastructural and molecular data.
Sequences of 16S rRNA and nifH genes are now available from natural populations of Hydrocoleum (Abed et al., Citation2003b, Citation2006) and from uni-cyanobacterial cultures (Foster et al., Citation2009). In the present contribution, we compare phenotypic (morphological and morphometric features), physiological and genotypic (16S rRNA and nifH gene sequences) properties of natural Hydrocoleum populations from geographically different localities with representatives of the genera Trichodesmium and Blennothrix. We provide here a detailed description of cultured and uncultured populations of Hydrocoleum including their molecular signatures toward a future introduction of this genus into Bergey’s Manual.
MATERIALS AND METHODS
Hydrocoleum populations
Eleven samples of Hydrocoleum populations were collected by scuba diving between 1998 and 2007 in water depths of up to 25 m in the Indian Ocean (Mayotte, Comores, Tulear Reef, Madagascar, and Réunion Island), Atlantic Ocean (Gran Canaria) and Adriatic Sea (Iz Island) (). Previously studied populations were included for comparison, including four populations from Tikehau, Tuamotu Archipelago (Abed et al., Citation2003b), five from New Caledonia (Abed et al., Citation2006), one from Réunion Island (Charpy et al., Citation2010) and one isolate from Highbourne Cay (Bahamas) (Foster et al., Citation2009). Additionally, five specimens of exsiccata: H. lyngbyaceum (L0055295, type material), H. cantharidosum L0571047 Gomont ex Gomont Citation1892 and H. brebissonii L0571047 Kützing ex Gomont Citation1892 from the National Herbarium of the Netherlands (NHN) as well as H. glutinosum HUC688061 and H. lyngbyaceum HUC795080 from the University of California Herbarium (HUC) were also included into the study. Three populations of Blennothrix ganeshi Watanabe & Komárek Citation1989, collected in different freshwater localities in Mexico as well as the type material of Blennothrix vermicularis from NHN were analysed for taxonomic comparison.
Table 1. Morphotypic properties of studied Hydrocoleum populations.
Hydrocoleum populations that commonly form dense colonies and mini-blooms dominated by a single species were identified microscopically and separated by micromanipulation immediately following collection. Subsamples for DNA analysis were preserved in guanidine thiocyanate solution (GES) in 2 ml Eppendorf tubes. GES-solution included 5 M guanidine thiocyanate, 100 mM EDTA at pH 8.0 and 3.4 mM N-lauroyl sarcosine. Subsamples for further microscopic analysis and morphometry were preserved in 3% formaldehyde solution in environmental water. Hydrocoleum cantharidosmum strain GC was isolated from a natural population growing in the lower part of a c. 2.5 m wide intertidal zone (1 m depth during mid-tide), on the coast of Gran Canaria Island by selecting single filaments under the dissecting microscope. The strain was isolated to observe morphotype changes caused by cultivation. The strain was purified mechanically by separation of filaments from accompanying microorganisms under a dissecting microscope. Purification by isolation of single filaments was attempted with medium PCR-Tu2, previously used for cultivation of Prochlorococcus Chisholm, Frankel et al., 1992 strains (Rippka et al., Citation2000). This artificial seawater medium based on Turks Island Salts solution (Merck Index no. 9954) gave optimal growth of the strain.
Microscopy, ultrastructures and morphometric analysis
Light-microscopic analysis and photomicrographic documentation were carried out using transmitted light, phase contrast, Nomarski differential interference contrast (DIC) illumination and epifluorescence. Transmission electron microscopy (TEM) was carried out for the following specimens: the strain H. cantharidosmum-GC, H. lyngbyaceum IZ obtained from natural population, exsiccata of National Herbarium of the Netherlands and University of California Herbaria (NHN and HUC), exsiccata of H. glutinosum (NHN and HUC), an exsiccatum of the freshwater H. brebissonii NHN and specimens from three populations of Blennothrix ganeshii from Mexico. Subsamples for TEM were prepared as previously described (Palinska & Surosz, Citation2008). Herbarium specimens were soaked in 0.1 M sodium phosphate buffer (pH 7.2) or 10% SDS solution for several days. Further sample preparation and embedding followed the procedure as described above.
Morphometric analysis of cell dimensions was carried out from in-scale camera lucida projections and scanned photomicrographs using Sigma Scan Image software (Jandel Scientific, Sausalito, CA) with its statistics package. The variations in dimensions are expressed as: mean ± standard deviation (n = 30–50) and diagrammatically presented as cell width vs cell length relations. Determination of cyanobacteria followed Gomont (Citation1892) as the botanical later starting point for non-heterocystous filamentous cyanobacteria, while consulting Geitler (Citation1932), Bergey’s Manual (Castenholz et al., Citation2001) and the determination manual of Komárek & Anagnostidis (Citation2005).
Molecular analyses
DNA was extracted from 1 ml of the Hydrocoleum suspensions in guanidine thiocyanate as described before (Abed et al., Citation2003a). A subsample of herbarium specimens was pulverized and rehydrated with 10–100 ml distilled water in a 0.5 ml Eppendorf vial. The slurry was homogenized and exposed to five freeze–thaw cycles, alternating immersion in liquid nitrogen and heating to 60°C. Rehydrated cells were either used directly in PCR amplifications or the mixture was purified with the help of a MolBio DNA extraction Kit.
16S rRNA gene fragments were amplified using cyanobacteria-specific primers Cya106F, Cya781R described in Nübel et al. (Citation1997) and 1494R published by Taton et al. (Citation2003). Purified (QIAquick PCR Purification Kit, Qiagen, Germany) PCR products (440–1400 bp, short) were commercially sequenced in both directions. The nifH gene fragment was amplified from four representative populations of Hydrocoleum: Hydrocoleum lyngbyaceum IZ, H. lyngbyaceum (NHN), Hydrocoleum majus Holden 1899, RU2007 and Hydrocoleum brebissonii (NHN) as well as from one population of Blennothrix ganeshii using degenerate oligonucleotides described by Zehr & McReynolds (Citation1989). In addition, three other nifH sequences from Hydrocoleum populations from New Caledonia (Abed et al., Citation2006) were included for comparison. The amplified nifH fragments (359 bp including primers) were purified by gel electrophoresis and cloned in competent Escherichia coli cells using the TOPO TA Cloning Kit, Invitrogen. The recombinant clones carrying the correct-sized insert were sequenced on both strands. A phylogenetic tree was constructed using all cyanobacterial nifH fragments published to date.
Sequences obtained were aligned and analysed using the ARB software (Ludwig et al., Citation1998) version 071207 and the official database (http://www.arb-silva.de) for small subunit RNA sequences (SSURef_NR99_115_SILVA_20_07_13_opt.arb) (Prüsse et al., Citation2007). Complete cyanobacterial 16S rRNA gene sequences available from GenBank were imported and aligned to the sequences in the ARB database. The contig cyanobacterial sequences obtained were aligned against each other in order to obtain consensus sequences. These sequences were then aligned with the sequences in the ARB database using the alignment ARB tool. The alignment was corrected manually. The phylogenetic trees were calculated by maximum likelihood (ML) method, based on long 16S rRNA gene sequences (> 1200 bp).
To evaluate the consistency of computed tree topologies, subsets of data were analysed using various algorithms as follows. A variety of single and multiple outgroup sequences representing phylogenetically diverse organisms were included in the analysis. To assess the influence of the most variable nucleotide positions they were excluded from some calculations by applying filters based on character frequency (ARB manual; Ludwig et al., Citation1998).
Bootstrap values were calculated based on 100 replicates. Ten partial sequences of 440 bp obtained in this study were inserted into the ML tree using the parsimony ARB tool, while maintaining the overall tree topology without changes (sequences marked with * in ).
Chromatic adaptation and nitrogenase activity
Phycobiliprotein absorption spectra were measured in cells growing at exponential phase. A 2–4 ml aliquot of culture was centrifuged at 14 000 rpm for 5 min at room temperature. The pellet was suspended in a buffer consisting of: 50 mM Tris/HCl (pH 8), 250 mM NaCl and 10 mM EDTA and the cells were broken ultrasonically. In order to include chlorophyll a peak in the spectrum, the broken cell suspension was incubated for 30 min at 28°C with 5% Triton, and finally centrifuged for 5 min at 14 000 rpm in an Eppendorf centrifuge to remove unbroken cells. The absorbance of the supernatant was measured from 400 to 750 nm at a Hitachi U 3000 spectrophotometer. Complementary chromatic adaptation (CCA) experiments were performed on strains after 6 weeks of accommodation to continuous light with fluorescent lamps (76 µmol photons m−2 s−1). Chromatic illumination experimental conditions were provided by red or green plastic filters as described by Tandeau de Marsac (Citation1977) interposed between the culture vessels and the fluorescent tubes.
To measure nitrogenase activity, c. 1 cm2 of a Hydrocoleum colony was incubated in 65 ml bottles fitted with a septum with 15 ml of 0.22 filtered seawater (DIN<0.5 µM). For heterotrophic nitrogenase activity measurements, 3 replicates were incubated in the dark for 24 h; afterwards 5 ml of C2H2 was added to observe any heterotrophic N2 fixation after 12 h incubation in the dark. For phototrophic nitrogenase activity measurements, cultures were kept 8 days in an incubation chamber (day and night) to remove the DIN. After 8 days, 5 ml of C2H2 was added to 2 replicates and C2H4 production was measured after 24 hours of incubation. The biomass of incubated benthic cyanobacteria was calculated from the chlorophyll a content. At the end of incubation with C2H2, the mat was dried using Whatman filter paper and frozen for later spectrophotometric analysis (Charpy et al., Citation2007). Dinitrogen fixation rate was calculated by using factor 4 in conversion from acetylene reduction rate (Mulholland, Citation2004).
RESULTS
Populations and morphospecies of Hydrocoleum
Hydrocoleum populations showed variation in colony formation ranging from firm to loose mats, cushions and tufts as well as in colour, ranging from olive-green to violet to bright red (–). Microscopically, Hydrocoleum mats were composed of interwoven filaments containing one to multiple trichomes within a common sheath, which they readily abandoned by gliding when under stress. The trichomes were unconstricted at the cross-walls, or mildly constricted, variable in colour due to different combinations of photosynthetic pigments and their expression, briefly attenuated at the tips differentiating a distinct calyptrate terminal cell (–).
Morphological characteristics of the genus Hydrocoleum conformed to the criteria defining non-heterocystous filamentous cyanobacteria (Oscillatoriales, section III of Rippka et al., Citation1979) that can be observed macro- and microscopically. The properties of this genus show considerable variability, as noted in determination manuals and was observed in the field populations compared here. They include the following features: (i) filaments joined to form flattened mats (strata), small compact cushions (pulvinuli, capituli) or upright tufts (caespituli); (ii) trichomes situated parallel to each other, one to several within a wide, gelatinous common sheath (EPS); (iii) trichomes gliding within sheaths, bypassing each other and/or separating while each secreting a new (thinner) sheath in the process of forming false branches (see Golubic et al., Citation1996); (iv) trichomes cylindrical, unconstricted, or only slightly constricted at the cross-walls, with or without granulation; (v) trichomes gradually or abruptly attenuated at the tips, with a distinct, narrower and compressed, often capitated terminal cell with a thickened outer cell wall or calyptra; (vi) cells of variable length, on the average shorter or longer than wide; (vii) pigmentation varying from different shades of blue-green to bright red. Formal botanical descriptions of species of Hydrocoleum assigned different combinations of the above properties as criteria for species recognition, including assignment of particular ranges in cell dimensions.
The cell width of filamentous cyanobacteria is considered a taxonomically stable property. We have tested the variability in cell width within the analysed field populations and found it to be consistently narrow, with standard deviation departing less than 10% of the corresponding mean values, with an average coefficient of variation (SD/mean) of 0.048. Such narrow variability within populations was in contrast to the extremely wide variability in filament width between populations, referring to taxa of similar morphology, but differing in cell size and proportions (). The total range of measured cell widths in populations under the present study was from 6.5 to 28.2 µm. Several populations, including the end-member ones within the above size range, which were often co-occurring, were often separated by sizable gaps, which were, in other habitats occupied by groups of intermittent-sized populations, often with overlapping margins. The size ranges of corresponding morphospecies designated by their original descriptors and accepted by later taxonomic reviews do overlap, and so do the sizes of natural populations we have analysed ().
Fig. 16. Cell dimensions: cell width (CW) vs. cell length (CL) plotted as ‘cross diagrams’ of means ± standard deviation for Hydrocoleum populations from three different geographic locations: Mayotte, Tulear and Noumea presented as cross diagrams of cell width vs cell length for each population. Cell dimensions are measured as means ± standard deviation. The area covered by the crosses in each diagram, with arms extending one standard deviation on each side of the mean, include about 63% of the sample (about 95% of the cells measured scatter over the field outlined by two standard deviation distance from the mean value). The areas marked by crosses include about 63% of the sample. The lowest diagram shows the size ranges of different Hydrocoleum species as described by Gomont (Citation1892).
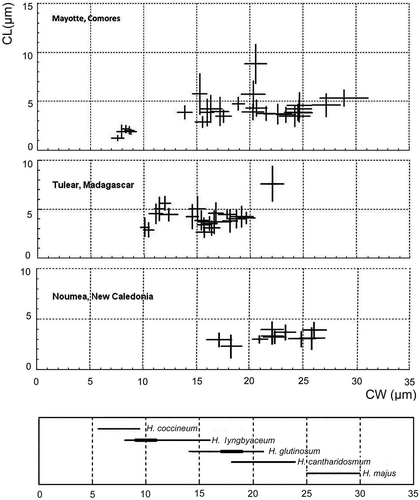
Ultrastructure comparison of Hydrocoleum, Trichodesmium and Blennothrix
The TEM imagery was necessary to observe the initiation and completion of cross-walls in the process of cell fission, which may have taxonomic implications, as this is difficult to detect in filamentous cyanobacteria. Cell division in cyanobacteria is a binary fission with cross-walls initiated at the periphery of the cell and closing toward the cell centre like an iris screen. In all specimens of Hydrocoleum analysed (covering the entire range of cell width), including the large forms with very short cells, the new cross-walls are initiated after the complete closure of the cross-wall of the preceding cell division (, ). Published TEM images of Trichodesmium (Van Baalen & Brown, Citation1969; Fredriksson & Bergman, Citation1997; Finzi-Hart et al., Citation2009) also show regular binary fission similar to that observed in Hydrocoleum. In contrast, the specimens of Blennothrix ganeshii exhibited multiple initiations of cross-wall closures (, , marked by sequence numbers) showing the two subsequent cell divisions (2 and 3) started before the first cross-wall (1) was completely closed.
Diversity of 16S rRNA and nifH gene sequences
The phylogenetic analysis of 16S rRNA gene sequences of Hydrocoleum populations produced a close cluster of morphologically distinct forms in the phylogenetic tree (), which also included the sequences of Trichodesmium. The topology of the nifH gene () produced a similar phylogeny to that of the 16S rRNA. Both placed Hydrocoleum and Trichodesmium populations within a tight cluster. Neither of the analysed genes brought any further resolution of phenotypically and ecologically distinguished forms. Several additional strains, listed in GenBank as members of genera Oscillatoria and Lyngbya, e.g. Oscillatoria sp. PAB-21, O. sancta Kützing ex Gomont Citation1892, PCC 7515, Lyngbya sp. BAN TS17, Oscillatoria sp. PAL08-3.2 with unclear strain history, also occurred within the same cluster. These strains and populations may have been incorrectly identified as they were used for biotechnological and pharmacological purposes and not for taxonomic comparison. In addition, many species of Oscillatoria and Lyngbya morphologically resemble representatives of the genus Hydrocoleum and this may have led to misidentification among these three genera.
Fig. 21. 16S rRNA gene-based phylogenetic reconstruction based on ML methods, showing the affiliation of Hydrocoleum populations obtained from Mayotte, Tulear, Iz, Gran Canaria and two herbarium specimens together with Blennothrix ganeshii populations and previously published sequences. Accession numbers are indicated in parentheses. The scale bar shows 10% sequence divergence. Sequences obtained in this study are marked in bold. Short sequences are marked with ’*’.
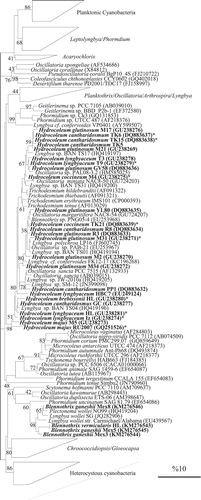
Fig. 22. Phylogenetic tree of nifH gene based on ML methods from four selected populations from the present study of Hydrocoleum: H. lyngbyaceum IZ, H. lyngbyaceum (NHN), Hydrocoleum majus RU2007 and Hydrocoleum brebissonii (NHN) together with three other nifH sequences from Hydrocoleum populations from New Caledonia (Abed et al., Citation2006) and Blennothrix ganeshii population from Mexico. The scale bar shows 10% sequence divergence. Sequences obtained in this study are marked in bold.
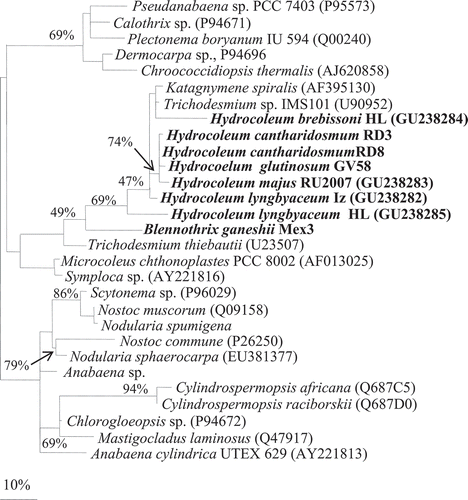
Hydrocoleum populations shared 95–99.9% similarity in16S rRNA sequences and 90.2–95.2% in nifH gene sequences. The clustering of the 16S rRNA gene sequences did not correspond to morphospecies as defined by cell size ranges (). The sequence of populations ascribed to H. lyngbyaceum occurred in two different places in the phylogenetic tree. Type material of H. lyngbyaceum-HL from the North Sea deposited in National Herbarium of the Netherlands (NHN) occurred close to the population H. lyngbyaceum-IZ (Adriatic) and also included the freshwater H. brebissonii (NHN). Two populations identified as H. lyngbyaceum from Tulear showed closer relation to H. cantharidosmum and H. glutinosum than to H. lyngbyaceum populations from the Bahamas. Three populations of H. cantharidosmum from Tikehau Atoll clustered together, but other populations of similar morphotypes and size were distributed on separate branches.
Blennothrix vermicularis (NHN, type species) and Blennothrix ganeshii from Mexico clustered together and showed 97–99% and 95% similarity in 16S rRNA and nifH, respectively. Sequence similarity with population of H. majus RU2007 and H. lyngbyaceum (herbarium NHN) revealed 90–94% similarity in 16S rRNA gene sequences. The display of the nifH gene fragment sequences maintained the close integration between Hydrocoleum and Trichodesmium.
Complementary chromatic adaptation (CCA) and nitrogen fixation rates
The isolate H. cantharidosmum-GC responded positively to CCA when cultured under green- and red-coloured filters, while adjusting for light intensity. The phycoerythrin/phycocyanin ratio was enhanced under green light, whereas the ratio of pigments was inversed under red light so that the trichomes became greenish in colour. The responses were recorded after 21 days of exposure to the experimental illuminations.
Nitrogenase activity was measured during the day and night for identified populations immediately following their collection. Most measured populations (entire range of sizes) fixed nitrogen. Out of 21 identified and measured Hydrocoleum populations, 18 showed positive nitrogen fixation rates (). All samples showed consistently higher nightly rates. The calculated rates of nitrogenase activity range from 0.15 (H. glutinosum-M21) to 2.75 nM N2 µg chl a−1 h−1 (H. cantharidosmum TK6). The Mayotte population of H. coccineum Gomont Citation1892 M4, showed N-fixing activity both during the day and night: 0.84 vs. 1.13 nM N2 µg−1chl a−1 h−1. The strain H. cantharidosmum-GC fixed dinitrogen at a rate of 4.6 nM N2 µg−1chl a−1 h−1.
DISCUSSION
We present here a comprehensive polyphasic study of natural populations of the marine benthic cyanobacteria of the genus Hydrocoleum that are common in shallow marine habitats of three world oceans. The targets of our investigation are natural populations recognized by morphological criteria. Our approach seeks to provide a ‘face to molecular signature’, which would otherwise remain anonymous or associated with the closest available name recorded in the GenBank.
Distribution pattern of Hydrocoleum populations
The cell dimension morphometry of Hydrocoleum populations demonstrated a narrow intra-population and an extensive inter-population variation. The standard deviation within a particular population departed less than 10% of the corresponding mean values, which identified these populations as clonal. The populations were distributed over a wide range of sizes showing no consistent grouping. The population grouping is different from region to region, including occurrences of gaps, which in other locations were filled by local populations. Thus, geographic distinctions are expressed by variation in frequency of populations of particular size ranges (). Different representation of size-defined populations in geographically separated sites suggests that the colonization of the habitat by these populations is determined historically by the availability of settlement area and propagules as ‘demographic replacements’ (Templeton, Citation1989). By including populations from different geographic regions, the total array of populations forms a continuum leaving no gaps between them. Such pattern in size distribution may suggest that, within the range of compared microbial mats, the cell sizes (and proportions) and their variations appear to be selectively neutral, permitting coexistence of clonal populations of different sizes.
The pattern is similar to the speciation in eukaryotic organisms when it occurs under reproductive isolation by distance. When populations expand at rates that exceed internal gene-flow, the marginal populations diverge into subspecies to form vicariant ‘ring’ species so that the end-members may become inter-sterile (Moritz et al., Citation1992; Irwin et al., Citation2001). This pattern seems more common in prokaryote populations with less regular horizontal gene flow. Similar clonal nature of populations and similar patterns of ‘continuous speciation’ measured by cell dimensions have been documented earlier for Trichodesmium (Golubic, Citation1977) and expressed by frequency distribution of taxa (Janson et al., Citation1999; Orcutt et al., Citation2002).
Hydrocoleum populations and strains examined in this study shared most of the generic properties, but they showed variations regarding colony formation, sheath consistency, and pigmentation in addition to variation in dimensions and proportions. These properties are distinctive, but they may also be subject to environmental influences. We have tested the ‘complementary chromatic adaptation’ in Hydrocoleum cantharidosmum-GC, under culture conditions, because it is difficult to evaluate these features in natural populations in terms of their stability and taxonomic value. Genetic control is suspected in cases when such distinctions persist in co-occurring organisms in addition to being correlated with differences in cell size, proportions or other properties. Whether similar patterns of diversification evolved in properties of cyanobacteria other than dimensions, and to what extent are they environmentally modifiable, remains to be tested.
Confronted with such continuous distribution of populations of similar organisms, the size ranges reported in species descriptions and proscribed in determination manuals (, bottom) appear incongruent with morphological data such as cell width. This pattern may have prompted Geitler (Citation1932) to coin the term ‘collective species’ (Sammelspecies) for diverging taxa that do not offer the convenience of separation by hiatus.
Diversity within the genus Hydrocoleum: morphology vs phylogeny
Our results suggest that the cell size, expressed as trichome diameter does not provide a good base for phylogenetic ordering of Hydrocoleum phenotypes, and therefore combinations of other phenotypically expressed properties need to be examined more closely. The marine species in the same size range such as H. lyngbyaceum from NHN, H. lyngbyaceum from the Adriatic Sea and the freshwater H. brebissonii, clustered together in the 16S rRNA-based phylogenetic tree, but the same cluster also contained much larger H. cantharidosmum and H. majus. Conversely, two populations of the same size range as H. lyngbyaceum from the Reef of Tulear (SW Madagascar) plotted separately, and so did other populations of the larger H. cantharidosmum and H. majus. Similarly inconsistent support by 16S rRNA gene grouping refers to geographic distribution of organisms of different sizes. Two populations of H. lyngbyaceum from Tulear grouped together as did three populations of H. cantharidosmum of the Tikehau Atoll. These populations conferred by size as well. However, populations conforming by size to H. glutinosum occurred separately in two different clusters and so did the larger H. cantharidosmum. Therefore, neither the sequences of the 16S rRNA nor of the nifH gene arrangements exhibited adequate resolution power to separate taxa within the genus Hydrocoleum. Similar discrepancies between morphotype characteristics and molecular clustering, including size ranges, have been documented for the populations of the planktonic Trichodesmium (Janson et al., Citation1999).
Hydrocoleum and Trichodesmium: similarities and differences
Our results confirmed the previous findings that 16S rRNA and and nifH gene sequence fragments of benthic Hydrocoleum populations plot closely together with those of the planktonic Trichodesmium, forming a well-defined Hydrocoleum/Trichodesmium cluster (Abed et al., Citation2006). The sequence similarities varied between 96.0 and 97.1%. According to the present bacteriological taxonomic practice (Stackebrandt & Ebers, Citation2006), they should be considered the same genus and, in some combinations, even the same species. However the conserved nature of rRNA gene has shown a tendency to underestimate natural diversity in cyanobacteria (Siegesmund et al., Citation2008; Turicchia et al., Citation2009; Strunecký et al., Citation2011, Citation2013; Hašler et al., Citation2012; Engene et al., Citation2013). Furthermore, other criteria, such as ecophysiology, biochemistry (Abed et al., Citation2006), and phenotypic differences have been proposed to be more decisive characters than only the percentage of genetic similarity (Hynes et al., Citation2012). The similarity of 97.6 to 99.7% in 16S rRNA gene sequences among different Trichodesmium populations (Janson et al., Citation1999; Hynes et al., Citation2012; Díez et al., Citation2012; Bergman et al., Citation2013) is generally higher than among the benthic populations of Hydrocoleum (96–99%) and are nested within a broader cluster of Hydrocoleum populations. This arrangement suggests that the planktonic niche is a more specialized one that may have derived from benthic populations, which are characterized by a wider genetic variability. The separation between benthic and planktonic niches within this cluster, which is presently well established, may have taken place in the not too distant past (Abed et al., Citation2006), but the timing needs to be determined.
Janson et al. (Citation1999) have determined that morphologically very different species of Trichodesmium were closely related regarding both 16S rRNA and hetR-based phylogenetic analyses. Lungren et al. (2005) and Hynes et al. (Citation2012) confirmed these observations using a larger number of natural populations and comparing morphology and phylogeny of cultured Trichodesmium with natural populations, respectively. Refinement in molecular distinction correlating closer to phenotypic expression among Trichodesmium morphotypes has been achieved using HIP1 fingerprinting (Orcutt et al., Citation2002). Recent studies of the whole genomes of marine picoplanctic cyanobacteria have confirmed the limited resolution power of the 16S rRNA gene sequences to identify ecotypes associated with light-utilization niches (Six et al., Citation2007; Dufresne et al., Citation2008).
Both Hydrocoleum and Trichodesmium are active and important contributors to dinitrogen fixation in the ocean, but show differences in timing of nitrogenase activity. The studied Hydrocoleum populations fix nitrogen by night, similar to other non-heterocystous benthic and mat-forming diazotrophs, such as Plectonema boryanum Gomont 1899 and Lyngbya majuscula Harvey ex Gomont Citation1892 (Lundgren et al., Citation2003). In contrast, Trichodesmium populations are most efficient in fixing nitrogen by day, similar to heterocystous cyanobacteria in timing but different in location of nitrogenase activity (Berman-Frank et al., Citation2001). Thus, like among heterocystous cyanobacteria, carbon and nitrogen fixation activities in Trichodesmium are separated in space, while the Hydrocoleum populations resort to a less efficient separation in time between their nitrogenase activity and oxygenic photosynthesis, adding an important distinction between these taxa.
Populations of Hydrocoleum form exclusively benthic colonies, such as mats, cushions and tufts, whereas those of Trichodesmium form floating spindle-shaped and radiating colonies (Capone et al., Citation1997) while occupying strictly planktonic niches in tropical oceans. The persistent planktonic mode of life in Trichodesmium is supported by intracellular packages of gas vesicles, which are lacking in Hydrocoleum, with the exception of small refractive inclusions observed occasionally in differentiated terminal cells of Hydrocoleum and some other benthic cyanobacteria (e.g. Pseudanabaena Lauterborn, 1915). The presence of genes coding for gas vesicle formation (e.g. Tandeau de Marsac et al., Citation1985) requires further study.
In the past, cyanobacteria obtained from new types of environments or geographic locations (e.g. tropical marine waters) were not recognized as new taxa, being forced into existing classification systems based on similar morphology (Richert et al., Citation2006). Based on our observations, we support the continued taxonomic distinction between the genera Hydrocoleum and Trichodesmium regardless of the close similarity of their 16S rRNA genes signatures, because they are characterized by distinct habitats, ecological roles and differently expressed phenotypes.
Taxonomic evaluation of the genera Hydrocoleum and Blennothrix
The relationships among the genera Hydrocoleum, Blennothrix and Trichodesmium have been rearranged in the recent revision of the system of filamentous cyanobacteria as proposed by Anagnostidis & Komárek (Citation1988), which entered the new determination manual for cyano-prokaryotes (Komárek & Anagnostidis, Citation2005), a formal procedure that is not supported by the results of this study. According to their taxonomic revision, the non-heterocystous cyanobacteria of Section III in the previously most frequently used system of Rippka et al. (Citation1979), nominally known as the order Oscillatoriales Elenkin Citation1934, was subdivided into six families, whereby the morphospecies of the three genera presently under study were placed in two separate families. According to the system of Komárek & Anagnostidis (Citation2005), the genus Trichodesmium and five species of Hydrocoleum were placed in a new family Phormidiaceae, characterized by a cell division cycle that is completed one at a time. Other Hydrocoleum species, including the marine forms presently under study, were reclassified as Blennothrix Kützing ex Anagnostidis & Komárek Citation1988, and placed into the revised family Oscillatoriaceae, in which they included forms with cell division ‘in rapid sequence’ which starts several subsequent cell division cycles by initiating construction of several cross-walls before the initial cell division was concluded by a complete cross-wall closure (Komárek & Anagnostidis, Citation2005, p. 32. fig. 1D and fig. 859 on p. 575).
We have analysed freshwater populations of Blennothrix ganeshii Watanabe & Komárek, collected in 2004 in two rivers in Mexico and found them to conform closely to the original description (Watanabe & Komárek, Citation1989). The TEM demonstrated that the Blennothrix we studied did indeed exhibit multiple initiations of cross-wall closures (, , numbered 1–3), as did the TEM images in the original description of this organism (loco citato, p. 76, fig. 6a), a pattern suggesting a temporary polyploidy during the cell division process. In contrast, the marine morphospecies of Hydrocoleum that we studied, including the large forms with very short cells, showed a regular cell division rhythm, with each cell division cycle completed before the next one was initiated. Thus, the proposed reclassification of the studied marine species of Hydrocoleum under the name Blennothrix was not supported by our results, and the taxonomic value of the distinction in cell division and the associated cross-wall initiation requires further study.
The 16S rRNA sequences of the compared marine and freshwater morphospecies placed Blennothrix separately from the Trichodesmium/Hydrocoleum cluster, which further supported the genotypic distinction between the compared taxa, as well as the fact that they also differ in morphology and ecology. In addition, the Blennothrix cluster also contained the sequence of the type species Blennothrix vermicularis Kützing obtained from the exsiccatum of the herbarium NHN. According to our results, the traditional taxonomic treatment of the benthic mat-forming cyanobacteria within the genus Hydrocoleum Kützing ex Gomont appears to be fully supported by morphological as well as by genotypic criteria, which calls for a brief historical review.
The cyanobacterial genera Hydrocoleum and Blennothrix were described by Friedrich Traugott Kützing in ‘Phycologia Generalis’ (1843) with the species: H. homoeotrichum, and H. heterotrichum described from freshwater cataracts, and B. vermicularis from the marine coast of the Gulf of Naples. Kützing described the marine species Hydrocoleum lyngbyaceum in his compendium ‘Species Algarum’ (Citation1849). Gomont (Citation1892) included Kützing’s species in his ‘Monographie des Oscillariées’ with an additional five marine and two freshwater species of Hydrocoleum, but he considered Kützing’s Blennothrix vermicularis to be a variant of H. glutinosum (C. Agardh) Gomont. Our results confirmed the original descriptions by Kützing.
Our practice of extracting DNA from historic herbarial exsiccata enabled the excellence of some historical morphotype characterizations to be illustrated (see Palinska et al., Citation2006), and thus to resolve a long standing taxonomic dispute, while testing the validity of more recent taxonomic arrangements. It may now be considered that Maurice Gomont (Citation1892) in a source that has been accepted as a botanical ‘later starting point’ for Cyanophyceae or cyanobacteria had placed all non-heterocystous filamentous cyanobacteria (Nostocacées homocystées) in one family (Oscillatoriées), later named Oscillatoriaceae (Gray) Kirchner 1900. Gomont, like many subsequent authors, placed Trichodesmium together with Oscillatoria in the tribus ‘Lyngbyaeae’, characterized by single trichomes within a sheath or without any sheath. He placed the genus Hydrocoleum in the other tribus ‘Vaginarieae’ together with other filamentous cyanobacteria with more than one trichome within a common sheath. His scheme has served reasonably well for more than a century, but now deserves to be re-evaluated with the available modern methods and approaches.
In conclusion, the proposed recent reclassification of Hydrocoleum forms under the name Blennothrix is not supported by either our observations or by gene sequence analyses performed on herbarium, cultured and fresh material. Therefore we insist that the Kützing’s generic description of Hydrocoleum including typical marine forms as H. lyngbyaceum, H. glutinosum, H. coccineum, H. cantharidosmum and H. majus remains valid. The genus Hydrocoleum was not listed in the last edition of Bergey’s Manual. It was mentioned, however, with the request that its validity be confirmed using cultured and uncultured populations (Castenholz et al., Citation2001). Here, we provide morphological, ultrastructural and molecular data for the representatives of this genus, which should justify its future place in bacteriological as well as in botanical systematics.
AUTHOR CONTRIBUTIONS
KP: original concept, experiments, writing manuscript, RMMA: molecular work; LC and M-JL: sampling N2 fixation, YB-M: data on Blennothrix; SG: statistics, population identification, drafting manuscript.
ACKNOWLEDGEMENTS
We very much appreciate the corrections, suggestions and stimulating discussion by two anonymous reviewers.
Additional information
Funding
REFERENCES
- Abed, R.M.M., Golubic, S., Garcia-Pichel, F., Camoin, G. & Seong-Joo, Lee (2003a). Identity and speciation in marine benthic cyanobacteria – the Phormidium complex. Algological Studies, 109: 35–56.
- Abed, R.M.M., Golubic, S., Garcia-Pichel, F., Camoin, G.F. & Sprachta, S. (2003b). Characterizaton of microbialite-forming Cyanobacteria in a tropical lagoon: Tikehau Atoll, Tuamotu, French Polynesia. Journal of Phycology, 39: 862–873.
- Abed, R.M.M., Palinska, K.A., Camoin, G. & Golubic, S. (2006). Common evolutionary origin of planktonic and benthic nitrogen-fixing oscillatoriacean cyanobacteria from tropical oceans. FEMS Microbiology Letters, 260: 171–177.
- Anagnostidis, K. & Komárek, J. (1988). Modern approach to the classification system of Cyanophytes 3 – Oscillatoriales. Archiv fur Hydrobiologie, Supplement, 80: 1–40.
- Bergman, B., Sandh, G., Lin, S., Larsson, J. & Carpenter, E.J. (2013). Trichodesmium- – a widespread marine cyanobacterium with unusual nitrogen fixation properties. FEMS Microbiology Review, 37: 286–302.
- Berman-Frank, I., Lundgren, P., Chen Y-B., Küpper, H., Kolber, Z., Bergman, B. & Falkowski, P. (2001). Segregation of nitrogen fixation and oxygenic photosynthesis in the marine cyanobacterium Trichodesmium. Science, 294: 1534–1537.
- Capone, D.G., Zehr, J.P., Pearl, H.W. & Bergman, B. (1997). Trichodesmium, a globally significant marine cyanobacterium. Science, 261: 1221–1229.
- Castenholz, R.W., Rippka, R., Herdman, M. & Wilmotte, A. (2001). Subsection III. (Formerly Oscillatoriales Elenkin 1934). In Bergey’s Manual of Systematic Bacteriology (Garrity, G.M., editor), 539–562. 2nd ed. Springer-Verlag, New York.
- Charpy, L., Alliod, R., Rodier, M. & Golubic, S. (2007). Benthic nitrogen fixation in the SW New Caledonia lagoon. Aquatic Microbial Ecology, 47: 73–81.
- Charpy, L., Palinska, K.A, Casareto, B., Langlade, M.-J., Suzuki, Y., Abed, R.M.M. & Golubic, S. (2010). Dinitrogen-fixing Cyanobacteria in microbial mats of two shallow coral reef ecosystems. Microbial Ecology, 59: 174–186.
- Charpy, L., Casareto, B.E., Langlade, M.J. & Suzuki, Y. (2012a). Cyanobacteria in coral reef ecosystems: a review. Journal of Marine Biology, doi:10.1155/2012/259571.
- Charpy, L., Palinska, K.A, Abed, R.M.M., Langlade, M.J. & Golubic, S. (2012b). Factors influencing microbial mat composition, distribution and dinitrogen fixation in three western Indian Ocean coral reefs. European Journal of Phycology, 47: 51–66.
- Díez, B., Bergman, B., Pedrós-Alió, C., Antó, M. & Snoeijs, P. (2012). High cyanobacterial nifH gene diversity in Arctic seawater and sea ice brine. Environmental Microbiology Reports, 4: 360–366.
- Dufresne, A., Ostrowski, M., Scanlan, D., Garczarek, L., Mazard, S., Palenik, B.P., Paulsen, I.T., Tandeau de Marsac, N., Wincker, P., Dossat, C., Ferriera, S., Johnson, J., Post, A.F., Hess, W.R. & Partansky, F. (2008). Unraveling the genomic mosaic of a ubiquitous genus of marine cyanobacteria. Genome Biology, 9: R90, 1–16. doi:10.1186/gb-2008-9-5-r90.
- Elenkin, A.A. (1934). Ob osnovnych principach sistemy Cyanophyceae. Sov. Bot., 5: 51–83.
- Engene, N., Paul, V.J., Byrum, T., Gerwick, W.H., Thor, A. & Ellisman, M.H. (2013). Five chemically rich species of tropical marine cyanobacteria of the genus Okeania gen. nov. (Oscillatoriales, Cyanoprokaryota). Journal of Phycology, 49: 1095–1106.
- Finzi-Hart, J.A., Pett-Ridge, J., Weber, P.K., Popa, R., Fallon, S.J., Gunderson, T., Hutcheon, I.D., Nealson, K.H. & Capone, D.G. (2009). Fixation and fate of C and N in the cyanobacterium Trichodesmium using nanometer-scale secondary ion mass spectrometry. Proceedings of the National Academy of Sciences, USA, 106: 6345–6350.
- Foster, J.S., Green, S.J., Ahrendt, S.R., Golubic, S., Reid, R.P., Hetherington, K.L. & Bebout, L. (2009). Molecular and morphological characterization of cyanobacterial diversity in the stromatolites of Highborne Cay, Bahamas. International Society for Microbial Ecology Journal, 3: 573–587.
- Fredriksson, C. & Bergman, B. (1997). Ultrastructural characterisation of cells specialised for nitrogen fixation in a non-heterocystous cyanobacterium, Trichodesmium spp. Protoplasma, 197: 76–85.
- Geitler, L. (1932). Cyanophyceae. In Dr L. Rabenhorst’s Kryptogamen-Flora von Deutschland, Osterreich und der Schweiz, vol. XIV. Akademischer Verlag, Leipzig (1985 reprint: Königstein Koeltz Scientific Books).
- Golubic, S. (1977). Speciation in Trichodesmium: occupation of all oceanic pelagic niches. Schweitz Zeitschrift Hydrology, 39: 141–143.
- Golubic, S., Hernandez-Marine, M. & Hoffmann, L. (1996). Developmental aspects of branching in filamentous Cyanophyta/Cyanobacteria. Algological Studies, 83: 303–329.
- Golubic, S., Abed, R.M.M., Palinska, K., Paullac, S., Chinain, M. & Laurent, D. (2009). Marine toxic cyanobacteria: diversity, environmental responses and hazards. Toxicon, 56: 836–841.
- Golubic, S., Hernandez-Marine, M. & Hoffmann, L. (1996). Developmental aspects of branching in filamentous Cyanophyta/Cyanobacteria. Algological Studies, 83: 303–329.
- Gomont, M. (1892). Monographie des Oscillariées, part 1. Annales des Sciences Naturelles, Botanique, Série 7, 15, 263–368.
- Hašler, P., Dvořák, P., Johansen, J.R., Kitner, M., Ondřej, V. & Poulíčková, A. (2012). Morphological and molecular study of epipelic filamentous genera Phormidium, Microcoleus and Geitlerinema (Oscillatoriales, Cyanophyta/Cyanobacteria). Fottea, 12: 341–358.
- Hynes, A.M., Webb, E.A., Doney, S.C. & Waterbury, J.B. (2012). Comparison of cultured Trichodesmium (Cyanophyceae) with species characterized from the field. Journal of Phycology, 48: 196–210.
- Irwin, D.E., Irwin, J.H. & Price, T.D. (2001). Ring species as bridges between microevolution and speciation. Genetica, 112–113:223–243.
- Janson, S., Bergman, B., Carpenter, E.J., Giovannoni, S.J. & Vergin, K. (1999). Genetic analysis of natural populations of the marine diazotrophic cyanobacterium Trichodesmium. FEMS Microbiology Ecology, 30: 57–65.
- Komárek, J. & Anagnostidis, K. (2005). Cyanoprokaryota, 2. Teil Oscillatoriales. In Süsswasserflora von Mitteleuropa, Band 19/2 (Büdel, B., Gärtner, G., Krienitz, L. & Schagerl, M., editors), 1–757. M. München: Elsevier Spectrum Akademischer Verlag, Munich.
- Kützing, F.T. (1843). Phycologia generalis: oder, Anatomie, Physiologie und Systemkunde der Tange. F.A. Brockhaus, Leipzig.
- Kützing, F.T. (1849). Species Algarum. F.A. Brockhaus, Leipzig.
- Laurent, D., Kerbrat, A.S., Darius, H.T., Rossi, F, Yeeting, B., Haddad, M., Golubic, S., Pauillac, S. & Chnain, M. (2012). Siguatera shellfish poisoning (CSP): a new ecotoxicological phenomenon from cyanobacteria to humans via giant clams. In Food Chains: New Research, (Jensen, M.A. & Muller D.W., editors), 1–11. Nova Science Publishers, New York.
- Ludwig, W., Strunk, O., Klugbauer, S., Klugbauer, N., Weizeneger, M., Neumaier, J., Bachleitner, M. & Schleifer, K.H. (1998). Bacterial phylogeny based on comparative sequence analysis. Electrophoresis, 19: 554–568.
- Lundgren, P., Bauer, K., Lugomela, C., Söderbäck, E. & Bergman, B. (2003). Reevaluation of the nitrogen fixation behavior in the marine non-heterocystous cyanobacterium Lyngbya majuscula. Journal of Phycology, 39: 310–314.
- Lundgren, P., Janson, S., Jonasson, S., Singer, A. & Bergmen, B. (2005). Unveiling of novel radiations within Trichodesmium cluster by hetR gene sequence analysis, Applied and Environmental Microbiology, 71: 190–196.
- Méjean, A., Peyraud-Thomas, C., Kerbrat, A-S., Golubic, S., Pauillac, S., Chinain, M. & Laurent, D. (2009). First identification of the neurotoxins anatoxin-a and homoanatoxin-a from Hydrocoleum lyngbyaceum (marine cyanobacterium) possibly linked to a giant clam poisoning in New Caledonia. Toxicon, 56: 829–835.
- Moritz, C., Schneider, C.J. & Wake, D.B. (1992). Evolutionary relationships within the Ensatina eschscholtzii complex confirm the ring species interpretation. Systematic Biology, 41: 273–291.
- Mulholland, M.R. (2004). The fate of nitrogen fixed by diazotrophs in the ocean. Biogeosciences, 4: 37–51.
- Nübel, U., Garcia-Pichel, F. & Muyzer, G. (1997). PCR primers to amplify 16S rRNA genes from cyanobacteria. Applied and Environmental Microbiology, 6: 3327–3332.
- Orcutt, K.M., Rasmussen, U., Webb, E.A., Waterbury, J.B., Gundersen, K. & Bergman, B. (2002). Characterization of Trichodesmium spp. by genetic techniques. Applied and Environmental Microbiology, 68: 2236–2245.
- Palinska, K.A., Thomasius, C.F., Marquardt, J. & Golubic, S. (2006). Phylogenetic evaluation of cyanobacteria preserved as historic herbarium exsiccata. International Journal of Systematic and Evolutionary Microbiology, 56: 2253–2263.
- Palinska, K.A. & Surosz, W. (2008). Population of Aphanizomenon from the Gulf of Gdansk (Southern Baltic Sea): differences in phenotypic and genotypic characteristics. Hydrobiology, 607: 163–173.
- Palinska, K.A., Abed, R.M.M., Wendt, K., Charpy, L., Lotocka, M. & Golubic, S. (2012). Opportunistic Cyanobacteria in benthic microbial mats of a tropical lagoon, Tikehau Atoll, Tuamotu Archipelago: minor in natural populations, major in cultures. Fottea, 12: 127–140.
- Paul, V.J., Thacker, R.W., Banks, K. & Golubic, S. (2005). Benthic cyanobacterial bloom impact the reefs of South Florida (Broward County, USA). Coral Reefs, 24: 693–697.
- Prüsse, E., Quast, C., Knittel, K., Fuchs, B.M., Ludwig, W., Peplies, J. & Glöckner, F.O. (2007). SILVA: a comprehensive online resource for quality checked and aligned ribosomal RNA sequence data compatible with ARB. Nucleic Acids Research, 35: 7188–7196.
- Richert, L., Golubic, S., de le Gue, R., Herve, A. & Payri, C. (2006). Cyanobacterial populations that build ‘kopara’ microbial mats in Rangiroa, Tuamotu Archipelago, French Polynesia. Eur. J. Phycol, 41: 259–279.
- Rippka, R., Deruelles, J., Waterbury, J.B., Herdman, M. & Stanier, R.Y. (1979). Generic assignments, strain histories and properties of pure cultures of cyanobacteria. Journal of General Microbiology, 111: 1–61.
- Rippka, R., Coursin, T., Hess, W., Lichtlé, C., Scanlan, D.J., Palińska, K.A., Iteman, I., Partensky, F., Houmard, J. & Herdman, M. (2000). Prochlorococcus marinus Chisholm et al., 1992, subsp. nov. pastoris, strain PCC 9511, the first axenic chlorophyll a2/b2 marine, chlorophyll b-containing cyanobacterium (Oxyphotobacteria). International Journal of Systematic and Evolutionary Microbiology, 50: 1833–1847.
- Siegesmund, M.A., Johansen, J.R., Karsten, U. & Friedl, T. (2008) Coleofasciculus gen. nov. (cyanobacteria): morphological and molecular criteria for revision of the genus Microcoleus Gomont. Journal of Phycology, 44: 1572–1585.
- Six, C., Thomas, J.C., Garczarek, L., Ostrowski, M., Dufresne, A., Blot, N., Scanlan, D.J. & Partensky, F. (2007). Diversity and evolution of phycobilisomes in marine Synechococcus spp.: a comparative genomics study. Genome Biology, 8: R259. doi:10.1186/gb-2007-8-12-r259.
- Stackebrandt, E. & Ebers, J. (2006). Taxonomic parameters revisited: tarnished gold standards. Microbiology Today, 33: 152–155.
- Strunecký, O., Elster, J. & Komárek, J. (2011). Taxonomic revision of the freshwater cyanobacterium “Phomidium” murrayi = Wilmottia murrayi. Fottea, 11: 57–71.
- Strunecký, O., Komárek, J., Johansen, J., Lukesová, A. & Elster, J. (2013). Molecular and morphological criteria for revision of the genus Microcoleus (Oscillatoriales, cyanobacteria). Journal of Phycology, 49: 1167–1180.
- Szmant, A.M. (2001). Why are coral reefs world-wide becoming overgrown by algae? “Algae, algae everywhere, and nowhere a bite to eat!”. Coral Reefs, 19: 299–302.
- Tandeau de Marsac, N. (1977). Occurrence and nature of chromatic adaptation in Cyanobacteria. Journal of Bacteriology, 130: 82–91.
- Tandeau de Marsac, N., Mazwl, D., Bryant, D.A. & Houmard, J. (1985). Molecular cloning and nucleotide sequence of a developmentally regulated gene from the cyanobacterium Calothrix PCC 7601: a gas vesicle protein gene. Nucleic Acid Research, 13: 7223–7236.
- Taton, A., Grubisic, S., Brambilla, E., De Wit, R. & Wilmotte, A. (2003). Cyanobacterial diversity in natural and artificial microbial mats of Lake Fryxell (McMurdo Dry Valleys, Antarctica): a morphological and molecular approach. Applied and Environmental Microbiology, 69: 5157–5169.
- Templeton, A.R. (1989). The meaning of species and speciation: a genetic perspective. In Speciation and its Consequences (Otte, D. & Endler, J.A., editors), 3–27. Sinauer, Sunderland, MA.
- Turicchia, S., Ventura, S., Komárková, J. & Komárek, J. (2009). Taxonomic evaluation of cyanobacteria microflora from alkaline marches of northern Belize. 2. Diversity of oscillatorialean genera. Nova Hedwigia, 89: 165–200.
- Van Baalen, C. & Brown, M.R. (1969). The ulrastructure of the marine blue green alga, Trichodesmium erythraeum, with special reference to the cell wall, gas vacuoles, and cylindrical bodies. Archives für Mikrobiologie, 69: 79–91.
- Watanabe, M. & Komárek, J. (1989). New Blennothrix-species (Cyanophyceae/Cyanobacteria) from Nepal. Bulletin of the National Science Museum Series B, Botany, 15: 67–79.
- Zehr, J.P. & McReynolds, L.A. (1989). Use of degenerate oligonucleotides for amplification of the nifH gene from the marine cyanobacterium Trichodesmium spp. Applied and Environmental Microbiology, 55: 2522–2526.