Abstract
Many of the marine species that were introduced to the Baltic Sea during the Littorina stage (c. 8500–3000 years BP), e.g. Fucus vesiculosus and F. serratus, have adapted to the present low salinity. These marine species have gone from marine conditions into lower salinity environments. In this paper we ask why the recently discovered endemic brown alga Fucus radicans shows the opposite pattern. Fucus radicans is only present in the northern parts of the Baltic Sea, the low salinity Bothnian Sea (4–6 psu). Potentially, the fitness of F. radicans might be reduced in higher salinities if it is better adapted to brackish conditions. We hypothesize, however, that the southern distribution limit of F. radicans is set by biotic factors, e.g. competition with F. vesiculosus and higher grazing pressure by Idotea balthica and not by salinity. Our results show that the reproductive output of F. radicans is limited by low salinity (4 psu) but increases in higher salinities. However, the southern distribution limit, i.e. the northern Baltic Proper, is regulated by biotic factors, where the additive effects from shading by taller F. vesiculosus thalli and grazing on F. radicans by the isopod I. balthica limit the biomass production of F. radicans. We suggest that F. radicans still maintains marine traits due to its ability to propagate clonally and is restricted to the Bothnian Sea by interactions with F. vesiculosus and I. balthica. We also propose that increased precipitation due to climate change might affect the northern range limit and that the distribution of F. radicans could be expected to shift further south into the Baltic Proper.
Introduction
The distribution of species is caused by a mixture of abiotic and biotic factors, fluctuating over time. The dynamics of biotic interactions, especially along environmental gradients, can affect how species respond to climate-induced changes in abiotic factors, and vice versa (Callaway et al., Citation2002; Crain et al., Citation2004; Brooker, Citation2006).
The main abiotic factor influencing distribution of macroalgae in the Baltic Sea is salinity (Hällfors et al., Citation1981; Gogina & Zettler, Citation2010). All species of marine origin in the Baltic Sea are derived from the marine waters of the North Sea and Skagerrak. The distance each species’ distribution extends into the brackish waters of the Baltic Sea depends on its osmotic tolerance (Remane & Schlieper, Citation1971; Snoeijs, Citation1999). The perennial brown macroalga Fucus radicans Bergström & Kautsky (Bergström et al., Citation2005) however, has an intriguing distribution pattern, opposite to other species of marine origin as it is found only in the Bothnian Sea, within the salinity range from 4 to 6 psu (Forslund et al., Citation2012).
Today, only three fucoid species are present in the Baltic Sea: Fucus serratus L., Fucus vesiculosus L. and Fucus radicans. Fucus radicans is considered to have diverged from its sister species F. vesiculosus within the last 400–5000 years in the Baltic Sea, thus being the first endemic in this brackish environment (Pereyra et al., Citation2009, Citation2013). Over time, both F. serratus (Malm et al., Citation2001) and F. vesiculosus have gradually adapted to the increasingly brackish environment (Bäck et al., Citation1992; Serrão et al., Citation1996a, Citation1999) although to different extents. There is substantial genetic differentiation between F. vesiculosus populations in the atidal Baltic Sea and North Sea tidal populations (Tatarenkov et al., Citation2007). The populations in the Baltic Sea have, for example, evolved reduced tolerance to emersion stresses i.e. desiccation and freezing (Pearson et al., Citation2000). The range margin of F. serratus is at 8 psu in the Baltic Sea, where it only produces a tenth as many eggs per receptacle as the sympatric Baltic Sea ecotype of F. vesiculosus (Malm & Kautsky, Citation2003) which is better adapted to the low salinity and can reproduce in salinities as low as 4 psu (Serrão et al., Citation1996a).
Two of the main biotic factors affecting macroalgal distribution are herbivorous grazing (Sjøtun et al., Citation2007) and competition for space or shading by taller thalli (Sjøtun et al., Citation2006; Pedersen et al., Citation2012). The availability of light determines production and growth (Wahl et al., Citation2011 and references therein) and sets the depth limit of Fucus distribution according to Kautsky et al. (Citation1986). Similarly, growth of juvenile F. vesiculosus is limited by shading from the taller adult canopy (Eriksson et al., Citation2006) and at sites where F. vesiculosus and F. radicans grow in sympatry, the often smaller F. radicans thalli might experience similar impact in dense belts (Forslund et al., Citation2012).
The reduction of light availability by shading not only affects the growth of the thallus but also increases the level of herbivory on fucoids by isopods (Jormalainen & Ramsay, Citation2009). The most prominent grazers of fucoids in the Baltic Sea are the isopods Idotea spp. (Salemaa, Citation1979; Kangas et al., Citation1982) which are also of marine origin, and thus more abundant as well as diverse in salinities > 6 psu (Naylor, Citation1955; Salemaa, Citation1979). Of the species of Idotea present in the Baltic Sea, Idotea balthica Pallas 1778 is the most tolerant to low salinity and has its northern distribution limit in the middle of the Bothnian Sea (Forslund et al., Citation2012; Leidenberger et al., Citation2012).
Although F. radicans is fully capable of sexual reproduction, as documented by Bergström et al. (Citation2005), it also reproduces asexually (up to 85%) by reattachment of fragments in the form of adventitious branches (Bergström et al., Citation2005; Tatarenkov et al., Citation2005; Ardehed et al., Citation2015). Asexual reproduction through fragmentation forming new attached individuals is uncommon in fucoids, although asexual reproduction per se is not uncommon in macroalgae (i.e. Santelices & Varela, Citation1993; Wright & Davis, Citation2006). Several of the marine macroalgal species found in the Baltic Sea (e.g. Ceramium tenuicorne Kützing, Furcellaria lumbricalis (Hudson) J.V. Lamouroux) have adapted their life cycles to the low salinity by shifting towards asexual reproduction at their range margin (Bergström et al., Citation2003; Kostamo, Citation2008). This strategy has also been documented for F. vesiculosus within a few of the absolute marginal populations in the northern Baltic Sea (Tatarenkov et al., Citation2005).
In this paper we ask if F. radicans is better adapted to the low salinity environment of the Bothnian Sea than to higher salinities and discuss if the high level of asexual reproduction (which implies fewer genetic re-combinations, hence a slower evolutionary pace and reduced selection pressure) has preserved any marine traits which might have been reduced or lost in its sister species, the Baltic Sea ecotype of F. vesiculosus. If this is the case then F. radicans could very well thrive in the more saline environments of the Baltic Proper, south of its present range. We also hypothesize that the southern distribution limit of F. radicans is set by biotic factors, i.e. competition by the larger F. vesiculosus thalli through shading and grazing by the isopod I. balthica, and not by salinity.
Materials and methods
Study area
The Baltic Sea is generally described as a marginal marine environment (Johannesson & Andre, Citation2006). The marine influenced history of the Baltic Sea is quite recent. Only about 8000 years ago a large inflow of salt water brought with it several marine species (Russell, Citation1985). Although the salinity has gradually declined since then, the salinity gradient has been relatively stable for the last 3000 years (Björck, Citation1995), contrary to estuarine environments where salinity varies with each tidal cycle (e.g. Crain et al., Citation2004), enabling marine species to adapt to the low salinity environment. The salinity gradient of the Baltic Sea surface waters starts at 12 psu at the Öresund and Danish Belts (). Steadily decreasing, the salinity in Baltic Proper is about 7 psu in the southern parts and 6 psu in the northern parts, with slightly higher values on the eastern coast due to the counter-clockwise flow of surface water. In the Bothnian Sea, north of the Archipelago Sea, the salinity is around 5 psu before it drops to 3 psu at the Quark where the Bothnian Bay begins. In the northernmost parts of the Bothnian Bay it is only about 1 psu (Wallentinus, Citation1978; Feistel et al., Citation2010).
Fig. 1. Map of the Baltic Sea showing the approximate distribution of Fucus radicans (orange), Fucus vesiculosus (green) and Fucus serratus (yellow), sampling locations (Järnäs, Drivan, Skagsudde and Gävle) and Askö Laboratory location. Numbers on map refer to surface water salinity in psu. Note that this is not an exact distribution as substrate availability has not been taken into account. Black dotted line marks northern distribution limit of I. balthica.
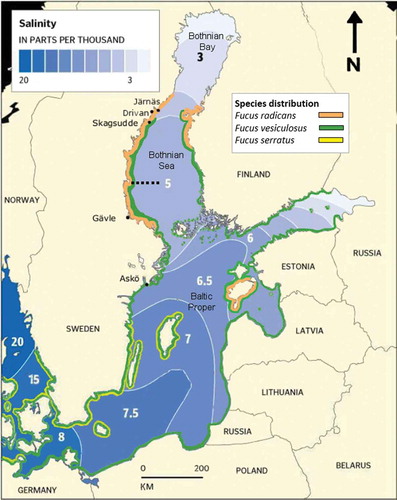
Collected material
For the experiment on eggs released per receptacle, F. radicans and F. vesiculosus were collected in the Bothnian Sea () outside Gävle at a site near a river outlet with fluctuating salinity (between 4–5 psu), where they grow in sympatry, during early May 2011. Fucus radicans was also gathered in the northern Bothnian Sea from Skagsudde, Drivan and Järnäs () during late July 2011 for both the experiment on fertilization success and that of grazing and shading. In both cases the material was transported in moist, cool conditions to the Askö Laboratory (58°49′25′′N, 17°38′13′′E) where it was kept outdoors in flow-through tanks and acclimated (common garden) with brackish water of about 6 psu for about one year until the experiments were performed.
Reproductive output experiment
In May 2012 fertile receptacle-bearing thalli were moved to Aquaria Water Museum (59°19’28.7”N, 18°5’38.3”E) and placed in aquaria with artificially made saltwater (Instant Ocean ®) of 6 psu. The thalli had 2 weeks acclimation until the start of the experiment testing the amount of eggs released per receptacle at different salinities, which was timed to start at the beginning of the reproductive period.
The three salinities used (4, 6 and 12 psu), were chosen because: 4 psu is roughly the lower salinity limit for both F. vesiculosus and F. radicans distribution, 6 psu is approximately the salinity at the southern limit of F. radicans (Forslund et al., Citation2012) and 12 psu (Öresund area, ) is within the salinity range of 10–20 psu where the Baltic Sea adapted ecotype of F. vesiculosus has reached 80–90% reproductive success (Andersson et al., Citation1992; Serrão et al., Citation1996a).
Three receptacles each of 10 randomly chosen female individuals per species were cut off and placed individually in water-filled test tubes with a salinity of either 4, 6 or 12 psu. The saltwater was made by diluting artificially made saltwater (Instant Ocean ®) from 33 psu down to the desired salinities using tap water. The test tubes were sealed with a stopper and kept at field-like conditions, placed in outdoor tanks with flow through seawater, for 50 days. The water in the test tubes was changed every 3–4 days, released eggs being transferred to a clean test tube. Samples were fixed with Lugol’s solution and the number of eggs counted under the dissecting microscope. To relate numbers of eggs released to receptacle size, receptacles were dried at the end of the experiment at 70°C for 24 hours and the dry mass (DM) determined. Some replicates were lost due to either being wrongly sexed (males) or deterioration during the experiment.
To assess the effect of salinity on fertilization success in F. radicans 20 thalli each at three different sites (Järnäs, Drivan and Skagsudde) were gathered. This was to make sure that not only the very dominant female clone was represented in the samples (Johannesson et al., Citation2011). Sampling was done before a full moon and the thalli were separated into males and females without regard to site and the mature receptacles were then cut off. The receptacles were placed in glass beakers and kept damp in dark and cold conditions until about 18:00 h, when water of eight different salinities (3, 3.5, 4, 5, 6, 12, 24 and 30 psu) was added to the beakers containing males and females. To assess whether there is a local adaptation in F. radicans to the low salinities caused by freshwater runoff we also included the two salinities of 3.5 and 3 psu in the experiment. The saltwater was made from natural brackish water from Norrbyn, Umeå (3 psu), which was made more saline by adding a saturated solution of sea salt. The salinity was measured using a digital salinometer (WTW TH 330i). Gamete release was obtained by placing the receptacles under light at 16°C for about 4 h during evening (19:00–22:00 h), when the peak in natural gamete release occurs (Forslund & Kautsky, Citation2013). After gametes were released, the receptacles were removed and each solution of eggs and sperm was cleaned of debris by sieving (mesh size 100 µm), before sperm solution from each salinity treatment was added to the corresponding salinity of egg solution for fertilization (Forslund & Kautsky, Citation2013). After fertilization a cell wall forms around the egg. This was stained with Calcofluor White to make the fertilized eggs fluorescent under UV light (Callow et al., Citation1978; Brawley & Bell, Citation1987). The number of fertilized eggs and total number of eggs were then counted under a UV microscope.
Natural egg release in Baltic Sea of ecotype F. vesiculosus occurs sometime between late afternoon and midnight around the full moon (Serrão et al., Citation1996b, Citation1999). It is not possible to stimulate gamete release in the Baltic Sea ecotype of F. vesiculosus outside its natural circadian rhythm, unlike F. vesiculosus on tidal shores (Brawley, Citation1991; Andersson et al., Citation1994). A small pilot experiment was set up during the natural reproductive period in June 2011 before a full moon to see if gamete release triggered by emersion (desiccation) occurred in F. radicans. Receptacles from several female thalli of F. radicans (Järnäs, Drivan and Skagsudde) and F. vesiculosus (Askö) were removed and placed in one of three treatments: dry (patted dry and placed on dry paper towel), medium (no paper towel), moist (placed on wet paper towel), and the control was completely submersed in water. Each treatment consisted of 50 cm3 of receptacles. The receptacles were left in light conditions at +15°C, for a period of exposure beginning at 14:45. After 2 h, water was added to all treatments and numbers of released eggs and oogonia were counted under a dissecting microscope after another hour.
Shading and grazing experiment
In a cage field experiment, the effects of shading by F. vesiculosus and grazing by the isopod Idotea balthica on F. radicans were tested. In the Bothnian Sea F. radicans can be found from depths of 2 m down to 6–8 m (Råberg & Kautsky, Citation2007). The algae were acclimatized for 2 years to shallow depth light conditions (approximately 2 m) prior to the experiment.
Four treatments: shading, grazing, grazing + shading and control were created, with n=6 for each treatment. Fronds of F. radicans were weighed (approximately 30–40 g wet mass (WM) per treatment) after being gently dried on a paper towel and then tied to a small ceramic tile to prevent drifting. The shading treatment was created by placing short apical branches of F. vesiculosus with air bladders and no marks of previous grazing in the cage. This floating cover at the top of the cage provided a light reduction of 85% (HOBO Pendant©, 8K, Onset, USA) with some variation due to water movement. Such an amount of shading has been shown to have an effect on F. vesiculosus according to Middelboe et al. (Citation2006). Grazing was created by adding seven large (0.53 ± 0.21 g WM per cage) I. balthica individuals to each replicate (n = 12) to provide an adequate grazing pressure of reported normal natural densities (Engkvist et al., Citation2000; Orav-Kotta & Kotta, Citation2004). The cages used were 20 ×20 ×25 cm (width ×depth ×height) with a mesh size of 1 mm and legs 20 cm in length. The cages were placed on soft sediment with the bottom situated 10 cm above the sediment surface. The top lid was completely sealed to the sides by Velcro, to ensure no escape or entry by small invertebrates. The shading caused by the mesh of the cage (control treatment) was equal to the light attenuation at about 2 m depth (LI-1000 DataLogger, LI-COR).
The closed cages were placed at 0.5 m depth in a sheltered bay outside the Askö Laboratory during late summer (July–September 2013) for 7 weeks in a salinity of about 6 psu. To avoid further shading by fouling and debris, the cages were checked several times during the experiment and after 3 weeks they were cleaned on the outside using a soft brush. At the end of the experiment, the fronds of F. radicans were untied from the tiles and gently dried on a paper towel before being weighed, measuring biomass production as change in g WM. We assumed that the F. vesiculosus used as shading was not grazed upon by I. balthica and would not bias the results, as I. balthica has been shown to prefer F. radicans over F. vesiculosus (Gunnarsson & Berglund, Citation2011; Forslund et al., Citation2012). When the F. vesiculosus tips were examined after the study no grazing marks were found.
Statistical analyses
Egg release and fertilization success were both analysed using a one-way ANOVA with salinity as fixed factor and Tukey HSD post hoc test. The egg release of the two species was analysed separately for each species. The change in biomass attributed to shading and grazing was analysed using a two-way ANOVA. All analyses were made using R version 2.12.1. The numbers of released eggs per receptacle DM at different salinities were log transformed to meet requirements for normal distribution.
Results
Reproductive output experiment
Fucus radicans released more eggs per mg DM reproductive tissue with increasing salinity (). In the low salinity treatment of 4 psu F. radicans released a lower total number of eggs per mg DM receptacle after 50 days compared with both 6 and 12 psu (P = 0.012 and p = 0.001 respectively, Tukey HSD post hoc). The higher salinities, however, did not differ from each other. Fucus vesiculosus showed no difference at all between salinities tested (), even when the outlier present in the 12 psu treatment was removed (F = 1.5148, P = 0.2452).
Fig. 2. Total number of eggs released per mg DM receptacle in three salinities (4, 6 and 12 psu) for (a) Fucus radicans and (b) Fucus vesiculosus measured over 50 days during the reproductive season (May–June) 2012. Error bars show mean and 95 % confidence interval. Letters (A–B) over bars in (a) show groups that differ significantly according to Tukey HSD post hoc test.
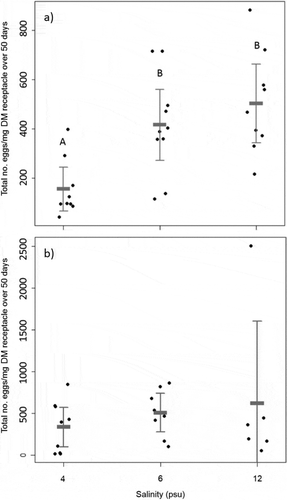
The number of eggs released per mg DM after 50 days, i.e. covering the whole reproductive period, did not differ between F. vesiculosus and F. radicans (). However, the receptacle DM of F. vesiculosus was more than four times larger than F. radicans, 1.23 ± 0.5 mg compared with 0.28 ± 0.07 mg average DM, which means that F. vesiculosus released a larger total number of eggs than F. radicans per receptacle.
Table 1. Two-way ANOVA of total number of eggs released per mg reproductive tissue (DM) between Fucus vesiculosus and F. radicans (Species) in three different salinities 4, 6 and 12 psu (Salinity). Data were log transformed to meet the assumption of variance homogeneity. Bold P values indicate significant effects (p < 0.05).
The success of fertilization in F. radicans increased with salinity (ANOVA, P < 0.001, n= 6), where the highest salinities of 24 and 30 psu had almost 100% success in fertilization (). The salinities ambient to the Bothnian Sea of 4 and 5 psu had less than 20% fertilized eggs. There was no difference between the two lowest salinities of 4 and 5 psu, or between the two highest, 24 and 30 psu (Tukey HSD post hoc). At 6 psu the fertilization success increased dramatically to about 70%, indicating a critical salinity barrier for sexual recruitment between salinities of 5–6 psu. The slight but significant decrease at 12 psu, however, was not expected. The results from treatments of 3.5 and 3 psu are not presented since the eggs underwent rapid lysis and could not be counted. Thus, salinity of around 3.5–4 psu was deemed the limit for successful fertilization.
Fig. 3. Fertilization success (%) in Fucus radicans at six different salinities (4, 5, 6, 12, 24 and 30 psu, n = 6). Error bars show mean and 95 % confidence interval. Letters (A–D) above bars show groups that differ significantly according to Tukey’s post hoc test.
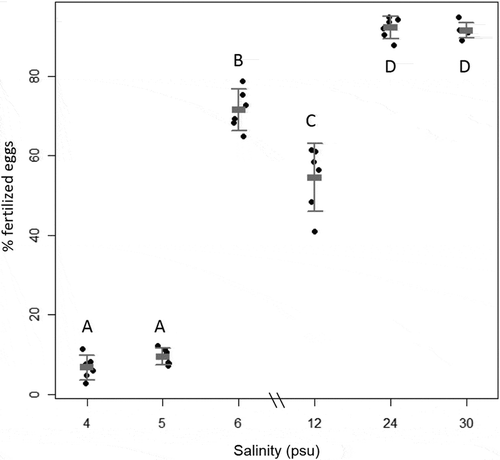
The dry treatment in the pilot experiment released over 1200 eggs and 200 oogonia in F. radicans, whereas the other treatments and the control only produced around 200–300 eggs and 70–200 oogonia each. The number of eggs and oogonia released did not differ between the treatments for F. vesiculosus, varying from 15 to 80, with slightly more oogonia released than eggs. This pilot should, however, only be seen as an observation since we lack replication and the time of day does not totally exclude the effect of natural release.
Shading and grazing
The cage experiment which ran for 7 weeks during July–September 2013 showed that both shading and grazing had a negative effect on F. radicans frond biomass (WM) production (n=6) (). Only the grazed treatment and the grazed+shaded treatment differed from the control (Tukey HSD post hoc), but these treatments did not differ from each other (). The shaded treatment resulted in a 16% increase in biomass, but did not differ from the control (two-way ANOVA), which increased by 22% in biomass, or from the grazed treatment (8.5% increase in biomass). The combined treatment of grazing and shading only increased by 0.8% in biomass and this was the only treatment in which some replicates showed a net loss in biomass.
Table 2. Two-way ANOVA of increase in biomass of F. radicans thalli in shaded (fixed) and grazed (fixed) treatments and a combination of both treatments. Bold P-values indicate significant effects (P < 0.05).
Discussion
High salinity promoted reproductive output of F. radicans. Both the number of eggs produced and the fertilization success of F. radicans were positively affected by an increase in salinity from 4 to 6 psu. The results for these traits are similar to those of the sister species, F. vesiculosus. Our two experiments on reproductive traits show that, as hypothesized, F. radicans performs better in more marine conditions and that F. radicans could very well thrive in more saline areas. The experiment determining the limiting effects of shading and grazing showed that grazing alone as well as the additive effect of both factors severely limited the biomass production in F. radicans.
Reproductive output
Even though F. radicans released fewer eggs in total per receptacle at all three tested salinities compared with F. vesiculosus, there was no difference between the two species regarding eggs produced per unit dry mass (DM) of reproductive tissue. The total number of eggs released per receptacle for F. vesiculosus was approximately 6000 during the reproductive period (May–July), similar to previous studies of F. vesiculosus (Serrão et al., Citation1996b; Malm & Kautsky, Citation2003) in the Baltic Proper. Our results show that the sexual reproductive success rate of F. radicans will be greatly reduced if the salinity drops below 4 psu. All the eggs in the 3.0 and 3.5 psu treatments underwent lysis, probably supporting the findings by Serrão et al. (Citation1996a) that fertilization in these low salinities is impeded by the low osmolality of the water. As the sexual reproduction of Fucus spp. is limited in time (Andersson et al., Citation1994), and also by dispersal range (Serrão et al., Citation1997), a decrease in salinity during the reproductive period, e.g. from increasing precipitation and river runoff, may have a large impact on the annual reproductive success. Thus a salinity of 4 psu seems to be the limit for F. radicans to successfully form a zygote, which also agrees with the northernmost distribution documented by Forslund et al. (Citation2012). Populations of F. radicans at sites where upwelling events occur might therefore have a higher sexual reproductive output and thus have higher genetic variation than those populations that rely more on clonal reproduction as seen in the genotype maps by Johannesson et al. (Citation2011) and Ardehed et al. (Citation2015).
However, F. radicans recruitment is not restricted by the sensitive sexual stage. The ability to reproduce asexually seems to play a vital part in the survival of the populations of F. radicans in the Bothnian Sea, north of the F. vesiculosus range limit (Forslund et al., Citation2012; Ardehed et al., Citation2015). This would explain the northern outlier population on the Finnish coast at Brahestad documented by Pekkari (Citation1973). The counter-clockwise surface current of the Baltic Proper and Bothnian Sea might also affect the northern limit of F. radicans on the Swedish coast, possibly preventing fragment dispersal northwards. The surface current direction could in the same way also prevent F. radicans from spreading further south on the Finnish coast, although salinity is higher than on the equivalent latitude on the Swedish coast (Pekkari, Citation1973; Feistel et al., Citation2010). The direction of the main surface current would, however, favour dispersal further southwards on the Swedish coast, and it is possible that since F. radicans is a young species (Pereyra et al., Citation2009) it is still moving south. We have shown that F. radicans sexual reproduction is not restricted by the higher salinity of the Baltic Proper. Thus, it might be biotic factors, i.e. competition by F. vesiculosus combined with grazing by Idotea spp. that prevent F. radicans from entering the Baltic Proper.
Shading and grazing
Increasing salinity also means higher densities of grazers, especially Idotea spp. and more competition with F. vesiculosus. Our results showed no direct negative effect of shading (i.e. competition) on F. radicans biomass production. This might be explained by seaweed thalli constantly moved to and fro by the water motion, providing enough light for photosynthesis and growth. In the Bothnian Sea F. vesiculosus and F. radicans also have about the same thallus height (15–20 cm). Thus, we propose that the impact of shading by F. vesiculosus is mainly expected to occur at the southern range margin in the Baltic Proper where F. vesiculosus achieves about twice the size of F. radicans (Forslund et al., Citation2012).
We also hypothesized that grazing would affect the southern distribution range. The increasing salinity promotes higher densities of grazers (Salemaa, Citation1979). Grazers living on F. vesiculosus might prefer to forage on F. radicans; studies by Gunnarsson & Berglund (Citation2011) and Forslund et al. (Citation2012) show that F. radicans is more heavily grazed by I. balthica than F. vesiculosus. The results of the field cage experiment showed that grazing by I. balthica, both alone and in combination with shading, had the most negative effect on net biomass production. The results support our hypothesis that the increased levels of shading and grazing in the higher salinity of the Baltic Proper might be a barrier preventing the southwards distribution of F. radicans, even though the reproductive output and fertilization success increased in the higher salinities. Large-scale decline of F. vesiculosus caused by grazing during high densities of Idotea spp. has been documented in the Baltic Sea (Rönnberg et al., Citation1985; Engkvist et al., Citation2000; Nilsson et al., Citation2004). Observations describe how the Idotea spp. first aggregate on the deepest growing Fucus thalli, i.e. the most shaded conditions (Kangas et al., Citation1982; Jormalainen & Ramsay, Citation2009), indicating that reduced light conditions might hamper the production of anti-grazing chemicals in fucoids (Hemmi et al., Citation2004; Wahl et al., Citation2011). This pattern was not seen in our results, since the two treatments with grazing as a factor did not differ from each other, even though some replicates in the combined treatment alone showed a net loss of biomass.
Fucus radicans is considered to be the only species endemic to the Baltic Sea (Pereyra et al., Citation2009, Citation2013) and as such, one might assume that it would be optimally adapted to its brackish conditions. We do not yet know if F. radicans has successful sexual reproduction every year, or how much asexual reproduction contributes annually to the population increase. There is an outlier population of F. radicans present around the island Saaremaa in Estonia (not part of this paper) which is believed to reproduce only sexually, as no clonality was recorded there by Johannesson et al. (Citation2011) or Pereyra et al. (Citation2013) although sample sizes were small. In this study we have shown that, in egg production as well as fertilization success, F. radicans shows a higher fitness with increasing salinities. This might indicate a somewhat stronger link to its marine origin than its sister species. Although many marine traits are similar in F. radicans and the Baltic Sea ecotype of F. vesiculosus (Serrão et al., Citation1999; Pearson et al., Citation2000) some traits present in North Sea populations of F. vesiculosus, like the stress-triggered gamete release (Anderson et al., 1994, and this study) are present in F. radicans but no longer found in the Baltic Sea ecotype of F. vesiculosus. We propose that since the rate of adaptation through recombination would be slower in F. radicans due to the extensive contribution of asexual reproduction, F. radicans has also been able to maintain more marine traits than F. vesiculosus despite the brackish conditions. The time of divergence between the two species possibly occurred quite soon after the marine inflow to the Baltic Sea, which would explain why some marine traits have remained in F. radicans, such as the stress-induced trigger of gametes which has since been lost in the Baltic ecotype of F. vesiculosus. If the salinity of the Baltic Sea decreases in the future, as predicted by some models (Rutgersson et al., Citation2002; Meier et al., Citation2012) an ensuing decrease in grazers and competition could enable the distribution of F. radicans to shift south, as it is capable of reproduction in lower salinities than F. vesiculosus. In a future Baltic Sea ecosystem, F. radicans might become the single foundation species.
Acknowledgements
The authors wish to thank the water museum Aquaria and its staff for letting space and helping out, and H. Forslund and P. Hansson for assistance in the field. Also, E. Serrão, O. Eriksson and S. Wikström are thanked for their valuable input to the manuscript.
DISCLOSURE STATEMENT
No potential conflict of interest was reported by the author(s).
Additional information
Funding
Notes on contributors
E. Schagerström
Both authors contributed equally to all parts of the paper.
L. Kautsky
Both authors contributed equally to all parts of the paper.
REFERENCES
- Andersson, S., Kautsky, L. & Kautsky, N. (1992). Effects of salinity and bromine on zygotes and embryos of Fucus vesiculosus from the Baltic Sea. Marine Biology, 114: 661–665.
- Andersson, S., Kautsky, L., & Kalvas, A. (1994). Circadian and lunar gamete release in Fucus vesiculosus in the atidal Baltic Sea. Marine Ecology Progress Series, 110: 195–201.
- Ardehed, A., Johansson, D., Schagerström, E., Kautsky, L., Johannesson, K. & Pereyra, R.T. (2015). Complex spatial clonal structure in the macroalgae Fucus radicans with both sexual and asexual recruitment. Ecology and Evolution, doi: 10.1002/ece3.1629.
- Bäck, S., Collins, J.C. & Russell, G. (1992). Effects of salinity on growth of Baltic and Atlantic Fucus vesiculosus. British Phycological Journal, 27: 39–47.
- Bergström, L., Bruno, E., Eklund, B. & Kautsky, L. (2003). Reproductive strategies of Ceramium tenuicorne near its inner limit in the brackish Baltic Sea. Botanica Marina, 46: 125–131.
- Bergström, L., Tatarenkov, A., Johannesson, K., Jonsson, R.B. & Kautsky, L. (2005). Genetic and morphological identification of Fucus radicans sp. nov. (Fucales, Phaeophyceae) in the brackish Baltic Sea. Journal of Phycology, 41: 1025–1038.
- Björck, S. (1995). A review of the history of the Baltic Sea, 13.0–8.0 ka BP. Quaternary International, 27: 19–40.
- Brawley, S.H. (1991). The fast block against polyspermy in fucoid algae is an electrical block. Developmental Biology, 144: 94–106.
- Brawley, S.H. & Bell, E. (1987). Partial activation of Fucus eggs with calcium ionophores and low-sodium seawater. Developmental Biology, 122: 217–226.
- Brooker, R.W. (2006). Plant–plant interactions and environmental change. New Phytologist, 171: 271–284.
- Callaway, R.M., Brooker, R., Choler, P., Kikvidze, Z., Lortie, C.J., Michalet, R., Paolini, L., Pugnaire, F.I., Newingham, B. & Aschehoug, E.T. (2002). Positive interactions among alpine plants increase with stress. Nature, 417: 844–848.
- Callow, M.E., Evans, L.V., Bolwell, G.P. & Callow, J.A. (1978). Fertilization in brown algae. I. SEM and other observations on Fucus serratus. Journal of Cell Science, 32: 45–54.
- Crain, C.M., Silliman, B.R., Bertness, S.L. & Bertness, M.D. (2004). Physical and biotic drivers of plant distribution across estuarine salinity gradients. Ecology, 85: 2539–2549.
- Engkvist, R., Malm, T. & Tobiasson, S. (2000). Density dependent grazing effects of the isopod Idotea baltica Pallas on Fucus vesiculosus L. in the Baltic Sea. Aquatic Ecology, 34: 253–260.
- Eriksson, B.K., Rubach, A. & Hillebrand, H. (2006). Community dominance by a canopy species controls the relationship between macroalgal production and species richness. Limnology and Oceanography, 51: 1813–1818.
- Feistel, R., Weinreben, S., Wolf, H., Seitz, S., Spitzer, P., Adel, B., Nausch, G., Schneider, B. & Wright, D. (2010). Density and absolute salinity of the Baltic Sea 2006–2009. Ocean Science, 6: 3–24.
- Forslund, H. & Kautsky, L. (2013). Reproduction and reproductive isolation in Fucus radicans (Phaeophyceae). Marine Biology Research, 9: 321–326.
- Forslund, H., Eriksson, O. & Kautsky, L. (2012). Grazing and geographic range of the Baltic seaweed Fucus radicans (Phaeophyceae). Marine Biology Research, 8: 386–394.
- Gogina, M. & Zettler, M.L. (2010). Diversity and distribution of benthic macrofauna in the Baltic Sea: data inventory and its use for species distribution modelling and prediction. Journal of Sea Research, 64: 313–321.
- Gunnarsson, K. & Berglund, A. (2011). The brown alga Fucus radicans suffers heavy grazing by the isopod Idotea baltica. Marine Biology Research, 8: 87–89.
- Hällfors, G., Niemi, Å., Ackefors, H., Lassig, J., & Leppäkoski, E. (1981). Biological oceanography. In The Baltic Sea (Voipio, A., editor), 219–274. Elsevier, Amsterdam.
- Hemmi, A., Honkanen, T., & Jormalainen, V. (2004). Inducible resistance to herbivory in Fucus vesiculosus – duration, spreading and variation with nutrient availability. Marine Ecology Progress Series, 273: 109–120.
- Johannesson, K. & Andre, C. (2006). Life on the margin: genetic isolation and diversity loss in a peripheral marine ecosystem, the Baltic Sea. Molecular Ecology, 15: 2013–2029.
- Johannesson, K., Johansson, D., Larsson, K.H., Huenchuñir, C.J., Perus, J., Forslund, H., Kautsky, L. & Pereyra, R.T. (2011). Frequent clonality in fucoids (Fucus radicans and Fucus vesiculosus; Fucales, Phaeophyceae) in the Baltic Sea. Journal of Phycology, 47: 990–998.
- Jormalainen, V. & Ramsay, T. (2009). Resistance of the brown alga Fucus vesiculosus to herbivory. Oikos, 118: 713–722.
- Kangas, P., Autio, H., Hällfors, G., Luther, H., Niemi, A. & Salemaa, H. (1982). A general model of the decline of Fucus vesiculosus at Tvaerminne, South Coast of Finland, in 1977–81. Acta Botanica Fennica, 118: 1–27.
- Kautsky, N., Kautsky, H., Kautsky, U. & Waern, M. (1986). Decreased depth penetration of Fucus vesiculosus (L.) since the 1940’s indicates eutrophication of the Baltic Sea. Marine Ecology Progress Series, 28: 1–8.
- Kostamo, K. (2008). The life cycle and genetic structure of the red alga Furcellaria lumbricalis on a salinity gradient. Walter and Andrée De Nottbeck Foundation Scientific Reports, 33: 1–34.
- Leidenberger, S., Harding, K. & Jonsson, P.R. (2012). Ecology and distribution of the isopod genus Idotea in the Baltic Sea: key species in a changing environment. Journal of Crustacean Biology, 32: 359–381.
- Malm, T. & Kautsky, L. (2003). Differences in life-history characteristics are consistent with the vertical distribution pattern of Fucus serratus and Fucus vesiculosus (Fucales, Phaeophyceae) in the central Baltic Sea. Journal of Phycology, 39: 880–887.
- Malm, T., Kautsky, L. & Engkvist, R. (2001). Reproduction, recruitment and geographical distribution of Fucus serratus L. in the Baltic Sea. Botanica Marina, 44: 101–108.
- Meier, H.E.M., Andersson, H.C., Arheimer, B., Blenckner, T., Chubarenko, B., Donnelly, C., Eilola, K., Gustafsson, B.G., Hansson, A., Havenhand, J., Höglund, A., Kuznetsov, I., Mackenzie, B.R., Müller-Karulis, B., Neumann, T., Niiranen, S., Piwowarczyk, J., Raudsepp, U., Reckermann, M., Ruoho-Airola, T., Savchuk, O.P., Schenk, F., Schimanke, S., Väli, G., Weslawski, J.M. & Zorita, E. (2012). Comparing reconstructed past variations and future projections of the Baltic Sea ecosystem – first results from multi-model ensemble simulations. Environmental Research Letters, 7(3): 034005.
- Middelboe, A.L., Sand-Jensen, K. & Binzer, T. (2006). Highly predictable photosynthetic production in natural macroalgal communities from incoming and absorbed light. Oecologia, 150: 464–476.
- Naylor, E. (1955). The ecological distribution of British species of Idotea (Isopoda). Journal of Animal Ecology, 24: 255–269.
- Nilsson, J., Engkvist, R. & Persson, L.E. (2004). Long-term decline and recent recovery of Fucus populations along the rocky shores of southeast Sweden, Baltic Sea. Aquatic Ecology, 38: 587–598.
- Orav-Kotta, H. & Kotta, J. (2004). Food and habitat choice of the isopod Idotea baltica in the northeastern Baltic Sea. Hydrobiologia, 514: 79–85.
- Pearson, G., Kautsky, L. & Serrão, E. (2000). Recent evolution in Baltic Fucus vesiculosus: reduced tolerance to emersion stresses compared to intertidal (North Sea) populations. Marine Ecology Progress Series, 202: 67–79.
- Pedersen, M.F., Nejrup, L.B., Fredriksen, S., Christie, H. & Norderhaug, K.M. (2012). Effects of wave exposure on population structure, demography, biomass and productivity of the kelp Laminaria hyperborea. Marine Ecology Progress Series, 451: 45–60.
- Pekkari, S. (1973). Effects of sewage water on benthic vegetation. OIKOS Supplementum, 15: 185–188.
- Pereyra, R.T., Bergström, L., Kautsky, L. & Johannesson, K. (2009). Rapid speciation in a newly opened postglacial marine environment, the Baltic Sea. BMC Evolutionary Biology, 9: 70.
- Pereyra, R.T., Huenchuñir, C., Johansson, D., Forslund, H., Kautsky, L., Jonsson, P.R. & Johannesson, K. (2013). Parallel speciation or long-distance dispersal? Lessons from seaweeds (Fucus) in the Baltic Sea. Journal of Evolutionary Biology, 26: 1727–1737.
- Remane, A. & Schlieper, C. (1971). Biology of brackish water. E Schweizerbart’sche Verlagsbuchhandlung (Nägele u. Obermiller), Stuttgart.
- Russell, G. (1985). Recent evolutionary changes in the algae of the Baltic Sea. British Phycological Journal, 20: 87–104.
- Rutgersson, A., Omstedt, A. & Raisanen, J. (2002). Net precipitation over the Baltic Sea during present and future climate conditions. Climate Research, 22: 27–39.
- Råberg, S. & Kautsky, L. (2007). A comparative biodiversity study of the associated fauna of perennial fucoids and filamentous algae. Estuarine Coastal and Shelf Science, 73: 249–258.
- Rönnberg, O., Lehto, J. & Haahtela, I. (1985). Recent changes in the occurrence of Fucus vesiculosus in the Archipelago Sea, SW Finland. Annales Botanici Fennici, 22: 231–244.
- Salemaa, H. (1979). Ecology of Idotea spp. (Isopoda) in the northern Baltic. Ophelia, 18: 133–150.
- Santelices, B. & Varela, D. (1993). Intra-clonal variation in the red seaweed Gracilaria chilensis. Marine Biology, 116: 543–552.
- Serrão, E.A., Kautsky, L. & Brawley, S.H. (1996a). Distributional success of the marine seaweed Fucus vesiculosus L in the brackish Baltic Sea correlates with osmotic capabilities of Baltic gametes. Oecologia, 107: 1–12.
- Serrão, E.A., Pearson, G., Kautsky, L. & Brawley, S.H. (1996b). Successful external fertilization in turbulent environments. Proceedings of the National Academy of Sciences USA, 93: 5286–5290.
- Serrão, E.A., Kautsky, L., Lifvergren, T. & Brawley, S.H. (1997). Gamete dispersal and pre-recruitment mortality in Baltic Fucus vesiculosus. Phycologia, 36: 101–102.
- Serrão, E.A., Brawley, S.H., Hedman, J., Kautsky, L. & Samuelson, G. (1999). Reproductive success of Fucus vesiculosus (Phaeophyceae) in the Baltic Sea. Journal of Phycology, 35: 254–269.
- Sjøtun, K., Christie, H. & Helge Fosså, J. (2006). The combined effect of canopy shading and sea urchin grazing on recruitment in kelp forest (Laminaria hyperborea). Marine Biology Research, 2: 24–32.
- Sjøtun, K., Eggereide, S.F. & Höisäter, T. (2007). Grazer-controlled recruitment of the introduced Sargassum muticum (Phaeophyceae, Fucales) in northern Europe. Marine Ecology Progress Series, 342: 127–138.
- Snoeijs, P. (1999). Marine and brackish waters. Acta Phytogeographica Suecia, 84: 187–212.
- Tatarenkov, A., Bergström, L., Jonsson, R.B., Serrão, E.A., Kautsky, L. & Johannesson, K. (2005). Intriguing asexual life in marginal populations of the brown seaweed Fucus vesiculosus. Molecular Ecology, 14: 647–651.
- Tatarenkov, A., Jonsson, R.B., Kautsky, L. & Johannesson, K. (2007). Genetic structure in populations of Fucus vesiculosus (Phaeophyceae) over spatial scales from 10 m to 800 km. Journal of Phycology, 43: 675–685.
- Wahl, M., Jormalainen, V., Eriksson, B.K., Coyer, J.A., Molis, M., Schubert, H., Dethier, M., Karez, R., Kruse, I., Lenz, M., Pearson, G., Rohde, S., Wikström, S.A. & Olsen, J.L. (2011). Stress ecology in Fucus: abiotic, biotic and genetic interactions. In Advances in Marine Biology (Michael, L., editor), 37–105. Academic Press, London.
- Wallentinus, I. (1978). Productivity studies on Baltic macroalgae. Botanica Marina, 21: 365–380.
- Wright, J.T. & Davis, A.R. (2006). Demographic feedback between clonal growth and fragmentation in an invasive seaweed. Ecology, 87: 1744–1754.