ABSTRACT
In Chile, 90% of the fish farms and major natural shellfish beds are located in the region surrounding the Inland Sea, where over the last few decades harmful phytoplankton blooms have often been observed. The onset and recurrence of bloom events are often related to the resuspension and germination of resting cysts that have accumulated in the sediments. The degree of cyst settling, accumulation and germination is highly variable between areas and depends on physical and environmental factors. To learn how differences in oceanographic exposure, amount of river runoff and bathymetry affect dinoflagellate cyst deposition, we examined the diversity and abundance of dinoflagellate resting cysts from two hydrographically contrasting coastal areas (oceanic Guaitecas Archipelago and estuarine Pitipalena Fjord) of the Chilean Inland Sea in September 2006, seven months after a bloom of Alexandrium catenella, a producer of paralytic shellfish toxin. Cyst species diversity consisted of 18 taxa, including A. catenella and the noxious species Protoceratium reticulatum, both of which have caused blooms in the study area. Our results revealed significant differences between the two study sites in terms of the abundance and diversity of resting cysts, suggesting that in the specific case of A. catenella, only Guaitecas stations have potential for cyst accumulation and successful growth of cells. However, there was no evidence of long-term resting cyst beds of A. catenella at either study site.
Introduction
Dinoflagellates are the source of dense and often toxic blooms in coastal waters worldwide (Smayda, Citation1997). The ability of some species to form resistant cysts is a common factor underlying three key recurrent ecological events: bloom initiation, termination and the long-range dispersal of dinoflagellate cells. Cyst germination may provide the inocula for new proliferation events, while cyst formation has been related to bloom decline and the transport of cells as resting cysts (Anderson et al., Citation2005). For cyst-forming species, the benthic life cycle conferred by resting cysts allows the cells to endure a period with environmental conditions unfavourable for growth, and serves as a genetic reservoir (review by Bravo & Figueroa, Citation2014). Consequently, resting cysts may serve as a local ‘seed bed’ by remaining viable in the sediments for years until the appropriate conditions trigger their germination (Dale, Citation1983; McQuoid et al., Citation2002; Lundholm et al., Citation2011; Ribeiro et al., Citation2011; Miyazono et al., Citation2012).
Heterogeneous, patchy spatial distributions are characteristic of dinoflagellate resting cyst deposits in sediments reflecting variations in the timing of cyst deposition, the activity of benthic animals, tidal scouring and sediment properties (Anderson et al., Citation1982). Therefore, understanding the role played by resting cysts in bloom events requires extensive studies of the sediments in affected areas along with the influence of local hydrodynamic factors, as both may be important determinants of high local cyst accumulations (‘hot spots’ of bloom initiation) (Dale, Citation1983; Nehring, Citation1993). The potential of an area to accumulate dinoflagellate resting cysts can be determined by scrutinizing sediments some time after the bloom of a dinoflagellate species which is known to produce them in important amounts during these episodes – as is the case for the genus Alexandrium. Accordingly, the present study was performed seven months after a bloom of Alexandrium catenella (Whedon & Kofoid) Balech 1985, a resting-cyst-forming species that is the causative agent of paralytic shellfish poisoning (PSP) in many areas of the world, including southern Chile. Recent studies conducted in north-western Patagonia failed to detect large A. catenella ‘cyst seed beds’ (Alves de Souza et al., Citation2008; Seguel et al., Citation2011; Díaz et al., Citation2014), but the role played by cysts in outbreaks of this species is not clearly established because it might depend on local physical and hydrological environments. Although high cyst abundances have been detected in specific areas shortly after bloom events, cyst densities decreased too rapidly to induce new outbreaks (Díaz et al., Citation2014; Mardones et al., Citation2016). Whether this pattern is widespread cannot be confirmed until many bloom locations are studied.
We examined dinoflagellate cyst abundance in two coastal systems of the Chilean Inland Sea that are characterized by very different oceanographic conditions: Guaitecas Archipelago and Pitipalena Fjord. The results provide a record of the diversity of dinoflagellate resting cysts in the area and demonstrate how differing hydrological environments can affect cyst accumulation in the sediments. We also discuss the potential for the reported dinoflagellate cyst deposits to originate local PSP blooms.
Materials and methods
Study area
Guaitecas Archipelago and Pitipalena Fjord are located in the north-west of the Chilean fjord and channels region (hereafter referred to as the ‘Chilean Inland Sea’) (). The complex coastal morphology and abrupt bathymetry, and freshwater runoff and rainfall are the main factors accounting for the highly stratified water column of the Inland Sea, especially along the mainland coast (Pickard, Citation1971; Silva et al., Citation1995). The Guaitecas Archipelago is located in the north-western Chilean Inland Sea () where effects of river runoff are negligible, average annual rainfall is ~1500 mm and salinity in the uppermost 50 m of the water column varies between 27 and 33 psu (Molinet et al., Citation2008). The 22 km-long Pitipalena Fjord is a semi-closed system located in the north-eastern part of the sea (), where the average annual rainfall is ~4500 mm. This is a deeper-water up-fjord, with the largest freshwater inputs occurring around its mouth at the confluence with the Palena River. The annual discharge rate of this river fluctuates between 600 and 1080 m3 s−1, with a mean of 800 m3 s−1 (Supplementary fig. S1). Other, smaller streams flow into the fjord and thus maintain a surface layer of fresh water in its inner region, where salinity in the uppermost 15 m of the water column varies between 5 and 32 psu (Díaz et al., Citation2011). Temperature, salinity and density profiles of the waters at Guaitecas and Pitipalena differ significantly, mainly in the upper 10 m (Supplementary fig. S2). As shown in fig. S2, water stratification is stronger in Pitipalena, a tendency also found in studies by Díaz et al. (Citation2011) and Molinet et al. (Citation2008). There are semidiurnal tides at both study sites with amplitudes ranging from 2 m (neap tides) to 4 m (spring tides) (www.shoa.cl).
Figs 1–3. Chilean Inland Sea. Fig. 1. The map shows a section of the Chilean Inland Sea, including the locations of the two study sites, Guaitecas Archipelago and Pitipalena Fjord. Fig. 2. The four sampling stations (1–4) in Guaitecas Archipelago. Fig. 3. The four sampling stations (5–8) in Pitipalena Fjord. Rivers, main streams and bathymetry are shown.
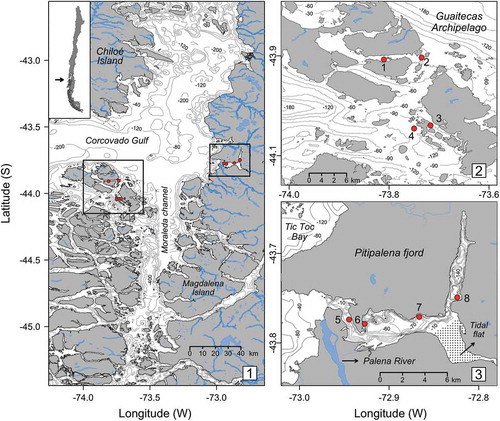
Sampling
Surface sediment samples were collected at four stations (1–4) in the Guaitecas Archipelago () and at four stations (5–8) in Pitipalena Fjord () in September 2006, seven months after the A. catenella bloom. At each station, three sediment samples were collected by an autonomous diver using a corer (8 cm length, 26 mm diameter) at two depths: shallow (5–8 m) and deep (15–20 m). The 48 samples were wrapped in aluminium foil to exclude light stimulation and transported to the laboratory. The first 3 cm of each core was transferred to 80 ml flasks and stored at 4°C until analysis.
Sediment processing and cyst counting
The sediments were cleaned and sonicated following Matsuoka & Fukuyo (Citation2000), with modifications. Sediment subsamples of 5 cm3 were suspended in filtered seawater and sonicated for 1 min. The sonicated material was washed through sieves of 106 μm and 20 μm mesh. The material collected on the 20 μm sieve was tranferred to a Petri dish and the lighter, cyst-containing particles were separated by adding filtered seawater and swirling the plates. The cyst-containing particles were suspended in the circulating water, while heavy sediment particles remained at the bottom in the centre of the dish. Finally, the cysts and other light-weight particles were transferred to a 50 ml tube and filtered seawater was added to reach a final volume of 20 ml, from which three 1 ml aliquots were analysed.
Dinoflagellate cysts were quantified using an Olympus BX40 phase-contrast microscope and a 1 ml Sedgewick-Rafter counting chamber. All cysts present in the chamber were counted. Three aliquots of each sample were processed. The examined cysts were isolated and then photographed using a digital camera (Olympus Camedia C-3040-ADU) coupled to the microscope (100× magnification). Cysts with a full, homogeneously distributed cytoplasmic content were considered viable, as cysts with a partially full or polarized cytoplasm are usually unable to germinate (Genovesi et al., Citation2009).
Statistical analysis
Non-metric multidimensional scaling (NMDS) based on the Bray–Curtis distance matrix was used to assess the similarity of the dinoflagellate cyst assemblages over the two coastal systems (Legendre & Legendre, Citation1998). To normalize the distribution, the data were first log-transformed [LN(x + 1)]. NMDS is appropriate for the analysis of ecological community data, which may contain non-normal or discontinuous scales (Clarke, Citation1993). Dimensionality is determined by a poorness-of-fit criterion (stress) calculated by determining the square root of a normalized residual sum of squares. An ordination with a stress of 0% indicates a perfect fit; a stress of 20% is unacceptable. Analysis of similarities (ANOSIM) was applied to determine differences between sites. ANOSIM is widely used to test hypotheses about the spatial differences in assemblages (Clarke, Citation1993), as it statistically tests whether two or more groups of sampling units differ significantly. The ANOSIM statistic R is based on the difference of mean ranks between and within groups: R=0 indicates no differences, and R=1 that all dissimilarities between groups are larger than the dissimilarity within groups. Similarity percentages and species contributions to the differences between sites were estimated using the similarity percentages (SIMPER) method. All statistical analyses and graphic representations were performed using the statistical and programming software R 2.1.12 (R Development Core Team, Citation2013), packages ‘vegan’ and ‘ggplot2’, available through the CRAN repository (www.r-project.org).
Results and discussion
Analyses of the composition of phytoplankton resting cyst beds provide information on the presence of novel taxa, confirm the recurrent bloom events of a species and may reveal patterns of species co-occurrence. As such, they are important as a species diversity record and to understand blooming patterns in coastal areas. In this study two coastal sites, Pitipalena Fjord and Guaitecas Archipelago, which differ in their oceanographic exposure, amount of river runoff and bathymetry (–), were compared in terms of their dinoflagellate cyst diversity and abundance. The possible relationship of either of these parameters to recent harmful algal bloom (HAB) events in the area was also assessed. Sediment cores were collected from the two sites and the dinoflagellate cyst content in the first 3cm was examined. This fraction is more prone to cyst resuspension events and, as demonstrated for Alexandrium fundyense bloom events in the Gulf of Maine (Anderson etal., Citation2014), correlates with the geographic extent of blooms. Our sediment samples contained cysts belonging to 18 different taxa, including members of 10 different genera: Alexandrium, Protoceratium, Gonyaulax spinifera complex (Nematosphaeropsis), Polykrikos, Archaeperidinium, Diplopelta, Niea, Pentapharsodinium, Protoperidinium and Scrippsiella (, –). Within the Alexandrium genus, the species referred to herein as A. catenella belongs to a species complex whose taxonomic designation has been subject to debate. Several works (e.g. Córdova & Müller, Citation2002; Aguilera-Belmonte et al., Citation2011; Varela et al., Citation2012; Mardones et al., Citation2016) have previously established that Chilean A. catenella corresponds to ribotype group I of the Alexandrium tamarense complex (Lilly et al., Citation2007). In this study, we followed the species name assignment of group I used by Fraga et al. (Citation2015).
Table1. Diversity of nutritional mode (a: autotroph; h: heterotroph) and mean abundance (cysts cm−3) of the 18 types of viable dinoflagellate resting cysts recorded at four stations in Guaitecas Archipelago and Pitipalena Fjord in September 2006. The abundance of empty dinoflagellate resting cysts is shown in parentheses.
Figs 4–23. The diversity of dinoflagellate resting cysts in surface sediments from the study area. Fig. 4 Alexandrium catenella. Fig. 5. Protoceratium reticulatum. Fig. 6 Gonyaulax spinifera complex (Nematosphaeropsis) (empty). Fig. 7. Polykrikos schwartzii. Fig. 8. Diplopelta parva. Fig. 9. Pentapharsodinium dalei. Fig. 10. Protoperidinium americanum (empty). Fig. 11. P. avellana (empty). Fig. 12. P. claudicans. Fig. 13. P. conicoide (empty). Fig. 14. P. conicum. Fig. 15. P. denticulatum (empty). Fig. 16. P. excentricum. Fig. 17. P. leonis (empty). Fig. 18. P. pentagonum. Fig. 19. Scrippsiella sp. Fig. 20. Archaeperidinium minutum. Fig. 21. Niea acanthocysta. Fig. 22. Unidentified cyst type 1. Fig. 23. Unidentified cyst type 2. Scale bars = 10 µm.
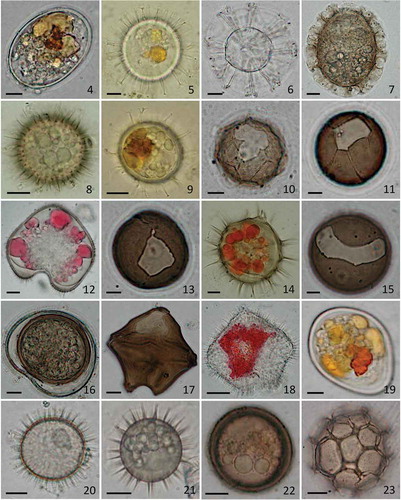
The dinoflagellate cyst diversity reported herein (10 genera) was higher than that determined in other studies carried out in the same region (Alves de Souza et al., Citation2008; Seguel et al., Citation2011; Díaz et al., Citation2014). Moreover, to our knowledge, ours is the first reported detection of Niea acanthocysta and Archaeperidinium minutum in sediments of the inshore seas of Southern Chile. In our samples, heterotrophic dinoflagellate cysts were the most abundant and diverse group (, ). Autotrophs were not identified at Pitipalena stations 5 and 6, and achieved a maximum percentage, close to 50%, at Guaitecas station 3 (). Viable cyst abundances per species and cyst type ranged from 1 to 53 cysts cm−3 at Pitipalena Fjord and from 1 to 44 cysts cm−3 at Guaitecas Archipelago ().
Fig 24. Relative contribution of autotrophs, heterotrophs and unidentified dinoflagellate resting cysts by sampling station. Dinoflagellate orders (Gonyaucales, Gymnodiniales and Peridiniales) are grouped by nutritional mode and are indicated in parentheses.
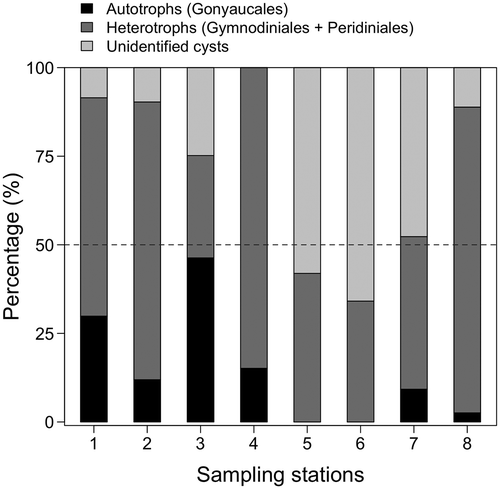
Differences in the abundance and diversity of dinoflagellate resting cysts between sites may result from a combination of factors, including the current system, sedimentation processes and mud content distribution (e.g. Kotani et al., Citation2006). They therefore reveal hotspots of cysts accumulation that should be targeted in future investigations of resting cyst dynamics, and allow monitoring to be intensified in those areas. A NMDS ecological community analysis supported marked differences in the distribution patterns of dinoflagellate cyst assemblages between Guaitecas Archipelago and Pitipalena Fjord, with a clear separation between the two sites (stress=0.08; ). ANOSIM analyses also revealed significant between-site differences in cyst assemblage similarity (R=0.3; ), which could be attributed to the relative contributions of the different species at each site, as determined in the SIMPER analysis (–). The largest percentages dissimilarity between sites (values over 6%, ) are due to the contributions of the species D. parva, P. dalei, A. catenella and P. excentricum, in accordance with the variability in cyst abundances recorded for each species at the studied sites (–). The group of unidentified cysts also contributed importantly to the dissimilarity between sites (), as these cysts were clearly more abundant at Ptipalena than at Guaitecas (–). As highlighted before, at the species scale, autotrophs contributed most to the differences between sites. The variation in cyst-forming dinoflagellate diversity may be related to differences in the surface salinity typically recorded in the two sites (Supplementary fig. S2). Indeed, a strong positive correlation between cyst diversity and surface salinity has been reported in other studies, with decreasing cyst diversity where salinity dropped significantly (Dale, Citation1996; Ellegaard, Citation2000; Sildever et al., Citation2015), demonstrating the low tolerance of some dinoflagellate species to large variations in surface salinity.
Figs 25–29. Fig. 25. Two-dimensional representation of the NMDS analysis. Fig. 26. Box-plot diagram of the ANOSIM results. Fig. 27. Dinoflagellate resting cysts with the greatest contributions to the average dissimilarity of the assemblages at Guaitecas Archipelago and Pitipalena Fjord based on a SIMPER analysis. Figs 28, 29. Mean abundance (cysts cm−3) per dinoflagellate species as determined at Guaitecas Archipelago (Fig. 28) and Pitipalena Fjord (Fig. 29).
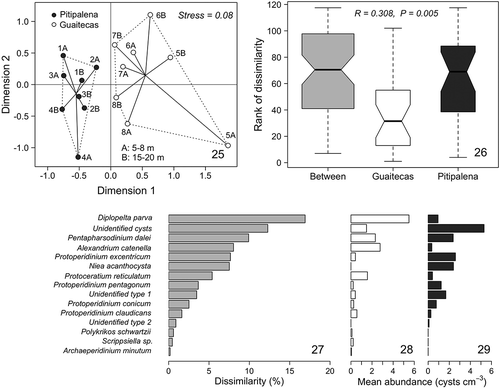
The abundance of dinoflagellate resting cysts (excluding in this first analysis both A. catenella and P. reticulatum) also differed significantly between the two sites with respect to the fraction of viable cysts found in shallow sediments (). The abundance in this layer was generally higher at Guaitecas Archipelago stations, in agreement with Mardones et al. (Citation2016) who previously described a tendency for low cyst concentrations in the inner fjords. The exceptions to this rule were Pitipalena station 6, for P. dalei, P. conicum and P. pentagonum as well as for some unidentified cysts, and Pitipalena station 7 for N. acanthocysta, P. excentricum and P. pentagonum. Niea acanthocysta cysts were only recorded at Pitipalena stations, whereas Polykrikos schawartzii, Archaeperidinium minutum and Scrippsiella sp. cysts were only recorded in shallow sediments of Guaitecas stations.
Fig 30. Total viable cyst abundance and mean abundance (cysts cm−3) of the 10 species and unidentified species recorded at Guaitecas Archipelago (stations 1–4) and Pitipalena Fjord (stations 5–8) in September 2006. Codes for the identified and unidentified species are defined in . Note that types of sediment are included in the top left panel.
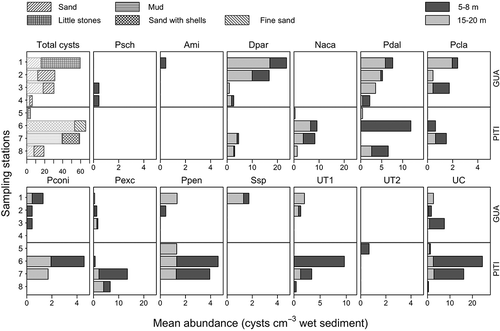
Specific analyses of A. catenella and P. reticulatum identified resting cysts of the two species at both Guaitecas Archipelago and Pitipalena Fjord, but with significant differences in abundances (–). Similar to the general tendency described above for other species, the density of viable A. catenella and P. reticulatum cysts at the two depths studied was significantly lower at Pitipalena Fjord than at Guaitecas Archipelago. Viable cysts of A. catenella reached a maximum abundance (8 cysts cm−3) at station 1 at a depth of 20 m in Guaitecas Archipelago (, ) whereas these cysts were rare and only observed at station 7 in the Pitipalena Fjord (, ). This difference in cyst abundance between sites is consistent with the documented absence of A. catenella HABs at Pitipalena (technical report D.Ac. no. 1267/2012) and their frequent occurrence at Guaitecas Archipelago (Molinet et al., Citation2006). Similarly, Horner et al. (Citation2011) did not find significant amounts of A. catenella cysts in areas where PSP events are not usually recorded. These findings can be interpreted as suggesting a link between cyst beds and bloom development or simply the existence of ‘growth niches’. Under certain conditions, the factors regulating cell division would be more important than the size of the inoculum of germinated cysts (Anderson et al., Citation2014). For example, based on knowledge of the environmental conditions that promote the growth of A. catenella, the fluctuating and low salinity levels of <15 psu that characterize the waters of Pitipalena Fjord could limit the development of A. catenella bloom events. In a previous study of Chilean A. catenella strains, growth rates and maximum cell densities were, in general, more sensitive to low salinities than to temperature fluctuations (Aguilera-Belmonte et al., Citation2013). Thus, the occurrence of HAB events would seem to depend not only on inputs of cells from cyst beds or other sources but also on the species-specific conditions that favour growth. Therefore it seems that in A. catenella both the potential for cyst accumulation and optimal growth of cells are restricted to Guaitecas stations. In contrast, members of the Peridiniales group are less sensitive to marked salinity gradients, such as those occurring at Pitipalena Fjord, and, with the exception of Diplopelta parva, were detected at higher densities there than at Guaitecas Archipelago ().
Figs 31–34. Box-plot diagrams of the mean abundance of: Figs 31, 33. viable cysts of Alexandrium catenella and Figs 32, 34. Protoceratium reticulatum recorded at Guaitecas Archipelago (stations 1–4) and Pitipalena Fjord (stations 5–8) in September 2006 at two different depths: shallow (5–8 m; upper panel) and deep (15–20 m; lower panels).
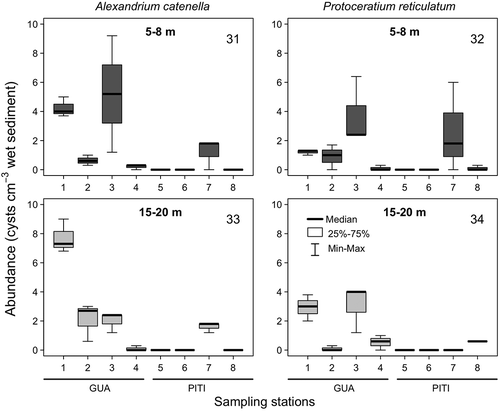
In addition, the local hydrodynamics of Pitipalena Fjord are mostly determined by the semidiurnal tides, together with the residual flow of water outside the fjord (Molinet et al., Citation2006; Díaz et al., Citation2011). Both may affect cyst dynamics by acting as natural (physical) barriers to the introduction of phytoplankton species that bloom outside the fjord. Support for this hypothesis comes from the fact that at station 5, the station with the highest flux (up to 1.2 m s–1) and increased turbulence (Díaz et al., Citation2011), dinoflagellate cysts were scarce (). Díaz et al. (Citation2011), in a study that included the same station, determined a phytoplankton assemblage composed mainly of diatoms (>95%) and a similar low-level presence of dinoflagellates in areas where shear stabilities seemed to dominate flows due to current speeds of high magnitude and the dominance of vertical shears over the sill.
Although granulometry was not analysed in the present study, type of sediment is another relevant factor to consider regarding cyst accumulation. General information about the type of sediment at each station is shown in (‘total cysts’). Guaitecas stations were dominated by sand and, in comparison to Pitipalena, were generally characterized by sediments with larger grain sizes at both depths analysed. However, the type of sediment and cyst concentration are not clearly related in our dataset as, for example, the stations with lower cyst concentrations (4 and 5) have sand and mud, respectively, as the dominant sediment type. In fact, differences in oceanographic exposure could be more relevant in explaining A. catenella resting cyst abundance than sediment type. For example, within one study, there was no correlation between A. catenella cyst abundance and sediment grain size in Puget Sound Bay, whereas at another location there was a correlation between finer grains and A. catenella cyst abundance – a contrasting result which may be because of both the typical patchy distribution of cyst abundances and differences in oceanographic exposure (Horner et al., Citation2011; Genovesi et al., Citation2013).
In general, densities of the viable resting cysts examined in this study (, ) and, specifically, those of A. catenella and P. reticulatum (, –) were very low. These results are in agreement with Seguel et al. (Citation2011) who detected 1.3 resting cysts cm−3 at a site 150 m to the south of our study area; the authors pointed to the lack of a HAB event during the previous summer as a cause of this low density. This was not the case in the present study, given that an intense bloom of this species occurred during the summer (December–March) of 2006 (maximum ~1×106 cells l−1). Therefore, the lack of recent bloom events could not be the reason behind the low resting cyst densities found in this area. A possible explanation for the absence of A. catenella cysts is the rapid germination of resting cysts. Resting cysts produced under laboratory conditions using local strains had a dormancy of 69 days (Mardones et al., Citation2016), which is in agreement with the finding by Díaz et al. (Citation2014) of empty cysts a few months after a bloom event. Environmental factors acting on cyst germination may not be important given that, unlike other cyst types, the germination of Alexandrium cysts does not seem to be affected by temperature, over a large range, or nutrients such as nitrogen and phosphates (Genovesi et al., 2009). However, temperature does seem to synchronize germination with the onset of favourable growth conditions (Anderson, Citation1998; Rathaille & Raine, Citation2011). In the case of sub-surface sediments, cyst resuspension can trigger germination under favourable temperatures, given that dissolved oxygen is a requirement for excystment in Alexandrium (Anderson et al., Citation1987).
Díaz et al. (Citation2014) studied the dynamics of A. catenella cysts after an intense bloom (~1.1×106 cells l–1) in the summer of 2009, comparing locations in NW Patagonia at which freshwater inputs were less important than in either of our study sites. The authors concluded that the waters of NW Patagonia did not serve as an important advection source of inoculum cells for future regional PSP outbreaks, given the low densities of viable cysts remaining in the sediments only a few months after the bloom event. We counted empty resting cysts as an indirect measurement of the extent of recent cyst germination and, therefore, to determine their role in bloom events. While empty cysts of A. catenella were not observed at Guaitecas Archipelago, 130 empty cysts cm−3 of P. reticulatum were counted at station 3 (). This finding suggests that A. catenella resting cysts were washed away from the sediments by the local currents (Pickard, Citation1971; Díaz et al., Citation2014), a hypothesis supported by the very low densities of viable cysts in the area despite the important bloom of the previous summer. Alternatively, the presence of P. reticulatum empty cysts could be explained by their faster settling velocity (up to 37% of the sinking rate), which presumably applies to all cysts with numerous short spines (Anderson et al., Citation1985), or because the production of resting cysts is more constant through time. However, a methodological problem affecting the counting of empty resting cysts of A. catenella cannot be ruled out. For example, in contrast to the readily identifiable characteristics of P. reticulatum resting cysts, A. catenella empty cysts lack colouration and distinct ornamentation, which may have hindered their detection. In addition, cysts of P. reticulatum are highly resistant to decay and thus over-represented in the cyst record (Dale, Citation1976). A well-established method to count empty Alexandrium cysts is primuline staining. However, in our experience, it is not useful for recording the entire dinoflagellate cyst diversity contained in one sample, due to the variable and ambiguous staining properties of some genera (Matsuoka, Citation1989; Matsuoka & Fukuyo, Citation2000; Anderson et al., Citation2003). Nonetheless, our results do highlight the important role that physical oceanographic conditions can play in determining cyst diversity and abundance at a small geographic scale and showed the low, long-term accumulation potential of Alexandrium resting cysts at both study sites.
To keep moving forward, comparative genetic studies of blooming vs. encysted populations of Chilean A. catenella are required to shed light on the origin and sources of the recurrent bloom events of this species in the area. This approach was well demonstrated in the Gulf of Mexico, where Karenia brevis blooms were more prevalent in one area than another. The genetic structure of the K. brevis population supported the hypothesis that the west and south-west coasts of Florida could have been the points of origin for blooms in other regions (Henrichs et al., Citation2013). Similarly, the development of highly polymorphic genetic markers to study the population-level genetic structure of the Alexandrium tamarense complex (Nagai et al., Citation2006; Masseret et al., Citation2009; Genovesi et al., Citation2015) is an interesting and useful approach to compare vegetative and cyst populations in an area. This type of study could confirm or rule out a link between resting cysts and bloom events of A. catenella in the highly dynamic systems of Guaitecas Archipelago and Pitipalena Fjord. Despite the low cyst abundances at both sites, exceptionally favourable conditions for cell growth might promote dense blooms, as occurred during an unusually dense bloom of the closely related Alexandrium fundyense in the shallow system of Northport Harbor, USA (Anglès et al., Citation2012). That study confirmed theoretical work showing that low percentages of resting cyst germination are sufficient for the reintroduction of a species (Estrada et al., Citation2010). However, the minimum number of vegetative cells needed to initiate exponential growth and a bloom event has yet to be determined.
Our study provided a record of the dinoflagellate resting cyst diversity in the study areas and thus of the important differences in resting cyst beds (abundance and diversity) of oceanic and estuarine systems. We found no proof of the existence of long-term A. catenella resting cyst beds, but short-term resting cyst accumulation may contribute to the maintenance of low cell densities in the water column. In this case, environmental conditions affecting cell growth would be the limiting factor in the development of blooms in the area.
Supplementary information
The following supplementary material is accessible via the Supplementary Content tab on the article’s online page at http://10.1080/09670262.2018.1455111
Supplementary fig. S1. Time series of the average daily discharge (m3 s−1) of Palena River from 2000 to 2015.
Supplementary fig. S2. (A) Vertical distribution of temperature (°C), salinity (psu) and sigma-t (kg m−3) at a fixed station in Pitipalena fjord from January 2005 to February 2006. (B) Vertical profiles of the temperature (°C), salinity (psu) and sigma-t (kg m−3) at sampling station in Pitipalena fjord during January 2005 and at sampling station in Guaitecas Island during January 2005, July 2006 and November 2006.
Supplementary figures S1 and S2
Download MS Word (1.3 MB)Acknowledgements
We thank Jaime Valencia and Victor Ruiz for sampling assistance. This work was funded by the Regional Government of Aysén (FNDR project, BIP: 30010880-0). We are grateful to the Chilean Navy Hydrographic Service for providing hydrographic data collecting during the sampling programme of the CIMAR fjord cruises.
Disclosure statement
No potential conflict of interest was reported by the authors.
Additional information
Funding
Notes on contributors
Patricio A. Díaz
P.A. Díaz: original concept, data analysis, drafting and editing manuscript; C. Molinet: original concept, sampling, data analysis and editing manuscript; M. Seguel: original concept, sediment processing, taxonomy and editing manuscript; M. Díaz: sampling, data analysis and editing manuscript; G. Labra: sediment processing, taxonomy, isolation of cysts and photography; R.I. Figueroa: data analysis, drafting and editing manuscript.
References
- Aguilera-Belmonte, A., Inostroza, I., Franco, J.M., Riobo, P. & Gómez, P.I. (2011). The growth, toxicity and genetic characterization of seven strains of Alexandrium catenella (Whedon and Kofoid) Balech 1985 (Dinophyceae) isolated during the 2009 summer outbreak in southern Chile. Harmful Algae, 12: 105–112.
- Aguilera-Belmonte, A., Inostroza, I., Saéz Carrillo, K., Franco, J.M., Riobo, P. & Gómez, P.I. (2013). The combined effect of salinity and temperature on the growth and toxin content of four Chilean strains of Alexandrium catenella (Whedon and Kofoid) Balech 1985 (Dinophyceae) isolated from an outbreak occurring in southern Chile in 2009. Harmful Algae, 23: 55–59.
- Alves de Souza, C., Varela, D., Navarrete, F., Fernández, P. & Leal, P. (2008). Distribution, abundance and diversity of modern dinoflagellate cysts assemblages from southern Chile (43–54°S). Botanica Marina, 51: 399–410.
- Anderson, D.M. (1998). Physiology and bloom dynamics of toxic Alexandrium species, with emphasis on life cycle transitions. In Physiological Ecology of Harmful Algal Blooms (Anderson, D.M., Cembella, A. & Hallegraeff, G., editors), 19–48. Springer-Verlag, Berlin.
- Anderson, D.M., Aubrey, G., Tyler, M.A. & Coats, D.W. (1982). Vertical and horizontal distributions of dinoflagellate cysts in sediments. Limnology and Oceanography, 27: 757–765.
- Anderson, D.M., Lively, J.J., Reardon, E.M. & Price, C.A. (1985). Sinking characteristics of dinoflagellate cyst. Limnology and Oceanography, 30: 1000–1009.
- Anderson, D.M., Taylor, C.D. & Armbrust, E.V. (1987). The effects of darkness and anaerobiosis on dinoflagellate cyst germination. Limnology and Oceanography, 32: 340–351.
- Anderson, D.M., Fukuyo, Y. & Matsuoka, K. (2003). Cyst methodologies. In Manual on Harmful Marine Microalgae: Monographs on Oceanographic Methodology (Hallegraeff, G.M., Anderson, D.M. & Cembella, A.D., editors), 229–249. UNESCO Publishing.
- Anderson, D.M., Stock, C., Keafer, B., Nelson, A., McGillicuddy, D., Keller, M., Thompson, B., Matrai, P. & Martin, J. (2005). Alexandrium fundyense cyst dynamics in the Gulf of Maine. Deep Sea Research II, 52: 2522–2542.
- Anderson, D.M., Keafer, B.A., Kleindinst, J.L., Mcgillicuddy Jr., D.J., Martin, J.L., Norton, K., Pilskaln, C.H., Smith, J.L., Sherwood, C.R. & Butman, B. (2014). Alexandrium fundyense cysts in the Gulf of Maine: long-term time series of abundance and distribution, and linkages to past and future blooms. Deep Sea Research II, 103: 6–26.
- Anglès, S., Garcés, E., Hattenrath-Lehmann, T.K. & Gobler, C.J. (2012). In situ life-cycle stages of Alexandrium fundyense during bloom development in Northport Harbor (New York, USA). Harmful Algae, 16: 20–26.
- Bravo, I. & Figueroa, R.I. (2014). Towards an ecological understanding of dinoflagellate cyst functions. Microorganisms, 2: 11–32.
- Clarke, K.R. (1993). Non-parametric multivariate analyses of changes in commmunity structure. Australian Journal of Ecology, 18: 117–143.
- Córdova, J.L. & Müller, I. (2002). Use of PCR and partial sequencing of the large-subunit rRNA gene to identify Alexandrium catenella (Dinophyceae) from the South of Chile. Harmful Algae, 1: 343–350.
- Dale, B. (1976). Cyst formation, sedimentation, and preservation: factors affecting dinoflagellate assemblages in recent sediments from Trondheims fjord, Norway. Review of Palaeobotany and Palynology, 22: 39–60.
- Dale, B. (1983). Dinoflagellate resting cysts: “benthic plankton”. In Survival Strategies of the Algae (Fryxell, G.A., editor), 69–136. Cambridge University Press, Cambridge.
- Dale, B. (1996). Dinoflagellate cyst ecology: modeling and geological applications. In Palynology: principles and applications (Jansonius, J. & McGregor, D.G., editors), 1249–1275. American Association of Stratigraphic Palynologists Foundation, Utah.
- Díaz, P., Molinet, C., Cáceres, M. & Valle-Levinson, A. (2011). Seasonal and intratidal distribution of Dinophysis spp. in a Chilean fjord. Harmful Algae, 10: 155–164.
- Díaz, P.A., Molinet, C., Seguel, M., Díaz, M., Labra, G. & Figueroa, R. (2014). Coupling planktonic and benthic shifts during a bloom of Alexandrium catenella in southern Chile: implications for bloom dynamics and recurrence. Harmful Algae, 40: 9–22.
- Ellegaard, M. (2000). Variations in dinoflagellate cyst morphology under conditions of changing salinity during the last 2000 years in the Limfjord, Denmark. Review of Palaeobotany and Palynology, 109: 65–81.
- Estrada, M., Solé, J., Anglès, S. & Garcés, E. (2010). The role of resting cysts in Alexandrium minutum population dynamics. Deep Sea Research II, 57: 308–321.
- Fraga, S., Sampedro, N., Larsen, J., Moestrup, Ø., & Calado, A.J. (2015). Arguments against the proposal 2302 by John & al. to reject the name Gonyaulax catenella (Alexandrium catenella). Taxon, 64: 634–635.
- Genovesi, B., Laabir, M., Masseret, E., Collos, Y., Vaquer, A. & Grzebyk, D. (2009). Dormancy and germination features in resting cysts of Alexandrium tamarense species complex (Dinophyceae) can facilitate bloom formation in a shallow lagoon (Thau, southern France). Journal Of Plankton Research, 31: 1209–1224.
- Genovesi, B., Mouillot, D., Laugier, T., Fiandrino, A., Laabir, M., Vaquer, A. & Grzebyk, D. (2013). Influences of sedimentation and hydrodynamics on the spatial distribution of Alexandrium catenella/tamarense resting cysts in a shellfish farming lagoon inpacted by toxic blooms. Harmful Algae, 25: 15–25.
- Genovesi, B., Berrebi, P., Nagai, S., Reynaud, N., Wang, J. & Masseret, E. (2015). Geographic structure evidences in the toxic dinoflagellate Alexandrium pacificum Litaker (A. catenella - group IV (Whedon & Kofoid) Balech) along Japanese and Chinese coastal waters. Marine Pollution Bulletin, 98: 95–105.
- Henrichs, D.W., Renshaw, M.A., Gold, J.R. & Campbell, L. (2013). Population-genetic structure of the toxic dinoflagellate Karenia brevis from the Gulf of Mexico. Journal of Plankton Research, 35: 427–432.
- Horner, R.A., Greengrove, C.L., Davies-Vollum, K.S., Gawel, J.E., Postel, J.R. & Cox, A.M. (2011). Spatial distribution of benthic cysts of Alexandrium catenella in surface sediments of Puget Sound, Washington, USA. Harmful Algae, 11: 96–105.
- Kotani, Y., Matsuyama, Y., Hayashi, M. & Matsuoka, K. (2006). Distribution and abundance of resting cysts of Alexandrium tamarense and/or A. catenella (Dinophyceae) in Tokyo Bay, Japan. Plankton and Benthos Research, 3: 147–154.
- Legendre, P. & Legendre, L. (1998). Numerical ecology. 2nd English ed. Elsevier Science BV, Amsterdam.
- Lilly, E.L., Halanych, K.L. & Anderson, D.M. (2007). Species boundaries and global biogeography of the Alexandrium tamarense complex (Dinophyceae). Journal of Phycology, 43: 1329–1338.
- Lundholm, N., Ribeiro, S., Andersen, T.J., Koch, T., Godhe, A., Ekelund, F. & Ellegaard, M. (2011). Buried alive – germination of up to a century-old marine protist resting stages. Phycologia, 50: 629–640.
- Mardones, J.I., Bolch, C., Guzmán, L., Paredes, J., Varela, D. & Hallegraeff, G.M. (2016). Role of resting cysts in Chilean Alexandrium catenella dinoflagellate blooms revisited. Harmful Algae, 55: 238–249.
- Masseret, E., Grzebyk, D., Nagai, S., Genovesi, B., Lasserre, B., Laabir, M., Collos, Y., Vaquer, A. & Berrebi, P. (2009). Unexpected genetic diversity among and within populations of the toxic dinoflagellate Alexandrium catenella revealed with nuclear microsatellite markers. Applied and Environmental Microbiology, 75: 2037–2045.
- Matsuoka, K. (1989). Micropaleontology and fluorescence: the meaning of autofluorescence show by fossils. Olympus Microscopy Review, 15: 1–9.
- Matsuoka, K. & Fukuyo, Y. (2000). Technical Guide for Modern Dinoflagellate Cyst Study. WESTPAC-HAB/WESTPAC/IOC, Japan.
- McQuoid, M.R., Godhe, A. & Nordberg, K. (2002). Viability of phytoplankton resting stages in the sediments of a coastal Swedish fjord. European Journal of Phycology, 37: 191–201.
- Miyazono, A., Nagai, S., Kudo, I. & Tanizawa, K. (2012). Viability of Alexandrium tamarensis cysts in the sediments of Funka bay, Hokkaido, Japan: over a hundred year survival times for cyts. Harmful Algae, 16: 81–88.
- Molinet, C., Torrijos, C., Díaz, P., Solari, M., Marticorena, F., Jerez, P. & Navarro, M. (2006). Seguimiento de la marea roja en dos localidades de la provincia de Aysén, Melinka y Raúl Marín Balmaceda. Universidad Austral de Chile, Coyhaique.
- Molinet, C., Niklitschek, E., Moreno, C. & Arévalo, A. (2008). Vertical distribution of early and competent larvae of Concholepas concholepas in two systems of Chilean inland seas. Marine Biology, 153: 779–787.
- Nagai, S., Sekino, M., Matsuyama, Y. & Itakura, S. (2006). Development of microsatellite markers in the toxic dinoflagellate Alexandrium catenella (Dinophyceae). Molecular Ecology Notes, 6: 120–122.
- Nehring, S. (1993). Mechanisms for recurrent nuisance algal blooms in coastal zones: resting cyst formation as life-strategy of dinoflagellates. In Interdisciplinary Discussion of Coastal Research and Coastal Managment Issues and Problems (Sterr, H., Hofstade, J. & Plag, H.-P., editors), 454–467. Peter Lang, Frankfurt.
- Pickard, G.L. (1971). Some physical oceanographic features of inlets of Chile. Journal of Fisheries Research Board of Canada, 28: 1077–1106.
- R Development Core Team (2013). R: A Language and Environment for Statistical Computing. R Foundation for Statistical Computing, Vienna. http://www.r-project.org/.
- Rathaille, A.N. & Raine, R. (2011). Seasonality in the excystment of Alexandrium minutum and Alexandrium tamarense in Irish coastal waters. Harmful Algae, 10: 629–635.
- Ribeiro, S., Berge, T., Lundholm, N., Andersen, T.J., Abrantes, F. & Ellegard, M. (2011). Phytoplankton growth after a century of dormancy illuminates past resilience to catastrophic darkness. Nature Communications, 2: 311. doi: 10.1038/n.comms1314.
- Seguel, M., Sfeir, A., González, J., Díaz, P., Molinet, C. & Labra, G. (2011). Quistes de dinoflagelados en sedimentos marinos del sur de Chile con énfasis en Alexandrium catenella y Protoceratium reticulatum. Ciencia y Tecnología del Mar, 34: 5–17.
- Sildever, S., Andersen, T.J., Ribeiro, S.A. & Ellegaard, M. (2015). Influence of surface salinity gradient on dinoflagellate cyst community structure, abundance and morphology in the Baltic Sea, Kattegat and Skagerrak. Estuarine and Coastal Marine Science, 155: 1–7.
- Silva, N., Sievers, H. & Prado, R. (1995). Características oceanográficas y una proposición de circulación, para algunos canales australes de Chile entre 41°20’S y 46°40’S. Revista de Biologia Marina, 30: 207–254.
- Smayda, T. (1997). Harmful algal blooms: their ecophysiology and general relevance to phytoplankton blooms in the sea. Limnology and Oceanography, 42: 1137–1153.
- Varela, D., Paredes, J., Alves De Souza, C., Seguel, M., Sfeir, A. & Frangópulos, M. (2012). Intraregional variation among Alexandrium catenella (Dinophyceae) strains from southern Chile: morphological, toxicological and genetic diversity. Harmful Algae, 15: 8–18.