Abstract
Two European species of Gracilaria possess flattened blades borne on cylindrical axes, namely, G. multipartita, known primarily from the Atlantic coast, and G. corallicola from the Mediterranean Sea. They are sister species that cluster with G. armata, G. bursa-pastoris and G. longa in rbcL analyses with strong bootstrap support. Blades of G. multipartita taper towards the tips, whereas those of G. corallicolla have broadly rounded tips. Spermatangia of G. corallicola are borne in shallow conceptacles (textorii-type) and data from the literature indicate that the same is true of G. multipartita. Cystocarp morphology is similar, with the gonimoblast filaments initially elongated, narrow and densely filled with cytoplasm, and with tubular nutritive cells issuing initially from lower gonimoblast cells and fusing with cells in the lowermost regions of the outer pericarp. Tetrasporangia are initiated terminally and displaced laterally with the production of side branches from the subterminal cell. The diagnostic characters of the Gracilariaceae are reviewed from a developmental perspective.
Introduction
Gracilaria multipartita (Clemente) Harvey and G. corallicola Zanardini are morphologically similar European species of Gracilaria (Gracilariales, Rhodophyta), having broadly flattened blades borne on cylindrical stipes. Gracilaria multipartita was first published as Fucus multipartitus by Clemente y Rubio (1807: 311) based on a series of collections from Andalusia, Spain. Previously, Fucus granateus J.V. Lamouroux (Citation1805: 68, pl. XXXIII; , ) was established upon a collection by M. Perrein from the port of La Coruña, Galicia, Spain and one by himself from Biarritz, Pyrénées-Atlantiques, France. The species was described as consisting of one to several firm, dichotomously branched narrow fronds that broadened into membranous undulating blades with rounded apices, and as having tubercles the size of poppy seeds scattered over both surfaces in the middle of the blades and surmounted by membranous creno-dentate coronae. Based on Lamouroux’s description, Turner (1808: 151) placed this plant under Fucus laceratus (= Cryptopleura ramosa (Hudson) L. Newton), γ granateus. Later, Turner (1819: pl. 215) realized that his and Lamouroux’s specimens were not thin and membranous but thick and cartilaginous, and bore no resemblance to F. laceratus. He corresponded with Clemente who sent him specimens of F. multipartitus and with Lamouroux who provided fertile material of F. granateus. A comparison of the two collections led Turner to conclude that they were referable to the same species with F. granateus Lamouroux being the earlier name. A comparison of Turner’s pl. 215, top figure, with a photograph of a Clemente specimen in Guiry & Freamhainn (Citation1986: ) indicates that Turner had portrayed the habit of F. granateus from material of F. multipartitus sent by Clemente and possibly illustrated the reproductive stages below with those sent by Lamouroux. Turner’s revised description could have combined the characters seen in the two collections. Guiry & Guiry (Citation2016) cite G. foliifera f. granatea (J.V Lamouroux) Børgesen (Citation1938: 226) as a current name for this plant; however, Børgesen cited forma granatea (Fucus granateus Turner, 1819: pl. 215), not Lamouroux. Earlier, Harvey (Citation1846: pl. XV) had recognized Fucus granatus sic! Turner, pl. 215 (excl. syn. Lamx.) under Gracilaria multipartita. The Lamouroux specimens have not been reinvestigated and their identities remain unconfirmed.
Fig. 1. Maximum likelihood tree resulting from analysis of rbcL sequences for 41 species of Gracilaria. GenBank accession numbers are shown before the species name for each sequence, and maximum likelihood bootstrap values and Bayesian posterior probabilities are shown for branches when > 50 or > 0.65 respectively.
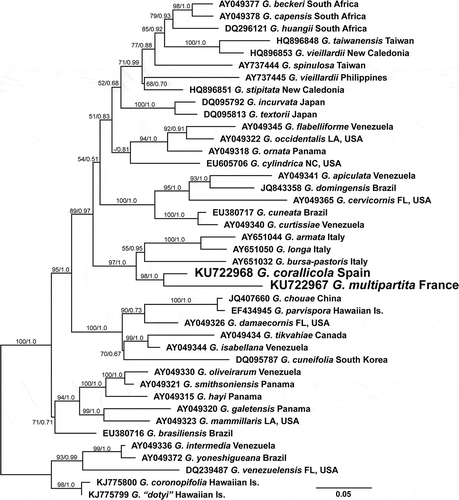
Figs 2–8. Gracilaria corallicola. Habit and vegetative anatomy. Fig. 2. Habit of small erect sterile specimen arising from a long stipe (arrow) (HGI–A 11025). Fig. 3. Adult specimen with spathulate proliferations (arrows) (HGI–A 13122). Fig. 4. Trawled slender specimen (HGI-A 16218). Fig. 5. Detail of branch of a female gametophyte with protruding cystocarps (HGI–A 16509). Fig. 6. Transverse section through median part of thallus (HGI–A 11320). Fig. 7. Cross-section showing inner medulla and transition to the pigmented outer cortex (HGI–A 10363). Fig. 8. Detail of a cross-section showing multinucleate cortical and medullary cells, a deciduous hair primordium and secondary pit connections between medullary and cortical cells and between medullary cells (arrows) (HGI–A 10329). Scale bars: Figs 2–5 = 1 cm; Figs 6, 7 = 100 µm; Fig. 8 = 20 µm. Abbreviations: cy = cystocarp; hp = hair primordium; n = nucleus. Figs 6–8, haematoxylin.
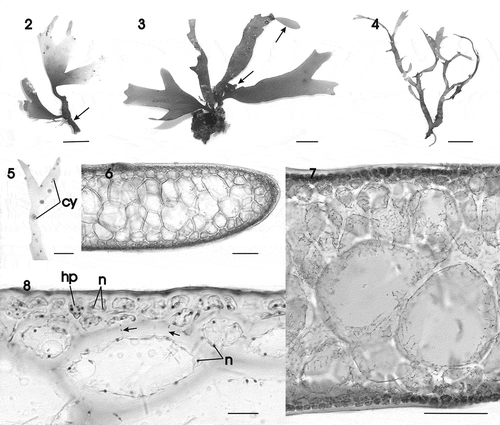
Figs 9–18. Gracilaria corallicola. Vegetative and male reproductive structures. Fig. 9. Apex of thallus in surface view (HGI–A 16242). Fig. 10. Outer cortical cells in surface view showing reticulate plastids and nuclei (HGI–A 10721). Fig. 11. Outer and inner cells of a hair primordium pit-connected to a deciduous hair (HGI–A 16798). Fig. 12. Multinucleate hair primordium in surface view (HGI–A 9645). Fig. 13. Medullary cells showing reticulate plastids containing many pyrenoid-like structures (arrows) (HGI–A 16509). Fig. 14. Multinucleate outer medullary cells linked by secondary pit connections between themselves and with cortical cells (arrows) (HGI–A 16242). Fig. 15. Surface view of thallus showing cortical cells producing uninucleate spermatangial parent cells bearing spermatangia (HGI–A 16312). Fig. 16. Young conceptacle with spermatangia (HGI–A 16312). Fig. 17. Confluent conceptacles containing spermatangia (HGI–A 16312). Fig. 18. Spermatangia bearing spermatia with a distal nucleus and proximal vesicle (HGI–A 16312). Abbreviations: h = hair; hp = hair primordium; n = nucleus; pl = plastid; s = spermatangium; spm = spermatangial mother cells ; v = vesicle. Scale bars: 20 µm. Haematoxylin.
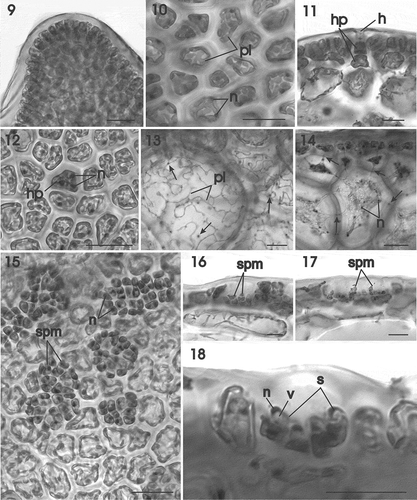
Fucus aeruginosa Turner (1811: pl. 147), whose syntype localities are Red Sea and Portugal, is depicted as a pseudodichotomous plant with numerous lateral proliferations. It is likely that the specimen illustrated by Turner was collected by Viscount Valentia in the Red Sea. This specimen is similar to G. multipartita, and J. Agardh (Citation1852: 600–603) recognized it as G. multipartita var. γ aeruginosa.
Sphaerococcus polycarpus Greville (Citation1822: pl. 352) from below Tait’s Hill, Plymouth, England is a pseudodichotomously branched plant with tapered tips and blades that lack orthogonal branchlets. As such, it is typical of the most common form of G. multipartita encountered in southern England. Greville (Citation1830: 87) transferred his species to Rhodymenia as Rhodomenia polycarpa Greville. Harvey (Citation1846: pl. XV) attributed both G. multipartita and G. polycarpa to J. Agardh (Citation1842: 151), who included both species in a listing of names transferable to Gracilaria. Dixon & Irvine (Citation1977: 208) attributed G. multipartita to Harvey as G. multipartita (Clemente) Harvey without comment. Guiry & Freamhainn (Citation1986: 634) commented that, according to Art. 34.3 of the ICBN (Stafleu & Voss, Citation1978), such incidental mention cannot be regarded as constituting a new name.
Although he was not followed by later authors, J. Agardh (Citation1852: 600–603) recognized the following varieties of Gracilaria multipartita:
α granatea fronds irregularly laciniate, axils acute, terminal ends of laciniae regularly acute;
γ aeruginosa fronds di-polychotomous, with subpinnate ligules (branchlets) along the margins;
β polycarpa fronds di-polychotomous, axils rounded, terminal ends of laciniae regularly obtuse.
Harvey (Citation1846) gave the distribution of G. multipartita as the shores of Europe from the south of England to Spain, East North America, from New York to Florida, California, Pernambuco (Brazil) and the Red Sea. Harvey clearly regarded G. multipartita as being a widely distributed species in warm temperate and tropical waters. When Børgesen (Citation1932) reinvestigated the Forsskål collection from the Red Sea he established G. foliifera (Forsskål) Børgesen as the earliest available name based on F. foliifer Forsskål (Citation1775: 19). His illustration (pl. 1) of the Forsskål type shows a pseudodichotomously branched plant with short lateral proliferations. Gracilaria foliifera retained the same wide distribution under Børgesen that it had under Harvey with extensions into the tropical waters of the Indian Ocean.
A combination of hybridization and morphological studies (McLachlan & Edelstein, Citation1977; McLachlan, Citation1979) established that G. tikvahiae McLachlan from the Atlantic coast of the United States and Canada is distinct from G. multipartita (as G. foliifera) from Devon, England. Guiry & Freamhainn (Citation1986) extended this observation by demonstrating that strains of G. foliifera from Texas and North Carolina were interfertile with a strain of G. tikvahiae from northern Nova Scotia, Canada and did not cross with interfertile strains of G. multipartita (as G. foliifera) from Ireland, England and France. These authors reinstated G. multipartita and also concluded that the European species was separate from G. foliifera from the Indian Ocean and Red Sea. This latter opinion requires confirmation from molecular studies.
Gracilaria corallicola Zanardini (Citation1865: 127, pl. LXXI, –) was established upon specimens growing on a coralline substrate collected in 1841 from the Adriatic Sea on the coast of Dalmatia. Zanardini recognized the similarity of his new species to G. multipartita as described by J. Agardh (Citation1852: 600–603). He differentiated G. corallicola mainly by the shape and location of the cystocarps: scattered over the thallus surface throughout and submersed and hemispherical (mammillate) in G. multipartita and restricted to the tips of the blades and emergent and globose-apiculate in G. corallicola. Hauck (Citation1885: 184) regarded G. corallicola as a variety of G. multipartita.
In this study we investigate Atlantic specimens of G. multipartita from Roscoff, France, and Mediterranean plants of G. corallicola from the Balearic Islands, Spain based on a detailed analysis of their vegetative and reproductive development. A phylogeny of representative species of Gracilaria from the Atlantic Ocean and Mediterranean Sea is presented and evaluated based on analyses of rbcL sequences.
Materials and methods
Morphological observations
Specimens of Gracilaria corallicola were collected by trawling on deep circalittoral detritic bottoms off Mallorca and Menorca (Balearic Islands), Spain, during seven different cruises between 2008 and 2012 (MEDITS_ES_05_08, CANAL 0209, MEDITS_ES_05_11, INDEMARES_CANAL0811, DRAGONSAL 2012, DRAGONSAL 2014), while those of G. multipartita were collected by the personnel of the Station Biologique de Roscoff (France) using scuba. Both species were quite abundant, but due to the collecting method, many specimens of G. corallicola (280 specimens), most of them sterile, were collected, but only 28 G. multipartita specimens were obtained, although in this case specimens were usually fertile. All specimens were studied to confirm their identifications, but only those used to illustrate this article are included here: 1 male gametophyte, 6 female gametophytes, 2 tetrasporophytes and 5 sterile individuals of G. corallicola, and 2 female gametophytes, 2 tetrasporophytes and 1 sterile individual of G. multipartita. Six of the specimens used to obtain morphological characters were also used in genetic analyses.
Specimens were fixed and stored in 5% formalin–seawater and 70% ethanol. Material for microscopic examination was observed in surface view or sectioned by hand, and preparations were stained either with 1% acidified aqueous aniline blue or with Wittmann’s aceto-iron-haematoxylin-chloral hydrate (Wittmann Citation1965) according to the method of Rodríguez-Prieto & Hommersand (Citation2009). Habit pictures were taken with a Canon EOS 350D (Canon, Tokyo, Japan) and photomicrographs were made with an AxioCam MRc attached to an Axioskop 2 plus microscope (Zeiss, Oberchohen, Germany). Voucher specimens were deposited in the herbarium of the University of Girona, Spain (HGI). Herbarium abbreviations follow Thiers (Citation2016).
Molecular methods
Total genomic DNA was extracted from specimens preserved in 70% ethanol using a modification of the Bioline Extract-PCR kit (Bioline USA Inc., Taunton, Massachusetts, USA). Small pieces of thallus (c. 3 mm2) were transferred through two water washes and allowed to soak for 30 min in order to remove the ethanol preservative. This thallus material was then placed in 100 µl of Bioline Extract-PCR kit buffer solution and incubated at 75°C for 1.5 hours with periodic vortexing. Intact thallus that remained after this incubation was broken up using plastic pellet pestles and the enzymatic solution deactivated by heating at 95°C for 10 min. The solution was centrifuged at 16 000 × g for 1 min and the supernatant removed to a clean tube and diluted 1:10 before use as template in polymerase chain reaction (PCR) amplifications.
Plastid-encoded rbcL, psbA and the universal plastid amplicon (UPA) were PCR amplified with MyTaq HS Red DNA polymerase and buffer (Bioline USA Inc.) and the following primers: rbcL – F57, F753, R1111, RrbcSstart (Freshwater & Rueness, Citation1994; Hommersand & Freshwater, Citation2009); psbA– psbAF1, psbAR2 (Yoon et al., Citation2002); UPA – p23SrV-f1, p23Sr-r1 (Sherwood & Presting, Citation2007). PCR products were enzymatically cleaned with illustra ExoStar (GE Healthcare, Buckinghamshire, UK), and sequence reactions were carried out using BDX64 enhancing buffer (Molecular Cloning Lab [MCLAB], San Francisco, California, USA) and BigDye v. 3 (Life Technologies Corp., Grand Island, New York, USA) following the MCLAB BDX64 protocol. Sequencing reactions were cleaned using the ZR DNA Sequencing Clean-up Kit (Zymo Research, Irvine, California, USA) and run on an ABI 3130xl Genetic Analyzer (Life Technologies). Sequence reactions were combined and edited using Sequencher (Gene Codes Corp., Ann Arbor, Michigan, USA) and deposited in GenBank (). The newly generated UPA and psbA sequences were included in GenBank to provide additional context in this database for future studies using molecular assisted alpha taxonomy or molecular assisted identification (Cianciola et al., Citation2010; Mamoozadeh & Freshwater, Citation2011). The rbcL sequences were aligned with Gracilaria species sequences available in GenBank and used for phylogenetic analyses.
Table 1. Collection information and GenBank accession numbers for sequenced specimens.
Phylogenetic analyses
Newly generated rbcL sequences for G. corallicola and G. multipartita were aligned with 39 rbcL sequences available in GenBank from the studies of Gurgel & Fredericq (Citation2004), Gargiulo et al. (Citation2006), Kim et al. (Citation2006), Gurgel et al. (Citation2008), Lin et al. (Citation2012) and Sfriso et al. (Citation2013) using MUSCLE as implemented in Geneious v.8 (Biomatters, Auckland, NZ). This alignment was truncated to sites 209 to 1350 of the 1467 bp rbcL because of variation in the amounts of missing data at the 5’ and 3’ ends of the GenBank sequences.
Maximum likelihood trees were generated using RAxML (Stamatakis, Citation2006) as implemented in Geneious with the data partitioned by codon position, GTR CAT I model, Rapid hill-climbing algorithm and 10 random starting trees. Maximum likelihood bootstrap values were derived from 1000 replications of searches with the same partitioning, Rapid Bootstrapping algorithm, and GTR CAT I model. Bayesian analyses were carried out using MrBayes v.3.2.2 (Huelsenbeck & Ronquist, Citation2001) as implemented in Geneious with the Codon substitution model, gamma rate variation with a proportion of invariable sites and running two simultaneous runs of 3 × 106 generations with sampling every 3000 generations and a burn-in of 750 000 generations.
Results
Molecular analyses
Partial DNA sequences for plastid-encoded rbcL were generated from one specimen of G. multipartita, three specimens of G. corallicola, and two specimens of unusual morphology identified as Gracilaria sp. (). The G. multipartita specimen was collected from Roscoff, France and its rbcL sequence was 0.2% different from that of a sequence of a G. multipartita specimen from nearby Carantec, France available in GenBank. All three sequences from the G. corallicola specimens as well as the two Gracilaria sp. sequences were identical. These five specimens were trawled from detritic bottoms at various sites between 47 and 70 m depth around the Balearic Islands. Interspecific rbcL sequence divergences between G. corallicola and G. multipartita specimens ranged from 2.6% to 3.2%.
The analysed 41-taxon rbcL alignment included 1142 sites of which 297 (26%) were variable. Maximum likelihood and Bayesian analyses resolved G. corallicola and G. multipartita as strongly supported sister species (maximum likelihood bootstrap [ML] = 98; Bayesian posterior probability [PP] = 1.0) within a strongly supported (ML = 97; PP = 1.0) clade that also included G. armata, G. longa and G. bursa-pastoris ().
Morphological observations
Gracilaria corallicola Zanardini Citation1865: 127–130, pl. 71, –.
–.
Figs 19–28. Gracilaria corallicola. Female reproductive structures and post-fertilization stages. Fig. 19. Supporting cell bearing a conical uninucleate carpogonial branch initial and two side branches. All cells are linked by pit connections (arrows) (HGI–A 11361). Fig. 20. Three-celled carpogonial branch composed of a supporting cell, terminal carpogonium and subterminal hypogynous cell (HGI–A 11361). Fig. 21. The carpogonium is elongated by an outwardly directed trichogyne that does not extend above the thallus surface (HGI–A 11361). Fig. 22. Binucleate carpogonium after fertilization (HGI–A 11361). Fig. 23. Post-fertilization stage showing the carpogonial branch flanked by side branches and surmounted by developing outer pericarp (HGI–A 16798). Fig. 24. Later stage showing fusion of the carpogonium with a side branch (arrowhead) and the initiation of a gonimoblast filament. Note the large presumably diploid nucleus inside the carpogonium (HGI–A 11361). Fig. 25. Multinucleate carpogonial fusion cell at base of outer pericarp (HGI–A 11361). Fig. 26. Initiation of cavity (arrow) between outer and inner pericarp (HGI–A 11361). Fig. 27. Young cystocarp with tubular nutritive cell (arrow) penetrating lower part of outer pericarp (HGI–A 16509). Fig. 28. Young cystocarp with tubular nutritive cell (arrow) penetrating upper part of outer pericarp (HGI–A 16798). Scale bars: Figs 19–26, 28 = 20 µm; Fig. 27 = 200 µm. Figs 19–22, 24–28 haematoxylin; Fig. 23 aniline blue. Abbreviations: ca = carposporangium; cbi = carpogonial branch initial, cfc = carpogonial fusion cell; cp = carpogonium; dn = diploid nucleus; gf = gonimoblast filament; hy = hypogynous cell; ip = inner pericarp; n = nucleus; op = outer pericarp; sb = side branch; sc = supporting cell; t = trichogyne.
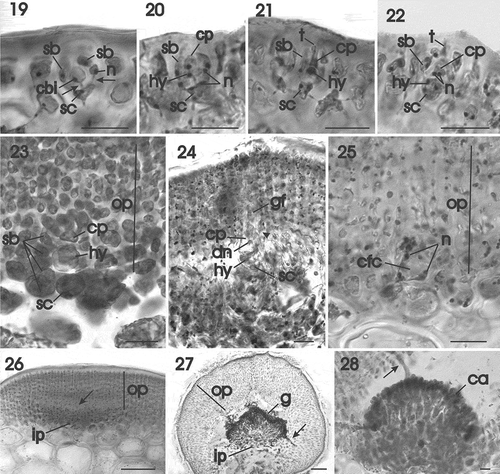
Figs 29–39. Gracilaria corallicola. Post-fertilization stages and tetrasporangia. Fig. 29. Carpogonial fusion cell incorporating vegetative cells (arrows) situated at base of mature gonimoblast filaments (HGI–A 10363). Fig. 30. Mature ostiolate cystocarp with narrow gonimoblast filaments bearing branched chains of carposporangia (HGI–A 10363). Fig. 31. Longitudinal section of inner pericarp and network of interconnected cells (arrow) from which the gonimoblast filaments arise (HGI–A 10363). Fig. 32. Multinucleate tubular filaments (arrows) originating from the gonimoblast and fusing with cells of the outer pericarp (HGI–A 16798). Fig. 33. Growing region of the outer pericarp with the inner cells connected by secondary pit connections (arrows) (HGI–A 16798). Fig. 34. Stellate cells of inner region of the outer pericarp, connected by secondary pit connections (arrows) (HGI–A 16798). Fig. 35. Gonimoblast filaments forming branched chains of carposporangia terminally (HGI–A 16798). Fig. 36. Scattered tetrasporangia in surface view (arrows) (HGI–A 10721). Fig. 37. Cross section of an inflated fertile area showing the latero–basal connection between a tetrasporangial initial and a subcortical cell (arrow) (HGI–A 11609). Fig. 38. Young once-divided tetrasporangium showing connection with subcortical cell (arrow) (HGI–A 11609). Fig. 39. Mature cruciately divided tetrasporangium pit-connected with subcortical cell (arrow) and surrounded by cortical filaments (HGI–A 11609). Scale bars: Figs 29, 37 = 50 µm; Fig. 30 = 200 µm; Fig. 31 = 100 µm; Figs 32–36, 38, 39 = 20 µm. Figs 29–30, 32–37 haematoxylin; Figs 31, 38, 39, aniline blue.
Abbreviations: ca = carposporangium; cfc = carpogonial fusion cell; g = gonimoblast; gf = gonimoblast filament; ip = inner pericarp; n = nucleus; op = outer pericarp; sb = side branch.
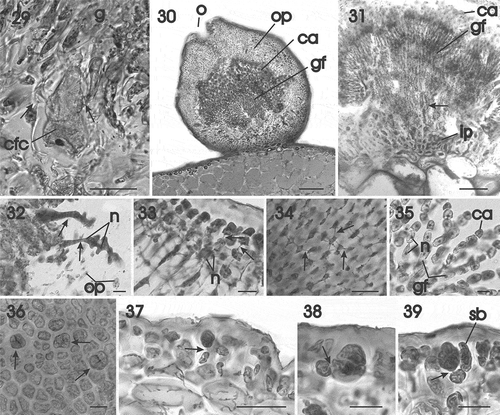
Synonymy: Rhodymenia corallicola (Zanardini) Ardissone Citation1878.
Holotype: Specimen from Dalmatia [Croatia] collected by Zanardini, no date indicated, no habitat data on type sheet; however, Gargiulo et al. (1992: 61) indicated that plants had been dredged and were located in the Zanardini herbarium (Zanardini-514), Museo Civico di Storia Naturale, Venice.
Distribution: Mediterranean Sea (Guiry & Guiry, Citation2016). Records from Eritrea (Ateweberhan & Prud’homme van Reine, Citation2005), Indonesia (Tseng & Xia, Citation1999), Britain (Newton, Citation1931) and California (Dawson, Citation1941) require verification.
Habitat: Circalittoral on dendritic substrates.
Specimens examined: Balearic Islands, Spain. Menorca channel, 5 March 2009, -68.7 m, S. Joher, HGI-A 11609, tetrasporophyte; 10 March 2009, -59 m, S. Joher, HGI-A 10721, tetrasporophyte; 05 September 2011, -73 m, S. Joher, HGI-A 11361, female gametophyte; 8 September 2011, -64 m, S. Joher, HGI-A 11320; 9 September 2011, -55 m, S. Joher, HGI-A 10363, female gametophyte; 13 September 2011, -59.6 m, S. Joher, HGI-A 11025; Sóller, Mallorca, 9 June 2008, - 63 m, S. Joher, HGI-A 9645; Santanyí, Mallorca, 10 June 2011, -94 m, C. Rodríguez-Prieto, HGI-A 13122 female gametophyte; South Mallorca, 3 September 2014, -56 m, C. Rodríguez-Prieto, HGI-A 16242, female gametophyte; HGI-A 16218; South Mallorca, 4 September 2014, -65 m, C. Rodríguez-Prieto, HGI-A 16312, male gametophyte; 7 September 2014, -56 m, C. Rodríguez-Prieto, HGI-A 16509, female gametophyte; 8 September 2014, -60 m, C. Rodríguez-Prieto, HGI-A 16798, female gametophyte; Cabrera, 8 June 2011, -57 m, C. Rodríguez-Prieto, HGI-A 10329.
Molecular vouchers: Specimen HGI-A 16312, rbcL – KU722970; Specimen HGI-A 16450, rbcL – KU722971; Specimen HGI-A 16602, rbcL – KU722972; Specimen HGI-A 16194, rbcL – KU722969; Specimen HGI-A 16172, rbcL – KU722968; psbA – KU745458; UPA – KU739403.
Habit and vegetative structure
Thallus erect, flattened, to 13 cm high, attached to the substratum by a discoid holdfast from which one to several axes arise (, ). Axes stipitate, with a cylindrical stipe up to 20 mm long and 2.5 mm in diameter (, arrow), expanding abruptly to flattened, subdichotomously to palmately branched blades with rounded apices (, ). Blades up to 10 mm wide between dichotomies and 0.6 mm thick at the base. Margins smooth, seldom proliferous (, arrows). Thallus firm, cartilaginous, semi-transparent, pink to dull purplish red, brittle when young (), becoming matted and epiphytized with age (). Some specimens from heavily trawled places appearing slender and more branched (). Blades smooth, except in fertile female gametophytes, where protruding cystocarps were scattered on the thallus surface ().
Thallus appearing multiaxial and pseudoparenchymatous throughout (–), an apical cell, if present, not observed due to abundant secondary pit connections present between the most distal cortical cell files (); cells in the outer one or two layers strongly pigmented (). Outer cortical cells rounded to irregularly shaped in transverse section () and mostly polygonal in surface view (), up to 8 (–16) µm high and 15 (–24) µm in diameter, uninucleate when small, becoming multinucleate with one or more reticulate plastids (); nuclei in outer cortical cells 1.6–2.5 µm in diameter (). Deciduous hairs scattered over thallus surface, arising from a basal multinucleate hair primordium dividing into two cells, each up to 17 µm high and 18 µm in diameter (), with the upper cell clearly distinguishable in surface view (). Medullary cells rounded or ovoid, highly vacuolate, with a thick wall, up to 245 (–260) µm in dimension, with a dissected ribbon-like plastid containing pyrenoid-like structures (, arrows), becoming multinucleate () and connected with neighbouring cells by abundant pit connections (, arrows). Stipe with the same internal structure as the branches.
Life cycle
Triphasic, with isomorphic gametophytes and tetrasporophytes. Gametophytes dioecious.
Male reproductive structures
Spermatangial parent cells developing from an outer cortical cell forming a solitary pit (), or the pits fusing laterally in clusters over the thallus surface (, ). Each fertile cortical cell producing up to 16 spermatangial parent cells with conspicuous nuclei (); the wall of the original fertile cortical cell often distinguishable at the centre of the pit once the spermatangial parent cells have formed. Separations between conceptacles disintegrating at times to form an extended cavity (). Mature spermatangia each containing a single spermatium 6 µm in their longest dimension with a distal nucleus and proximal vacuole ().
Female reproductive structures
Cystocarps scattered over the thallus surface, usually near the apex, or situated along the thallus margins (). Cystocarps infrequent in our material with more than 5 cystocarps seen in only two specimens. Procarps consisting of a supporting cell bearing 2–3 side branches and a central two-celled carpogonial branch terminated by the carpogonium. A procarp is initiated from a surface cell that enlarges and undergoes a transverse division to produce a carpogonial branch initial and a supporting cell linked obliquely to 2–3 sterile side branches composed of uninucleate cells (). A pit connection links each sterile side branch to the supporting cell (, arrows). The carpogonial branch initial divides transversely to produce a rectilinear hypogynous cell and a terminal carpogonium () that is extended by a straight trichogyne directed toward the thallus surface (). The supporting cell is subspherical and multinucleate at maturity, and distinguishable from surrounding cortical cells by its larger size ().
After presumed fertilization, two haploid nuclei (presumably, the male and female nuclei) can be observed inside the carpogonium (), the carpogonium and the hypogynous cell inflate (), and a conspicuous, presumably diploid, nucleus is distinguishable inside the swollen carpogonium (). The sterile branches and surrounding cortical filaments grow forming longitudinal files of cells that initiate the pericarp. The lowermost cells of the sterile branches flanking the carpogonial branch fuse with the expanding carpogonium () forming a carpogonial fusion cell (). Carpogonial fusion cells are multinucleate, and their nuclei are a mixture of diploid derivatives of the fertilization nucleus and haploid nuclei from the secondarily incorporated vegetative cells (). At this stage, the cavity of the cystocarp forms by the breakdown of pit connections along a line separating the outer pericarp from the inner pericarp, with the latter staining more darkly than the former (). Young, developing cystocarps produce tubular cells from sterile gonimoblast cells at the base of newly differentiated carposporangial chains, which extend and penetrate deeply inside the lower (, arrow) or the upper (, arrow) part of the outer pericarp. The carpogonial fusion cell expands during gonimoblast development by continued incorporation of vegetative cells from the surrounding pericarp, often leaving projections behind (, arrows).
Developing gonimoblast cells cut off initials that produce small clusters of cells that initiate the carposporangial chains. Mature cystocarps reach up to 950 µm in diameter and consist of inner gonimoblast filaments and outer chains of carposporangia that mature basipetally and separate into free, independent carposporangia to the outside, enclosed within an osteolate pericarp (). Maturing inner gonimoblast filaments consist of elongate, non-vacuolate cells that retain significant amounts of cytoplasm bordered by an outer region of rows of free, fully differentiated carposporangia and an inner region in which the gonimoblast cells cross-link by secondary pit connections (, arrow).
Tubular nutritive cells originate inside the developing gonimoblasts and penetrate the outer pericarp. illustrates two such filaments (arrows) that have broken away from the outer pericarp but in which individual pericarp cells can be seen as protrusions still attached to a tubular cell. The outer pericarp is composed of files of rectilinear cells () in which secondary pit connections are quickly established between neighbouring files. Pericarp cells become stellate in response to the abundant formation of secondary pit connections (, arrows). At higher magnification it is seen that carposporangia are initiated acropetally in branched chains and are rounded or ovoid and uninucleate, with a nucleus up to 5 µm in diameter and measuring 39 µm in largest dimension ().
Tetrasporangia
Tetrasporangia are scattered over the thallus surface (), in slightly irregularly swollen regions (), and are cut off terminally and shifted to a lateral position as the cortex continues to grow (). The pit connection between the tetrasporangium and the subcortical cell is situated close to the base of the tetrasporangium (, arrow). Mature tetrasporangia are rounded, cruciately decussately divided, and up to 40 µm broad by 48 µm high ().
Gracilaria multipartita (Clemente) Harvey Citation1846: pl. XV
–.
Figs 40–46. Gracilaria multipartita. Habit and vegetative structure. Fig. 40. Habit of an adult specimen with many ligulate marginal proliferations (arrows) (HGI–A 10230). Fig. 41. Female gametophyte with cystocarps (HGI–A 16029). Fig. 42. Branch of female gametophyte with protruding cystocarps (HGI–A 16029). Fig. 43. Cross-section through median part of thallus (HGI–A 16029). Fig. 44. Cross-section showing progressive transition between medullary and cortical layers (HGI–A 16029). Fig. 45. Darkly staining multinucleate surface cells (HGI–A 16029). Fig. 46. Apex of thallus in surface view (HGI–A 2300). Scale bars: Figs 40, 41 = 5 cm; Fig. 42 = 1 cm; Fig. 43 = 200 µm; Figs 44–46 = 20 µm. Figs 43–45, haematoxylin. Abbreviations: cy = cystocarp; n = nucleus.
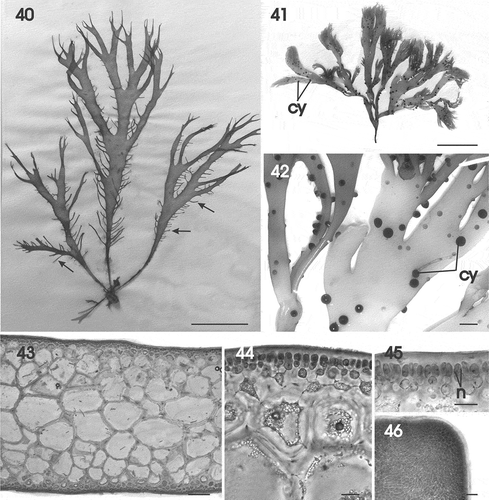
Figs 47–60. Gracilaria multipartita. Vegetative development and female prefertilization and early post-fertilization stages. Fig. 47. Surface view showing parietal plastids in multinucleate cells, and some cells with reticulate plastid (arrow) (HGI–A 2300). Fig. 48. Surface view of thallus with long deciduous hairs (HGI–A 16029). Fig. 49. Multinucleate hair primordium pit connected (arrow) to a deciduous hair (HGI–A 16029). Fig. 50. Deciduous hairs, each with a median nucleus (HGI–A 16029). Fig. 51. Surface view showing multinucleate hair primordia (arrows) (HGI–A 2300). Fig. 52. Medulla in transverse section showing secondary pit connections (arrows) (HGI–A 16029). Fig. 53. Transverse section of stipe (HGI–A 16029). Fig. 54. Supporting cell pit connected (arrow) to two side branches (HGI–A 16029). Fig. 55. Uninucleate supporting cell bearing a carpogonial branch initial and one side branch (HGI–A 16029). Fig. 56. Supporting cell initiating a two-celled carpogonial branch consisting of a terminal carpogonium and subterminal hypogynous cell surrounded by side branches (HGI–A 16029). Fig. 57. Binucleate carpogonium (HGI–A 16029). Fig. 58. Early post-fertilization stage showing broadening of the pit connection (arrow) between the hypogynous cell and the supporting cell (HGI–A 16029). Fig. 59. Post-fertilization stage showing an enlarged carpogonial fusion cell that has grown by incorporating cells from the associated outer pericarp (HGI–A 16029). Fig. 60. Initiation of cavity (arrow) between outer and inner pericarps (HGI–A 16029). Scale bars: Figs 47, 48, 50–52, 54–60 = 20 µm; Fig. 49 = 50 µm; Fig. 53 = 500 µm. Haematoxylin. Abbreviations: cbc = carpogonial branch cell; cp = carpogonium; cpi = carpogonial branch initial; gfi = gonimoblast filament initial; h = hair; hp = hair primordium; hy = hypogynous cell; ip = inner pericarp; n = nucleus; op = outern pericarp; pl = plastid; sb = side branch; sc = supporting cell.
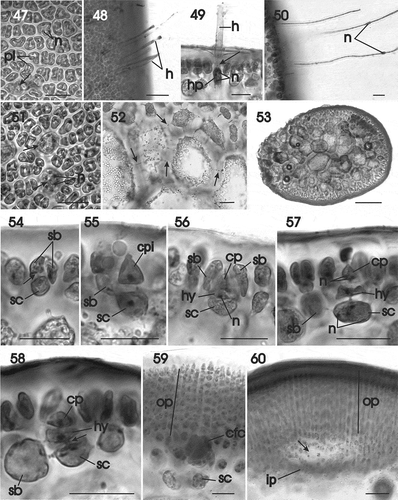
Figs 61–73. Gracilaria multipartita. Cystocarp development and tetrasporangia. Fig. 61. Carpogonial fusion cell that is continuing to enlarge by incorporation of cells of the outer pericarp (arrowheads) (HGI–A 16029). Fig. 62. Portion of a carpogonial fusion cell bearing clusters of young gonimoblast filaments and a tubular nutritive cell (arrow) (HGI–A 16029). Fig. 63. Lobed carpogonial fusion cell bearing clusters of young carposporangial chains and tubular nutritive cells (arrows) issuing from the carpogonial fusion cell and an inner gonimoblast cell (HGI–A 16029). Fig. 64. Transverse section of a young cystocarp composed of expanding inner gonimoblast cells, a tubular nutritive cell (arrow) and clusters of young carposporangia (HGI–A 16029). Fig. 65. Developing cystocarp in a median transverse section showing expanding inner gonimoblast cells, a tubular nutritive cell (arrow), a cluster of carposporangia, and an outer pericarp with an ostiole (HGI–A 16029). Fig. 66. Inner pericarp, expanding, vacuolate gonimoblast cells and branched chains of carposporangia (HGI–A 16029). Fig. 67. Enlarged view of vacuolate inner gonimoblast cells interconnected by numerous secondary pit connections (arrows) (HGI–A 16029). Fig. 68. Enlarged view of inner gonimoblast filaments bearing a row of tubular nutritive cells (arrows) penetrating the lower region of the outer pericarp. Fig. 69. Multinucleate tubular nutritive cell (arrow) penetrating between cells of the outer pericarp and fusing with individual pericarp cells (HGI–A 16029). Fig. 70. Non-median section of a mature cystocarp with vacuolate inner gonimoblast cells, a tubular nutritive cell (arrow), and mature clusters of carposporangia surrounded by inner and outer pericarps (HGI–A 16029). Fig. 71. Scattered tetrasporangia in surface view (arrows) (HGI–A 18008). Fig. 72. Cross-section of a fertile area showing the latero-basal connection between a tetrasporangial initial and a subcortical cell (arrow) (HGI–A 18066). Fig. 73. Mature cruciately divided tetrasporangium surrounded by cortical filaments (HGI–A 18066). Scale bars: Figs 61, 62, 66, 67, 68, 70, 72, 73 = 20 µm; Figs 63, 64, 70 = 50 µm; Figs 65, 69 = 100 µm; Fig. 71 = 200 µm. Figs 61–70, 72, 73 haematoxylin. Abbreviations: ca = carposporangium; cfc = carpogonial fusion cell; g = gonimoblast; ip = inner pericarp; n = nucleus; o = ostiole; op = outer pericarp; sb = side branch.
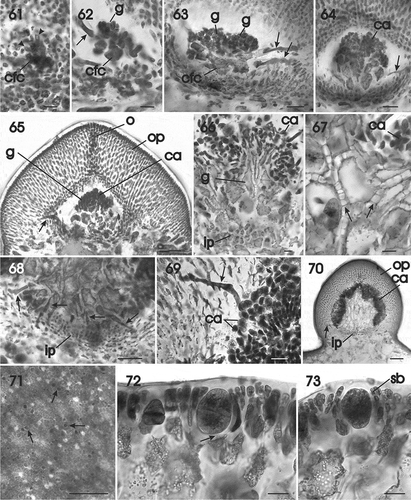
Basionym: Fucus multipartitus Clemente 1807.
Homotypic synonyms: Fucus multipartitus γ granateus sensu Turner 1819; Chondrus multipartitus (Clemente) Harvey Citation1834.
Heterotypic synonyms: Sphaerococcus polycarpus Greville Citation1828; Rhodymenia polycarpa (Greville) Greville Citation1830.
Type locality: Andalusia (Athanasiadis, Citation1996: 64). Lectotype: MA.
Distribution: Western Atlantic, from Ireland to Cape Verde, and the Mediterranean Sea (Guiry & Guiry Citation2016). The northern boundary is temperature limited with stable populations reported for the English Channel in Cornwall, Devon and Finistère, France extending sporadically into Dorset, England, Normandy, France and Galway, Ireland (Steentoft & Farnham, Citation1997).
Habitat: Specimens occurring isolated or in small groups both subtidally and intertidally on rocks and shells in sand (Steentoft & Farnham, Citation1997) and subtidally on maërl bottoms.
Specimens examined: Ghéreon, France: 6 July 2000, –10 m, C. Rodríguez-Prieto, HGI-A 2300, female gametophyte; 21 July 2009, C. Rodríguez-Prieto, HGI-A 10230; 12 July 2014, –9 m, C. Rodríguez-Prieto, HGI-A 16029 female gametophyte; 6 July 2015, –9 m, C. Rodríguez-Prieto, HGI-A 18008, tetrasporophyte, HGI-A 18066, tetrasporophyte.
Molecular vouchers: HGI-A 16029, rbcL – KU722967; psbA – KU745457; UPA - KU739402.
Habit and vegetative structure
Thallus erect, flattened, to 25 (–30) cm high, attached to the substratum by a discoid holdfast from which one to several axes arise (, ). Axes stipitate, with a stipe up to 35 mm long and 2 mm in diameter, expanding gradually to complanate, the blades subdichotomously to palmately branched and up to 10 mm wide with obtuse to acute-attenuate apices (, ). Margins smooth, seldom proliferous (, arrows). Texture firm to brittle, cartilaginous, semi-transparent, dull purplish red or reddish-brown or sometimes bleached. Blades smooth, except in fertile female gametophytes, where protruding mammillate cystocarps are scattered on the thallus surface (, ).
Thallus structure appearing multiaxial, pseudoparenchymatous throughout (–); an apical cell, if present, not distinguishable (). Outer one or two layers of cells conspicuously pigmented (), rounded to irregularly shaped in transverse section (), mostly polygonal in surface view (), and up to 12 (–14) µm high and 11 (–13) µm in diameter. Surface cells uninucleate when small, becoming multinucleate later on, with a few parietal plastids () and occasionally a small reticulate plastid (, arrow). Nuclei in outer cortical cells 1.6–2.3 µm in diameter (). Hairs scattered over the thallus surface near the apices (), usually deciduous below. Hairs pit-connected to a basal hair primordium, hyaline, with a thick wall () and a median nucleus (). Hair primordia up to 16 µm high and 18 µm in diameter, multinucleate, and clearly distinguishable in surface view (, arrows). Medullary cells rounded or ovoid up to 300 (–390) µm in widest dimension, multinucleate and vacuolate with a thick wall and a dissected ribbon-like plastid, connected to neighbouring cells by abundant pit connections (, arrows). Anatomy of the stipe similar to that of the blades ().
Life cycle
Probably triphasic, with isomorphic gametophytes and tetrasporophytes. Male plants not seen.
Female reproductive structures
Fertile areas and cystocarps scattered over the thallus surface and margins (). A fertile surface cell enlarges and undergoes two oblique divisions to produce a basal cell (= the supporting cell) and two sterile uninucleate one-celled side branch initials () that divide to produce unbranched side branches (). Pit connections link the sterile branches to the supporting cell (, arrow). The uninucleate supporting cell divides transversely cutting off a conical carpogonial branch initial (), that divides to produce a rectilinear hypogynous cell, and a carpogonium extended by a short straight trichogyne directed toward the thallus surface (). At maturity, the supporting cell is multinucleate and subspherical ().
After presumed fertilization, two haploid nuclei (presumably, the male and female nuclei) can be seen inside the carpogonium (). Subsequently, the carpogonium, the hypogynous cell and the supporting cell enlarge and the pit connection between the hypogynous cell and the supporting cell broadens preparatory to their ultimate fusion (, arrow). The two sterile branches and surrounding cortical filaments undergo a succession of transverse divisions to form files of cells that initiate the pericarp and the lowermost cells of the sterile branches flanking the carpogonium fuse onto the carpogonium introducing haploid nuclei into the expanding carpogonial fusion cell (). A cavity is initiated inside the cystocarp by the breakdown of pit connections between files of cortical cells surrounding the carpogonial fusion cell, separating the files into inner and outer pericarp (). The carpogonial fusion cell continues to enlarge, becoming lobed as pericarp cells are added along its margin (, arrowheads). Cytoplasmic projections from the carpogonial fusion cell cut off irregularly shaped uninucleate cells containing large, presumably diploid nuclei that function as gonimoblast initials. Each of the primary gonimoblast cells produces a small cluster of cells that initially form separate gonimolobes (), which later merge () and become confluent (). Some gonimoblast cells situated near the base of developing carposporangial chains elongate into tubular nutritive cells, which penetrate and fuse with cells in the lower part of the outer pericarp (–).
Young cystocarps are sessile, protruding and ostiolate (). Maturing inner gonimoblast cells elongate vertically and produce clusters of carposporangia in branched chains apically that mature basipetally (). The inner sterile gonimoblast cells connect laterally by means of secondary pit connections (). A longitudinal section of developing gonimoblasts reveals the presence of numerous tubular cells that link the inner gonimoblast cells with lower cells of the inner pericarp (). Tubular nutritive cells penetrate deeply inside the pericarp layers and fuse with pericarp cells along their lengths (). Carposporangia are rounded or ovoid and 48 µm in their largest dimension and uninucleate, with a nucleus up to 6 µm in diameter ().
Mature gonimoblasts reach up to 1050 µm in diameter (). The inner sterile gonimoblast cells expand and become vacuolate spreading the carposporangial chains into clusters that line the outer pericarp (, ). An outer pericarp is composed of files of rectilinear cells in which secondary pit connections are quickly established between neighbouring files, such that the inner cells become stellate ().
Tetrasporangia: Tetrasporangia are scattered over the thallus surface (, arrows) and are cut off terminally and shifted to a subterminal position as the surrounding cortical cells continue to grow (). The pit connection between the tetrasporangium and the subcortical cell is situated close to the base of the tetrasporangium (, arrow). Files of cortical cells elongate and divide adjacent to the tetrasporangium as it enlarges. Mature tetrasporangia are rounded, cruciately or decussately divided, and up to 48 µm broad by 70 µm high (, ).
DISCUSSION
Gurgel & Fredericq (Citation2004) identified nine evolutionary lineages within Gracilaria sensu lato from an analysis of rbcL sequence data. Only two of these were represented among the indigenous flora of Western Europe and the Mediterranean Sea: lineage V (= subgroup G. gracilis) and lineage IX (= subgroup G. bursa-pastoris). Subgroup Gracilaria gracilis includes G. gracilis (Stackhouse) M. Steentoft, L.M. Irvine & W.F. Farnham and G. dura (C. Agardh) J. Agardh sensu Destombe et al. (Citation2010), but not sensu Gargiulo et al. (Citation2006). Earlier, Gargiulio et al. (1992) had placed G. conferta (Schousboe ex Montagne) J. Feldmann & G. Feldmann, G. dendroides Gargiulo, De Masi & Tripodi, and G. longa Gargiulo in the verrucosa-group (= the G. gracilis subgroup) owing to the presence in these species of spermatangia borne in deep pits (verrucosa-type, sensu Yamamoto, 1978); however, in their later molecular study Gargiulio et al. (2006) observed that G. longa was closely aligned with G. bursa-pastoris, a species shown to have textorii-type (sensu Yamamoto, Citation1978) spermatangial conceptacles. These results cast doubt on the utility of spermatangial type as a character for determining the phylogenetic placement of Gracilaria species.
Gargiulo et al. (Citation1992) described G. corallicola as having ‘chorda-type (sensu Yamamoto (Citation1975, Citation1978) spermatangia, originating from outer cortical cells, and frequently forming continuous sori or sori separated by unmodified cortical cells’. Whereas spermatangial development was characteristic of Gracilariopsis, G. corallicola was said to have a fusion cell and tubular nutritive cells typical of Gracilaria. In contrast, we found that the spermatangia originated in shallow pits from a single central cell in the manner characteristic of textorii-type spermatangia. Gargiulio et al. (Citation1992) may have failed to recognize the textorii-type spermatangial character in their material owning to its obscurity in their ultra-thin sections.
The rbcL sequence divergence between G. corallicola and G. multipartita is greater than that between some closely related Gracilaria species (), but the two are resolved as sister species in the rbcL phylogeny, and form a strongly supported species cluster together with G. bursa-pastoris, G. armata and G. longa. A relationship between G. multipartita and G. bursa-pastoris was previously shown by Weinberger et al. (Citation2010).
Gracilaria corallicola and G. multipartita possess strongly flattened pseudodichotomously to palmately branched blades borne on one to several long cylindrical stipes that arise from a discoid holdfast. The most obvious difference between the two is that the apices are broadly rounded in G. corallicola and attenuate with pointed or blunt tips in G. multipartita. Their distributions do not appear to overlap, with G. corallicola apparently restricted to the Mediterranean Sea and with G. multipartita found predominantly in Atlantic Western Europe. The range of distribution of G. multipartita in the Mediterranean Sea has not been clarified.
Gracilaria corallicola and G. multipartita share a variety of characters in common with G. bursa-pastoris. Hair cells develop in a similar manner in all three species. An undifferentiated surface cell elongates, becomes multinucleate and cleaves into two cells as described in G. bursa-pastoris by Liao & Hommersand (Citation2003). The apical cell (hair primordium) cuts off a hair-cell initial that elongates into a long, uninucleate hair while the basal cell may become lobed and fuses with neighboring subcortical cells. Although this pattern of hair-cell development is not found in all Gracilaria species, it is not known how widespread these features are. Spermatangial development is textorii-type in all three species; however, conceptacle sizes vary with those of G. corallicola being shallow (10–12 µm deep) and often laterally confluent, and those of G. bursa-pastoris being deeper (20–25 µm deep) and tending to remain separate (Gargiulo et al., Citation1992). Spermatangia are situated in pits about 50 µm in diameter in G. multipartita (Dixon & Irvine, Citation1977), and are separate and about 20–25 μm deep and 35–40 μm broad as illustrated in Bird & McLachlan (Citation1984, , as G. foliifera), similar to the dimensions found in G. bursa-pastoris.
Cystocarp development follows a similar pattern in all three species (Gargiulo et al., Citation1992; this work). The cystocarp cavity is initiated in the plane of the carpogonial fusion cell where files of adjacent cortical filaments separate into inner and outer pericarp layers; the exterior gonimoblast cells form tubular cells at an early stage of gonimoblast development that penetrate and fuse with cells of the outer pericarp; the inner gonimoblast region below the files of differentiating carposporangia remain narrow, whether short or long in young, developing cystocarps and densely filled with cytoplasm.
Species differences are difficult to distinguish because different stages of gonimoblast development have been captured in different studies with the earliest stages mainly present in our material. Gracilaria bursa-pastoris from France possesses a thick inner pericarp; young gonimoblast cells remain short throughout their development, and tubular nutritive cells extend from outer gonimoblast cells and fuse with cells of the outer region of the outer pericarp even in young stages (Liao & Hommersand, Citation2003). The inner pericarp is not as thick in G. multipartita and G. corallicola as in G. bursa-pastoris, sterile gonimoblast cells elongate in the course of their development, and tubular cells arise from the lowermost gonimoblast cells and fuse primarily with cells in the lower region of the outer pericarp. Tubular nutritive cells were illustrated fusing with cells of the inner pericarp in G. armata, G. bursa-pastoris and G. corallicola by Gargiulio et al. (Citation1992). The early stages of cystocarp development are very similar in G. multipartita to those in G. corallicola. The inner pericarp is even thinner and the lowermost gonimoblast cells fuse extensively with one another to produce a ramified fusion cell and numerous tubular cells that penetrate and fuse with cells in the lower region of the outer pericarp. Cells of the inner pericarp elongate and are very narrow before interconnecting, whereupon they mature into a network of vacuolate cells at the final stages of their development. Elongated inner gonimoblast cells cut off secondary pit connections linking them to neighbouring cells. These cells later expand and become vacuolate in G. multipartita (; Bird & McLachlan, Citation1984: ). Our material of G. corallicola was so young that this stage was missing; however, it was clearly seen by Gargiulo et al. (Citation1992: ). Tubular nutritive cells continue to be formed and attach to cells in the outer pericarp in mature cystocarps in both G. multipartita (Bird & McLachlan, Citation1984: , ) and in G. corallicola (Gargiulo et al., Citation1992: ).
Tetrasporangia are initiated terminally from surface cells in the G. bursa-pastoris species cluster and may shift to a lateral position upon the production of side branches from the subterminal cells. They are cruciately or decussately divided in all members of the Gracilariaceae.
Development of the carpogonial branch and the earliest post-fertilization stages were first described in the Gracilariaceae by Sjöstedt (Citation1926) in Gracilariopsis andersonii (Grunow) E.Y. Dawson (as Gracilaria robusta Setchell) and confirmed by Fredericq & Hommersand (Citation1989) in the same species (as Gracilariopsis lemaneiformis (Bory) Dawson (see Gurgel et al., Citation2003). The carpogonial branch system is initiated by the concavo-convex division of a uninucleate surface cell followed by concavo-convex divisions of the subsurface cell to form 2–3 (4) sterile branch initials that will grow into files of lateral sterile branches. The subsurface cell then divides transversely into the supporting cell and a terminal cell that will function as the initial of the carpogonial branch. Transverse division of the carpogonial branch initial yields a two-celled carpogonial branch consisting of the terminal carpogonium bearing an elongating trichogyne and a subterminal hypogynous cell surrounded by the developing sterile branches. The trichogyne may or may not project beyond the thallus surface. Perhaps spermatia attach to the outer layer and form a tubular extension that penetrates the cuticle and attaches to the trichogyne depositing the sperm nucleus; however, this feature is not well documented. Files of cortical cells in the region of a fertilized carpogonium grow out in straight lines that branch infrequently, burying the carpogonium deep inside the developing pericarp. Cells of the sterile side branches fuse with the fertilized carpogonium and deposit their haploid nuclei while the diploid fertilization nucleus continues to divide. If the numbers of fusions are few, the resulting carpogonial fusion cell will remain small, as in Gracilariopsis andersonii. When fusions continue with the incorporation of numerous nuclei from pericarp cells the fusion cell enlarges, often becoming lobed as in Gracilaria multipartita. The size and shape of the carpogonial fusion cell is often characteristic of a given species.
With only minor variations the development of the carpogonial branch before fertilization and the formation of a carpogonial fusion cell containing intermixed haploid and diploid nuclei after fertilization are universal and a diagnostic features of the family Gracilariaceae. Even though the carpogonial fusion cell contains a mixture of haploid and diploid nuclei, the many gonimoblast initials it cuts off each contain a single diploid nucleus, such that separation of diploid from haploid nuclei is achieved at the stage of gonimoblast initiation for each gonimoblast filament produced. Inner gonimoblast cells that remain sterile may later become multinucleate while cells in the outer files that mature into carposporangia remain uninucleate. The development of the carpogonial branch before fertilization was illustrated for both Gracilaria corallicola and G. multipartita in this study. Fusion cell initiation was seen in both; however, the further elaboration of the fusion cell was illustrated only in G. multipartita in a process that led to the formation of a large, lobed carpogonial fusion cell. Numerous workers have commented that female reproductive characters cannot be relied on for the recognition of species or, more particularly, for the delineation of phylogenetic lines owing to convergent evolution. This may be true but the absence of critical stages in many collections and the employment of different methods of slide preparation are also certainly factors responsible for the problematic nature of cystocarp characters for systematic and phylogenetic comparisons of species groups within the Gracilariaceae. Although morphological studies of cystocarp development are laborious and difficult, we believe that their pursuit will, in time, contribute greatly to our understanding of the phylogenetic systematics of the Gracilariaceae at different taxonomic levels, especially when carried out in the light of appropriate molecular evidence.
ACKNOWLEDGEMENTS
We thank Sergi Joher, who collected some of the samples used in this study. We thank the crews of the research vessels Cornide de Saavedra, Miguel Oliver, and Ramón Margalef.
Disclosure statement
No potential conflict of interest was reported by the author(s).
Additional information
Funding
Notes on contributors
Conxi Rodríguez-Prieto
C. Rodríguez-Prieto: original concept, drafting and editing manuscript, optical microscope; D.W. Freshwater: generation and analysis of molecular data, editing and writing; M.H. Hommersand: drafting and editing manuscript.
REFERENCES
- Agardh, J.G. (1842). Algae maris Mediterranei et Adriatici, observationes in diagnosin specierum et dispositionem generum. pp. [i] –x, 1–164. Parisiis [Paris]: Apud Fortin, Masson et Cie.
- Agardh, J.G. (1852). Species genera et ordines algarum, seu descriptiones succinctae specierum, generum et ordinum, quibus algarum regnum constituitur. Volumen secundum: algas florideas complectens. Part 2, fasc. 2. pp. 577–700. C.W.K. Gleerup, Lundae [Lund].
- Ardissone, F. (1878). Le Floridee italiche descritte ed illustrate. 8, Rhodomelaceae. Tipografia editrice Lombarda, Milano.
- Ateweberhan, M. & Prud’homme van Reine, W.F. (2005). A taxonomic survey of seaweeds from Eritrea. Blumea, 50: 65–111.
- Athanasiadis, A. (1996). Taxonomisk litteratur och biogeografi av Skandinaviska rödalger och brunalger. Algologia, Göteborg.
- Bird, C.J. & McLachlan, J. (1984). Taxonomy of Gracilaria: evaluation of some aspects of reproductive structure. Hydrobiologia, 116/117: 41–46.
- Børgesen, F. (1932). A revision of Forsskål’s algae mentioned in Flora Aegyptiaco-Arabica and found in his herbarium in the Botanical Museum of the University of Copenhagen. Dansk botaniske Arkiv., 8: 1–14.
- Børgesen, F. (1938). Contributions to a South Indian marine flora. Journal of the Indian Botanical Society, 17: 205–242.
- Cianciola, E.N., Popolizio, T.R., Schneider, C.W. & Lane, C.E. (2010). Using molecular-assisted alpha taxonomy to better understand the red algal biodiversity in Bermuda. Diversity, 2: 946–958.
- Clemente y Rubio, S. de Rojas (1807). Ensayo sobre las variedades de la vid comun que vegetan en Andalucía, con un índice etimológico y tres listas de plantas en que se caracterizan varias especies nuevas. Imprenta de Villalpando, Madrid.
- Dawson, E.Y. (1941). A review of the genus Rhodymenia with descriptions of new species. Allan Hancock Pacific Expeditions, 3: 123–181, pls 18–30.
- Destombe, C., Valero, M. & Guillemin, M.L. (2010). Delineation of two sibling red algal species, Gracilaria gracilis and Gracilaria dura (Gracilariales, Rhodophyta), using multiple DNA markers: resurrection of the species G. dura previously described in the northern Atlantic 200 years ago. Journal of Phycology, 46: 720–727.
- Dixon, P.S. & Irvine, L.M. (1977). Seaweeds of the British Isles. Volume 1. Rhodophyta. Part 1. Introduction, Nemaliales, Gigartinales. [i]v–xi, [1]4–252, 90 figs. British Museum (Natural History), London.
- Forsskål, P. (1775). Flora Aegyptiaca-Arabica. Sive descriptiones plantarum, quas per Aegyptum inferiorem et Arabium delicem detexit illustravit Petrus Forskål. Prof. Haun. Post mortem auctoris edidit Carsten Niebuhr. Ex officina Mölleri, Hauniæ [ Copenhagen].
- Fredericq, S. & Hommersand, M.H. (1989). Proposal of the Gracilariales ord. nov. (Rhodophyta) based on an analysis of the reproductive development of Gracilaria verrucosa. Journal of Phycology, 25: 213–227.
- Freshwater, D.W. & Rueness, J. (1994). Phylogenetic relationships of some European Gelidium (Gelidiales, Rhodophyta) species, based on rbcL nucleotide sequence analysis. Phycologia, 33: 187–194.
- Gargiulo, G.M., de Masi, F. & Tripodi, G. (1992). Morphology, reproduction and taxonomy of the Mediterranean species of Gracilaria (Gracilariales, Rhodophyta). Phycologia, 31: 53–80.
- Gargiulo, G.M., Morabito, M., Genovese, G. & De Masi, F. (2006). Molecular systematics and phylogenetics of Gracilariacean species from the Mediterranean Sea. Journal of Applied Phycology, 18: 497–504.
- Greville, R.K (1822). Scottish cryptogamic flora, or coloured figures and descriptions of cryptogamic plants, belonging chiefly to the order Fungi; and intended to serve as a continuation of English Botany. Vol. 1 (fasc. 1–6). MacLachlan & Stewart; Baldwin, Craddock & Joy, Edinburgh & London.
- Greville, R.K. (1828). Scottish cryptogamic flora, or coloured figures and descriptions of cryptogamic plants, belonging chiefly to the order Fungi; and intended to serve as a continuation of English Botany. Vol. 6 (fasc. 67–72). MacLachlan & Stewart; Baldwin, Craddock & Joy, Edinburgh & London.
- Greville, R.K. (1830). Algae britannicae, or descriptions of the marine and other inarticulated plants of the British islands, belonging to the order Algae; with plates illustrative of the genera. McLachlan & Stewart; Baldwin & Cradock, Edinburgh & London.
- Guiry, M.D. & Freamhainn, M.T. (1986). Biosystematics of Gracilaria foliifera (Gigartinales, Rhodophyta). Nordic Journal of Botany, 5: 629–637.
- Guiry, M.D. & Guiry, G.M. (2016). AlgaeBase. World-wide electronic publication, National University of Ireland, Galway. http://www.algaebase.org. Accessed on 30 June 2015.
- Gurgel, C.F.D. & Fredericq, S. (2004). Systematics of the Gracilariaceae (Gracilariales, Rhodophyta): a critical assessment based on rbcL sequence analyses. Journal of Phycology, 40: 138–159.
- Gurgel, C.F.D., Fredericq, S. & Norris, J.N. (2003). Gracilariopsis silvana sp. nov., G. hommersandii sp. nov., and G. cata-luziana sp. nov., three new species of Gracilariaceae (Gracilariales, Rhodophyta) from the western Atlantic. Hydrobiologia, 13: 57–68.
- Gurgel, F.D.G., Fredericq, S., Norris, J.N. & Yoneshigue-Valentin, Y. (2008). Two new flat species of Gracilaria (Gracilariales, Rhodophyta) from Brazil: G. abyssalis sp. nov. and G. brasiliensis sp. nov. Phycologia, 47: 249–264.
- Harvey, W.H. (1834). Algological illustrations. No. I. Remarks on some British algae, and descriptions of new species recently added to our flora. Journal of Botany [Hooker], 1: 296–305.
- Harvey, W.H. (1846). Phycologia britannica, or, a history of British sea-weeds: containing coloured figures, generic and specific characters, synonymes, and descriptions of all the species of algae inhabiting the shores of the British Islands. Reeve & Benham, London.
- Hauck, F. (1885). Die Meeresalgen Deutschlands und Österreichs. In Kryptogamen-Flora von Deutschland, Österreich und der Schweiz. Zweite Auflage (Rabenhorst, L., editor), Vol. 2, 513–575. Eduard Kummer, Leipzig.
- Hommersand, M.H. & Freshwater, D.W. (2009). Gracilaria hummii sp. nov. (Gracilariales, Rhodophyta), a new name for the agarophyte “Gracilaria confervoides” harvested in North Carolina during World War II. Journal of Phycology, 45: 503–516.
- Huelsenbeck, J.P. & Ronquist, F. (2001). MrBayes: Bayesian inference of phylogenetic trees. Bioinformatics, 17: 754–755.
- Kim, M.-S., Yang, E.-C. & Boo, S.-M. (2006). Taxonomy and phylogeny of flattened species of Gracilaria (Gracilariaceae, Rhodophyta) from Korea based on morphology and protein-coding plastid rbcL and psbA sequences. Phycologia, 45: 520–528.
- Lamouroux, J.V.F. (1805). Dissertations sur plusieurs espèces de Fucus, peu connues ou nouvelles; avec leur description en latin et en français. Imprimerie de Raymond Nouvel & Chez Treuttel et Würtz, Agen & Paris.
- Liao, L.M. & Hommersand, M.H. (2003). A morphological study and taxonomic reassessment of the generitype species in the Gracilariaceae. Journal of Phycology, 39: 1207–1232.
- Lin, S.-M., Liu, L.-C & Payri, C. (2012). Characterization of Gracilaria vieillardii (Gracilariaceae, Rhodophyta) and molecular phylogeny of foliose species from the western Pacific Ocean, including a description of G. taiwanensis sp. nov. Phycologia, 51: 421–431.
- Mamoozadeh, N.R. & Freshwater, D.W. (2011). Taxonomic note on Caribbean Neosiphonia and Polysiphonia (Ceramiales, Florideophyceae): five species from Florida, USA and Mexico. Botanica Marina, 54: 269–292.
- McLachlan, J. (1979). Gracilaria tikvahiae sp. nov. (Rhodophyta, Gigartinales, Gracilariaceae), from the northwestern Atlantic. Phycologia, 18: 19–23.
- McLachlan, J. & Edelstein, T. (1977). Life-history and culture of Gracilaria foliifera (Rhodophyta) from south Devon. Journal of the Marine Biological Association of the United Kingdom, 57: 577–586.
- Newton, L. (1931). A Handbook of the British Seaweeds. The Trustees of the British Museum, British Museum (Natural History), London.
- Rodríguez-Prieto, C. & Hommersand, M.H. (2009). Behaviour of the nuclei in pre- and post-fertilization stages in Kallymenia (Kallymeniaceae, Rhodophyta). Phycologia, 48: 138–155.
- Sfriso, A., Wolf, M.A., Sciuto, K., Morabito, M., Andreoli, C. & Moro, I. (2013). Gracilaria viridis sp. nov. (Gracilariales, Rhodophyta): a new red algal species from the Mediterranean Sea. Phycologia 52: 65–73.
- Sherwood, A.R. & Presting, G.G. (2007). Universal primers amplify a 23S rDNA plastid marker in eukaryotic algae and cyanobacteria. Journal of Phycology, 43: 605–608.
- Sjöstedt, L.G. (1926). Floridean studies. Acta Universitatis Lundensis, 22: 1–95.
- Stamatakis, A. (2006). RAxML-VI-HPC: maximum likelihood-based phylogenetic analyses with thousands of taxa and mixed models. Bioinformatics, 22: 2688–2690.
- Stafleu, F.A. & Voss, E.G. eds. 1978. International code of botanical nomenclature adopted Leningrad 1975. Regnum vegetabile 97. Bohn, Scheltema and Holkema, Utrecht.
- Steentoft, M. & Farnham, W.F. (1997). Northern distribution boundaries and thermal requirements of Gracilaria and Gracilariopsis (Gracilariales, Rhodophyta) in Atlantic Europe and Scandinavia. Nordic Journal of Botany, 17: 87–93.
- Thiers, B. (2016). (continuously updated) Index Herbariorum: A global directory of public herbaria and associated staff. New York Botanical Garden’s Virtual Herbarium. http://sweetgum.nybg.org/ih/.
- Tseng, C.K. & Xia, B.M. (1999). On the Gracilaria in the western Pacific and southeastern Asia region. Botanica Marina, 42: 209–218.
- Turner, D. (1807–1808 ‘1808’). Fuci sive plantarum fucorum generi a botanicis ascriptarum icones descriptiones et historia. Fuci, or coloured figures and descriptions of the plants referred by botanists to the genus Fucus. Vol. I pp. [i, iii], [1]-164, [1]-2, pls 1–71 (col. copp. W.J. Hooker). Typis J. M’Creery, impensis J. et A. Arch., Londini [London].
- Turner, D. (1811–1819 ‘1819’). Fuci sive plantarum fucorum generi a botanicis ascriptarum icones descriptiones et historia. Fuci, or coloured figures and descriptions of the plants referrred by botanists to the genus Fucus. Vol. 3 (1811) pls. 135 – 196, Vol. 4 (1819) pls. 197 – 257. Typis J. M’Creery, impensis J. et A. Arch., Londini [London].
- Weinberger, F., Guillemin, M.-L., Destombe, C., Valero, M., Faugeron, S., Correa, J.A., Pohnert, G., Pehlke, C., Kloareg, B. & Potin, P. (2010). Defense evolution in the Gracilariaceae (Rhodophyta): substrate-regulated oxidation of agar oligosaccharides is more ancient than the oligoagar-activated oxidative burst. Journal of Phycology, 46: 958–968.
- Wittmann, W. (1965). Aceto-iron-haematoxylin-chloral hydrate for chromosome staining. Stain Technology, 40: 161–164.
- Yamamoto, H. (1975). The relationship between Gracilariopsis and Gracilaria from Japan. Bulletin of the Faculty of Fisheries Hokkaido University, 26: 217–222.
- Yamomoto, H. (1978). Systematic and anatomical study of the genus Gracilaria in Japan. Memoirs of the Faculty of Fisheries Hokkaido University, 25: 97–152.
- Yoon, H.S., Hackett, J.D. & Bhattacharya, D. (2002). A single origin of the peridinin- and fucoxanthin-containing plastids in dinoflagellates through tertiary endosymbiosis. Proceedings of the National Academy of Sciences USA, 99: 11724–11729.
- Zanardini, G. (1865). Scelta di Ficee nuove o più rare dei mari Mediterraneo ed Adriatico, Decade VI. Memorie del Reale Istituto Veneto di Scienze, Lettere ed Arti, 12: [375]–410.