ABSTRACT
Polysiphonia is the largest genus of red algae, and several schemes subdividing it into smaller taxa have been proposed since its original description. Most of these proposals were not generally accepted, and currently the tribe Polysiphonieae consists of the large genus Polysiphonia (190 species), the segregate genus Neosiphonia (43 species) and 13 smaller genera (< 10 species each). In this paper, phylogenetic relationships of the tribe Polysiphonieae are analysed, with particular emphasis on the genera Carradoriella, Fernandosiphonia, Melanothamnus, Neosiphonia, Polysiphonia sensu stricto, Streblocladia and Vertebrata. We evaluated the consistency of 14 selected morphological characters in the identified clades. Based on molecular phylogenetic (rbcL and 18S genes) and morphological evidence, two speciose genera are recognized: Vertebrata (including the type species of the genera Ctenosiphonia, Enelittosiphonia, Boergeseniella and Brongniartella) and Melanothamnus (including the type species of the genera Fernandosiphonia and Neosiphonia). Both genera are distinguished from other members of the Polysiphonieae by synapomorphic characters, the emergence of which could have provided evolutionarily selective advantages for these two lineages. In Vertebrata trichoblast cells are multinucleate, possibly associated with the development of extraordinarily long photoprotective trichoblasts. Melanothamnus has 3-celled carpogonial branches and plastids lying exclusively on radial walls of the pericentral cells, which similarly may improve resistance to damage caused by excessive light. Other relevant characters that are constant in each genus are also shared with other clades. The evolutionary origin of the genera Melanothamnus and Vertebrata is estimated as 75.7–95.78 and 90.7–138.66 Ma, respectively. Despite arising in the Cretaceous, before the closure of the Tethys Seaway, Melanothamnus is a predominantly Indo-Pacific genus and its near-absence from the north-eastern Atlantic is enigmatic. The nomenclatural implications of this work are that 46 species are here transferred to Melanothamnus, six species are transferred to Vertebrata, and 13 names are resurrected for Vertebrata.
Introduction
The Rhodomelaceae Areschoug is the largest family of red algae, currently including more than 1000 species (Guiry & Guiry, Citation2016). It consists of the tribes Amansieae Schmitz (1889), Bostrychieae Falkenberg (Citation1901), Chondrieae Schmitz & Falkenberg (Citation1897), Herposiphonieae Schmitz & Falkenberg (Citation1897), Heterocladieae Falkenberg (Citation1901), Laurencieae Schmitz (1889), Lophothalieae Schmitz & Falkenberg (Citation1897), Neotenophyceae Kraft & I.A.Abbott (Citation2002), Pleurostichidieae (Hommersand, Citation1963), Polysiphonieae Schmitz (1889), Polyzonieae Schmitz & Falkenberg (Citation1897), Pterosiphonieae Falkenberg (Citation1901), Rhodomeleae Schmitz & Falkenberg (Citation1897), Sonderelleae L.E.Phillips (Citation2001) and Streblocladieae nom. nud. (Hommersand, Citation1963; Kraft & Abbott, Citation2002; Womersley, Citation2003), of which the most speciose is the Polysiphonieae with over 300 species in 15 currently recognized genera (Guiry & Guiry, Citation2016).
Within the Polysiphonieae the genus Polysiphonia Greville (Citation1824), nom. cons., has representatives throughout the world, in the majority of photic marine benthic habitats including brackish ones (e.g. Hollenberg, Citation1942, Citation1944, Citation1968a, Citation1968b; Womersley, Citation1979; Maggs & Hommersand, Citation1993; Lam et al., Citation2013). Polysiphonia is poorly circumscribed, and has remained in a state of taxonomic flux since its original description. Numerous schemes for subdividing this large and morphologically diverse genus into smaller and more manageable groups have been proposed (e.g. Segi, Citation1951; Hollenberg, Citation1968a, Citation1968b), based mostly on the number of periaxial cells, either four (subgenus Oligosiphonia) or more than four (subgenus Polysiphonia). These schemes have generally been rejected and several generic names (e.g. Orcasia Kylin (Citation1941), based on Polysiphonia senticulosa Harvey) are currently regarded as synonyms of Polysiphonia. However, despite having been subsumed within Polysiphonia in most classification schemes, Vertebrata S.F.Gray (Citation1821) is currently recognized as a monospecific genus containing only the type species, V. lanosa.
The segregate genus Neosiphonia M.-S.Kim & I.K.Lee (Kim & Lee, Citation1999) has been widely accepted and is now the second largest in the Polysiphonieae (Guiry & Guiry, Citation2016). Neosiphonia (type species: N. flavimarina from Korea) is characterized by the following features: (1) thalli erect with a main axis bearing branches; (2) branches or trichoblasts formed on every segment; (3) rhizoids cut off from pericentral cells; (4) carpogonial branches 3-celled; (5) spermatangial branches formed on a branch of modified trichoblasts; (6) tetrasporangia in spiral arrangement (Kim & Lee, Citation1999). These features contrast markedly with the key characters of Polysiphonia sensu stricto, exemplified by the type species P. stricta: prostrate axes with rhizoids in open connection with pericentral cells; carpogonial branches 4-celled; spermatangial branches borne directly on axes; tetrasporangia in straight rows (Kim et al., Citation2000). In addition to describing the new species N. flavimarina, Kim & Lee (Citation1999) also transferred 11 species of Polysiphonia to Neosiphonia, all based on material from Korea, and there are 43 currently recognized species (Guiry & Guiry, Citation2016), not all of which exhibit the six key characters of Neosiphonia listed above.
Kim & Lee (Citation1999) considered Neosiphonia (also referred to as the ‘Polysiphonia japonica complex’ sensu Yoon (Citation1986)) to be related to Fernandosiphonia Levring, which was erected for F. unilateralis from the Juan Fernández Islands off Chile on the basis of its unilateral development of ultimate branches (Levring, Citation1941) and which currently consists of three species. They reported that Neosiphonia differed from Fernandosiphonia principally in its branching pattern, the origin of spermatangial branches, and the 3-celled carpogonial branches. Kim & Lee (Citation1999) did not comment, however, on the possible relationship between Fernandosiphonia and Streblocladia F.Schmitz (in Schmitz & Falkenberg, Citation1897). Hommersand (Citation1963) and Norris (Citation1994) compared Fernandosiphonia (trichoblasts formed spirally) with Streblocladia (trichoblasts borne only adaxially). Choi et al. (Citation2001) drew attention to the relationship in their 18S tree between N. japonica and Polysiphonia virgata, the type species of Carradoriella P.C.Silva (Kylin, Citation1956, as Carradoria; Silva et al., Citation1996), and suggested that Neosiphonia might either be subsumed into Carradoriella or be resolved as a sister to it. Recent searches of DNA sequence databases unexpectedly showed a possible relationship between Neosiphonia species and Melanothamnus somalensis, the type species of the genus Melanothamnus Bornet & Falkenberg, which was regarded as incertae sedis (Falkenberg, Citation1901).
Given the taxonomic and nomenclatural complexity within the Polysiphonieae, our aims were to re-evaluate the morphological features of Neosiphonia and Vertebrata in relation to those of Fernandosiphonia, Streblocladia, Carradoriella, Melanothamnus and Polysiphonia sensu stricto within a phylogenetic analysis of the Polysiphonieae using sequences of the plastid-encoded rbcL gene and the ribosomal DNA 18S gene (SSU). We surveyed within the Polysiphonieae the distribution of a striking characteristic of the ‘Polysiphonia japonica complex’, the position of plastids on radial walls of the periaxial cells and their absence from the outer walls such that nuclei are clearly visible after staining (Maggs & Hommersand, Citation1993; McIvor et al., Citation2001). Likewise, we analysed the multinucleate vs. uninucleate character of trichoblast cells, which seems to be taxonomically significant (Maggs & Hommersand, Citation1993).
Materials and methods
Field collections, morphological studies and literature review
Samples of Polysiphonieae (Table S1) were collected from European Atlantic coasts, New Zealand, Australia, Taiwan, Japan, Chile, USA, South Africa and Oman and processed fresh, desiccated in silica gel or preserved in ethanol.
Type material of Fernandosiphonia unilateralis was obtained from the Herbarium, Botanical Museum, Göteborg, Sweden (GB) by correspondence with the curator. It consisted of four permanent slides, a herbarium sheet and liquid-preserved material. Furthermore, we studied recent collections from Juan Fernández Islands, the type locality. We also studied the type material in US and TCD of several species currently assigned to Neosiphonia (Table S2; herbarium abbreviations as in Thiers, Citation2016) for which the key morphological characteristics () could not be clearly ascertained from published literature, in order to determine their correct generic assignment. For this purpose, we exclusively considered the descriptions provided for material from type localities or near them. To ensure the accuracy of our interpretation of the genera, our concept of them is based on material of their type species obtained from their type localities. For Streblocladia, we used material of, and sequences from, the type species S. glomerulata from New Zealand. Carradoriella (i.e. Polysiphonia virgata) was obtained from the type locality in South Africa, and the type species of Vertebrata and Melanothamnus came from Ireland and Oman respectively.
Table 1. Comparison of selected morphological characteristics among the Polysiphonia sensu stricto 1 and 2, Vertebrata, Carradoriella, Streblocladia, ‘Polysiphonia’ schneideri and Melanothamnus clades.
Fresh material and herbarium samples were prepared as squashes, either unstained or stained with aqueous aniline blue, post-fixed in 1% HCl, and mounted in 80% Karo corn syrup (Bestfoods Inc., NJ, USA). Permanent slide mounts were prepared as vouchers and deposited in: BM, MICH, SANT, WNC and MEL.
A systematic review was carried out to identify relevant phycological literature from around the world from which to assess for each species of Polysiphonieae the 14 vegetative and reproductive features relevant to Neosiphonia and Vertebrata.
Nomenclatural authorities for the species mentioned in the manuscript are provided in – and S1–S2.
Table 2. Genus Vertebrata with new combinations resulting from the present study printed in bold, followed by basionyms and taxonomic synonyms.
Table 3. Genus Vertebrata with resurrected names resulting from the present study and V. lanosa printed in bold, followed by basionyms and taxonomic synonyms.
Table 4. Genus Melanothamnus with new combinations resulting from the present study printed in bold, followed by basionyms and taxonomic synonyms.
Table 5. New combinations in Melanothamnus made for formal reasons (because the current genus is here placed in synonymy with Melanothamnus) although type material has not been examined. They are printed in bold, followed by basionyms and taxonomic synonyms.
Fig. 1. Phylogenetic tree estimated with ML analysis of rbcL sequences. Values at nodes indicate bootstrap support (BP)/posterior probability (PP) (only shown if > 60/0.6). Branches marked with an asterisk received 100% (BP)/1.00 (PP) support. Species names printed in bold correspond to type species of genera.
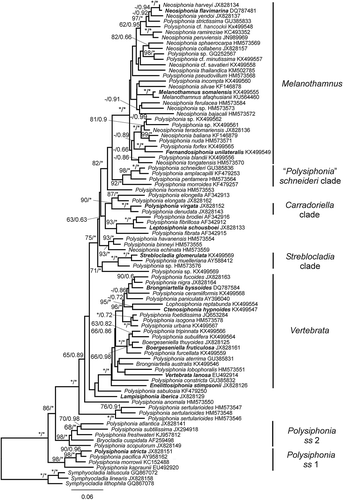
Fig. 2. Phylogenetic tree estimated with ML analysis of 18S sequences. Values at nodes indicate bootstrap support/posterior probability (only shown if > 60%/0.6 PP). Branches marked with an asterisk received 100%/1.00 PP support. Species names printed in bold correspond to type species of genera.
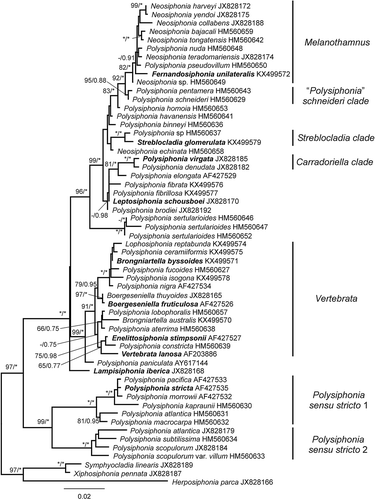
Figs 3–8. Melanothamnus somalensis, the type species of Melanothamnus. Fig. 3. Herbarium specimen MICH 662774. Fig. 4. Apical part of a specimen with alternately arranged branches. Figs 5–6. Apices of branches with (Fig. 5) or without (Fig. 6) abundant trichoblasts. Fig. 7. Apex of a lateral branch with trichoblasts. Fig. 8. Surface view of cells with the plastids lying exclusively on radial walls while the outer walls appear transparent (arrows). Scale bars: Fig. 3, 6 cm; Fig. 4, mm; Figs 5 and 6, 350 µm; Fig. 7, 200 µm; Fig. 8, 100 µm.
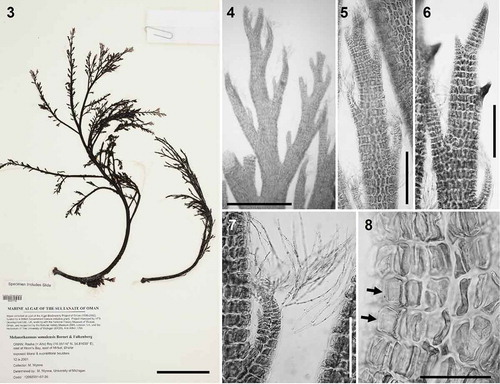
Figs 9–18. Fernandosiphonia unilateralis type material, the type species of Fernandosiphonia. Fig. 9. Herbarium specimen. Figs 10–11. Branches unilaterally arranged. Fig. 12. Axis with scar cells of trichoblasts (arrows). Figs 13–14. Surface view of pericentral cells with plastids lying only on the radial walls, so that the outer walls appear transparent (Fig. 13, arrows) and cells have a dark flank (Fig. 14). Fig. 15. Young spermatangial branch formed on the first dichotomy of a trichoblast, the other branch remaining vegetative (arrow). Fig. 16. Procarp (su = supporting cell; cp = carpogonium). Fig. 17. Cystocarp. Fig. 18. Tetrasporangia arranged in short spiral series. Scale bars: Fig. 9, cm; Fig. 10, mm; Fig. 11, 450 µm; Figs 12, 14, 17 and 18, 100 µm; Figs 13 and 15, 40 µm; Fig. 16, 20 µm.
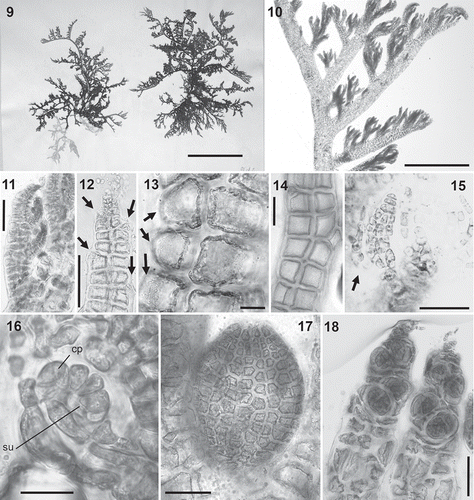
Figs 19–24. Rhizoid anatomy in the Polysiphonieae. In open connection with pericentral cells in Polysiphonia stricta (Fig. 19, Polysiphonia sensu stricto clade 1). Cut off from pericentral cells in P. foetidissima (Fig. 20, Vertebrata clade), P. denudata (Fig. 21, Carradoriella clade), Polysiphonia sp. (Fig. 22, Streblocladia clade), P. schneideri (Fig. 23, ‘P.’ schneideri clade) and P. incompta (Fig. 24, Melanothamnus clade). Scale bars: Figs 19–23, 100 µm; Fig. 24, 500 µm.
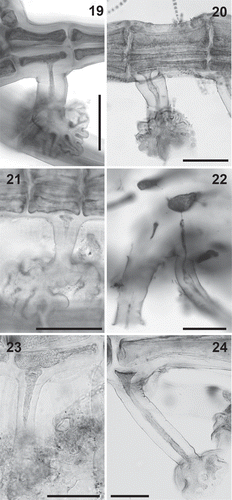
Figs 25–39. Plastid arrangement in the Polysiphonieae. Scattered against all cell walls of the pericentral cells in Polysiphonia stricta (Figs 25–26, Polysiphonia sensu stricto clade 1), Vertebrata lanosa (Figs 27–28, Vertebrata clade), P. virgata (Figs 29–30, Carradoriella clade), Polysiphonia sp. (Fig. 31, Streblocladia clade) and P. schneideri (Figs 32–33, ‘P.’ schneideri clade). Lying exclusively on the radial walls of the pericentral cells in species of the Melanothamnus clade: Neosiphonia collabens (Figs 34–35), N. harveyi (Figs 36–38) and P. forfex (Fig. 39). Scale bars: Figs 25, 27, 29, 38 and 39, 500 µm; Figs 26, 28 and 30, 800 µm; Figs 31, 32, 34, 35 and 37, 100 µm; Fig. 33, 300 µm; Fig. 36, 50 µm.
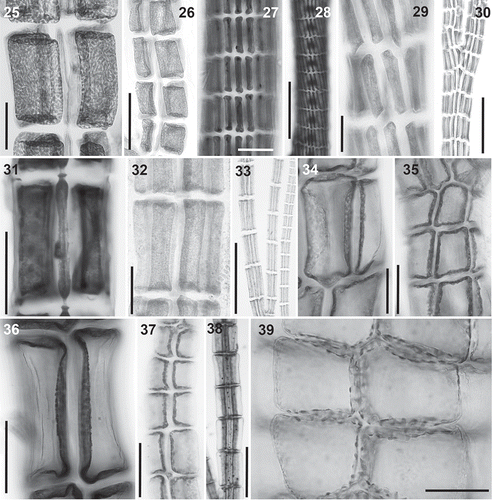
Figs 40–46. Trichoblast nuclei (arrows) in the Polysiphonieae. Uninucleate trichoblast cells in Polysiphonia scopulorum (Fig. 40, Polysiphonia sensu stricto clade 1), P. denudata (Fig. 44, Carradoriella clade), P. schneideri (Fig. 45, ‘P.’ schneideri clade) and P. blandii (Fig. 46, Melanothamnus clade). Multinucleate trichoblast cells in species of the Vertebrata clade: P. nigra (Fig. 41), Boergeseniella fruticulosa (Fig. 42) and P. foetidissima (Fig. 43). Scale bars: Figs 40–43, 60 µm, Fig. 44, 30 µm; Fig. 45, 20 µm; Fig. 46, 100 µm.
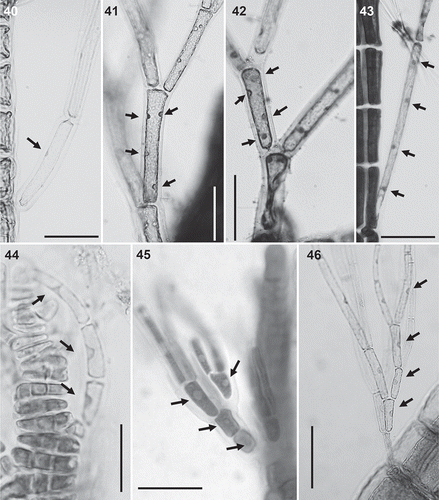
Figs 47–52. Spermatangial branches in the Polysiphonieae. Replacing trichoblasts and with sterile apical filaments in Polysiphonia stricta (Fig. 47, Polysiphonia sensu stricto clade 1). Replacing trichoblasts and lacking sterile apical cells in Vertebrata lanosa (Fig. 48, Vertebrata clade). On a branch of a trichoblast and with sterile apical cells in P. fucoides (Fig. 49, Vertebrata clade), P. denudata (Fig. 50, Carradoriella clade), P. schneideri (Fig. 51, ‘P.’ schneideri clade) and Neosiphonia harveyi (Fig. 52, Melanothamnus clade). Scale bars: 100 µm. Arrows show the apical sterile cells and arrowheads the sterile branch of fertile trichoblasts.
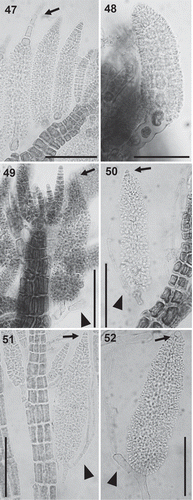
Figs 53–58. Carpogonial branches in the Polysiphonieae. Four-celled in Polysiphonia stricta (Fig. 53, Polysiphonia sensu stricto clade 1), P. nigra (Fig. 54, Vertebrata clade), P. denudata (Fig. 55, Carradoriella clade) and P. schneideri (Fig. 56, ‘P.’ schneideri clade). Three-celled in species of the Melanothamnus clade: Neosiphonia harveyi (Fig. 57) and P. blandii (Fig. 58). Su = supporting cell; st = sterile basal cell; 1–4 cells of carpogonial branches. Scale bars: Fig. 53, 30 µm; Figs 54–58, 20 µm.
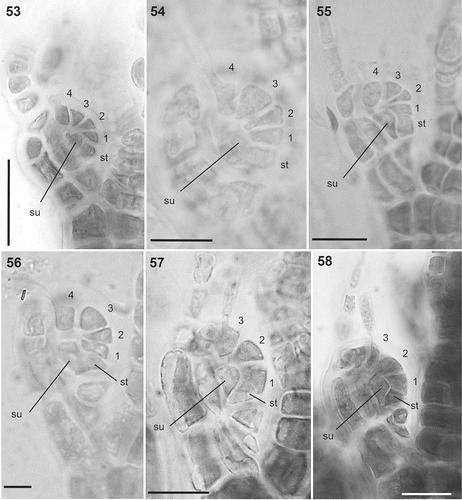
Figs 59–64. Cystocarps in the Polysiphonieae. Urceolate in Polysiphonia stricta (Fig. 59, Polysiphonia sensu stricto clade 1). Ovoid in Vertebrata lanosa (Fig. 60, Vertebrata clade), P. denudata (Fig. 61, Carradoriella clade), Streblocladia glomerulata (Fig. 62, Streblocladia clade). Globose in Polysiphonia schneideri (Fig. 63, ‘P.’ schneideri clade) and Neosiphonia collabens (Fig. 64, Melanothamnus clade). Scale bars: Figs 59–62 and 64, 200 µm; Fig. 63, 100 µm.
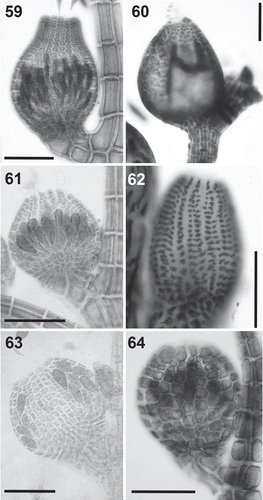
DNA extraction, PCR amplification and sequencing
This was carried out in four different laboratories using different protocols as described below.
At Queen’s University Belfast, DNA was extracted from fresh, silica gel-dried or ethanol-preserved material using the Qiagen DNeasy Plant Mini Kit (Qiagen GmbH, Hilden, Germany), according to the manufacturer’s instructions, or by a CTAB method, modified after Doyle & Doyle (Citation1987). For PCR amplification, a PTC-200 DNA Engine (MJ Research Inc.) was used. Except for material of Fernandosiphonia unilateralis, all PCR amplifications were carried out using rbcLFC as the forward primer, and rbcLRD as the reverse primer (Nam et al., Citation2000; McIvor et al., Citation2001). All reactions contained 200 µM each of dATP, dCTP, dGTP and dTTP, 0.3 µM of each primer, 2.5 mM MgCl2, and 1.6 units of BiotaqTM DNA polymerase (Bioline, UK). The PCR amplification followed Nam et al. (Citation2000) and McIvor et al. (Citation2001). About 1250 base pairs (bp) of the rbcL gene were amplified and the PCR fragments for sequencing were purified using the High Pure PCR Product Purification Kit (Roche Diagnostics Ltd, Lewes, UK), according to the manufacturer’s instructions. The PCR products were directly sequenced commercially by MWG-Biotech, Ebersberg, Germany.
Type material of Fernandosiphonia unilateralis had been preserved in formalin by Levring (Citation1941) prior to long-term storage in ethanol (A. Athanasiados, personal communication). At the Leiden herbarium, various protocols for retrieving DNA from formalin-preserved specimens were attempted (Kirby & Reid, Citation2001); the most successful was to soak and wash the material repeatedly in clean sterile water, prior to DNA extraction using using a Chelex-100 (Biorad, Hercules, California) protocol (Goff & Moon, Citation1993; Zuccarello et al., Citation1999). Applying a strategy for amplifying degraded ‘ancient’ DNA (Provan et al., Citation2008), primers were designed from an alignment of Neosiphonia harveyi and related species in order to amplify 100-bp fragments. We used the primers F183 (5′ TGCAGGTGAATCTTCTACAGCT 3′) and R383 (5′ ACGTTACCAATAATTGAAGCTGTT 3′).
At the University of Melbourne, DNA was extracted from silica gel-dried material following Saunders & McDevit (Citation2012). PCR amplification was carried out for rbcL using the primers F7/RrbcStart or F57/rbcLrevNEW (Freshwater & Rueness, Citation1994; Saunders & Moore, Citation2013) and for 18S using the primers F47 (5′ AGCCATGCAAGTGCCTGTAT 3′) and R1867 (5′ CGCAGGTTCACCTACGGAAA 3′). Reactions were performed in a total volume of 25 µl, consisting of 5 µl 5× MyTaqTM reaction buffer, 0.7 µl 10 µM of forward and reverse primers, 0.125 µl 1U µl–1 My TaqTM DNA Polymerase (Bioline), 17.475 µl MilliQ® water and 1 µl template DNA. The PCR profile consisted of initial denaturation (93ºC for 3 min); 35 cycles of denaturation (94ºC for 30 s), primer annealing (45ºC for 30 s), and extension (74ºC for 90 s); and final extension (74ºC for 5 min). The PCR products were purified and sequenced commercially by Macrogen.
At A Coruña, Melanothamnus from Oman was extracted using the CTAB protocol (Doyle & Doyle, Citation1987) and rbcL was amplified using the primers F7-R753 and F57-rbcLrevNEW (Freshwater & Rueness, Citation1994; Saunders & Moore, Citation2013). The PCR products were purified and sequenced commercially by the sequencing service of the University of A Coruña.
DNA extraction, amplification and sequencing at UNCW were as described by Stuercke & Freshwater (Citation2008).
Sequence alignment and phylogenetic analysis
A total of 65 rbcL and 48 18S sequences were downloaded from GenBank and 25 new rbcL and 10 new 18S sequences were generated in this study. The sequences and their corresponding GenBank accession numbers are listed in Table S1.
Sequences were aligned using Muscle in Geneious 6.1.8 (Kearse et al., Citation2012). Identical sequences and those that diverged by less than 1.1% were removed from the rbcL analysis, except for Neosiphonia flavimarina and N. harveyi (0.4% divergence), the two selected representatives of the ‘N. japonica complex’ (Kim & Lee, Citation1999), which also includes N. decumbens, N. harlandii and P. akkeshiensis Segi (McIvor et al., Citation2001; Kim & Yang, Citation2006; Bárbara et al., Citation2013; Savoie & Saunders, Citation2015). Identical sequences were also removed from the 18S analysis. The sequences included in the final alignment were selected after considering their quality in terms of both length and the presence of ambiguous bases. Phylogenetic trees for rbcL and 18S were estimated with Maximum likelihood (ML) using RAxML 8.1.6 (Stamatakis, Citation2014). GTR-Gamma was selected as the best nucleotide model; branch support was estimated with 100 bootstrap replicates. Three species of Symphyocladia Falkenberg (in Schmitz & Falkenberg, Citation1897) were selected as the outgroup in the rbcL phylogeny and one species each of Symphyocladia, Xiphosiphonia Savoie & Saunders (Citation2016) and Herposiphonia Nägeli (Citation1846) were selected as outgroups for the 18S analysis. This outgroup selection was based on our phylogenomic analyses of the major lineages of the Rhodomelaceae which resolve a clade formed by the Herposiphonieae and Pterosiphonieae as sister to the Polysiphonieae (Díaz-Tapia et al., Citation2015).
We used MrBayes v.3.2.2 for Bayesian phylogenetic inference (Ronquist et al., Citation2011). The rbcL alignment was analysed using a single (unpartitioned) GTR+Γ+I as well as completely unlinked GTR+Γ+I for each codon position. We used a single GTR+Γ+I model for 18S. All analyses were run for 5 million generations, sampling every 1000th generation and using two independent runs each consisting of four incrementally heated Metropolis-coupled (MCMC) chains. Convergence and stationarity of runs were evaluated with Tracer v.1.6.0 (Rambaut et al., Citation2013), resulting in the use of a burn-in of 500k generations for all analyses. Post-burn-in trees were summarized with the sump command in MrBayes, using the all-compatible-groups consensus type.
Trees were calibrated in geological time using relaxed molecular clock analyses. The calibration was derived from node ages inferred by Yang et al. (Citation2016), which estimated the earliest split in Ceramiales (between Spyridia Harvey (1833) and the remaining Ceramiales) to be 292 Ma old (st dev ≈ 24.6 Ma). After adding the rbcL sequences of Ceramiales from the Yang et al. (Citation2016) study to our alignment and setting Spyridia as the outgroup, node ages were inferred with two Bayesian methods. The first analysis used an autocorrelated model of molecular evolutionary rate change (Thorne & Kishino, Citation2002) as implemented in PhyloBayes v.3.3f (Lartillot et al., Citation2009). The MCMC chain was run for 50k cycles, stationarity was assessed with Tracer, and the node ages summarized with the readdiv command, discarding the first 25k cycles as burn-in. The second analysis used uncorrelated rates of evolution sampled from a lognormal distribution (Drummond et al., Citation2006) as implemented in BEAST v.1.8.2. The MCMC chain was run for 10 million generations, used a Yule tree prior, and an unpartitioned GTR+Γ+I model of sequence evolution. Stationarity was assessed with Tracer. A maximum clade credibility tree and median node heights were inferred with TreeAnnotator, discarding the first 1 million generations as burn-in and using a posterior probability limit of zero.
Results
DNA sequences and alignments
DNA extraction and PCR amplification of type material of Fernandosiphonia unilateralis that had been initially formalin/seawater fixed and then stored in ethanol for several decades yielded a 95 bp partial rbcL sequence with seven ambiguous nucleotides. The sequence was unique by comparison with other taxa sequenced either at QUB or in Leiden, confirming that there had been no contamination.
Twenty-five new rbcL sequences and ten 18S sequences were obtained from members of the Polysiphoniae (Table S1), including an rbcL sequence from Melanothamus somalensis, and four rbcL and two 18S sequences from new collections of F. unilateralis from the type locality. Alignments for the rbcL were unambiguous, with no insertions or deletions.
Phylogenetic analyses
The ML rbcL tree () has three strongly supported major clades within the Polysiphonieae: Polysiphonia sensu stricto 1 (including P. stricta, the type of the genus), Polysiphonia sensu stricto 2 (with morphological features corresponding to those defining Polysiphonia sensu stricto: Kim et al., Citation2000) and a third clade grouping all the other taxa. The third clade comprised a large number of lineages, many with low or intermediate support. The two most speciose lineages, here named Vertebrata and Melanothamnus, however, are both robustly supported (). The Vertebrata clade includes V. lanosa, the current name for the type species of Vertebrata, V. fastigiata S.F.Gray (Citation1821), as well as the type species of several other genera: Brongniartella Bory (Citation1822), Boergeseniella Kylin (Citation1956), Enelittosiphonia Segi (Citation1949) and Ctenosiphonia Falkenberg (in Schmitz & Falkenberg, Citation1897). The Melanothamnus clade includes Fernandosiphonia unilateralis, Neosiphonia flavimarina and M. somalensis, the type species of their corresponding genera. In addition to these two large clades, six other lineages containing 3–4 species are highly supported (BP/PP > 90/0.95); among these are the Carradoriella clade including Polysiphonia virgata, the type species of Caradoriella, and the Streblocladia clade, which includes the type species S. glomerulata. Our phylogenetic tree also resolved five individual species as sisters to the other clades with low support.
The Melanothamnus clade receives support of 100/1.00 (). In addition to F. unilateralis, N. flavimarina and M. somalensis, this clade includes 27 other species currently assigned to Neosiphonia and Polysiphonia. The 95 bp sequence obtained from the type material of F. unilateralis analysed separately showed that this sequence was positioned unequivocally within the Melanothamnus clade, but sequence ambiguities due to the quality of the DNA made it impossible to determine its precise position.
The phylogenetic relationships among species within the Melanothamnus clade are generally poorly resolved, with a few exceptions. Although the lineage formed by ‘Polysiphonia’ schneideri, ‘P.’ amplacapilli, ‘P.’ pentamera and ‘P.’ morroides is very weakly positioned as sister to the Melanothamnus clade in the rbcL tree, in 18S analyses this position is robustly supported (see below).
The RAxML 18S tree () has a similar topology to the rbcL phylogeny, with three strongly supported major clades: Polysiphonia sensu stricto 1 and 2 and a third clade with all the other taxa. Polysiphonia sensu stricto clades 1 and 2 are placed robustly together (99/1.00). Within the third clade, the Vertebrata clade receives full support, while support is lower for Melanothamnus (82/1.00). The sister relationship between the Melanothamnus and ‘P.’ schneideri clades is strongly supported in the 18S phylogeny. In addition, the Carradoriella and Streblocladia clades are highly supported.
The time-calibrated phylogenies (Figs S1, S2) estimated the divergence in Vertebrata to be more ancient than in Melanothamnus (90.7–138.66 vs 75.7–95.78 Ma). Furthermore, the radiation of major lineages in Vertebrata and Melanothamnus was gradual and took place over periods of c. 20 and 12 Ma, respectively.
Morphological observations
An overview of the distribution of selected morphological characters within clades of the Polysiphonieae is shown in .
Habit: There is considerable variation within and among clades (), with the exception of the Carradoriella and Streblocladia clades, in which all species are erect. True prostrate axes giving rise to erect axes, as opposed to decumbent axes that themselves become erect, are confined to Polysiphonia sensu stricto and Vertebrata. Most species of the Melanothamnus clade are completely erect or have a very short prostrate system. However, some taxa are decumbent (e.g. Polysiphonia blandii, P. simplex), forming extensive prostrate systems with rhizoids in the basal parts of the erect axes. Members of the Polysiphonieae are typically smaller than 10 cm. As an exception, M. afaqhusainii can exceed 1 m in length.
Rhizoids: The connection between the rhizoids and the pericentral cells from which they originate is a uniform character within each clade, so far as it can be observed (). Rhizoids are in open connection with pericentral cells in Polysiphonia sensu stricto (), while they are cut off from pericentral cells in the other clades (–). Observations on rhizoids cannot be made in mature specimens of some species, such as Vertebrata lanosa which is an obligate hemi-parasite that lacks rhizoids, and Fernandosiphonia unilateralis, Streblocladia glomerulata and Melanothamnus somalensis, which all have compact basal discs without individual rhizoids.
Pericentral cells and cortication: The number of pericentral cells and the presence of cortication are variable in most of the clades (). All species in the Vertebrata clade have six or more pericentral cells, while members of the Polysiphonia sensu stricto clades have four pericentral cells, with the exception of Bryocladia cuspidata (6–8 pericentrals). Cortication is uniformly absent in the Polysiphonia sensu stricto and ‘P.’ schneideri clades. Cortication is variable within the other clades, absent or slight in small species of Fernandosiphonia but very heavy in Melanothamnus, and absent or slight in most species of Vertebrata with the exception of Boergeseniella, in which cortication is elaborate.
Plastid arrangement: The arrangement of plastids in the cells is a synapomorphy for the Melanothamnus clade. The species in this clade have the plastids lying exclusively on radial walls of pericentral cells so the outer walls appear transparent (, , , –). This particular arrangement of the plastids can be easily observed under the microscope as the cells show a dark flank when observed in detail (, , ), as well as a transparent halo when the pericentral cells are observed in a suitable position (). All the other taxa of the Polysiphonieae have plastids against all the cell walls including the outer wall (, –). The revision of the type materials listed in Table S2, currently assigned to Neosiphonia, allowed us to verify the plastid character in the species Polysiphonia concinna, P. eastwoodiae, P. gorgoniae, P. harlandii and P. johnstonii. Conversely, the species Lophosiphonia mexicana, P. beaudettei, P. confusa, P. poko, P. profunda and P. rubrorhiza have the plastids scattered within the cells, including against the outer wall cells.
Branch/trichoblast arrangement: Whether trichoblasts and/or branches are formed on every segment or are separated by naked segments is variable in three clades, Melanothamnus, Carradoriella and Vertebrata. Most species of the Melanothamnus clade have branches or trichoblasts on every segment, which is a key feature of Neosiphonia. However, there are exceptions in this clade such as Neosiphonia collabens, Polysiphonia nuda and P. pseudovillum from Panama, in which there are interspersed naked segments. Conversely, the formation of trichoblasts/branches with naked segments between them is a uniform character in the Streblocladia, ‘Polysiphonia’ schneideri and Polysiphonia sensu stricto clades (). Within all clades except Melanothamnus, branches may form in a position axillary to trichoblasts, but although constant at the species level, this character is variable within clades. In the Melanothamnus clade this character is absent, and branches are never axillary.
Trichoblast nuclei: The proximal cells of trichoblasts are multinucleate in the Vertebrata clade (, –), with up to 8 or more nuclei in the basal cell and decreasing in number towards the apices, which can be uninucleate. The nuclei are uniformly distributed inside the cells, each appearing to have a domain within the cell. Conversely, all the cells of trichoblasts are uninucleate in other clades of the Polysiphonieae (, –). The only known exception is Leptosiphonia schousboei, which sometimes has two nuclei in the trichoblast cells.
Branching pattern: Despite the great significance previously placed on dorsiventral vs radial branching in the Rhodomelaceae, this character varies within all our clades. A primary dorsiventral branching pattern characterizes some species of the clades Melanothamnus (F. unilateralis and N. collabens; , ), Streblocladia (S. glomerulata) and Vertebrata (Ctenosiphonia hypnoides) (). However, this characteristic is not significant in delineating these three genera, as our phylogenetic tree reveals that each of these four species is closely related to others that have spirally or pseudodichotomously arranged branches. For example, the branching pattern of members of the Streblocladia clade varies from dorsiventral in S. glomerulata, the type species, to spiral or pseudodichotomous in Polysiphonia muelleriana and Polysiphonia sp. Likewise, the dorsiventral Neosiphonia collabens is related to species with spiral or pseudodichotomous branching patterns.
Spermatangial branches: Whether spermatangial branches replace trichoblasts completely or replace only one branch of a dichotomously branched trichoblast is a constant character in all clades (). In the two Polysiphonia sensu stricto and Streblocladia clades, spermatangial branches almost uniformly replace trichoblasts (). In the other clades, they are formed on the first dichotomy of modified trichoblasts (, –), with the exception of Vertebrata () as V. lanosa has no trichoblasts in male thalli – they can be observed only occasionally in females. The presence or absence of apical sterile cells on spermatangial branches is variable within clades, except for Carradoriella in which they are present (–, ).
Carpogonial branches: The Melanothamnus clade is characterized by having 3-celled carpogonial branches (). In our study, we observed this character in N. harveyi, N. collabens, P. blandi and P. forfex (, ). By contrast, the other Polysiphonieae uniformly have 4-celled carpogonial branches like the majority of the Rhodomelaceae (, –).
Cystocarps: The outline morphology of cystocarps varies from globose to ovoid in all the clades analysed here (, –). Urceolate cystocarps are exclusive to the Polysiphonia sensu stricto clades (, ). Cells around the ostiole are conspicuously larger than (more than twice the size of) the cells of the pericarp immediately below in most species of the Melanothamnus clade (). However, Neosiphonia harveyi is an exception, and the cells of the ostiole in this species are only slightly larger than the other cells of the pericarp. This character is also seen in Streblocladia glomerulata (). Conversely, the cells of the ostiole in the other four clades are uniformly similar to the cells below (–, ).
Figs 65–70. Cells surrounding the ostiole in the Polysiphonieae. Similar or slightly larger than the cells of the pericarp inmediately below in Polysiphonia stricta (Fig. 65, Polysiphonia sensu stricto clade 1), Vertebrata lanosa (Fig. 66, Vertebrata clade), P. denudata (Fig. 67, Carradoriella clade), and P. schneideri (Fig. 69, ‘P.’ schneideri clade). They are much larger in Streblocladia glomerulata (Fig. 68, Streblocladia clade) and Neosiphonia collabens (Fig. 70, Melanothamnus clade). Scale bars: Figs 65–68 and 70, 100 µm; Fig. 69, 60 µm.
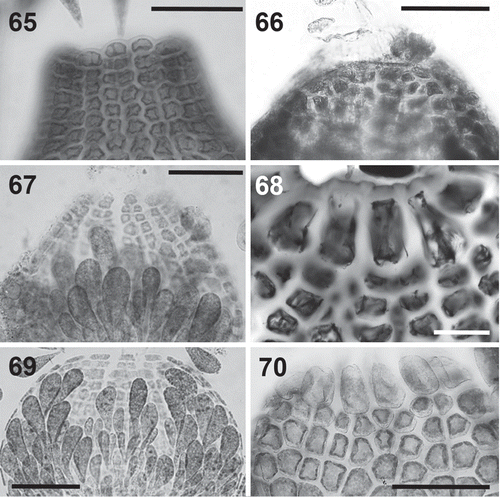
Tetrasporangia: The formation of tetrasporangia in straight or spiral rows is variable in all clades (, –). It must be noted that very long straight series of tetrasporangia are typically observed only in members of the Polysiphonia sensu stricto clade (). However, straight series can also form in other clades, for example in Neosiphonia collabens and Polysiphonia nuda within the Melanothamnus clade, whereas tetrasporangia in Fernandosiphonia unilateralis form short and markedly spiral series (). The third tetrasporangial cover cell is exclusive to the Polysiphonia sensu stricto clade, but this character has not been examined in all the species.
Figs 71–76. Tetrasporangia in the Polysiphonieae. Forming long straight series in Polysiphonia stricta (Fig. 71, Polysiphonia sensu stricto clade 1). Forming spiral series in Vetebrata lanosa (Fig. 72, Vertebrata clade), Polysiphonia sp. (Fig. 74, Streblocladia clade) and Neosiphonia harveyi (Fig. 76, Melanothamnus clade). Forming short straight series in P. denudata (Fig. 73, Carradoriella clade), and P. schneideri (Fig. 75, P. schneideri clade). Scale bars: Figs 71, 74 and 76, 200 µm; Figs 72, 73 and 75, 400 µm.
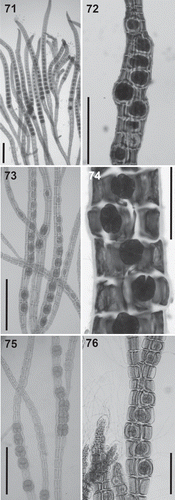
Discussion
Phylogenetic analysis
Amongst the Polysiphonieae studied here, the early-branching clade/clades Polysiphonia sensu stricto 1 and 2 were resolved as separate lineages in rbcL analyses () but together formed a robust clade in 18S analyses (). The marked discordance between rbcL and 18S trees regarding the monophyly/paraphyly of the Polysiphonia sensu stricto lineages requires additional research for a more accurate assessment of relationships and character evolution. Because the Polysiphonia sensu stricto lineages occur near the base of the tree, it is possible that the outgroups (which are relatively distant taxa compared with the ingroup) could have attached to the ingroup in the wrong position in one of the analyses (Shavit et al., Citation2007). Future work should focus on including a wider range of taxa from across the Rhodomelaceae as well as using larger, multi-gene datasets to infer the correct branching order of the two Polysiphonia sensu stricto lineages.
The Vertebrata and Melanothamnus clades were resolved as large, speciose clades with strong support using rbcL. The 18S phylogeny also resolves the Vertebrata clade with robust support, while Melanothamnus is moderately well supported. Both clades are clearly distinguished from Polysiphonia sensu stricto by the rhizoid anatomy (cut off from pericentral cells). Both clades are identified by distinct morphological synapomorphies. The Vertebrata clade is characterized by the multinucleate cells of trichoblasts; the other key feature of Vertebrata, that all species have six or more pericentral cells, is shared with members of some other clades. The Melanothamnus clade is unequivocally distinguished from other Polysiphonieae by two synapomorphic characteristics: the plastid arrangement and the 3-celled carpogonial branches. Furthermore, branch origin is independent from trichoblasts in all the species of this clade, and the majority of species have enlarged ostiolar cells.
In addition to the above-mentioned clades, the rbcL phylogeny resolved six small (3–4 species) but highly supported clades, as well as indicating five species that are uncertainly positioned. The generic assignment of these lineages requires further taxon and gene sampling in order to better understand their phylogenetic relationships and establish a natural classification – it would be premature to speculate on the outcomes of these investigations at present. One of the major shortcomings in Polysiphonieae sequence databases is the uneven geographical sampling, as the majority of sequenced taxa come from Atlantic Europe and central to north-estern America. The generation of molecular data from additional regions could contribute to acquiring a more realistic perspective of the magnitude of unplaced lineages and to delineating their corresponding genera. Also, the resolution of the commonly employed molecular markers in the Polysiphonieae is not sufficient to resolve the phylogenetic relationships among numerous lineages, which could be improved using larger gene datasets (Díaz-Tapia et al., Citation2015).
Taxonomic position of Vertebrata
Vertebrata lanosa is placed in a strongly supported clade that also includes Brongniartella byssoides, Boergeseniella fruticulosa, Ctenosiphonia hypnoides and Enelittosiphonia stimpsonii, the type species of their corresponding genera, and Lophosiphonia reptabunda (which is not the type species). All members of this clade have a synapomorphic characteristic that was previously overlooked in relation to systematics (but see Maggs & Hommersand, Citation1993): multinucleate trichoblast cells. We conclude from molecular and morphological evidence that members of this clade represent a single genus. Vertebrata is the oldest name among those available for this clade, as noted before (Choi et al., Citation2001), and the new combinations proposed in are required. Furthermore, the Vertebrata binomials previously established by Kuntze (Citation1891) should be reinstated for the other 13 species included in this clade ().
Interestingly, Brongniartella is not monophyletic despite its distinctive persistent and pigmented trichoblasts that led to its classification in the tribes Lophothalieae (Falkenberg, Citation1901; Womersley, Citation2003) or Brongniartelleae Parsons (Parsons, Citation1975; Maggs & Hommersand, Citation1993).
Although trichoblasts are typically considered unpigmented in the Polysiphonieae, they are commonly pigmented when young before they enlarge and become colourless (Delivopoulos, Citation2002). The two currently recognized species of Brongniartella, B. byssoides and B. australis, were separated within the Vertebrata clade, respectively placed with V. lanosa and Polysiphonia nigra.
Ctenosiphonia is a monotypic genus segregated from Polysiphonia due to its very peculiar morphological characteristics, including a dorsiventral thallus and two tetrasporangia per segment (Falkenberg, Citation1901; Díaz-Tapia & Bárbara, Citation2013). This genus, together with Lophosiphonia Falkenberg (Citation1897), is currently positioned within the ‘Lophosiphonia group’ (Falkenberg, Citation1901). Boergeseniella and Enelittosiphonia were distinguished from other Polysiphonieae by their particular branching patterns (Segi, Citation1949; Kylin, Citation1956), but our molecular evidence () does not support their recognition as independent genera.
The diversity of currently recognized genera included in this clade reflects the high variability among Vertebrata species in trichoblast characteristics (pigmented/unpigmented; persistent/deciduous; spirally/dorsiventrally arranged) and branching patterns (spiral/dorsiventral; presence or absence of alternating branches of determinate and indeterminate growth), which classical authors considered important at levels of genus and tribe (Falkenberg, Citation1901; Kylin, Citation1956; Hommersand, Citation1963).
The main morphological character delineating the Vertebrata group is that trichoblast cells are multinucleate. In the Polysiphonieae and some other Ceramiales, the apical cell is uninucleate, whereas the cells cut off from it undergo nuclear divisions during elongation, becoming multinucleate, with the number of nuclei being proportional to the volume of the cell (Goff & Coleman, Citation1986; McIvor et al., Citation2002). The trichoblasts of the Rhodomelaceae are usually uninucleate, whereas the polysiphonous parts of the thalli are multinucleate (Coomans & Hommersand, Citation1990; Garbary & Clarke, Citation2001; Delivopoulos, Citation2002). A plausible advantage of having multinucleate trichoblasts in Vertebrata is that their cells can reach larger sizes. In fact, trichoblasts in this genus are sometimes extremely well developed, exceeding 10 mm in length in species such as Vertebrata (Lophosiphonia) reptabunda and V. (Ctenosiphonia) hypnoides. In the red algae, cell streaming is slow compared with other algae (Pueschel, Citation1990), and multinuclearity of large cells may facilitate the regulation of cellular activities. Several potential functions have been attributed to the vegetative hairs of the red algae or trichoblasts of the Rhodomelaceae including desiccation resistance, nutrient uptake, metabolite secretion, shading, trapping of spermatia, mucilage stabilization and monitoring of phosphorus status (Delivopoulos, Citation2002, and references therein). Physically, trichoblasts can form a dense network around the apices that could potentially restrict access to the cells by small grazers, such as amphipods and copepods.
Taxonomic position of Neosiphonia, Fernandosiphonia and Melanothamnus
The presence of the type species of the genus Melanothamnus (M. somalensis) in a strongly supported clade with the type species of the genus Neosiphonia (N. flavimarina) and Fernandosiphonia (F. unilateralis) indicates that Neosiphonia, Fernandosiphonia and Melanothamnus are not distinct monophyletic genera. Neosiphonia is a later heterotypic synonym of Fernandosiphonia. However, the name Melanothamnus is older than both Fernandosiphonia and Neosiphonia, and the new combinations proposed in and are required. These new combinations include 31 species that were previously assigned to Neosiphonia; two species known to be closely related to Neosiphonia but that had been retained in Polysiphonia because their morphology conflicted with Kim & Lee (Citation1999); six species for which molecular data are presented here for the first time; and three species that are transferred to Melanothamnus on the basis of their morphology.
On the other hand, 10 species that are currently placed in Neosiphonia should be replaced in Polysiphonia for formal purposes pending clarification of their phylogenetic affinities and generic assignment. Polysiphonia beaudettei, P. confusa, P. echinata, P. elongella, P. poko, P. rubrorhiza and P. profunda were assigned to Neosiphonia based on morphological characteristics (Kim & Lee, Citation1999; Abbott et al., Citation2002; Kim & Abbott, Citation2006; Mamoozadeh & Freshwater, Citation2011; Norris, Citation2014). However, they lack the plastid character, and furthermore molecular data for P. echinata and P. elongella show that they do not belong to the Melanothamnus clade (). Likewise, Polysiphonia sertularioides was transferred to Neosiphonia based on the morphology of Korean material attributed to this species (Nam & Kang, Citation2012). However, its type locality is in the Mediterranean, and Atlantic sequences for this species are not in the Fernandosiphonia clade (; Mamoozadeh & Freshwater, Citation2012). Polysiphonia paniculata was transferred to Neosiphonia (Norris, Citation2014), but again it is not in the Melanothamnus clade (, ). Finally, our study of the type material of Lophosiphonia mexicana, also transferred to Neosiphonia (Norris, Citation2014; Table S2), indicates that this species is probably not a member of the Polysiphonieae. As noted by Norris (Citation2014), further studies are needed to clarify the generic placement of this unusual species, and meanwhile we propose to leave it in Lophosiphonia until more information is available.
Specimens of Melanothamnus collected in Oman and housed in MICH were initially assigned to M. somalensis (Wynne & Banaimoon, Citation1990), before the description of M. afaqhusainii from Pakistan (Shameel, Citation1999). Revision of the Omani materials leads us to conclude that both M. somalensis and M. afaqhusainii are represented in Oman, and their morphology agrees with the criteria proposed by Shameel (Citation1999) and Afaq-Husain & Shameel (Citation2000) for distinguishing them. Their rbcL sequences diverged by 1.4% (18 bp).
The Melanothamnus clade is morphologically distinguished from other members of the tribe Polysiphonieae by an unequivocal synapomorphic character: plastids lie exclusively on the radial walls of the pericentral cells and are absent from outer walls. The plastid character was previously noted by Hollenberg (Citation1961, Citation1968a), who described ‘hyaline cell walls’ for several species (e.g. P. pseudovillum, P. bajacali), and by Maggs & Hommersand (Citation1993). However, its significance at higher taxonomic levels has not previously been highlighted. We observed this character in a total of 35 species, and we conclude that it is uniform in the Melanothamnus clade. Conversely, other Polysiphonieae and most of the Rhodomelaceae have plastids distributed within the cytoplasm, some lying against outer cell walls. In the family, the only other exception is some species of Herposiphonia in which the plastids form transverse bands (Hollenberg, Citation1968c; Womersley, Citation2003; Díaz-Tapia & Bárbara, Citation2013).
Carpogonial branches are typically 4-celled throughout the family Rhodomelaceae. Three-celled carpogonial branches were described for the first time in Polysiphonia platycarpa (Iyengar & Balakrishnan, Citation1950), and later this was one of the features proposed to delineate the genus Neosiphonia (Kim & Lee, Citation1999). Three-celled carpogonial branches have been reported in 17 species (four of them in the ‘japonica-complex’), all of which are placed here in Melanothamnus. Alternative interpretations of the carpogonial branch configuration were found in the literature for F. unilateralis, as Levring (Citation1941) described and illustrated a 4-celled structure, while Morrill (Citation1976, plate 37, figs E and H) illustrated 3-celled carpogonial branches in the same species, also from the type locality. This character can be easily misinterpreted if the carpogonial branches are not observed at the right developmental stage. In our study of the type material, a single procarp was observed in a permanent slide (), most probably the same one illustrated by Levring. It is unclear how this procarp should be interpreted because it is too mature, and so it is difficult to determine with certainty which cell corresponds to the sterile basal cell and which to the basal cell of the carpogonial branch. From the evidence of the presence of both the plastid character and 3-celled carpogonial branches, Kintarosiphonia fibrillosa Uwai & Masuda (Citation1999), based on Pterosiphonia fibrillosa, and Polysiphonia platycarpa are also here transferred to Melanothamnus ().
The other morphological characters proposed by Kim & Lee (Citation1999) to delineate the genus Neosiphonia vary among closely related species, except for the rhizoid anatomy. Rhizoids are cut off from the pericentral cells in all Polysiphonieae except for Polysiphonia sensu stricto in which they are in open connection with the pericentral cells. After the establishment of Neosiphonia, numerous species were transferred to the new genus based on morphology, but commonly overlooking the number of cells in carpogonial branches. Excluding this trait, several species have all five characteristics proposed by Kim & Lee (Citation1999) to delineate Neosiphonia but nevertheless are not in the Melanothamnus clade (e.g. P. brodiei, P. echinata, P. elongella), while several species are clearly in the clade (e.g. M. collabens, M. nuda, M. pseudovillum) but lack this combination of traits.
The key morphological feature of Melanothamnus is the restriction of plastids to the radial walls of the pericentral cells and their absence from the outer walls. Algae demonstrate a notable decline in photosynthesis at higher light levels possibly due to damage to the photosynthetic apparatus caused by excessive light delivery to photosystem II (Lüning, Citation1990; Hurd et al., Citation2014). Many green and brown algal plastids have phototropic reactions to blue and UV light in order to protect them from irradiation damage (Lüning, Citation1990). Plastid movement, however, has never been demonstrated for the vast majority of red algae (Pueschel, Citation1990), and it appears that red algae have evolved other types of protection against UV damage. Red algae including Polysiphonia species have high concentrations of various mycosporine-like amino acids (MAAs) that respond rapidly to environmental change and act as defences against the photooxidative effects of sunlight (Karsten et al., Citation1998; Navarro et al., Citation2014). The movement of the plastids onto the radial walls of the periaxial cells, in combination with MAAs, may have given the Melanothamnus ancestor a selective advantage over other Polysiphonieae, allowing it to exploit new ecological niches. The prevalence of Melanothamnus species in habitats with exposure to high light levels, such as in Hawaii or turfs on coral reefs (Price & Scott, Citation1992; Kim & Abbott, Citation2006), supports this speculation.
Biogeography of Vertebrata and Melanothamnus
The genus Vertebrata is distributed worldwide, and representatives have been described from all regions where there has been a detailed study of the tribe Polysiphonieae. The majority of our sequences are from Europe, but our systematic review and unpublished data suggest that this genus is widespread. BEAST and PhyloBayes calibrations indicate radiation of the major lineages of the Vertebrata clade over a 20 MA period starting about 140 or 90 Ma (estimates from different methods; see Figs S1, S2). Further conclusions as to its origins and centres of diversity would be premature, pending more comprehensive sampling.
In contrast, Melanothamnus is predominantly Indo-Pacific (). Although few molecular data are available from Indian coasts, some species occur in South Africa (M. incompta), Oman (M. somalensis and M. afaqhusainii), India (M. platycarpa) and Thailand (M. thailandica). Among the regions for which there is a comprehensive study of the Polysiphonieae, the diversity of Melanothamnus is particularly high in Korea, Japan and Hawaii (14, 11 and 14 species, respectively). This genus is also well represented on North American Atlantic coasts (4–5 species), but it is almost completely absent from Atlantic and Mediterranean Europe, where only two species have been reported, which are both most probably examples of old human-mediated introductions. Melanothamnus harveyi is native to south-eastern Asia and has been introduced by multiple events onto northern Atlantic coasts (McIvor et al., Citation2001; Savoie & Saunders, Citation2016). Similarly, M. collabens is likely to be an old introduction into the Atlantic, where it extends from the Bay of Biscay to Cape Verde, including the western Mediterranean (Díaz-Tapia & Bárbara, Citation2013). The finding of M. collabens in California (as P. johnstonii, see ) supports this hypothesis, but although Polysiphonia johnstonii was first collected from the Gulf of California in 1921 (Setchell & Gardner, Citation1924), California was probably not the original source of the introduction. An investigation of Polysiphonia species from the Northern Gulf of California (Hollenberg & Norris, Citation1977) suggested that since its initial collection and description, P. johnstonii has extended its range along the Pacific coast of North America, fulfilling one of the criteria for an invasive species (Chapman & Carlton, Citation1991; Ribera & Boudouresque, Citation1995). How far this species has spread along the Pacific coast of America and along North Atlantic coastlines remains to be determined, and further sampling is needed to establish its origin.
Fig. 77. World map representing the proportion of Melanothamnus (black) and other Polysiphonieae (grey) species in selected regions where the Polysiphonieae were studied in detail. Data were obtained from the following references after updating the species names: Alaska: Lindstrom (http://www.seaweedsofalaska.com); Brazil (Espírito Santo-São Paulo): Guimâraes et al. (Citation2004); California: Abbott & Hollenberg (Citation1976); Florida: Schneider & Searles (Citation1991); Hawaii: Abbott (Citation1999); Japan: Yoshida (Citation1998); Korea: Nam & Kang (Citation2012); Panama: Mamoozadeh & Freshwater (Citation2012); South Africa: Stegenga et al. (Citation1997); southern France: Lauret (Citation1967, Citation1970) Spain (Galicia): Bárbara et al. (Citation2005); British Isles: Maggs & Hommersand (Citation1993).
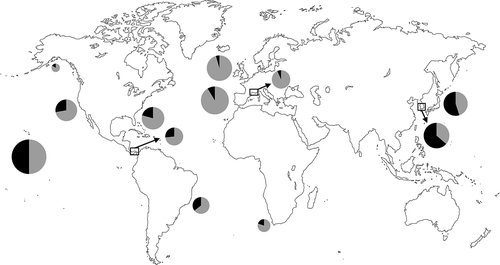
The absence of naturally occurring Melanothamnus species in the Mediterranean and north-eastern Atlantic might suggest that Melanothamnus is of recent origin, having evolved in the Pacific Ocean after the closure of the Tethys Seaway, between 60 and 20 million years ago, particularly as the sister ‘P.’ schneideri clade is also primarily Pacific in distribution. In our rbcL phylogeny (), the ‘P.’ schneideri clade includes two Korean species and two species distributed in the Pacific and North America with one of them introduced in Europe (Díaz-Tapia et al., Citation2013a). Furthermore, our surveys in Australia revealed five other Indo-Pacific species belonging to this clade (unpublished data). However, BEAST and PhyloBayes calibrations indicate radiation of the major lineages of the Melanothamnus clade over a 12 Ma period starting about 95 or 75 Ma (the two methods providing different estimates), with divergence from the ‘P.’ schneideri clade 140 or 95 Ma (Figs S1, S2). The distribution resembles a Tethyan one that originated during the Cretaceous 125–75 Ma (Lüning, Citation1990; Hommersand, Citation2007) when the Tethys Ocean formed a tropical girdle around the earth. Unlike typical Tethyan distributions, in addition to its wide occurrence throughout the tropics, Melanothamnus occupies more temperate regions in the North Pacific (e.g. Japan, Korea) and the South Pacific/Oceania (e.g. South Australia, New Zealand). The question of whether Melanothamnus failed to colonize the north-eastern Atlantic as it opened up during the Cretaceous, or whether north-eastern Atlantic lineages evolved but became extinct, perhaps during Pleistocene glaciations, cannot be answered at present.
Supplementary information
The following supplementary material is accessible via the Supplementary Content tab on the article’s online page at http://dx.doi.org/10.1080/09670262.2016.1256436
Supplementary Table S1. Sample information for the species included in the phylogenetic analysis.
Supplementary Table S2. List of herbarium specimens examined for comparison with Melanothamnus species.
Supplementary Table S3. Percentage of bases that differ (below) and are identical (above) for the species sequences of the Melanothamnus clade included in our rbcL alignment.
Supplementary Table S4. Percentage of bases that differ (below) and are identical (above) for the species sequences of the Vertebrata clade included in our rbcL alignment.
Supplementary Fig. S1. Chronogram resulting from the Bayesian relaxed molecular clock analysis performed with BEAST.
Supplementary Fig. S2. Chronogram resulting from the autocorrelated molecular clock analysis performed with PhyloBayes.
1256436_-_supplementary_material.zip
Download Zip (246.6 KB)Acknowledgements
We are pleased to recognize here that Professor Max H. Hommersand (UNC Chapel Hill) independently discovered the key morphological character of the position of plastids in the Melanothamnus clade. We thank Joana Costa for providing us samples of Polysiphonia spp. from South Africa, and Dr Erasmo Macaya for supplying material from his recent collections of Fernandosiphonia unilateralis from the type locality. We are grateful to Dr Cynthia Trowbridge for providing samples from Japan, and Dr Show-Mei Lin for collecting samples in Taiwan. Professor Gary Saunders is warmly thanked for generously sharing sequences for Melanothamnus afaqhusainii from Oman. We acknowledge the curators of the herbaria BM(NH), TFC, US and TCD; Dr Joe Zuccarello provided host support for Lynne McIvor during her Marie Curie fellowship at Leiden Herbarium. We thank two anonymous reviewers for their valuable comments on the manuscript.
Disclosure statement
No potential conflict of interest was reported by the author(s).
Additional information
Funding
Notes on contributors
Pilar Díaz-Tapia
P. DÍaz-Tapia: original concept, drafting and editing manuscript; determination and analysis of molecular data; L. McIvor: original concept; determination of molecular data; D.W. Freshwater: determination and analysis of molecular data; editing manuscript; H. Verbruggen: analysis of molecular data; editing manuscript; M.J. Wynne: collection and study of Melanothamnus somalensis and M. afaqhusianii; editing manuscript; C. Maggs: original concept, drafting and editing manuscript.
Lynne McIvor
P. DÍaz-Tapia: original concept, drafting and editing manuscript; determination and analysis of molecular data; L. McIvor: original concept; determination of molecular data; D.W. Freshwater: determination and analysis of molecular data; editing manuscript; H. Verbruggen: analysis of molecular data; editing manuscript; M.J. Wynne: collection and study of Melanothamnus somalensis and M. afaqhusianii; editing manuscript; C. Maggs: original concept, drafting and editing manuscript.
D. Wilson Freshwater
P. DÍaz-Tapia: original concept, drafting and editing manuscript; determination and analysis of molecular data; L. McIvor: original concept; determination of molecular data; D.W. Freshwater: determination and analysis of molecular data; editing manuscript; H. Verbruggen: analysis of molecular data; editing manuscript; M.J. Wynne: collection and study of Melanothamnus somalensis and M. afaqhusianii; editing manuscript; C. Maggs: original concept, drafting and editing manuscript.
Heroen Verbruggen
P. DÍaz-Tapia: original concept, drafting and editing manuscript; determination and analysis of molecular data; L. McIvor: original concept; determination of molecular data; D.W. Freshwater: determination and analysis of molecular data; editing manuscript; H. Verbruggen: analysis of molecular data; editing manuscript; M.J. Wynne: collection and study of Melanothamnus somalensis and M. afaqhusianii; editing manuscript; C. Maggs: original concept, drafting and editing manuscript.
Michael J. Wynne
P. DÍaz-Tapia: original concept, drafting and editing manuscript; determination and analysis of molecular data; L. McIvor: original concept; determination of molecular data; D.W. Freshwater: determination and analysis of molecular data; editing manuscript; H. Verbruggen: analysis of molecular data; editing manuscript; M.J. Wynne: collection and study of Melanothamnus somalensis and M. afaqhusianii; editing manuscript; C. Maggs: original concept, drafting and editing manuscript.
Christine A. Maggs
P. DÍaz-Tapia: original concept, drafting and editing manuscript; determination and analysis of molecular data; L. McIvor: original concept; determination of molecular data; D.W. Freshwater: determination and analysis of molecular data; editing manuscript; H. Verbruggen: analysis of molecular data; editing manuscript; M.J. Wynne: collection and study of Melanothamnus somalensis and M. afaqhusianii; editing manuscript; C. Maggs: original concept, drafting and editing manuscript.
References
- Abbott, I.A. (1999). Marine Red Algae of the Hawaiian Islands. Bishop Museum Press, Honolulu.
- Abbott, I.A. & Hollenberg, G.J. (1976). Marine Algae of California. Stanford University Press, Stanford, CA.
- Abbott, I.A., Fisher, J. & McDermid, K.J. (2002). New reported and revised marine algae from the vicinity of Nha Trang, Vietnam. In Taxonomy of Economic Seaweeds with Reference to some Pacific Species (Abbott, I.A. & McDermid, K. J., editors), 291–321. California Sea Grant College, La Jolla.
- Adams, N.M. (1991). The New Zealand species of Polysiphonia Greville (Rhodophyta). New Zealand Journal of Botany, 29: 411–427.
- Adams, N.M. (1994). Seaweeds of New Zealand. An Illustrated Guide. Canterbury University Press, Christchurch.
- Afaq-Husain, S. & Shameel, M. (2000). Further investigations on the red alga Melanothamnus afaqhusainii (Ceramiales) from the coast of Pakistan. Pakistan Journal of Botany, 32: 15–26.
- Agardh, C.A. (1824). Systema algarum. Berlinginiana, Lundae.
- Agardh, J.G. (1842). Algae maris Mediterranei et Adriatici, observationes in diagnosin specierum et dispositionem generum. Fortin, Masson et Cie, Paris.
- Agardh, J.G. (1863). Species Genera et Ordines Algarum, seu descriptiones succinctae specierum, generum et ordinum, quibus algarum regnum constituitur. Volumen secundum: algas florideas complectens. C.W.K. Gleerup, Lundae.
- Bailey, J.W. (1848). Continuation of the list of localities of algae in the United States. American Journal of Science and Arts, series 2, 6: 37–42.
- Bárbara, I., Cremades, J., Calvo, S., López-Rodríguez, M.C. & Dosil, J. (2005). Checklist of the benthic marine and brackish Galician algae (NW Spain). Anales del Jardín Botánico de Madrid, 62: 69–100.
- Bárbara, L, Choi, H.-G., Secilla, A., Díaz-Tapia, P. Gorostiaga, J.M., Seo, T.-K., Jung, M.Y. & Berecibar, E. (2013). Lampisiphonia iberica gen. et sp. nov. (Ceramiales, Rhodophyta) based on morphology and molecular evidence. Phycologia, 52: 137–155.
- Børgesen, F. (1918). The marine algae of the Danish West Indies. Part 3. Rhodophyceae (4). Dansk Botanisk Arkiv, 3: 241–304.
- Børgesen, F. (1934). Some Indian Rhodophyceae especially from the shores of the Presidency of Bombay-IV. Bulletin of Miscellaneous Information, Royal Botanic Gardens, Kew, 1934: 1–30.
- Bornet, É. (1892). Les algues de P. K. A. Schousboe, récoltées au Maroc & dans la Méditerranée de 1815 a 1829, et déterminées par M. Édouard Bornet. Mémoires de la Société Nationale des Sciences naturelles et Mathématiques de Cherbourg, 28: 165–376.
- Bory de Saint-Vincent, J.B.G.M. (1822). Brongniartelle. Brongniartella. Bot. crypt. (Céramiaires.). In Dictionnaire Classique d’Histoire Naturelle (Audouin, I. et al., editors), 516–517. Rey et Gravier; Baudoin frères, Paris.
- Bustamante, D.E., Won, B.Y., Ramirez, M.E. & Cho, T.O. (2012). Neosiphonia peruviensis sp. nov. (Rhodomelaceae, Rhodophyta) from the Pacific coast of South America. Botanica Marina, 55: 359–366.
- Bustamante, D.E., Won, B.Y. & Cho, T.O. (2013a). Neosiphonia ramirezii sp. nov. (Rhodomelaceae, Rhodophyta) from Peru. Algae, 28: 73–82.
- Bustamante, D.E., Won, B.Y. & Cho, T.O. (2013b). Neosiphonia baliana sp. nov. and N. silvae sp. nov. (Rhodomelaceae, Rhodophyta) from Bali, Indonesia. Botanica Marina, 56: 515–524.
- Chapman, J.W. & Carlton, J.T. (1991). A test of criteria for introduced species: the global invasion by the isopod Synidotea laevidorsalis (Miers, 1881). Journal of Crustacean Biology, 11: 386–400.
- Choi, H.G., Kim, M.S., Guiry, M.D. & Saunders, G.W. (2001). Phylogenetic relationships of Polysiphonia (Rhodomelaceae, Rhodophyta) and its relatives based on anatomical and nuclear small-subunit rDNA sequence data. Canadian Journal of Botany, 79: 1465–1476.
- Coomans, R.J. & Hommersand, M.H. (1990). Vegetative growth and organization. In Biology of the Red Algae (Cole, K.M. & Sheath, R.G., editors), 275–304. Cambridge University Press, Cambridge.
- Dawes, C.J. & Mathieson, A.C. (2008). The Seaweeds of Florida. University Press of Florida, Gainesville, FL.
- Dawson, E.Y. (1944). The marine algae of the gulf of California. The University of Southern California Publications, 3: 189–359.
- Delivopoulos, S. (2002). Ultrastructure of trichoblasts in the red alga Osmundea spectabilis var. spectabilis (Rhodomelaceae, Ceramiales). European Journal of Phycology, 37: 329–338.
- Díaz-Tapia, P. & Bárbara, I. (2013). Seaweeds from sand-covered rocks of the Atlantic Iberian Peninsula. Part 1. The Rhodomelaceae (Ceramiales, Rhodophyta). Cryptogamie, Algologie, 34: 325–422.
- Díaz-Tapia, P., Kim, M.S., Secilla, A., Bárbara, I. & Cremades, J. (2013a). Taxonomic reassessment of Polysiphonia foetidissima (Rhodomelaceae, Rhodophyta) and similar species, including P. schneideri, a newly introduced species in Europe. European Journal of Phycology, 48: 345–362.
- Díaz-Tapia, P., Bárbara, I. & Berecibar, E. (2013b). Vegetative and reproductive morphology of Polysiphonia tripinnata (Rhodmelaceae, Rhodophyta): a new record from the European Atlantic coast. Botanica Marina, 56: 151–160.
- Díaz-Tapia, P., Maggs, C.A., West, J.A. & Verbruggen, H. (2015). Tackling rapid radiations with chloroplast phylogenomics in the Rhodomelaceae. European Journal of Phycology, 50: 54–55.
- Doyle, J.J. & Doyle, J.L. (1987). A rapid DNA isolation procedure for small quantities of fresh leaf tissue. Phytochemical Bulletin, 1: 11–5.
- Drummond, A.J., Ho, S.Y.W., Phillips, M.J. & Rambaut, A. (2006). Relaxed phylogenetics and dating with confidence. PLoS Biology, 4: e88.
- Falkenberg, P. (1901). Die Rhodomelaceen des Golfes von Neapel und der angrenzenden Meeres-Abschnitte. Fauna und Flora des Golfes von Neapel, Monographie 26. Berlin.
- Freshwater, D.W. & Rueness, J. (1994). Phylogenetic relationships of some European Gelidium (Gelidiales, Rhodophyta) species, based on rbcL nucleotide sequence analysis. Phycologia, 33: 187–194.
- Garbary, D.J. & Clarke, B. (2001). Apoptosis in trichoblast development in Polysiphonia harveyi (Rhodophyta). Phycologia, 40: 324–329.
- Goff, L.J. & Coleman, A.W. (1986). A novel pattern of apical cell polyploidy, sequential polyploidy reduction and intercellular nuclear transfer in the red alga Polysiphonia. American Journal of Botany, 73: 1109–1130.
- Goff, L.J. & Moon, D.A. (1993). PCR amplification of nuclear and plastid genes from algal herbarium specimens and algal spores. Journal of Phycology, 29: 381–384.
- Gray, S.F. (1821). A Natural Arrangement of British Plants. … Baldwin, Cradock & Joy, Paternoster-Row, London.
- Greville, R.K. (1824). Flora Edinensis …. William Blackwood, Edinburgh, & T. Cadell, London.
- Grunow, A. (1874). Algen der Fidschi-, Tonga- und Samoa-Inseln, gesammelt von Dr. E. Graeffe. Journal des Museum Godeffroy, 3: 23–50.
- Guimarâes, S.M.P.D.B., Fujii, M.T., Pupo, D. & Yokoya, N.S. (2004). Reavaliaçao das características morfológicas e suas implicaçoes taxonômicas no gênero Polysiphonia sensu lato (Ceramiales, Rhodophyta) do litoral dos estados de Sao Paulo e Espírito Santo, Brasil. Revista Brasileira de Botânica, 27: 163–183.
- Guiry, M.D. & Guiry, G.M. (2016). AlgaeBase. World-wide electronic publication, National University of Ireland, Galway. http://www.algaebase.org; searched on 7 February 2016.
- Hariot, P. (1891). Liste des algues marines rapportés de Yokoska (Japon) par M. le Dr Savatier. Mémoires de la Société nationale des sciences naturelles de Cherbourg, 27: 211–230.
- Harvey, W.H. (1847). Nereis Australis, or Algae of The Southern Ocean … [Part 1]. Reeve Brothers, London.
- Harvey, W.H. (1853). Nereis boreali-americana; or, contributions towards a history of the marine algae of the Atlantic and Pacific coasts of North America. Part II. Rhodospermeae. Smithsonian Contributions to Knowledge, 5: [i–ii], [1]–258, pls XIII–XXXVI.
- Harvey, W.H. (1855). Algae. In The botany of the Antarctic voyage of H.M. discovery ships Erebus and Terror, in the years 1839–1843, under the command of Captain Sir James Clark Ross. II. Flora Novae-Zelandiae. Part II. Flowerless plants (Hooker, J.D., editor), pp. 211–266. Reeve, London.
- Harvey, W.H. (1857 ‘1856’). Algae. In: Account of the Botanical specimens (Gray, A., editor) Narrative of the expedition of an American squadron to the China Seas and Japan, performed in the years 1852, 1853 and 1854, under the command of Commodore M.C. Perry, United States Navy. Volume II – with illustrations. (Anon, editors), pp. 331–332. Senate of the Thirty-third Congress, Second Session, Executive Document. House of Representatives, Washington.
- Harvey, W.H. (1859). Phycologia Australica. Vol. 2. Lovell Reeve & Co, London.
- Harvey, W.H. (1860). Characters of new algae, chiefly from Japan and adjacent regions, collected by Charles Wright in the North Pacific Exploring Expedition under Captain James Rodgers. Proceedings of the American Academy of Arts and Sciences, 4: 327–335.
- Harvey, W.H. (1862). Phycologia australica.Vol. 4. Lovell Reeve & Co, London.
- Hollenberg, G.J. (1942). An account of the species of Polysiphonia on the Pacific coast of North America. I. Oligosiphonia. American Journal of Botany, 29: 772–785.
- Hollenberg, G.J. (1944). An account of the species of Polysiphonia on the Pacific coast of North America. II. Polysiphonia. American Journal of Botany, 31: 474–483.
- Hollenberg, G. J. (1961). Marine red algae of Pacific Mexico, Part 5: The genus Polysiphonia. Pacific Naturalist, 2: 345–375.
- Hollenberg, G.J. (1968a). An account of the species of Polysiphonia of the central and western tropical Pacific ocean. II Oligosiphonia. Pacific Science, 22: 56–98.
- Hollenberg, G.J. (1968b). An account of the species of Polysiphonia of the central and western tropical Pacific ocean. I. Polysiphonia. Pacific Science, 22: 198–207.
- Hollenberg, G. J. (1968c). An account of the species of the red alga Herposiphonia occurring in the Central and Western tropical Pacific Ocean. Pacific Science, 22: 536–559.
- Hollenberg, G.J. & Norris, J.N. (1977). The red alga Polysiphonia (Rhodomelaceae) in the Northern Gulf of California. Smithsonian Contributions to the Marine Sciences, 1: 1–21.
- Hommersand, M.H. (1963). The morphology and classification of some Ceramiaceae and Rhodomelaceae. University of California Publications in Botany, 35: 165–366.
- Hommersand, M.H. (2007). Global biogeography and relationships of the Australian marine macroalgae. In Algae of Australia (McCarthy, P.M. & Orchard, A.E., editors), 511–542. Australian Biological Resources Study, Canberra.
- Hooker, J.D. & Harvey, W.H. (1845). Algae Novae Zelandiae. London Journal of Botany, 4: 521–551.
- Hudson, W. (1762). Flora anglica; exhibens plantas per regnum angliae sponte crescentes, distributas secundum systema sexuale: cum differentiis specierum, synonymis auctorum, nominibus incolarum, solo locorum, tempore florendi, offìcinalibus pharmacopoeorum. J. Nourse & C. Moran, London.
- Hurd, C.L., Harrison, P.J., Bischof, K. & Lobban, C.S. (2014). Seaweed Ecology and Physiology. 2nd ed. Cambridge University Press, Cambridge.
- Iyengar, M. O. P., Balakrishnan, M. S. (1949). Morphology and cytology of Polysiphonia platycarpa Boergs. Preliminary note. Proceedings of the Indian Academy of Sciences, Section B 29: 105–108.
- Iyengar, M.O.P. & Balakrishnan, M.S. (1950). Morphology and cytology of Polysiphonia platycarpa Boerges. Proceedings of the Indian Academy of Science B, 31: 135–161.
- Karsten, U., Sawall, T., Hanelt, D., Bischof, K., Figueroa, F.L., Flores-Moya, A. & Wiencke, C. (1998). An inventory of UV-absorbing mycosporine-like amino acids in macroalgae from polar to warm-temperate regions. Botanica Marina, 41: 443–454.
- Kearse, M., Moir, R., Wilson, A., Stones-Havas, S., Cheung, M., Sturrock, S., Buxton, S., Cooper, A., Markowitz, S., Duran, C., Thierer, T., Ashton, B., Mentjies, P. & Drummond, A. (2012). Geneious Basic: an integrated and extendable desktop software platform for the organization and analysis of sequence data. Bioinformatics, 28:1647–1649.
- Kim, M.S. (2003). Taxonomic reassessment of the genus Polysiphonia (Rhodomelaceae, Rhodophyta): morphology and reproduction of Neosiphonia decumbens and N. harlandii. Algae, 18: 107–119.
- Kim, M.S. (2005). Taxonomy of a poorly documented alga, Neosiphonia savatieri Rhodomelaceae, Rhodophyta) from Korea. Nova Hedwigia, 81: 163–176.
- Kim, M.S. & Abbott, I.A. (2006). Taxonomic notes on Hawaiian Polysiphonia, with transfer to Neosiphonia (Rhodomelaceae, Rhodophyta). Phycological Research, 54: 32–39.
- Kim, M.S. & Lee, I.K. (1999). Neosiphonia flavimarina gen. et sp. nov. with a taxonomic reassessment of the genus Polysiphonia (Rhodomelaceae, Rhodophyta). Phycological Research, 47: 271–281.
- Kim, B. & Kim, M.S. (2014). Three new species of Polysiphonia sensu lato (Rhodophyta) based on the morphology and molecular evidence. Algae, 29: 183–195.
- Kim, B. & Kim, M.S. (2016). Neosiphonia yongpilii sp. nov. (Rhodomelaceae, Rhodophyta), known as Neosiphonia simplex from Korea, with an emphasis on cystocarp development. Phycologia, 55: 323–332.
- Kim, M.S. & Yang, E.C. (2006). Taxonomy and phylogeny of Neosiphonia japonica (Rhodomelaceae, Rhodophyta) based on rbcL and cpeA/B gene sequences. Algae, 21: 287–294.
- Kim, M.S., Lee, I.K. & Boo, S.M. (1994). Morphological studies of the red alga Polysiphonia morrowii Harvey on the Korean coast. Korean Journal of Phycology, 9: 185–192.
- Kim, M.-S., Maggs, C.A., McIvor, L. & Guiry, M.D. (2000). Reappraisal of the type species of Polysiphonia (Rhodomelaceae, Rhodophyta). European Journal of Phycology, 35: 83–92.
- Kirby, R.R. & Reid, P.C. (2001). PCR from the CPR offers a historical perspective on marine population ecology. Journal of the Marine Biological Association of the United Kingdom, 81: 539–540.
- Kraft, G.T. & Abbott, I.A. (2002). The anatomy of Neotenophycus ichthyosteus gen. et sp. nov. (Rhodomelaceae, Ceramiales), a bizarre red algal parasite from the central Pacific. European Journal of Phycology, 37: 269–278.
- Kuntze, O. (1891). Revisio generum plantarum. Pars II. Arthur Felix, Dulau & Co., U. Hoepli, Gust. A. Schechert, Charles Klincksieck, Leipzig, London, Milan, New York, Paris.
- Kützing, F.T. (1863). Tabulae phycologicae; oder, Abbildungen der Tange. Vol. XIII. Gedruckt auf kosten des Verfassers (in commission bei W. Köhne), Nordhausen.
- Kützing, F.T. (1864). Tabulae phycologicae; oder, Abbildungen der Tange. Vol. XIV. Gedruckt auf kosten des Verfassers (in commission bei W. Köhne), Nordhausen.
- Kylin, H. (1941). Californische Rhodophyceen. Acta Universitatis Lundensis, 37(2): 1–71.
- Kylin, H. (1956). Die Gattungen der Rhodophyceen. CWK Gleerups Förlag, Lund.
- Lam, W.D., García-Fernández, M.E., Aaboal, M. & Vis, M.L. (2013). Polysiphonia subtilissima (Ceramiales, Rhodophyta) from freshwater habitats in North America and Europe is confirmed as conspecific with marine collections. Phycologia, 52: 156–160.
- Lartillot, N., Lepage, T. & Blanquart, S. (2009). PhyloBayes 3: a Bayesian software package for phylogenetic reconstruction and molecular dating. Bioinformatics, 25: 2286–2288.
- Lauret, M. (1967). Morphologie, phénologie, répartition des Polysiphonia marins du littoral Languedocien. I. Section Oligosiphonia. Naturalia Monspeliensia, Bot., 18: 347–373.
- Lauret, M. (1970). Morphologie, phenologie, repartition des Polysiphonia marins du littoral Languedocien. II. Section Polysiphonia. Naturalia Monspeliensia, Bot., 21: 121–163.
- Lee, Y. (2008). Marine Algae of Jeju. Academy Publication, Seoul.
- Levring, T. (1941). Die Meeresalgen der Juan Fernandez-Inseln. Die Corallinaceen der Juan Fernandez-Inseln. In The Natural History of Juan Fernandez and Easter Island, Vol. 2 (Skottsberg, C., editor), 601–670, 753–757. Almqvist & Wiksells Boktryckeri, Uppsala.
- Lüning, K. (1990). Seaweeds: Their Environment, Biogeography and Ecophysiology. Wiley Interscience Publications, New York.
- Maggs, C.A. & Hommersand, M.H. (1993). Seaweeds of the British Isles. Volume 1. Rhodophyta, Part 3A Ceramiales. Natural History Museum/HMSO, London.
- Mamoozadeh, N.R. & Freshwater, D.W. (2011). Taxonomic notes on Caribbean Neosiphonia and Polysiphonia (Ceramiales, Florideophyceae): five species from Florida, USA and Mexico. Botanica Marina, 54: 269–292.
- Mamoozadeh, N.R. & Freshwater, D.W. (2012). Polysiphonia sensu lato (Ceramiales, Florideophyceae) species of Caribbean Panama including Polysiphonia lobophoralis sp. nov. and Polysiphonia nuda sp. nov. Botanica Marina, 55: 317–347.
- Masuda, M., Kudo, T., Kawaguchi, S. & Guiry, M. D. (1995). Lectotypification of some marine red algae described by W. H. Harvey from Japan. Phycological Research, 43: 191–202.
- Masuda, M., Abe, T., Kawaguchi, S. & Phang, S. M. (2001). Taxonomic notes on marine algae from Malaysia. VI. Five species of Ceramiales (Rhodophyceae). Botanica Marina, 44: 467–478.
- McIvor, L., Maggs, C.A., Provan, J. & Stanhope, M.J. (2001). rbcL sequences reveal multiple cryptic introductions of the Japanese red alga Polysiphonia harveyi. Molecular Ecology, 10: 911–919.
- McIvor, L., Maggs, C. A., Stanhope, M. J. (2002). RbcL sequences indicate a single evolutionary origin of multinucleate cells in the red algal tribe Callithamnieae. Molecular Phylogenetics and Evolution 23: 433–446.
- Millar, A.J.K. (1990). Marine red algae of the Coffs Harbour region, northern New South Wales. Australian Systematic Botany, 3: 293–593.
- Morrill, J.F. (1976). Comparative morphology and taxonomy of some dorsiventral and some parasitic Rhodomelaceae. Thesis, University of North Carolina, Chapel Hill.
- Muangmai, N., Yamagishi, Y., Maneekat, S. & Kaewsuralikhit, C. (2014). The new species Neosiphonia thailandica sp. nov. (Rhodomelaceae, Rhodophyta) from the Gulf of Thailand. Botanica Marina, 57: 459–467.
- Nägeli, C. (1846). Über Polysiphonia und Herposiphonia. Zeitschrift für wissenschaftliche Botanik, 4: 207–256.
- Nam, K.W. & Kang, P.J. (2012). Algal flora of Korea. Volume 4, Number 4. Rhodophyta: Ceramiales: Rhodomelaceae: 18 genera including Herposiphonia. National Institute of Biological Resources, Incheon.
- Nam, K.W., Maggs, C.A., McIvor, L. & Stanhope, M.J. (2000). Taxonomy and phylogeny of Osmundea (Rhodomelaceae, Rhodophyta) in Atlantic Europe. Journal of Phycology, 36: 759–772.
- Navarro, N.P., Mansilla, A., Figueroa, F., Korbee, N., Jofre, J. & Plastino, E. (2014). Short-term effects of solar UV radiation and NO3– supply on the accumulation of mycosporine-like amino acids in Pyropia columbina (Bangiales, Rhodophyta) under spring ozone depletion in the sub-Antarctic region, Chile. Botanica Marina, 57: 9–20.
- Noda, M. & Kitami, T. (1971). Some species of marine algae from Echigo Province facing the Japan Sea. Scientific Reports Niigata University, Ser. D. (Biology), 8: 35–52.
- Norris, R.E. (1994). Some cumophytic Rhodomelaceae (Rhodophyta) occuring in Hawaiian surf. Phycologia, 33: 434–443.
- Norris, J.N. (2014). Marine algae of the northern Gulf of California, II: Rhodophyta. Smithsonian Institution Scholarly Press, Washington D.C.
- Okamura, K. (1912). Icones of Japanese Algae. Vol. II (10), pls. 96–100. Privately published, Tokyo.
- Parsons, M.J. (1975). Morphology and taxonomy of the Dasyaceae and the Lophothalieae (Rhodomelaceae) of the Rhodophyta. Australian Journal of Botany, 23: 549–713.
- Parsons, M. J. (1980). The morphology and taxonomy of Brongniartella Bory sensu Kylin (Rhodomelaceae, Rhodophyta). Phycologia, 19: 273–295.
- Phillips, L.E. (2001). Morphology and molecular analysis of the Australasian monotypic genera Lembergia and Sonderella (Rhodomelaceae, Rhodophyta), with a description of the tribe Sonderelleae trib. nov. Phycologia, 40: 487–499.
- Phillips, L.E., Choi, H.G., Saunders, G.W. & Kraft, G.T. (2000). The morphology, taxonomy and molecular phylogeny of Heterocladia and Trigenea (Rhodomelaceae, Rhodophyta),with delineation of the little known tribe Heterocladieae. Journal of Phycology, 36: 199–219.
- Price, I.R. & Scott, F.J. (1992). The turf algal flora of the Great Barrier Reef. Part I. Rhodophyta. Townsville, Australia: Botany Department, James Cook University.
- Provan, J., Booth, D., Todd, N.P., Beatty, G.E. & Maggs, C.A. (2008). Tracking biological invasions in space and time: elucidating the invasive history of the green alga Codium fragile using old DNA. Diversity and Distributions, 14: 343–354.
- Pueschel, C. M. (1990). Cell structure. In Biology of the Red Algae (Cole, K.M. & Sheath, R.G., editors), 7–41. Cambridge University Press, Cambridge.
- Rambaut, A., Suchard, M.A., Xie, W. & Drummond, A.J. (2013). Tracer MCMC Trace Analysis Tool, Version v1.6.0. Available from: http://tree.bio.ed.ac.uk/software/tracer/
- Ribera, M.A. & Boudouresque, C.F. (1995). Introduced marine plants, with special reference to macroalgae: mechanisms and impact. In Progress in Phycological Research (Round, F.E. & Chapman, D.J., editors), 187–268. Biopress Ltd.
- Ronquist, F., Teslenko, M., Mark, P. van der, Ayres, D., Darling, A., Höhna, S., Larget, B., Liu, L., Suchard, M.A. & Huelsenbeck, J.P. (2011). MrBayes 3.2: efficient Bayesian phylogenetic inference and model choice across a large model space. Systematic Biology, 61: 539–542.
- Savoie, A.M. & Saunders, G.W. (2015). Evidence for the introduction of the Asian red alga Neosiphonia japonica and its introgression with Neosiphonia harveyi (Ceramiales, Rhodophyta) in the Northwest Atlantic. Molecular Ecology, 24: 5917–5937.
- Savoie, A.M. & Saunders, G.W. (2016). A molecular phylogenetic and DNA barcode assessment of the tribe Pterosiphonieae (Ceramiales, Rhodophyta) emphasizing the Northeast Pacific. Botany, 94: 917–939.
- Saunders, G.W. & McDevit, D.C. (2012). Methods for DNA barcoding photosynthetic protists emphasizing the macroalgae and diatoms. Methods in Molecular Biology, 858: 207–222.
- Saunders, G.W. & Moore, T.E. (2013). Refinements for the amplification and sequencing of red algal DNA barcode and RedToL phylogenetic markers: a summary of current primers, profiles and strategies. Algae, 28: 31–43.
- Schmitz, F. & Falkenberg, P. (1897). Rhodomelaceae. In Die natürlichen Pflanzenfamilien nebst ihren Gattungen und wichtigeren Arten insbesondere den Nutzpflanzen unter Mitwirkung zahlreicher hervorragender Fachgelehrten, Teil 1, Abteilung 2 (Engler, A. & Prantl, K. editors), 421–480. Verlag von Wilhelm Engelmann, Leipzig.
- Schneider, C. W. & Searles, R. B. (1991). Seaweeds of the Southeastern United States: Cape Hatteras to Cape Cañaveral. Duke University Press, Durham and London.
- Segi, T. (1949). On the systematic position of the so-called Polysiphonia hakodatensis Yendo from Japan. Siebutsu, 14: 134–139.
- Segi, T. (1951). Systematic study of the genus Polysiphonia from Japan and its vicinity. Journal of the Faculty of Fisheries, Prefectual University of Mie, 1: 169–272.
- Setchell, W.A. (1926). Tahitian algae collected by W.A. Setchell, C.B. Setchell and H.E. Parks. University of California Publications in Botany, 12: 61–142.
- Setchell, W.A. & Gardner, N.L. (1924). XXIX Expedition of the California Academy of Sciences to the Gulf of California in 1921. The Marine Algae. Proceeding of the California Academy of Science, 4: 695–949.
- Setchell, W.A. & Gardner, N.L. (1930). Marine algae of the Revillagigedo Islands expedition in 1925. Proceedings of the California Academy of Sciences, 4: 109–215.
- Shameel, M. (1999). Melanothamnus afaqhusainii, a new red alga from the coast of Karachi. Pakistan Journal of Botany, 31: 211–214.
- Shavit, L., Penny, D., Hendy, M.D. & Holland, B.R. (2007). The problem of rooting rapid radiations. Molecular Biology and Evolution, 24: 2400–2411.
- Sherwood, A.R., Kurihara, A., Conklin, K.Y., Sauvage, T. & Presting, G.G. (2010). The Hawaiian Rhodophyta biodiversity survey (2006–2010): a summary of principal findings. BMC Plant Biology, 10: 258.
- Silva, P.C., Basson, P.W. & Moe, R.L. (1996). Catalogue of the benthic marine algae of the Indian Ocean. University of California Publications in Botany, 79: 1–1259.
- Stamatakis, A. (2014). RAxML Version 8: a tool for phylogenetic analysis and post-analysis of large phylogenies. Bioinformatics, 30: 1312–1313.
- Stegenga, H., Bolton, J.J. & Anderson, R.J. (1997). Seaweeds of the South African west coast. Bolus Herbarium, University of Cape Town, Cape Town.
- Stuercke, B. & Freshwater, D.W. (2008). Consistency of morphological characters used to delimit Polysiphonia sensu lato species (Ceramiales, Florideophyceae): analyses of North Carolina, USA specimens. Phycologia, 47: 541–559.
- Stuerke, B. & Freshwater, D.W. (2010). Two new species of Polysiphonia (Ceramiales, Florideophyceae) from the western Atlantic. Botanica Marina, 53: 301–311.
- Suhr, J.N. von (1831). Beschreibung einiger neuen Algen. Flora, 14: 673–687, 709–716, 725–731.
- Thiers, B. (2016) [continuously updated]. Index Herbariorum: A global directory of public herbaria and associated staff. New York Botanical Garden’s Virtual Herbarium. http://sweetgum.nybg.org/science/ih/.
- Thorne, J.L. & Kishino, H. (2002). Divergence time and evolutionary rate estimation with multilocus data. Systematic Biology, 51: 689–702.
- Uwai, S. & Masuda, M. (1999). Kintarosiphonia (Rhodomelaceae, Ceramiales), a new red algal genus based on Pterosiphonia fibrillosa Okamura from Japan. Phycologia, 38: 225–233.
- Womersley, H.B.S. (1979). Southern Australian species of Polysiphonia Greville (Rhodophyta). Australian Journal of Botany, 27: 459–528.
- Womersley, H.B.S. (2003). The Marine Benthic Flora of Southern Australia. Rhodophyta. Part IIID. Ceramiales- Delesseriaceae, Sarcomeniaceae, Rhodomelaceae. Australian Biological Resources Study & State Herbarium of South Australia, Canberra & Adelaide.
- Wulfen, F.X. (1789). Plantae rariores carinthiacae. In Collectanea ad botanicam, chemiam, et historiam naturalem, spectantia cum figuris (Jacquin, J.N., editor). Ex Officina Wappleriana, Vienna.
- Wynne, M.J. & Banaimoon, S.A. (1990). The occurrence of Jolyna laminarioides (Phaeophyta) in the Arabian Sea and the Indian Ocean and a new report of Melanothamnus somalensis (Rhodophyta). Botanica Marina, 33: 213–218.
- Yang, E.U., Boo, S.M., Bhattacharya, D., Saunders, G.W., Knoll, A.H., Fredericq, S., Graf, L. & Yoon, S.H. (2016). Divergence time estimates and the evolution of major lineages in the florideophyte red algae. Scientific Reports, 6: 21361.
- Yoon, H.Y. (1986). A taxonomic study of genus Polysiphonia (Rhodophyta) from Korea. Korean Journal of Phycology, 1: 3–86.
- Yoshida, T. (1998). Marine Algae of Japan. Uchida Rokakuho Publishing Co., Tokyo.
- Zuccarello, G.C., West, J.A., Kamiya, M. & King, R.J. (1999). A rapid method to score plastid haplotypes in red seaweeds and its use in determining parental inheritance of plastids in the red alga Bostrychia (Ceramiales). Hydrobiologia, 401: 207–214.