ABSTRACT
Given the growing body of evidence on the general decline of kelp beds worldwide, it is crucial to understand the physiological responses of kelp gametophyte stages to environmental parameters. We investigated the physiological responses to light and temperature of gametophytes from two populations of Laminaria digitata in contrasting environments along the French coast of the English Channel. Gametophytes of both populations were highly tolerant of high light through an efficient down-regulation of photosynthesis triggered by the activation of the xanthophyll cycle. Temperature increases promoted photosynthesis and photosystem II showed high resistance to short-term exposure to high temperatures currently encountered in the field. Gametophytes from the two sites displayed some differences in their pigment content and photosynthetic characteristics, but low replication and difference in time of sampling precluded tests of potential local adaptation to the light conditions at each site, as observed in previously published results on adult sporophytes. Gametophytes of L. digitata appeared to be resistant to irradiation and temperature conditions currently experienced in the field, confirming their role in persistence of kelp species under stressful environmental conditions.
INTRODUCTION
Kelps are major structural components of cold temperate and boreal coastal communities (Lüning, Citation1990). They form a highly productive habitat (Mann, Citation1973) that harbours a rich biodiversity (Christie et al., Citation2003). The ecological role of kelp is threatened by their expected vulnerability to changes in the physical environment caused by rapid climate change (Wernberg et al., Citation2011a; Raybaud et al., Citation2013) or extreme events (Wernberg et al., Citation2013), especially near range edges where populations are on the front line of environmental change (Wernberg et al., 2011b).
The persistence of kelp forests mainly depends on recruitment, growth, competitiveness and physiological tolerance to environmental factors of the microscopic stage (Reed & Foster, Citation1984; Ladah & Zertruche-González, Citation2007; Matson & Edwards, Citation2007), which is the crucial phase of the complex heteromorphic life cycle of kelp (Sauvageau, Citation1915). Gametophytes and embryonic sporophytes can delay their development and reproduction for several months (Carney, Citation2011) until favourable conditions occur that therefore promote rapid recruitment (Edwards, Citation2000). Kelp microscopic stages are subject to environmental conditions that differ from those experienced by the macroscopic sporophytes (Reed & Foster, Citation1984; Martinez & Santelices, Citation1998) and can therefore have different physiological optima and tolerance levels (Hanelt et al., Citation1997; Altamirano et al., Citation2004; Matson & Edwards, Citation2007). Even under similar environmental conditions, the response of haploid stages differs from those of diploid stages among various macroalgae (Roleda et al., Citation2008; Wang et al., Citation2011). Hence, in the context of environmental change, enhancing the understanding of the physiological tolerance of kelp must incorporate the study of all life-stages, including microscopic phases (Harley et al., Citation2012; Wernberg et al., Citation2012).
Among potentially adverse environmental conditions, light and temperature can greatly affect the development and survival of microscopic stages, the vulnerability of which generally determines the ecological success of the species (Bartsch et al., Citation2008). Extremely high light levels have been shown to be adverse for the development and viability of post-settlement stages (gametophytes and embryonic sporophytes: Lüning & Neushul, Citation1978; Fejtek et al., Citation2011). Likewise, extreme high-temperature events (such as El Niño events) have been shown to affect the reproductive success of gametophytes (Ladah & Zertruche-González, Citation2007; Oppliger et al., Citation2012).
The photosynthetic apparatus is one of the main targets of these abiotic stressors (Walters, Citation2005). Rapid fluctuation of light and temperature can cause disruptive stresses (Davison & Pearson, Citation1996) and affect the survival of kelp gametophytes. Drastic and rapid changes in light exposure and temperature are frequently experienced within a single day (Gevaert et al., Citation2003; Delebecq et al., Citation2011) and especially during sunny spring tides.
Therefore, the ability to withstand stressful conditions and to recover from them is crucial for preventing damage to the photosynthetic apparatus and maintaining sufficient photosynthetic performance. After the onset of stressful conditions, the regulation of energy absorption and utilization is essential (Raven & Geider, Citation2003). Photoinhibition is the down-regulation of photosynthesis, the extent of which is determined by the balance between the rate of photodamage and the rate of repair of photosystem II (PSII) (Takahashi & Murata, Citation2008). Photoinhibition has been observed in the field for macroscopic sporophyte stages (Gevaert et al., Citation2003; Delebecq et al., Citation2011), in the laboratory on zoospores (Roleda, Citation2009) and in the gametophytic and embryonic sporophyte stages of kelp (Hanelt et al., Citation1997; Altamirano et al., Citation2004). Factors other than light, such as temperature, can accelerate photoinhibition by altering the PSII repair mechanisms (Takahashi & Murata, Citation2008). Toxic active derivatives of oxygen (oxygen radicals), by-products of photosynthesis, can also be over-produced under adverse environmental conditions (when concentrations exceed the scavenging potential of cells) and can alter the biological integrity of cells (Ledford & Niyogi, Citation2005; Allakhverdiev et al., Citation2008).
To cope with excess light, energy is dissipated in the form of heat to rapidly regulate light harvesting; this mechanism is widespread in photoautotrophs (Raven & Geider, Citation2003). Increased thermal energy dissipation of excess light involves the xanthophyll cycle in brown algae, which plays a major role in the fast-dynamic acclimation to change in light, temperature and desiccation in macroscopic sporophytes (Fernández-Marin et al., Citation2011). However, the role of the xanthophyll cycle in the photoprotection process in kelp gametophytes has only been mentioned (Hanelt et al., Citation1997; Altamirano et al., Citation2004), without being clearly demonstrated.
Laminaria digitata (Hudson) Lamouroux (1813), a kelp species with high ecological and economic value, has been shown to be retreating from several sites along the French coasts (Arzel, Citation1998; Davoult et al., Citation2011), sparking research to determine the reasons for this decline. Physical stress and environmental changes may contribute to a reduction in the fitness of the gametophytic developmental stages of L. digitata. In this study, we tested the sensitivity of L. digitata gametophytes to changing photon flux density and temperature. The investigations were carried out on two populations of L. digitata along the French coast of the English Channel inhabiting contrasting environmental conditions (Delebecq et al., Citation2013). The differences in local environmental conditions may result in different sensitivities among the populations that need to be taken into account in understanding the effect of environmental factors on organisms. In this study, we also measured the role of the xanthophyll cycle in the non-photochemical quenching in gametophytes of L. digitata in response to excess light.
MATERIALS AND METHODS
Study site
A complete description of the two sites and environmental conditions during experiments is given in Delebecq et al. (Citation2013); consequently, we describe only the main characteristics of both sites here. We collected the seaweed material from two populations of L. digitata, growing in the upper subtidal zone (0–1 m) of a rocky shore at Roscoff (48°5′ N, 3°6′ W) and at Wissant (50°5′ N, 1°4′ E), located in the western and eastern parts of the English Channel, respectively. The sites were selected for their large kelp stands and also for their contrasting environmental conditions. Light attenuation (m−1) of photosynthetically active radiation (PAR, 400–700 nm) ranged from 0.09 to 0.57 m−1 at Roscoff and from 0.19 to 0.96 m−1 at Wissant (Delebecq et al., Citation2013) due to high turbidity in the eastern English Channel. Seawater surface temperature displays high seasonal variation at both sites, with a slightly broader annual temperature range at Wissant, from 5°C in winter to 20°C in summer, than at Roscoff, from 8°C in winter to 17°C in summer, due to the influence of the North Atlantic Ocean and depth of the continental shelf. Environmental conditions at the two sites at the time of collection and one month prior to sampling are given in .
Table 1. Environmental conditions at the two sites at the time of collection and one month prior to sampling.
Sampling and culture conditions
Fertile sporophytes of L. digitata were collected at low tide in November 2008 at Roscoff, and in February 2009 at Wissant. Laminaria digitata is reproductive most of the year at Roscoff, but the main spore/gamete release events generally occur in August–September and November–December in Northern Brittany (Arzel, Citation1998). At Wissant, L. digitata is reproductive at the end of winter and throughout spring. Mature sori were cut, cleaned, and dried at 10°C in the dark for several hours. Sori were subsequently washed with distilled water and sterile seawater, and placed in 50 ml Falcon tubes (BD Biosciences, San Jose, California), filled with sterile Provasoli enriched seawater (PES) (Provasoli, Citation1968), and maintained overnight in the dark on a rotary shaking table to induce zoospore release. Zoospores in suspension were checked under an inverted microscope to ensure that zoospores were viable, before being placed in Petri dishes (BD Falcon, Franklin Lakes, New Jersey). Zoospores were allowed to settle and developing gametophytes were cultured in a constant temperature chamber at 10°C under an irradiance of 35 µmol photons m−2 s−1 (photon flux density, PFD, 400–700 nm), produced by fluorescent tubes (L8W/840, cool white, Osram, Germany) in a light:dark cycle of 12:12 h. PFD was measured with a cosine-corrected quantum sensor (Li-192SA, LiCor, Lincoln, Nebraska), connected to a data logger (Li 1400, LiCor, Lincoln, Nebraska). The medium was changed once a week. Experiments started after approximately 1 month of cultivation when the density was high enough for fluorescence measurements. The density of the gametophytes was 4711 ± 1140 individuals (ind) m−2 for Roscoff (43 ± 3% cover) and 3600 ± 691 ind m−2 for Wissant (41 ± 7% cover). Gametophytes were composed of few cells and arranged as a thick homogeneous layer on the bottom of the Petri dishes. Gametophytes were isolated from three different parents at each site (n = 3) and were cultivated separately to ensure independent replicates.
Oxygen production
Dark respiration (Rd) and net oxygen production (NP) were measured polarographically at the culture temperature (10°C), using a water-jacket thermostatic DW2/2 chamber combined with a ‘Clark-type’ oxygen electrode and a computer-interfaced box CB1 (Hansatech Instruments, Kings Lynn, Norfolk, UK). The gametophytes were first detached from the substratum and resuspended several days prior to the experiment. Detaching gametophytes from their substratum does not affect their growth, their photosynthetic performance or their respiration (Fain & Murray, Citation1982). The gametophytes were held for 12 h in the dark prior to the experiment, and then placed in the measurement chamber filled with 2 ml of PES and mixed with a magnetic stirrer. To prevent oxygen saturation, a quarter of the medium was renewed after each light step. To do so, a silk filtering mesh was used to retain the gametophytes in the media. Respiration was measured in the dark, and gametophytes then underwent increasing irradiance steps of 20 min each (13 light steps, ranging from 2.5 to 500 µmol photons m−2 s−1) using a halogen lamp (KL 2500 LCD, Schott, Germany) with a daylight cut-off filter (Schott, Germany). Rd, NP and gross oxygen production (GP) rates were calculated based on fresh weight (FW, µmol O2 gFW−1 h−1) and chlorophyll a content (chl a, µmol O2 nmoles chl a−1 h−1). FW was measured after collecting gametophytes on a silk filtering mesh that had previously been weighed.
Chlorophyll fluorescence
In vivo chl a fluorescence of photosystem II (PSII) of gametophytes was measured using an underwater fluorometer (diving PAM; Heinz Walz, Effeltrich, Germany). The optimal quantum yield (Fv/Fm) of PSII (Genty et al., Citation1989), a measure of the maximum efficiency of PSII, was measured using a 0.8 s saturating pulse (2500 µmol photons m−2 s−1) of white light. We calculated the relative Fv/Fm ratio (rel. Fv/Fm) by dividing all data by the initial value measured at the beginning of the experiment in the dark.
The effective quantum yield of PSII (фPSII), the efficiency of PSII photochemistry, was measured using a custom-made clip for Petri dishes to ensure a constant distance of 5 mm between the probe and the sample. The фPSII was calculated according to Genty et al. (Citation1989) and used to estimate the linear electron transport rate (relative electron transport rate, rETR) (Gevaert et al., Citation2003), an estimator of photosynthesis.
Non-photochemical quenching (NPQ) indicates thermal dissipation of excess light in the PSII antennae, a photoprotective mechanism. We assumed that a stable NPQ level is reached after a 10-min illumination period, as shown in several microalgal species (Casper-Lindley & Björkman, Citation1998). Fm’ values measured under very weak irradiance were higher than Fm values measured after dark-adaptation; therefore NPQ values were computed using the higher Fm’ value instead of Fm (Serôdio et al., Citation2005).
rETR and NPQ were measured on gametophytes at the end of each light-increasing step of 10 min each (12 light steps, ranging from 2.5 to 250 µmol photons m−2 s−1). The NPQ value measured under the maximal irradiance is referred to as NPQ250.
P-I curves, rETR-I
The light-saturated maximum rate of GP (Pmax), the light-saturated maximum rate of relative electron transfer (rETRmax), the light-limited initial slope (α), and the saturation onset irradiance level (Ik) were calculated by plotting computed oxygen production rates and rETR against irradiance. Pmax represents the maximal oxygen production, including all photosynthetic processes, while rETRmax is an estimation of the linear electron transfer in PSII, an indication of the overall photosynthetic capacity. Data were fitted using the model of Eilers & Peeters (Citation1988) to each replicate with a least-square regression, using the Simplex method in the Statistica computer package (Statsoft, Tulsa, Oklahoma).
Response to high irradiance
To study high light stress, the settled gametophytes in a Petri dish filled with PES at cultivation temperature were exposed to 500 µmol photons m−2 s−1 for 2 h, and then to dim light (10 µmol photons m−2 s−1) to allow recovery. Fv/Fm, фPSII, rETR and NPQ were measured every 30 min. Samples for pigment analyses were simultaneously collected at the start of the experiment, at the end of the light stress, and 2 h after the return to dim light. Gametophytes were detached from the substratum and the gametophyte suspension was filtered on a 20 µm silk filtering mesh and subsequently deep-frozen in liquid nitrogen for further pigment analyses.
Response to temperature
First, rETR-I curves were constructed for gametophytes acclimated for 4 h at four different temperatures (5°C, 10°C, 15°C and 20°C) controlled by a thermo-fluid circulator bath. During acclimation, Fv/Fm was measured hourly. Then, gametophytes underwent a progressive increase or decrease in temperature (2°C every 15 min) with an initial temperature set at 10°C. Fv/Fm was measured at the end of each temperature step.
Pigment analysis
Pigment content of gametophytes was extracted by sonication and grinding in a cold mortar with methanol and methylene chloride. Extracts were centrifuged and supernatants were collected and dry-evaporated under nitrogen. Salt contents of the extracts were removed and the organic phase was evaporated and dissolved in methanol for injection. Pigment analysis was performed by high performance liquid chromatography (HPLC) (Beckman, system Gold, 126) with a reverse-phase column (C 18 Allure, Restek). Separation was performed with a solvent delivery profile adapted from Arsalane et al. (Citation1994). Pigment contents were quantified using specific absorption coefficients and normalized to the total pigment content. The conversion of violaxanthin into antheraxanthin and zeaxanthin was estimated by calculating the de-epoxidation ratio (DR):
DR = (antheraxanthin + zeaxanthin) / (violaxanthin + antheraxanthin + zeaxanthin)
Total chl a concentrations were normalized to the FW of samples. Fucoxanthin and chl c pigment concentrations normalized to chl a (moles per 100 mol of chl a) were pooled, and referred to as the antenna pigment pool. Violaxanthin, antheraxanthin and zeaxanthin concentrations normalized to chl a were pooled, referred to as the xanthophyll cycle pool.
Statistical analyses
Student’s t-test (t) (with Welch correction) was used to test for the difference between means of the photosynthetic parameters (Pmax, rETRmax, α and Ik), the Fv/Fm, NPQ values and pigment contents after the dataset was tested for normality using the Shapiro–Wilk test (Shapiro & Wilk, Citation1965). Response to light or temperature was fitted and response parameters were compared using Student’s t-test. The DR response was compared within sites using a t-test for paired samples (t(p)) after checking the data for normality. Adjustment of P values for multiple testing was done using the Holm method. The effect of temperature on the response parameters of light-response curves was analysed using a PERMANOVA (Anderson, Citation2001) in the vegan package of R software. Post hoc power analysis (1-β) was performed on non-significant results using G*Power software which automatically determines the effect size (Faul et al., Citation2007). All experimental units had three replicates (n = 3).
RESULTS
Comparison of the photosynthetic activity between the two sites
Gametophytes from the two sites showed differences in their photosynthetic parameters (). When normalized to chl a, Pmax(chla) values, the light-saturated maximal gross oxygen production values were higher in Roscoff than in Wissant cultures (t = 8.0, Holm-adjusted P = 0.003), with 0.27 ± 0.01 µmol O2 nmoles chl a−1 h−1 in Wissant, and 0.95 ± 0.15 µmol O2 nmoles chl a−1 h−1 in Roscoff (). rETRmax values (22.74 ± 5.37 µmol e− m−2 s−1 in Wissant and 15.90 ± 0.77 µmol e− m−2 s−1 in Roscoff), the light-saturated maximal electron transfer rates, and Pmax(FW) on a FW basis (56.47 ± 13.18 µmol O2 gFW−1 h−1 in Wissant and 38.33 ± 1.12 µmol O2 gFW−1 h−1 in Roscoff), were not significantly different between the two sites (t = 2.9, Holm-adjusted P = 0.133 for rETRmax; t = 2.8, Holm-adjusted P = 0.142 for Pmax(FW) values) (), probably due to low statistical power resulting from small sample sizes (0.39 for rETRmax and 0.32 for Pmax(FW)).
Fig. 1. Photosynthesis-irradiance curves (P-I curves) of gametophytes of Laminaria digitata from Wissant (□) and Roscoff (●) (n = 3), expressed as (A) relative electron transport rate (rETR, µmol e– m–2 s–1), (B) gross oxygen production on a fresh weight basis (GP, µmol O2 gFW–1 h–1) and (C) gross oxygen production per unit chl a (GP, µmol O2 nmoles chl a–1 h–1).
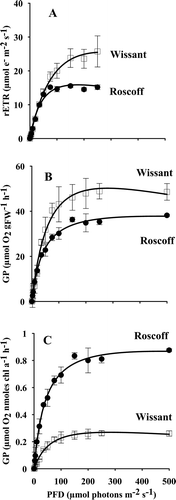
The same pattern appeared for the ascending slope under light limitation (α values). α(chla) values were significantly higher in Roscoff than in Wissant cultures (respectively 0.005 ± 0.001 in Wissant and 0.023 ± 0.002 in Roscoff) (t = 13.9, Holm-adjusted P = 0.001) when expressed on a chl a basis, whereas no differences were observed when expressed in electron transport rates or on a FW basis (respectively 0.37 ± 0.06 in Wissant and 0.40 ± 0.03 in Roscoff; t = 0.68, Holm-adjusted P = 0.534 for α(rETR) and 1.15 ± 0.17 in Wissant and 0.99 ± 0.09 in Roscoff t = 1.39, Holm-adjusted P = 0.305 for α(FW)) which may also result from very low statistical power (respectively 0.09 and 0.19).
Considering the light saturation parameter (Ik), no significant differences were found between the two sites but the low statistical power suggests that there was insufficient replication to resolve the differences (t = 1.95, Holm-adjusted P = 0.245 and 1-β = 0.30 for Ik(rETR), t = 1.77, Holm-adjusted P = 0.152 and 1-β = 0.28 for Ik(FW) and t = 1.13, Holm-adjusted P = 0.322 and 1-β = 0.14 for Ik(chla)). Despite the lack of significant differences, average Ik values in gametophytes from Wissant were always greater than Ik values in gametophytes from Roscoff (62.67 ± 20.20 µmol photons m−2 s−1 in Wissant, and 39.71 ± 1.73 µmol photons m−2 s−1 in Roscoff on an electron rate basis; 49.44 ± 9.83 µmol photons m−2 s−1 in Wissant, and 38.83 ± 3.42 µmol photons m−2 s−1 in Roscoff on a FW basis and 52.39 ± 13.49 in Wissant and 41.98 ± 8.56 in Roscoff on a chl a basis).
Despite high irradiance (500 µmol photons m−2 s−1) in comparison with the culture conditions, no decrease in oxygen production was observed.
Comparison of pigment contents
Pigment contents were similar in gametophytes from both sites () except for chl c and violaxanthin contents, which were higher in Wissant than in Roscoff cultures (t = 4.69, Holm-adjusted P = 0.047 for chl c and t = 4.26, Holm-adjusted P = 0.047 for violaxanthin). When expressed on a total pigment content basis, no differences between sites were observed in chl a contents, but chl a contents were slightly lower in Wissant and statistical power was low (0.33). Therefore a higher ΣXC:chl a pigment ratio might have been expected in Wissant cultures, but the lack of significant difference is believed to be the result of low statistical power (0.53).
Table 2. Pigment composition (moles per 100 moles of all pigments) and pigment ratios (×100) of gametophytes in L. digitata from Roscoff and Wissant (n = 3).
When chl a contents were expressed per unit fresh weight (respectively 47.74 ± 6.63 nmoles gFW−1 in Roscoff and 155.95 ± 38.22 nmoles gFW−1 in Wissant), they were significantly higher in Wissant (t = 4.83, Holm-adjusted P = 0.036).
Comparison of photoprotective capacities
NPQ was measured along with rETR (). NPQ values after prolonged darkness (12 h, corresponding to the end of the dark period of culture conditions) were higher than those under weak irradiances. Maximal Fm’ values were reached under an average irradiance of 20 µmol photons m−2 s−1 in Roscoff gametophytes, and 50 µmol photons m−2 s−1 in those from Wissant.
Fig. 2. NPQ of gametophytes of L. digitata from Wissant (□) and Roscoff (●) (n = 3) measured during the data collection for the rETR-I curves.
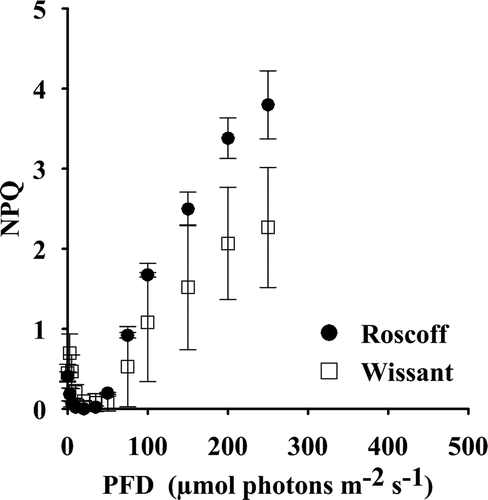
NPQ progressively developed with increasing irradiance. Maximal NPQ values (NPQ250) were reached at the highest applied irradiance (250 µmol photons m−2 s−1) and these values were significantly higher in gametophytes from Roscoff than in gametophytes from Wissant (t = 3.5, Holm-adjusted P = 0.047), with NPQ250 values of 3.80 ± 0.42 in Roscoff and of 2.37 ± 0.56 in Wissant.
Intrinsic efficiency of PSII was altered in both populations, as indicated by the strong decline of relative Fv/Fm values () when gametophytes were exposed to 500 µmol photons m−2 s−1 for 2 h. It reached a constant level, decreasing by 70% of the initial Fv/Fm value after 2 h in both populations. In dim light, Fv/Fm recovered to a level within 5% of the initial Fv/Fm value after 1.5 h in Roscoff and after 4 h in Wissant.
Fig. 3. (A) Relative optimal quantum yield (rel. Fv/Fm) and (B) de-epoxidation ratio of violaxanthin into antheraxanthin and zeaxanthin of gametophytes of L. digitata from Wissant (□) and Roscoff (●) (n = 3). Gametophytes were first exposed to high irradiance (500 µmol photons m–2 s–1) for 2 h and then to dim white light (10 µmol photons m–2 s–1).
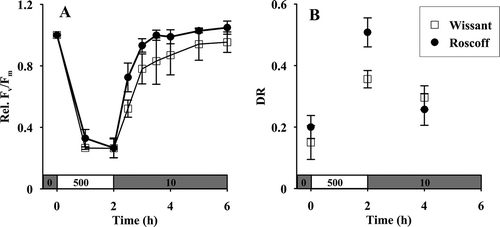
During light stress, there was an increase in DR in gametophytes from Roscoff and from Wissant, corresponding to the progressive de-epoxidation of violaxanthin into antheraxanthin and zeaxanthin (t(p) = 7.9, Holm-adjusted P = 0.025 for gametophytes from Wissant and t(p) = 8.9, Holm-adjusted P = 0.025 for gametophytes from Roscoff) (). The increase in intrinsic efficiency of PSII under dim light was accompanied by a decrease in the DR values in gametophytes from Roscoff (t(p) = 11.6, Holm-adjusted P = 0.022), indicating the reconversion of zeaxanthin and antheraxanthin into violaxanthin. In spite of the difference in the absolute values of DR measured at both sites after 2 h of strong illumination, the percentage increase in DR was similar in gametophytes from both sites, 160% of the initial DR values. There was no significant decrease in DR values in Wissant after 2 h under dim light.
Comparison of the response to temperature
Increasing the temperature resulted in a decrease in α values in Wissant (), and an increase in rETRmax and Ik values for both sites (respectively and ) ().
Table 3. Results of multivariate PERMANOVA analysis to test for differences in temperature and sites.
Fig. 4. Photosynthetic parameters (α (A), rETRmax (B) and Ik (C)) and maximal NPQ (NPQ250) (D) of gametophytes of L. digitata from Wissant (□) and Roscoff (■) (n = 3). Gametophytes acclimated at 5°C, 10°C, 15°C and 20°C were exposed to increasing irradiance at the temperature of acclimation. Photosynthetic parameters (α, rETRmax and Ik) were calculated using the model of Eilers & Peeters (Citation1988).
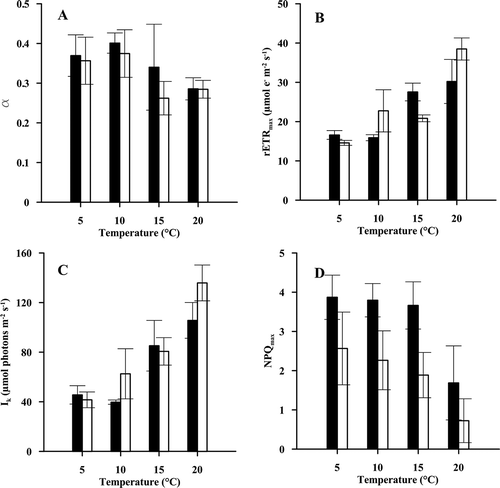
NPQ250 was calculated at the end of each rETR-E curve (). When exposed to 20°C, there was a slight decrease in the NPQ250 values, in comparison with the values reached at 10°C.
The sensitivity of Fv/Fm to temperature was tested over a broad range of temperatures (). At 10°C, Fv/Fm values in the dark were 0.52 ± 0.09 in gametophytes from Roscoff and 0.53 ± 0.06 in those from Wissant. With the progressive increase in temperature (from 10°C to 32°C), the rel. Fv/Fm declined for temperatures above 20°C. There were no differences between sites in the response parameters from the regression analysis.
Fig. 5. Relative optimal quantum yield (rel. Fv/Fm) of gametophytes of L. digitata from Wissant (□) and Roscoff (●) (n = 3). Gametophytes initially kept at 10°C were separately exposed to increases and decreases in temperature (2°C steps at 15 min intervals). Data were fitted using a non-linear regression analysis (y = a*e(b*x/c) + d).
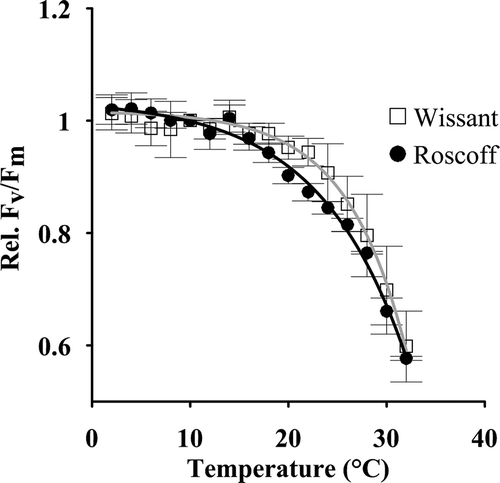
DISCUSSION
Microscopic stages are thought to be the hardiest life-cycle stages of kelp (tom Dieck, Citation1993). They form a ‘seed bank’ which persists through stressful environmental conditions and ensures the survival of species when unfavourable conditions occur, as may happen during unusual heat waves (Ladah & Zertuche-González, Citation2007; Bartsch et al., Citation2013). Therefore, tolerance of L. digitata gametophytes to stressful environmental conditions is essential. In this study, we tested their physiological tolerance to irradiance and temperature stress in two populations with different environmental conditions along the French coast. The main result of the present study is that gametophytes of L. digitata were highly resistant to the irradiance and temperature treatments that might be locally encountered in the field. This conclusion is reinforced by the fact that sori were sampled during months when irradiance and temperature stresses are not prevalent: their temperature and irradiation resistance may be even higher during summer.
We set out to study the effects of high light stress on the photosynthesis of L. digitata gametophytes. Our results demonstrate that gametophytes can cope with prolonged high irradiance stress, confirming the potential for high light tolerance in kelp gametophytes (Iizumi & Sakanishi, Citation1994; Hanelt et al., Citation1997; Altamirano et al., Citation2004). This tolerance arises from the efficient thermal dissipation of excess light (NPQ), mainly through the formation of antheraxanthin and zeaxanthin, which induce conformational change in the light-harvesting complexes (LHC) II (Jahns & Holzwarth, Citation2009). This zeaxanthin-dependent quenching is a slow component of NPQ, and Garcia-Mendoza & Colombo-Pallota (2007) have shown that kelp may lack fast energy or pH-dependent quenching. However, the large xanthophyll cycle pigment pool may accelerate NPQ development and thus compensate for this lack. Under dim light, epoxidation of zeaxanthin and antheraxanthin was virtually complete in less than 1 h. The slowly relaxing component of photoinhibition generally corresponds to the progressive re-activation of PSII or possibly to a conformational change of the LHC, and probably the aggregation of LHC due to zeaxanthin binding (Garcia-Mendoza & Colombo-Pallotta, Citation2007). Therefore, our study provides further evidence that the efficient reversible conversion between violaxanthin, antheraxanthin and zeaxanthin in gametophytes (previously suggested by Hanelt et al., Citation1997 and Altamirano et al., Citation2004) may be a widespread mechanism of fast-dynamic acclimation to abiotic stress in macroalgae (Fernández-Marín et al., Citation2011).
Another possible mechanism protecting L. digitata gametophytes from rapid light fluctuations is the maintenance of NPQ in the dark, a mechanism previously observed in microphytobenthos (Serôdio et al., Citation2005) and in several phytoplankton species (Casper-Lindley & Björkman, Citation1998; Cruz et al., Citation2011). In Pelvetia canaliculata, the NPQ of chl a fluorescence in the dark depends on the activation of violaxanthin de-epoxidase (Fernández-Marín et al., Citation2011), which is triggered by acidification of the lumen due to metabolic activity in the dark (Cruz et al., Citation2011). This activation may thus sustain ATP synthase activity (Casper-Lindley & Björkman, Citation1998; Serôdio et al., Citation2005), and prevent the formation of oxygen radicals. NPQ in the dark may therefore represent a type of sustained photoprotection, maintaining a dissipative state through pre-formed zeaxanthin or antheraxanthin, as observed here.
Acclimation to higher irradiances than the culture irradiance used in this experiment and/or an intermittent light exposure may have also provided a higher tolerance to the two-hour light stress of 500 µmol photons m−2 s−1 (Han and Kain, Citation1996; Lavaud et al., Citation2002). Along the French coast of the English Channel, irradiance levels of 500 µmol photons m−2 s−1and more can be recorded during spring and summer low spring tides (Gevaert et al., Citation2003; Delebecq et al., Citation2011, Citation2013), although actual incident irradiances are generally greatly attenuated by the sporophyte canopy (Gerard, Citation1984).
This study also highlighted that the photosynthetic performance of L. digitata gametophytes was not negatively affected by short-term fluctuations in water temperature such as may be encountered locally in the field during low tide periods. Gametophyte PSII – essential for the whole photochemistry process (Havaux & Tardy, Citation1996; Roleda, Citation2009) – shows high resistance to the temperatures currently encountered in the field. Increased temperature promotes electron transfer via the PSII through an increase in thylakoid membrane fluidity (Havaux & Tardy, Citation1996) and results in an increase in maximum relative electron transfer rates (rETRmax) and in the onset of saturation parameters (Ik), as observed here. An increase in gross oxygen production with temperature can therefore be expected up to an optimum (Sukenik et al., Citation1987), since the relationship between electron transport rate and gross oxygen production is robust, provided that the temperature changes are not extreme (Morris & Kromkamp, Citation2003).
Above an optimal temperature (20°C in this study), a complex set of inactivation and denaturation events occur in the thylakoid, accompanied by the generation of elevated levels of reactive oxygen species, which reduces photosynthetic capacity (Sharkey, Citation2005; Allakhverdiev et al., Citation2008) and also the ability to cope with high light stress (Roleda, Citation2009). Superoptimal temperature also promotes electron-consuming processes (such as cyclic electron transfer) and dark respiration (Fain & Murray, Citation1982; Sharkey, Citation2005; Henkel & Hofmann, Citation2008), which can result in negative net photosynthesis.
However, short-term temperature responses do not provide insight into long-term individual survival with regard to temperature: temperature optima for photosynthesis can be several degrees higher than temperature optima for survival and growth (Davison, Citation1991) as it is not possible to evaluate the extent of sublethal stress. Concerning L. digitata gametophytes, tom Dieck (Citation1993) showed that they were able to survive 8 weeks at 23°C, demonstrating that gametophytes are moderately resistant to temperature extremes that are rarely encountered across the geographic range of this species. Moreover, there is seasonal acclimation of the temperature response of gametophytes (Lee & Brinkhuis, Citation1988) and temperature resistance of PSII may be even higher in gametophytes sampled in warmer months or acclimated to higher temperatures (Mohring et al., Citation2013).
The two sites from which gametophytes were obtained are known to display different seasonal ranges of abiotic conditions (Berx & Hughes, Citation2009; Delebecq et al., Citation2013), and strong photoacclimation to local environmental conditions of sporophytes of L. digitata from the two same sites was previously highlighted (Delebecq et al., Citation2013). Here, we found some differences in the physiological characteristics of gametophytes between the two sites, however, low replication levels did not permit a full investigation of the physiological diversity of the populations, and replication was clearly insufficient to resolve the differences as indicated by the very low statistical power of our analysis (never exceeding 53%). Moreover, results in the present study are confounded by the different environmental conditions at the two sites at the time of sampling (), which may influence the physiological response of gametophytes even after one month’s cultivation under lab conditions, depending on the timescale of acclimation to new light and temperature treatments. More information on the potential seasonal changes in the gametophytes from Roscoff and Wissant would be necessary for a more complete picture of the responses of L. digitata gametophytes in the English Channel, taking into account the variation of the whole set of past and present environmental conditions, and to test if the photosynthetic characteristics measured in sporophytes may be conserved in gametophytes.
The higher chl a concentrations and fucoxanthin contents in gametophytes from Wissant may suggest a greater light-harvesting efficiency and a higher density of reaction centres (Gerard, Citation1988), which generally result in higher photosynthetic rates. Although maximal photosynthetic rates (rETRmax and PmaxFW) in Wissant were not significantly different from those in Roscoff, this is believed to result from low statistical power. Pmaxchla was higher in Roscoff gametophytes than in Wissant gametophytes, indicating more efficient light use per chl a molecule. This difference may be due to the self-shading of the LHC, i.e. the fact that light absorption does not increase despite a higher pigment concentration (Falkowski & Raven, Citation1997). Daily underwater irradiances were similar at both sites at the time of sampling (Delebecq et al., Citation2013). As thermal acclimation is known to induce changes in photoacclimation status (Machalek et al., Citation1996), those differences in photosynthetic characteristics could be partly due to the lower sea surface temperature at Wissant at the time of sampling (), if field temperature still influences gametophyte responses to light, even after one month of cultivation.
The greater violaxanthin content in Wissant may then compensate for the large antenna size (higher fucoxanthin contents) which is known to decrease the probability of encounters between violaxanthin and violaxanthin de-epoxidase and the speed of de-epoxidation under high light (Garcia-Mendoza & Colomba-Palotta, Citation2007). It may also be due to the faster changing light conditions imposed by higher light attenuation at Wissant. Violaxanthin is also involved in the response to other abiotic stressors (Havaux & Tardy, Citation1996; Fernández-Marín et al., Citation2011). Thus, a larger pool of violaxanthin may help gametophytes from Wissant to cope with other abiotic stressors not tested in this study.
We found no differences in the temperature response of gametophytes from the two sites with respect to the resistance of PSII quantum efficiency and increasing temperature. We expected that the wider temperature range encountered at Wissant would have induced a difference in thermostability of PSII. However, the small difference in temperature range may not have been sufficient to produce detectable differences. Moreover, at the time of sampling, temperature was lower at Wissant (5°C) than at Roscoff (12°C) and this difference may have influenced the photosynthetic response of gametophytes in our study (Lee & Brinkhuis, Citation1988; Mohring et al., Citation2013). As already noted, the low level of replication in our experiments may have obscured differences.
Regarding the temperature response of gametophytes, Bolton & Lüning (Citation1982) suggested that Laminaria species may show sufficient phenotypic plasticity to adjust to the temperature range along their distribution, rather than being composed of differentiated temperature-adapted ecotypes throughout their ranges. However, notwithstanding similar growth patterns, physiological differences in the response to temperature may nevertheless occur among populations of perennial brown seaweeds (Henkel & Hofmann, Citation2008; Staehr & Wernberg, Citation2009). In order to test for differences in temperature responses of L. digitata populations along the French coast, further experiments are needed to compare gametophytes from (1) the two sites at different times of the year during their respective sporulation periods (Mohring et al., Citation2013) and (2) sites with more contrasting temperature ranges (Staehr & Wernberg, Citation2009). More generally, investigating the joint effect of light and temperature requires a multifactorial experiment (Fredersdorf et al., Citation2009).
Gametophytes appeared to be resistant to the light and temperature conditions currently experienced in the field. Regarding vulnerability to environmental conditions, physiological studies on the interactive effects of multiple abiotic stressors are required to improve our understanding of the microscopic stages in kelp (Fredersdorf et al., Citation2009). For instance, desiccation tolerance (Contreras-Porcia et al., Citation2012), ultraviolet radiation (Roleda et al., Citation2006) and burial in sediment (Roleda & Dethleff, Citation2011) all affect microscopic stages. While the southern geographic range limit of L. digitata is thought to be set by inhibition of reproduction, Bartsch et al. (Citation2013) observed that when the temperature reaches 18°C, survival of microscopic stages is essential to cope with unusual stressful conditions. There is also an important need to obtain more information on the persistence of these stages in the field. It could help in defining which environmental conditions are really encountered in the field by the different microscopic developmental stages. Despite the failure of the present study to report local variation in the physiological response of L. digitata, it remains essential to take into account local variation in predicting the impact of fast-changing conditions in coastal areas.
DISCLOSURE STATEMENT
No potential conflict of interest was reported by the author(s)
ACKNOWLEDGEMENTS
We would like to thank the BEDIM Team of the Station Biologique de Roscoff and especially M. Valero and C. Destombe for their valuable support and also for receiving and providing seaweed material. We also thank the Service Mer et Observation at the Station Biologique de Roscoff and A. Migné for their valuable support during field sampling. We greatly thank Marie Cachera for her help in statistical analyses. We thank the three reviewers for their remarks and advice that helped to improve the manuscript.
Additional information
Funding
Notes on contributors
François Gevaert
G Delebecq: experimental design, culture experiment, analyses, manuscript drafting, manuscript editing; D Davoult: overall supervision, experimental design, manuscript editing; MA Janquin: culture experiment, analyses; LV Oppliger: culture experiment, manuscript editing; D Menu: culture experiment, analyses; JC Dauvin: overall supervision, manuscript editing; F Gevaert: overall supervision, experimental design, analyses, manuscript editing.
REFERENCES
- Allakhverdiev, S.I., Kreslavski, V.D., Klimov, V.V., Los, D.A., Carpentier, R. & Mohanty, P. (2008). Heat stress: an overview of molecular responses in photosynthesis. Photosynthesis Research, 98: 541–550.
- Altamirano, M., Murakami, A. & Kawai, H. (2004). High light stress in the kelp Ecklonia cava. Aquatic Botany, 79: 125–135.
- Anderson, M.J. (2001). A new method for non-parametric multivariate analysis of variance. Austral Ecology, 26: 32–46.
- Arsalane, W., Rousseau, B. & Duval, J.C. (1994). Influence of the pool size of the xanthophyll cycle on the effect of light stress in a diatom: competition between photoprotection and photoinhibition. Photochemistry and Photobiology, 60: 237–243.
- Arzel, P. (1998). Les laminaires sur les côtes bretonnes. Evolution de l’exploitation et de la flottille de pêche, état actuel et perspectives. Plouzané, France.
- Bartsch, I., Wiencke, C., Bischof, K., Buchholz, C., Buck, B. H., Eggert, A., Feuerpfeil, P., Hanelt, D., Jacobsen, S., Karez, R., Karsten, U., Molis, M., Roleda, M., Schumann, R., Schubert, H., Valentin, K.U., Weinberger, F. & Wiese, J. (2008). The genus Laminaria sensu lato: recent insights and developments. European Journal of Phycology, 43: 1–86.
- Bartsch, I., Vogt, J., Pehlke, C. & Hanlet, D. (2013). Prevailing surface temperatures inhibit summer reproduction of the kelp Laminaria digitata at Helgoland (North Sea). Journal of Phycology, 49: 1061–1073.
- Berx, B. & Hughes, S.L. (2009). Climatology of surface and near-bed temperature and salinity on the North-West European continental shelf for 1971–2000. Continental Shelf Research, 29: 2286–2292.
- Bolton, J.J. & Lüning, K. (1982). Optimal growth and maximal survival temperatures of Atlantic Laminaria species (Phaeophyta) in culture. Marine Biology, 66: 89–94.
- Carney, L.T. (2011). A multispecies laboratory assessment of rapid sporophyte recruitment from delayed kelp gametophytes. Journal of Phycology, 47: 244–251.
- Casper-Lindley, C. & Björkman, O. (1998). Fluorescence quenching in four unicellular algae with different light-harvesting and xanthophyll-cycle pigments. Photosynthesis Research, 56: 277–289.
- Christie, H., Jorgensen, N.M., Norderhaug, K.M. & Waage-Nielsen, E. (2003). Species distribution and habitat exploitation of fauna associated with kelp (Laminaria hyperborea) along the Norwegian Coast. Journal of the Marine Biological Association of the United Kingdom, 83: 687–699.
- Contreras-Porcia, L., Callejas, S., Thomas, D., Sordet, C., Pohnert, G., Contreras, A., Lafuente, A., Flores-Molina, M. & Correa, J. (2012). Seaweeds early development: detrimental effects of desiccation and attenuation by algal extracts. Planta, 235: 337–348.
- Cruz, S., Goss, R., Wilhelm, C., Leegood, R., Horton, P. & Jakob, T. (2011). Impact of chlororespiration on non-photochemical quenching of chlorophyll fluorescence and on the regulation of the diadinoxanthin cycle in the diatom Thalassiosira pseudonana. Journal of Experimental Botany, 62: 509–519.
- Davison, I.R. (1991). Environmental effects on algal photosynthesis: temperature. Journal of Phycology, 27: 2–8.
- Davison, I.R. & Pearson, G.A. (1996). Stress tolerance in intertidal seaweeds. Journal of Phycology, 32: 197–211.
- Davoult, D., Engel, C.R., Arzel, P., Knoch, D. & Laurans, M. (2011). Environmental factors and commercial harvesting: exploring possible links behind the decline of the kelp Laminaria digitata in Brittany, France. Cahiers de Biologie Marine, 52: 429–434.
- Delebecq, G., Davoult, D., Menu, D., Janquin, M.-A., Migné, A., Dauvin, J.-C. & Gevaert, F. (2011). In situ photosynthetic performance of Laminaria digitata (Phaeophyceae) during spring tides in Northern Brittany. Cahiers de Biologie Marine, 52: 405–414.
- Delebecq, G., Davoult, D., Menu, D., Janquin, M.-A., Dauvin, J.C. & Gevaert, F. (2013). Influence of local environmental conditions on the seasonal acclimation process and the daily integrated production rates of Laminaria digitata (Phaeophyta) in the English Channel. Marine Biology, 160: 503–517.
- tom Dieck (Bartsch), I. (1993). Temperature tolerance and survival in darkness of kelp gametophytes (Laminariales, Phaeophyta): ecological and biogeographical implications. Marine Ecology Progress Series, 100: 253–264.
- Edwards, M.S. (2000). The role of alternate life-history stages of a marine macroalga: a seed bank analogue? Ecology, 81: 2404–2415.
- Eilers, P.H.C. & Peeters, J.C.H. (1988). A model for the relationship between light intensity and the rate of photosynthesis in phytoplankton. Ecological Modelling, 42: 199–215.
- Fain, S.R. & Murray, S.N. (1982). Effects of light and temperature on net photosynthesis and dark respiration of gametophytes and embryonic sporophytes of Macrocystis pyrifera. Journal of Phycology, 18: 92–98.
- Falkowski, P.G. & Raven, J.A. (1997). Aquatic Photosynthesis. Blackwell Science, Malden, MA.
- Faul, F., Erdfelder, E., Lang, A.-G. & Buchner, A. (2007). G*Power 3: a flexible statistical power analysis program for the social, behavorial and biomedical sciences. Behavior Research Methods, 39: 175–191.
- Fejtek, S.M., Edwards, M.S. & Kim, K.Y. (2011). Elk kelp, Pelagophycus porra, distribution limited due to susceptibility of microscopic stages to high light. Journal of Experimental Marine Biology and Ecology, 396: 194–201.
- Fernández-Marín, B., Míguez, F., Becerril, J.M. & García-Plazaola, J. (2011). Activation of violaxanthin cycle in darkness is a common response to different abiotic stresses: a case study in Pelvetia canaliculata. BMC Plant Biology, 11: 181.
- Fredersdorf, J., Müller, R., Becker, S., Wiencke, C. & Bischof, K. (2009). Interactive effects of radiation, temperature and salinity on different life history stages of the Arctic kelp Alaria esculenta (Phaeophyceae). Oecologia, 160: 483–492.
- Garcia-Mendoza, E. & Colombo-Pallotta, M.F. (2007). The giant kelp Macrocystis pyrifera presents a different nonphotochemical quenching control than higher plants. New Phytologist, 173: 526–536.
- Genty, B., Briantais, J.M. & Baker, N.R. (1989). The relationship between the quantum yield of photosynthetic electron transport and quenching of chlorophyll fluorescence. Biochimica et Biophysica Acta, 990: 87–92.
- Gerard, V.A. (1984). The light environment in a giant kelp forest: influence of Macrocystis pyrifera on spatial and temporal variability. Marine Biology, 84: 189–195.
- Gerard, V.A. (1988). Ecotypic differentiation in light-related traits of the kelp Laminaria saccharina. Marine Biology, 97: 25–36.
- Gevaert, F., Créach, A., Davoult, D., Migné, A., Levavasseur, G., Arzel, P., Holl, A.-C. & Lemoine, Y. (2003). Laminaria saccharina photosynthesis measured in situ: photoinhibition and xanthophyll cycle during a tidal cycle. Marine Ecology Progress Series, 247: 43–50.
- Han, T. & Kain, J.M. (1996). Effect of photon irradiance and photoperiod on young sporophytes of four species of the Laminariales. European Journal of Phycology, 31: 233–240.
- Hanelt, D., Wiencke, C., Karsten, U. & Nultsch, W. (1997). Photoinhibition and recovery after high light stress in different developmental and life-history stages of Laminaria saccharina (Phaeophyceae). Journal of Phycology, 33: 387–395.
- Harley, C.D.G., Anderson, K.M., Demes, K.W., Jorve, J.P., Kordas, R.L., Coyle, T.A. & Graham, M.H. (2012). Effects of climate change on global seaweed communities. Journal of Phycology, 48: 1064–1078.
- Havaux, M. & Tardy, F. (1996). Temperature-dependent adjustment of the thermal stability of the photosystem II in vivo: possible involvement of xanthophyll-cycle pigments. Planta, 198: 324–333.
- Henkel, S.K. & Hofmann, G.E. (2008). Thermal ecophysiology of gametophytes cultured from invasive Undaria pinnatifida (Harvey) Suringar in coastal California harbors. Journal of Experimental Marine Biology and Ecology, 367: 164–173.
- Iizumi, H. & Sakanishi, Y. (1994). Temperature dependence of photosynthesis-irradiation (P-I) relationship of gametophytes of Laminaria religiosa Miyabe. Bulletin of Hokkaido National Fisheries Research Institute, 58: 45–51.
- Jahns, P. & Holzwarth, A.R. (2009). The role of xanthophylls cycle and of lutein in the photoprotection of photosystem II. Biochimica et Biophysica Acta, 1817: 182–193.
- Ladah, L.B. & Zertruche-González, J.A. (2007). Survival of microscopic stages of a perennial kelp (Macrocystis pyrifera) from the centre and the southern extreme of its range in the northern hemisphere after exposure to simulated El Nino stress. Marine Biology, 152: 677–686.
- Lavaud, J., Rousseau, B., van Gorkom, H.J. & Etienne, A.-L. (2002). Influence of the diadinoxanthin pool size on photoprotection in the marine planktonic diatom Phaeodactylum tricornutum. Plant Physiology, 129: 1398–1406.
- Ledford, H.K. & Niyogi, K.K. (2005). Singlet oxygen and photo-oxidative stress management in plants and algae. Plant, Cell and Environment, 28: 1037–1045.
- Lee, J.A. & Brinkhuis, B.H. (1988). Seasonal light and temperature interaction effects on development of Laminaria saccharina (Phaeophyta) gametophytes and juvenile sporophytes. Journal of Phycology, 24: 181–191.
- Lüning, K. (1990). Seaweeds: Their Environment, Biogeography and Ecophysiology. Wiley, New York.
- Lüning, K. & Neushul, M. (1978). Light and temperature demands for growth and reproduction of laminarian gametophytes in Southern and Central California. Marine Biology, 45: 297–309.
- Machalek, K.M., Davison, I.R. & Falkowski, P.G. (1996). Thermal acclimation and photoacclimation of photosynthesis in the brown alga Laminaria saccharina. Plant, Cell and Environment, 19: 1005–1016.
- Mann, K.H. (1973). Seaweeds: their productivity and strategy for growth. The role of large marine algae in coastal productivity is far more important than has been suspected. Science, 182: 975–981.
- Martinez, E.A. & Santelices, B. (1998). Selective mortality on haploid and diploid microscopic stages of Lessonia nigrescens Bory (Phaeophyta, Laminariales). Journal of Experimental Marine Biology and Ecology, 229: 219–239.
- Matson, P.G. & Edwards, M.S. (2007). Effects of ocean temperature on the southern range limits of two understory kelps, Pterygophora californica and Eisenia arborea, at multiple life-stages. Marine Biology, 151: 1941–1949.
- Mohring, M.B., Kendrik, G.A., Wernberg, T., Rule, M.J. & Vanderklift, M.A. (2013). Environmental influences on kelp performance across the reproductive period: an ecological trade-off between gametophyte survival and growth? PLoS ONE, 8: e65310.
- Morris, E.P. & Kromkamp, J.C. (2003). Influence of temperature on the relationship between oxygen- and fluorescence-based estimates of photosynthetic parameters in a marine benthic diatom (Cylindrotheca closterium). European Journal of Phycology, 38: 133–142.
- Oppliger, L.V., Correa, J.A., Engelen, A.H., Tellier, F., Vieira, V., Faugeron, S., Valero, M., Gomez, G. & Destombe, C. (2012). Temperature effects on gametophyte life-history traits and geographic distribution of two cryptic kelp species. PLoS One, 7: e39289.
- Provasoli, L. (1968). Media and prospects for the cultivation of marine algae. In Cultures and Collections of Algae. Proceedings of the US–Japan Conference, Hakone, Japan, September 1966 (Watanabe, A. & Hattori, A., editors), 63–75. Japanese Society of Plant Physiology, Hakone.
- Raven, J.A. & Geider, R.J. (2003). Adaptation, acclimation and regulation in algal photosynthesis. In Photosynthesis in Algae (Larkum, W.D., Douglas, S.E. & Raven, J.A., editors), 385–412. Kluwer Academic Publishers, Dordrecht.
- Raybaud, V., Beaugrand, G., Goberville, E., Delebecq, G., Destombe, C., Valero, M., Davoult, D., Morin, P. & Gevaert, F. (2013). Decline in kelp in West Europe and climate. PLoS ONE, 8: e66044.
- Reed, D.C. & Foster, M.S. (1984). The effects of canopy shading on algal recruitment and growth in a giant kelp forest. Ecology, 65: 937–948.
- Roleda, M.Y. (2009). Photosynthetic response of Arctic kelp zoospores exposed to radiation and thermal stress. Photochemical and Photobiological Sciences, 8: 1302–1312.
- Roleda, M.Y. & Dethleff, D. (2011). Storm-generated sediment deposition on rocky shores: simulating burial effects on the physiology and morphology of Saccharina latissima sporophytes. Marine Biology Research, 7: 213–223.
- Roleda, M.Y., Hanelt, D. & Wiencke, C. (2006). Exposure to ultraviolet radiation delays photosynthetic recovery in Arctic kelp zoospores. Photosynthesis Research, 88: 311–322.
- Roleda, M.Y., Zacher, K., Wulff, A., Hanelt, D. & Wiencke, C. (2008). Susceptibility of spores of different ploidy levels from Antarctic Gigartina skottsbergii (Gigartinales, Rhodophyta) to ultraviolet radiation. Phycologia, 47: 361–370.
- Sauvageau, C. (1915). Sur la sexualité heterogamique d’une Laminaire (Saccorhiza bulbosa). Comptes Rendus de l’Académie des Sciences de Paris, 161: 796–799.
- Serôdio, J., Cruz, S., Vieira, S. & Brotas, V. (2005). Non-photochemical quenching of chlorophyll fluorescence and operation of the xanthophyll cycle in estuarine microphytobenthos. Journal of Experimental Marine Biology and Ecology, 326: 157–169.
- Shapiro, S.S. & Wilk, M.B. (1965). An analysis of variance test for normality (complete samples). Biometrika, 52: 591–611.
- Sharkey, T.D. (2005). Effects of moderate heat stress on photosynthesis: importance of thylakoid reactions, rubisco deactivation, reactive oxygen species, and thermotolerance provided by isoprene. Plant, Cell & Environment, 28: 269–277.
- Staehr, P.A. & Wernberg, T. (2009). Physiological responses of Ecklonia radiata (Laminariales) to a latitudinal gradient in ocean temperature. Journal of Phycology, 45: 91–99.
- Sukenik A., Bennett J. & Falkowski, P.G. (1987). Light-saturated photosynthesis – limitation of electron transport or carbon fixation? Biochimica et Biophysica Acta, 891: 205–215.
- Takahashi, S. & Murata, N. (2008). How do environmental stresses accelerate photoinhibition? Trends in Plant Science, 13: 178–182.
- Walters, R.G. (2005). Towards an understanding of photosynthetic acclimation. Journal of Experimental Botany, 56: 435–447.
- Wang, C., Fan, X., Wang, G., Niu, J. & Zhou, B. (2011). Differential expression of rubisco in sporophytes and gametophytes of some marine macroalgae. PLoS ONE, 6: e16351.
- Wernberg, T., Russell, B.D., Moore, P.J., Ling, S.D., Smale, D.A., Campbell, A., Coleman, M.A., Steinberg, P.D., Kendrick, G.A. & Connell, S.D. (2011a). Impact of climate change in a global hotspot for temperate marine biodiversity and ocean warming. Journal of Experimental Marine Biology and Ecology, 400: 7–16.
- Wernberg, T., Russell, B.D., Thomsen, M.S., Gurgel, F.D., Bradshaw, C.J.A, Poloczanska, E.S. & Connell, S.D. (2011b). Seaweed communities in retreat from ocean warming. Current Biology, 21: 1828–1832.
- Wernberg, T., Smale, D.A. & Thomsen, M.S. (2012). A decade of climate change experiments on marine organisms: procedures, patterns and problems. Global Change Biology, 18: 1491–1498.
- Wernberg, T., Smale, D.A., Tuya, F., Thomsen, M.S., Langlois, T.J., de Bettignies, T., Bennett, S. & Rousseaux, C.S. (2013). An extreme climatic event alters marine ecosystem structure in a global biodiversity hotspot. Nature Climate Change, 3: 78–82.