ABSTRACT
Haslea ostrearia (Gaillon) Simonsen is famous among diatoms for producing a blue pigment. Genome sequencing of different strains of H. ostrearia led to the discovery of the complete genomes of two new bacilladnaviruses, HOV-148 and HOV-235. The DNA sequences of these viruses were identified in H. ostrearia strains that had been maintained for several years in a culture collection. Some of these strains are the products of in vitro episodes of auxosporulation and thus have never been in contact with their natural biotope. At 4567 and 4538 bp, the HOV-148 and HOV-235 genomes are shorter than those of most diatom-infecting viruses known to date. They each contain four open reading frames and display highest similarities with sequences from viruses found in a gastropod from New Zealand, a proximity confirmed by phylogenetic analysis. HOV-148 and HOV-235 were identified in three clones of H. ostrearia that were collected in oyster ponds from distinct locations in the Bay of Bourgneuf (France). Given that our search for similar viral sequences in genomic data derived from other sub-populations or species of the genus Haslea throughout the world was unsuccessful, we hypothesize that these viruses could have a link with the peculiar biotope of the oyster ponds.
Introduction
Diatoms are ecologically important microalgae whose populations and blooms, like those of other types of phytoplankton, can be regulated by viruses (Eissler et al., Citation2009; Miranda et al., Citation2016; Arsenieff et al., Citation2019). Research on diatoms has uncovered diatom-infecting viruses (DIVs) among different host species, with an increasing number of discoveries reported during the last decade (Nagasaki et al., Citation2004, Citation2005; Bettarel et al., Citation2005; Shirai et al., Citation2008; Tomaru et al., Citation2008, Citation2009, Citation2011a, Citationb, Citationc, Citation2012, Citation2013; Eissler et al., Citation2009; Toyoda et al., Citation2012; Kimura & Tomaru, Citation2013). Two different types of DIVs have been identified so far among centric or pennate diatoms: single-stranded RNA (ssRNA) and single-stranded DNA (ssDNA) viruses (Tomaru et al., Citation2012; Kimura & Tomaru, Citation2013). Some of these DIVs were first discovered by direct observation of viral structures within cells using transmission electron microscopy, followed in several cases by isolation and sequencing of their genomes (Tomaru et al., Citation2011a, Citationb, Citationc, Citation2013). Other DIVs were detected during the course of metagenomic studies of samples from complex environments using next generation sequencing techniques (McDaniel et al., Citation2014). Apart from their critical role in the regulation of diatom populations, DIVs are interesting targets in applied research aiming at producing improved strains of diatoms by genetic modification (Kadono et al., Citation2015).
Blue diatoms from the genus Haslea are species of pennate marine diatoms that possess non-photosynthetic blue-green pigments, including the marennine produced and released in seawater by the type species Haslea ostrearia (Gaillon) Simonsen (Poulin et al., Citation2019). H. ostrearia () is also known for the greening of cultured oysters, which is caused by binding of marennine to the gills. Marennine displays allelopathic, antibacterial and antioxidant properties (Pouvreau et al., Citation2007, Citation2008; Gastineau et al., Citation2012a, Citation2014a), implying that the blue pigments of Haslea might have valuable applications in aquaculture and blue technologies (Gastineau et al., Citation2012b, Citation2014b; Turcotte et al., Citation2016; Prasetiya et al., Citation2017; Falaise et al., Citation2019a, Citationb). Recent studies revealed unsuspected biodiversity within the Haslea genus, leading to the description of several new species of blue Haslea and providing insights into their molecular phylogeny and patterns of mitochondrial DNA inheritance during sexual reproduction (Gastineau et al., Citation2012Citationa, Citation2013, Citation2014Citationa, b, Citation2016; Prasetiya et al., Citation2019). Some of these studies are part of an ongoing multidisciplinary project dedicated to blue diatoms and although genome sequencing in the frame of this project is primarily targeted at organellar genomes (e.g. see Prasetiya et al., Citation2019), the resulting data have the potential to lead to unanticipated discoveries.
Strikingly, analysis of genomic data from different strains of H. ostrearia uncovered over-represented sequences that are related to DIVs within strains originating from the Bay of Bourgneuf (French Atlantic Coast). These sequences are from two new bacilladnaviruses, which we refer to as HOV-148 and HOV-235. We report here the discovery and characterization of these viral DNA sequences. We have also searched for related sequences of ssDNA viruses among the genomic data gathered from H. ostrearia strains collected in the Kattegat Strait (Sweden) and Leschenault Estuary (Australia) (Gastineau et al., Citation2013, Citation2014Citationa, b) as well as the new species Haslea nusantara (Mouget, Gastineau & Syakti) from Indonesia (Prasetiya et al., Citation2019).
Materials and methods
Origin of the strains of Haslea ostrearia
The strains of H. ostrearia from the Bay of Bourgneuf were provided by the Nantes Cultures Collection (NCC, University of Nantes, France). The NCC 148.78 strain was isolated following a possible episode of homothallic reproduction occurring in 2007 in a culture of the clone NCC 148, which was obtained in October 2000 from a sample picked in an oyster pond (Claire Tharaud-Port des Brochets-Bouin, 46°59’51”N, 02°01’50’‘W). Clone NCC 235.1 was isolated in 2007 from a different oyster pond in the same area (Claire C. Penisson, 46°59’19’’N, 02°14’14’’W). The NCC 153.8 clone is a product of heterothallic reproduction performed in laboratory in 2005 between clones NCC 141 and NCC 171, both of which were isolated in 2003 from natural populations of the oyster pond Lainard (La Barre de Monts 46°53’33’’N, 02°07’59’’W). The H. ostrearia strain NCC 320 from the Kattegat Strait (Sweden) was described in Gastineau et al. (Citation2013). The strain LE9A2 was isolated from the Leschenault Estuary (Australia) (33°16′0″S, 115°42′0″E) during August 2015. H. nusantara was obtained as described in Prasetiya et al. (Citation2019).
Culture conditions
NCC 148.78, NCC 235.1, NCC 320, LE9A2 and H. nusantara were grown at the Le Mans University at 15°C in sterile modified ASW medium (Perkins et al., Citation2006) under an irradiance of 60 µmol photons m−2 s−1 (14 h/10 h light/dark cycle). Irradiance was measured using a Li-Cor LI-189 quantum meter and a Li-Cor Q21284 quantum sensor, and was provided by fluorescent tubes (Philips TLD 36W/965). NCC 148.78 and NCC 235.1 cultures were supplemented with 1 ml of Antibiotic Antimycotic Solution (Sigma-Aldrich A5955) per 100 ml of culture medium to inhibit bacterial growth. The NCC 153.8 clone was cultured at the University of Heraklion, Greece, in f/2 medium (Guillard Citation1975) at 20°C under an irradiance of 50 µmol photons m−2 s−1 (8 h/16 h light/dark cycle).
DNA extraction and sequencing
For all clones, total cellular DNA was extracted following Doyle & Doyle (Citation1990). For the NCC 148.78 and NCC 235.1 clones, library construction and paired-end sequencing of these libraries (inserts of 500 bp, average read length of 91 bp) on the Illumina HiSeq 2000 platform were performed at the Beijing Genomics Institute (BGI) in Shenzhen. The NCC 320 and LE9A2 clones, as well as H. nusantara, were also sequenced at BGI’s facilities, but on HiSeq 4000, yielding reads of 150 bp from 300-bp inserts. For all these two clones, only clean reads whose quality was verified using FastQC (Andrews, Citation2010) were obtained from BGI. For the NCC 153.8 clone, sequencing took place at the Institute of Applied Biosciences in Thessaloniki. DNA was quantified using the Qubit® dsDNA BR Assay Kit (Invitrogen, Carlsbad, California, USA). Absorbance at 260 nm was measured using a spectrophotometer (BioPhotometer, Eppendorf, Hamburg, Germany). Two libraries were constructed using the Nextera™ DNA Sample Preparation Kit (Illumina Inc, San Diego, California), according to the manufacturer’s protocol with slight modifications concerning the total amount of input DNA (100 ng) and the tagmentation time; 2 or 4 min at 55°C for libraries with average insert sizes of 520 bp and 350 bp, respectively. All PCR reactions were performed in a PTC-200 DNA Engine Thermal Cycler (Biorad, Hercules, California, USA). Targeted DNA was purified from transposomes with DNA Clean & Concentrate™ (Zymo Research; Irvine, California, USA), whereas indexed libraries were purified using NucleoMag® NGS Bead Suspension (Macherey-Nagel, Germany), stained with ethidium bromide, visualized on a 1% agarose gel under UV light and their average size was determined using the UVIDoc Mw software (version 99.04). Quantitative PCR (qPCR) was conducted on a Rotor-Gene Q thermocycler (Qiagen, Hilden, Germany) with the KAPA Library Quantification kit for Illumina sequencing platforms (KAPA BIOSYSTEMS Woburn, Massachusetts, USA). Libraries were loaded on a MiSeq platform (Illumina, San Diego) using the MiSeq® reagent kit v2 (2 × 150 cycles) at 12.5pM and a NextSeq500. Generation of fastq files was performed either by the MiSeq Reporter software or by the bcl2fastq software. Prior to assembly, quality control and trimming were performed using AfterQC (Chen et al., Citation2017).
Bioinformatic analyses and phylogenetic inference
Reads were assembled using SPAdes 3.13.1 (Bankevich et al., Citation2012) with a k-mer of 81. Small contigs displaying much higher sequencing coverage than all other contigs in the same DNA assemblies were subjected to the following analyses. Open reading frames (ORFs) of these contigs, which proved to correspond to viral genomes, were identified using ExPASy (Gasteiger et al. Citation2003) and GETORF from the EMBOSS package (Rice et al., Citation2000). The predicted sequences of the ORF protein products were compared with available sequences using BLASTp online (Boratyn et al., Citation2013) on the NCBI portal. In order to check whether or not viral sequences could be integrated in the genome of either H. ostrearia or remaining bacteria, and thus flanked by distinctive sequences, clean reads were aligned to the viral genomes using the addSolexaReads.perl function of the Consed package (Gordon et al., Citation1998). The complete genome sequences of the viruses were also subjected to a BLASTn search on the TARA blast server (http://bioinfo.szn.it/tara-blast-server/) against the prokaryotic and eukaryotic databases. Viral genomes were annotated using Sequin 13.70 and gene maps were drawn using OGDRAW (Greiner et al., Citation2019). The predicted sequences of putative replication-associated proteins from 18 taxa and the putative capsid proteins from 20 taxa were used to infer a Maximum likelihood phylogeny, following the protocol described in Kazlauskas et al. (Citation2017). The protein sequences were aligned using MAFFT (Katoh & Standley, Citation2016) with the option ‘G-INS-i’ and trimmed using TrimAl (Capella-Gutiérrez et al., Citation2009) with gap threshold of 0.05. Phylogenetic analyses were conducted using RaxML 8 (Stamatakis, Citation2014) with the rtREV model (Dimmic et al., Citation2002). Best trees out of 100 were computed for 100 bootstrap replications. The genomes of HOV-148 and HOV-235 were aligned with Amphibola crenata associated bacilladnavirus 2 (KY405007), Amphibola crenata associated bacilladnavirus 1 (KY405006), Avon-Heathcote estuary associated bacilladnavirus isolate AHEaBavV1 (KY405008) and bacillariodnavirus LDMD-2013 (KF133809), using the Mauve aligner (Darling et al., Citation2010) from the Geneious platform. Sequence logos were also produced for the replication-associated proteins and the capsid proteins, using complete alignments of the amino-acid sequences obtained from HOV-148, HOV-235 and AcrBV2 viruses on the web portal of WebLogo 3 (Crooks et al., Citation2004). To search whether viral sequences similar to those present in the H. ostrearia strains from the Bay of Bourgneuf are also found in other H. ostrearia populations or different Haslea species, DNA assemblies from the NCC 320 and LE9A2 clones and from H. nusantara were searched. Assemblies were subjected to BLASTx searches using as queries the replication-associated proteins encoded by the HOV-148 and HOV-235 genomes (e-value: 1e–50). To check for the presence of bacterial DNA within the reads obtained from the sequencing of NCC 148.78 and NCC 235.1 clones (axenic cultures), a database was built using 16S genes of marine bacteria similar to those evidenced among the contigs retrieved from NCC 153.8 sequencing results (non-axenic culture), and which were mostly Marimonas sp., Pseudoalteromonas sp. and Alteromonas sp. The contigs obtained from NCC 148.78 and NCC 235.1 were subjected to a BLASTn analysis against this database, without any filter of e-value.
Results
About 10 million paired-end reads were obtained for each of the NCC 148.78 and NCC 235.1 clones, 18 million for NCC 320, 22 million for LE9A2 and 83 million for NCC 153.8. Following read assemblies, contigs were sorted according to their coverage. In the cases of the NCC 148.78 and NCC 235.1 strains, two contigs of ~5 kb were found to display the highest coverage depths. Both contigs had overlapping sequences at their termini, indicating that they exist as circular molecules. After trimming and circularization, the NCC 148.78 and NCC235.1 sequences were 4567 and 4538 bp in size, respectively; hereafter, they are referred to as HOV-148 (GenBank: MN229504) and HOV-235 (GenBank: MN229503). A graphical map representing HOV-148 is given as an example (). The coverage depths of HOV-148 and HOV-235 as calculated by SPAdes were 1496 X and 593 X, respectively. In comparison, the coverage values for other contigs of the same assemblies from the two strains were as follows: 110–125 X for the contig sequences corresponding to the large and small single-copy regions of the plastid genome (two contigs), 100–110 X for the mitogenome (one contig), and about 120 X for the nuclear ribosomal gene cluster (one contig). Partial sequences of HOV-235 were identified among the contigs obtained from the NCC 148.78. strain. Also, partial sequences of HOV-235 and HOV-148, were retrieved in the assembly obtained from the NCC 153.8 clone, with an extremely high coverage depth (27 700 X) observed for the HOV-235 contig carrying the replication-associated protein gene. The large and small single-copy regions of the plastid genome of the same clone mapped to several contigs of ~10 kb, all with a coverage depth of 450 X and similarly, several contigs were observed for the mitogenome, with a 560 X coverage value. The block of nuclear ribosomal genes, however, was found in a single large contig displaying a coverage of 610 X.
The BLASTn search for bacterial sequences among contigs obtained from NCC 148 and NCC 235 gave no match, suggesting that the cultures were indeed axenic or with only very weak bacterial contamination. Consed failed at providing evidence that the viral genomes contigs could be flanked by other genomic sequences. It did not align any read that could overlap two distinct genomes, but only reads related to the viral genomes themselves.
Four long ORFs (>450 bp) were identified in both the HOV-148 and HOV-235 sequences ( and ). Bp analyses of these ORFs revealed strong similarities with the genome sequences of two viruses, AcrBV1 (NC_033746) and AcrBV2 (NC_033741), both of which were found to be associated with the gastropoda Amphibola crenata (Dayaram et al., Citation2015; Kazlauskas et al. Citation2017). At 4729 bp and 4576 bp, these viral genomes are comparable in size to those of the HOV-148 and HOV-235 sequences. The alignment performed with MAUVE () also illustrates the similarities between HOV-148, HOV-235 and especially AcrBV2. The putative proteins encoded by the HOV-148 and HOV-235 ORFs showed especially high identities with the AcrBV2 proteins (identity values of 93% and 88% with the replication-associated proteins, 82.35% and 84.62% with the capsid proteins, 90.85% and 94.77% with the ORF1 protein products, and 84.83% and 89.83% with the ORF2 products). These high identities are illustrated in the Logos representation obtained from the alignments of the corresponding amino-acid sequences of the replication-associated proteins () and the capsid proteins () of HOV-148, HOV-235 and AcrBV2. In addition, BLASTp analyses revealed that the HOV-148 and HOV-235 ORFs share sequence similarity, but to a lesser extent, with the four ORFs in the 5749-kb genome of bacillariodnavirus LDMD-2013 (KF133809). The size of this viral genome, which is slightly larger than the HOV-148 and HOV-235 sequences, corresponds to the average genome size of the ssDNA viruses listed in Kimura & Tomaru (Citation2013). The comparisons with the TARA databases was unsuccessful. The best result was a match with EUK:MATOU-v_58866788, however with a high e-value of 7.68 × 10–05.
Fig. 3. MAUVE alignment of the complete genomes of HOV-148, HOV-235 and related viruses. The Y axis represents the percentage of identity between the compared genomes
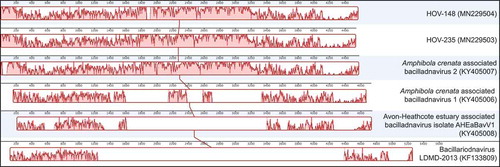
Fig. 4. Sequence motifs of the replication-associated associated proteins of HOV-148, HOV-235 and AcrBV2 viruses. The frames indicate the positions of the conserved motifs of the replication-associated proteins. Frames 1, 2 and 3: motifs I, II and III of the endonuclease domain. Frames 4–7: helicase domain. Frame 4: Walker A motif. Frame 5: Walker B motif. Frame 6: motif C. Frame 7: Arginine finger
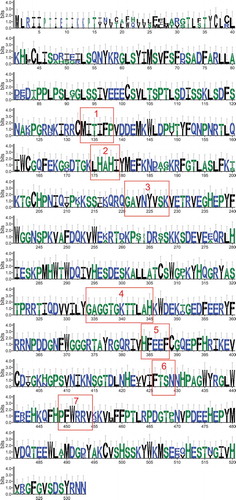
To investigate more closely the relationships of the HOV-148 and HOV-235 sequences with other bacilladnaviruses including DIVs, we inferred a Maximum likelihood tree using an alignment of the replication-associated proteins from 18 taxa () and the capsid proteins from 20 taxa (). Consistent with the results of the above-mentioned BLASTp analyses, HOV-148 and HOV-235 clustered together, forming a strongly supported clade that is sister to the Amphibola crenata-associated bacilladnavirus 2 AcrBV2. With the phylogeny inferred from the replication-associated proteins, Amphibola crenata-associated bacilladnavirus 1 AcrBV1 and bacillariodnavirus LDMD-2013 affiliated with the latter sequences to form a clade that received 73% bootstrap support. On a higher level, the latter clade associated with two other clades including DIVs, mostly bacilladnaviruses infecting Chaetoceros species. A clade containing Halorubrum phage GNf2, the Avon-Heathcote Estuary-associated circular virus 13 and a Gull circovirus represented the outgroup. With the phylogeny inferred from the capsid proteins, HOV-235 associates with AcrBV2 in a couple itself affiliated with HOV-148, and forming a strongly supported larger clade with AcrBV1 (bootstrap support of 100%). The capsid-inferred phylogeny discriminated this clade from its sister clade containing bacillariodnavirus LDMD-2013 and the Avon-Heathcote estuary associated bacilladnavirus isolate AHEaBavV1 with also strong support (90%).
Fig. 6. Maximum likelihood phylogeny inferred from an alignment of 18 replication-associated proteins. The best-scoring RAxML tree (log likelihood = −13107.354663) is presented. Bootstrap support values are reported on the nodes. Evolutionary analyses were conducted using RaxML version 8, with the rtREV evolutive model and 100 bootstrap replications. The scale indicates the number of substitutions per site
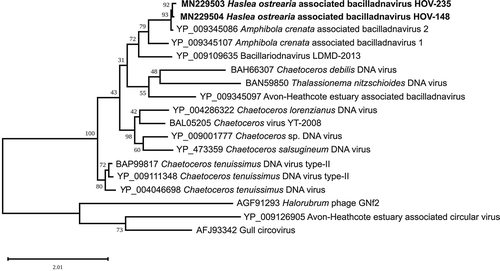
Fig. 7. Maximum likelihood phylogeny inferred from an alignment of 20 capsid proteins. The best-scoring RAxML tree (log likelihood = −13512.785351) is presented. Bootstrap support values are reported on the nodes. Evolutionary analyses were conducted using RaxML version 8, with the rtREV evolutive model and 100 bootstrap replications. The scale indicates the number of substitutions per site
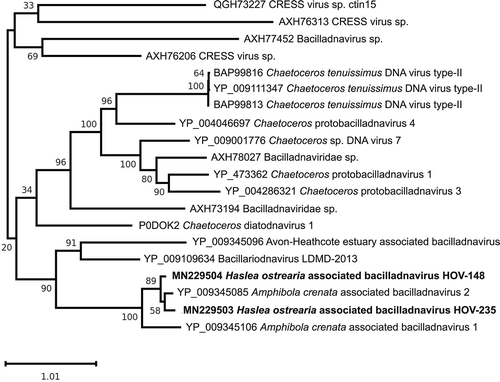
To determine whether viral sequences similar to those present in the H. ostrearia strains collected in the Bay of Bourgneuf are found in sub-populations of this species, genome assemblies of H. ostrearia isolates from the Kattegat Strait and Leschenault Estuary were subjected to BLASTx searches using as queries the replication-associated proteins encoded by the HOV-148 and HOV-235 genomes (e-value: 1e–50). No significant match was obtained in these analyses; moreover, a similar search using genomic contigs of H. nusantara was also unsuccessful. Insufficient sequencing depth is not likely to explain these negative results, as the total number of paired-end reads obtained for each of these strains was about two-fold higher compared with those derived from the NCC 148 and NCC 235 clones.
Discussion
Origin of the viral sequences present in H. ostrearia
By revealing that blue H. ostrearia strains from the Bay of Bourgneuf harbour overly represented circular DNA molecules that are highly similar in gene content and gene organization to bacilladnavirus genomes, our study provides new evidences for the existence of single-stranded DNA viruses (HOV-148 and HOV-235) in pennate diatoms, as only a single species, Thalassionema nitzschioides, was known to host bacilladnaviruses TnitDNAV (Tomaru et al., Citation2012). Our results were not anticipated considering that the examined diatom cultures were monoclonal and axenic, and that no special protocol was used to isolate and concentrate viruses or to extract viral DNA. In comparison, the previously reported bacillariodnavirus LDMD-2013 (McDaniel et al., Citation2014) and the recently described viruses of Guinardia delicatula (Arsenieff et al., Citation2019) were discovered in samples of seawater filtered through a 0.22 μm filter, a protocol designed to eliminate all large cells, including diatoms and bacteria. In our study, we employed a standard protocol for total DNA extraction from the algal biomass and directly used the resulting DNA preparations to produce DNA libraries for Illumina sequencing. Obviously, in the absence of a step allowing the conversion of single-strand DNA viral genomes into double-stranded DNA templates, the former molecules were not expected to be represented in the DNA libraries. Therefore, to explain our successful recovery of complete bacilladnavirus genome sequences, we assume that double-strand DNA intermediates of these genomes, possibly replication intermediates, were present in abundance in the DNA preparations of the blue H. ostrearia strains from the Bay of Bourgneuf.
Host specificity and geographic origin
However, the approaches we used did not allow us to identify DIVs similar to HOV-148 and HOV-235 in isolates of H. ostrearia from the Leschenault Estuary and Kattegat Strait and in the newly described H. nusantara species (Prasetiya et al., Citation2019) from Indonesia. Unlike the other diatoms in which DIVs were previously documented, Haslea species are mostly benthic, sometimes tychopelagic or epiphytic, and inhabit coastal shallow waters. Viral infections are less likely to spread among species with a limited capacity for dispersal compared with planktonic/pelagic species. Importantly, HOV-148 and HOV-235 were observed among H. ostrearia strains that share the same geographic origin (oyster ponds dispersed on a 10 km coastal area) but have completely different life histories. While NCC 235.1 was a wild strain, the NCC 153.8 and NCC 148.78 clones resulted from auxosporulation events. NCC 153.8 was obtained following heterothallic (interclonal) auxosporulation, a controlled event triggered in the laboratory following a routine protocol (Davidovich et al., Citation2009; Mouget et al., Citation2009), and NCC 148.78 was derived from a bimodal population that appeared in a culture of the clone NCC 148, probably resulting from homothallic (intraclonal) auxosporulation, which is known to occur occasionally in this species (Neuville & Daste, Citation1975, Citation1979). Furthermore, it is worth noting that NCC 148.78 was sequenced 12 years after its presumed ancestor was isolated from a natural sample. Taken together, these data suggest that the HOV-148 and HOV-235 viruses have a perennial presence in oyster ponds, are transmitted during both asexual and sexual reproduction, and that under the cultivation conditions used in our laboratories, these viruses are not a threat to the cultures.
The H. ostrearia bacilladnavirus genomes are most closely related to those of the bacilladnaviruses AcrBV1 and AcrBV2, which have been found to be associated with the snail Amphibola crenata, a very peculiar pulmonate gastropod endemic in New Zealand and known for inhabiting mud-flats at the interface of sea and land (Dayaram et al., Citation2015; Kazlauskas et al., Citation2017). Also, the HOV-148 and HOV-235 genomes show significant similarities with the Avon-Heathcote estuary associated bacilladnavirus AHEaBavV1, which was identified in the same area where Amphibola crenata was sampled. Given this common geographic location, we hypothesize that A. crenata can capture viruses by grazing biofilms made of benthic diatoms harbouring bacilladnaviruses. However, considering the long distance between the Bay of Bourgneuf and the Avon-Heathcote estuary, no simple hypothesis can yet reconcile the bacilladnavirus genome similarity and host diversity found at these locations.
Perspectives
Unfortunately, H. ostrearia belongs to the kind of diatoms whose long-term conservation is made impossible because of the MacDonald–Pfitzer rule. This phenomenon, resulting from vegetative growth, leads to a progressive decrease of the average cell size of the cultures. In the absence of an auxosporulation event, when the size becomes too small (~25 µm in the case of H. ostrearia), cultures die. All the clones used in the current study, including the other species of Haslea spp. and the clones from Kattegat and Australia, died several years ago, making it impossible to perform complementary analyses on them after the discovery of HOV-148 and HOV-235. Given the serendipity of this first discovery of DIVs in blue diatoms, several questions remain to be addressed. For instance, what are their subcellular localization and host range? All single-stranded DNA diatom viruses characterized to date appear to aggregate inside the nucleus (Kimura & Tomaru Citation2013). The presence of a possible double-stranded DNA region, as demonstrated for other DIVs, should also be investigated (Kimura & Tomaru, Citation2013). The host range of HOV-148 and HOV-235, or related DIVs, could be studied by examining other diatoms from the oyster ponds of the Bay of Bourgneuf or similar environments. To determine whether these viruses can occur outside their hosts, it would be interesting to sample different compartments of the ponds (water column, superficial substrate, sediments). Because these ponds are semi-closed environments with only occasional contact with seawater, they may provide ideal systems to study the interactions between bacilladnaviruses and different genera of diatoms.
Author contributions
R. Gastineau: original concept, bioinformatic analyses, drafting; C. Lemieux: drafting and editing; M. Turmel: drafting and editing, E. Grypioti: cultivation and DNA extraction; F. Verret: cultivation and DNA extraction; A. Makris: sequencing; A. Argiriou: sequencing; D. Kafetzopoulos: sequencing; I. Stratidaki: sequencing; G. Carrier: bioinformatic analyses; B. Jacquette: cultivation, axenization and DNA extraction; Jean-Luc Mouget: drafting and editing.
Disclosure statement
No potential conflict of interest was reported by the authors.
Additional information
Funding
References
- Andrews, S. (2010). FastQC: a quality control tool for high throughput sequence data. Available online at: http://www.bioinformatics.babraham.ac.uk/projects/fastqc.
- Arsenieff, L., Simon, N., Rigaut-Jalabert, F., Le Gall, F., Chaffron, S., Corre, E., Com, E., Bigeard, E. & Baudoux, A.-C. (2019). First viruses infecting the marine diatom Guinardia delicatula. Frontiers in Microbiology, 9: 3235. doi: 10.3389/fmicb.2018.03235.
- Bankevich, A., Nurk, S., Antipov, D., Gurevich, A.A., Dvorkin, M., Kulikov, A.S., Lesin, V.M., Nikolenko, S.I., Pham, S., Prjibelski, A.D., Pyshkin, A.V., Sirotkin, A.V., Vyahhi, N., Tesler, G., Alekseyev, M.A. & Pevzner, P.A. (2012). SPAdes: a new genome assembly algorithm and its applications to single-cell sequencing. Journal of Computational Biology, 19: 455–477.
- Bettarel, Y., Kan, J., Wang, K., Williamson, K., Cooney, S., Ribblett, S., Chen, F., Wommack, K.E. & Coats D.W. (2005). Isolation and preliminary characterisation of a small nuclear inclusion virus infecting the diatom Chaetoceros cf. gracilis. Aquatic Microbial Ecology, 40: 103–114.
- Boratyn, G.M., Camacho, C., Cooper, P.S., Coulouris, G., Fong, A., Ma, N., Madden, T.L., Matten, W.T., McGinnis, S.D., Merezhuk, Y., Raytselis, Y., Sayers, E.W., Tao, T., Ye, J. & Zaretskaya, I. (2013). BLAST: a more efficient report with usability improvements. Nucleic Acids Research, 41: W29–W33. doi: 10.1093/nar/gkt282.
- Capella-Gutiérrez, S., Silla-Martínez, J.M. & Gabaldón, T. (2009). trimAl: a tool for automated alignment trimming in large-scale phylogenetic analyses. Bioinformatics, 25: 1972–1973.
- Chen, S., Huang, T., Zhou, Y., Han, Y. & Gu, J. (2017). AfterQC: automatic filtering, trimming, error removing and quality control for fastq data. BMC Bioinformatics, 18: 80. doi: 10.1186/s12859-017-1469-3.
- Crooks, G.E., Hon, G., Chandonia, J.M. & Brenner, S.E. (2004). WebLogo: a sequence logo generator. Genome Research, 14: 1188–1190.
- Darling, A.E., Mau, B. & Perna, N.T. (2010). ProgressiveMauve: multiple genome alignment with gene gain, loss, and rearrangement. PLoS ONE, 5(6): e11147.
- Davidovich, N.A., Mouget, J.-L. & Gaudin, P. (2009). Heterothallism in the pennate diatom Haslea ostrearia (Bacillariophyta). European Journal Phycology, 44: 251–261.
- Dayaram, A., Goldstien, S., Argüello-Astorga, G.R., Zawar-Reza, P., Gomez, C., Harding, J.S. & Varsani, A. (2015). Diverse small circular DNA viruses circulating amongst estuarine molluscs. Infection, Genetics and Evolution, 31: 284–295.
- Dimmic, M.W., Rest, J.S., Mindell, D.P. & Goldstein, R.A. (2002). rtREV: an amino acid substitution matrix for inference of retrovirus and reverse transcriptase phylogeny. Journal of Molecular Evolution, 55: 65–73.
- Doyle, J.J. & Doyle, J.L. (1990). Isolation of plant DNA from fresh tissue. Focus, 12: 13–15.
- Eissler, Y., Wang, K., Chen, F., Wommack, E. & Coats, W. (2009). Ultrastructural characterization of the lytic cycle of an intranuclear virus infecting the diatom Chaetoceros cf. wighamii (bacillariophyceae) from Chesapeake Bay, USA. Journal of Phycology, 45: 787–797.
- Falaise, C., Cormier, P., Tremblay, R., Audet, C., Deschênes, J.S., Turcotte, F., François, C., Seger, A., Hallegraeff, G., Lindquist, N., Sirjacobs, D., Gobert, S., Lejeune, P., Demoulin, V. & Mouget, J.L. (2019a). Harmful or harmless: biological effects of marennine on marine organisms. Aquatic Toxicology, 209: 13–25.
- Falaise, C., James, A., Travers, M.-A., Zanella, M., Badawi, M. & Mouget, J.-L. (2019b). Complex relationships between the blue pigment marennine and marine bacteria of the genus Vibrio. Marine Drugs, 17: 160. doi: 10.3390/md17030160.
- Gasteiger, E., Gattiker, A., Hoogland, C., Ivanyi, I., Appel, R.D. & Bairoch, A. (2003). ExPASy: the proteomics server for in-depth protein knowledge and analysis. Nucleic Acids Research, 31: 3784–3788.
- Gastineau, R., Davidovich, N.A., Bardeau, J.-F., Caruso, A., Leignel, V., Hardivillier, Y., Jacquette, B., Davidovich, O., Rincé, Y., Gaudin, P., Cox, E. & Mouget, J.-L. (2012a). Haslea karadagensis (Bacillariophyta): a second blue diatom, recorded from the Black Sea and producing a novel blue pigment. European Journal of Phycology, 47: 469–479.
- Gastineau, R., Pouvreau, J.-B., Hellio, C., Morançais, M., Fleurence, J., Gaudin, P., Bourgougnon, N. & Mouget, J.-L. (2012b). Biological activities of purified marennine, the blue pigment responsible for the greening of oysters. Journal of Agricultural and Food Chemistry, 60: 3599–3605.
- Gastineau, R., Leignel, V., Jacquette, B., Bendahmane, D., Hardivillier, Y., Wulff, A., Gaudin P., Kaczmarska, I., Davidovich, N.A. & Mouget, J.-L. (2013). Inheritance of mitochondrial DNA in the pennate diatom Haslea ostrearia (Naviculaceae) during auxosporulation suggests a uniparental transmission. Protist, 164: 340–351.
- Gastineau, R., Davidovich, N.A., Hansen, G., Rines, J., Wulff, A., Kaczmarska, I., Ehrman, J., Hermann, D., Maumus, F., Hardivillier, Y., Leignel, V., Jacquette, B., Méléder, V., Hallegraeff, G., Yallop, M., Perkins, R., Cadoret, J.-P., Saint-Jean, B., Carrier, G. & Mouget, J.-L. (2014a). Haslea ostrearia-like diatoms: biodiversity out of the blue. Advances in Botanical Research, 71: 441–446.
- Gastineau, R., Turcotte, F., Pouvreau, J.-B., Morançais, M., Fleurence, J., Windarto, E., Semba Prasetiya, F., Arsad, S., Jaouen, P., Babin, M., Coiffard, L., Couteau, C., Bardeau, J.-F., Jacquette, B., Leignel, V., Hardivillier, Y., Marcotte, I., Bourgougnon, N., Tremblay, R., Deschênes, J.-S., Badawy, H., Pasetto, P., Davidovich, N.A., Hansen, G., Dittmer, J. & Mouget, J.-L. (2014b). Marennine, promising blue pigments from a widespread Haslea diatom species complex. Marine Drugs, 12: 3161–3189.
- Gastineau, R., Hansen, G., Davidovich, N.A., Davidovich, O., Bardeau, J.-F., Kaczmarska, I., Ehrman, J. M., Leignel, V., Hardivillier, Y., Jacquette, B., Poulin, M., Morançais, M., Fleurence, J. & Mouget, J.-L. (2016). A new blue-pigmented hasleoid diatom, Haslea provincialis, from the Mediterranean Sea. European Journal of Phycology, 51: 156–170.
- Gordon, D., Abajian, C. & Green, P. (1998). Consed: a graphical tool for sequence finishing. Genome Research, 3: 195–202.
- Greiner, S., Lehwark, P. & Bock, R. (2019). OrganellarGenomeDRAW (OGDRAW) version 1.3.1: expanded toolkit for the graphical visualization of organellar genomes. Nucleic Acids Research, 47: W59–W64. doi: 10.1093/nar/gkz238.
- Guillard, R.R.L. (1975). Culture of phytoplankton for feeding marine invertebrates. In Culture of Marine Invertebrate Animals (Smith, W.L. & Chanley, M.H., editors), 26–60. Plenum Press, New York.
- Kadono, T., Miyagawa-Yamaguchi, A., Kira, N., Tomaru, Y., Okami, T., Yoshimatsu, T., Hou, L., Ohama, T., Fukunaga, K., Okauchi, M., Yamaguchi, H., Ohnishi, K., Falciatore, A. & Adachi, M. (2015). Characterization of marine diatom-infecting virus promoters in the model diatom Phaeodactylum tricornutum. Scientific Reports, 5: 18708. http://doi.org/10.1038/srep18708.
- Katoh, K. & Standley, D.M. (2016). A simple method to control over-alignment in the MAFFT multiple sequence alignment program. Bioinformatics, 32: 1933–1942.
- Kazlauskas, D., Dayaram, A., Kraberger, S., Goldstien, S., Varsani, A. & Krupovic, M. (2017). Evolutionary history of ssDNA bacilladnaviruses features horizontal acquisition of the capsid gene from ssRNA nodaviruses. Virology, 504: 114–121.
- Kimura, K. & Tomaru, Y. (2013). Isolation and characterization of a single-stranded DNA virus infecting the marine diatom Chaetoceros sp. strain SS628-11 isolated from Western Japan. PLoS ONE 8(12): e82013. doi: 10.1371/journal.pone.0082013.
- McDaniel, L.D., Rosario, K., Breitbart, M. & Paul, J.H. (2014). Comparative metagenomics: natural populations of induced prophages demonstrate highly unique, lower diversity viral sequences. Environmental Microbiology, 16: 570–585.
- Miranda, J.A., Culley, A.I., Schvarcz, C.R. & Steward, G.F. (2016). RNA viruses as major contributors to Antarctic virioplankton. Environmental Microbiology, 18: 3714–3727.
- Mouget, J.-L., Gastineau, R., Davidovich, O., Gaudin, P. & Davidovich, N.A. (2009). Light is a key factor in triggering sexual reproduction in the pennate diatom Haslea ostrearia. FEMS Microbiology Ecology, 69: 194–201.
- Nagasaki, K., Tomaru, Y., Katanozaka, N., Shirai, Y., Nishida, K., Itakura, S. & Yamaguchi, M. (2004). Isolation and characterization of a novel single-stranded RNA virus infecting the bloom-forming diatom Rhizosolenia setigera. Applied and Environmental Microbiology, 70: 704–711.
- Nagasaki, K., Tomaru, Y., Takao, Y., Nishida, K., Shirai, Y., Suzuki, H. & Nagumo, T. (2005). Previously unknown virus infects marine diatom. Applied and Environmental Microbiology, 71: 3528–3535.
- Neuville, D. & Daste, P. (1975). Observations préliminaires concernant l’auxosporulation chez la diatomée Navicula ostrearia (Gaillon) Bory en culture in vitro. Comptes Rendus de l’Académie des Sciences de Paris, 281: 1753–1756.
- Neuville, D. & Daste, P. (1979). Observations concernant les phases de l’auxosporulation chez la diatomée Navicula ostrearia (Gaillon) Bory en culture in vitro. Comptes Rendus de l’Académie des Sciences de Paris, 288: 1496–1498.
- Perkins, R.G., Mouget, J.-L., Lefebvre, S. & Lavaud, J. (2006). Light response curve methodology and possible implications in the application of chlorophyll fluorescence to benthic diatoms. Marine Biology, 149: 703–712.
- Poulin, M., Méléder, V. & Mouget, J.-L. (2019). Typification of the first recognized blue pigmented diatom, Haslea ostrearia (Bacillariophyceae). Plant Ecology and Evolution, 152: 402–408.
- Pouvreau, J.B., Housson, E., Le Tallec, L., Morançais, M., Rincé, Y., Fleurence, J. & Pondaven, P. (2007). Growth inhibition of several marine diatom species induced by the shading effect and allelopathic activity of marennine, a blue-green polyphenolic pigment of the diatom Haslea ostrearia (Gaillon/Bory) Simonsen. Journal of Experimental Marine Biology and Ecology, 352: 212–225.
- Pouvreau, J.B., Morançais, M., Taran, F., Rosa, P., Dufossé, L., Guérard, F., Pin, S., Fleurence, J. & Pondaven, P. (2008). Antioxidant and free radical scavenging properties of marennine, a blue-green polyphenolic pigment from the diatom Haslea ostrearia (Gaillon/Bory) Simonsen responsible for the natural greening of cultured oysters. Journal of Agricultural and Food Chemistry, 56: 6278–6286.
- Prasetiya, F.S., Comeau, L.A., Gastineau, R., Decottignies, P., Cognie, B., Morançais, M., Turcotte, F., Mouget, J.-L. & Tremblay, R. (2017). Effect of marennine produced by the blue diatom Haslea ostrearia on behavioral, physiological and biochemical traits of juvenile Mytilus edulis and Crassostrea virginica. Aquaculture, 467: 138–148.
- Prasetiya, F., Gastineau, R., Poulin, M., Lemieux, C., Turmel, M., Syakti, A., Hardivillier, Y., Widowati, I., Risjani, Y., Iskandar, I., Subroto, T., Falaise, C., Arsad, S., Safitri, I., Mouget, J.-L. & Leignel, V. (2019). Haslea nusantara (Bacillariophyceae), a new blue diatom from the Java Sea, Indonesia: morphology, biometry and molecular characterization. Plant Ecology and Evolution, 152: 188–202.
- Rice, P., Longden, I. & Bleasby, A. (2000). EMBOSS: The European Molecular Biology Open Software Suite. Trends in Genetics, 16: 276–277.
- Shirai, Y., Tomaru, Y., Takao, Y., Suzuki, H., Nagumo, T. & Nagasaki, K. (2008). Isolation and characterization of a single-stranded RNA virus infecting the marine planktonic diatom Chaetoceros tenuissimus Meunier. Applied and Environmental Microbiology, 74: 4022–4027.
- Stamatakis, A. (2014). RAxML Version 8: a tool for phylogenetic analysis and post-analysis of large phylogenies. Bioinformatics, 30: 1312–1313.
- Tomaru, Y., Shirai, Y., Suzuki, H., Nagumo, T. & Nagasaki, K. (2008). Isolation and characterization of a new single-stranded DNA virus infecting the cosmopolitan marine diatom Chaetoceros debilis. Aquatic Microbial Ecology, 50: 103–112.
- Tomaru, Y., Takao, Y., Suzuki, H., Nagumo, T. & Nagasaki, K. (2009). Isolation and characterization of a single-stranded RNA virus infecting the bloom-forming diatom Chaetoceros socialis. Applied and Environmental Microbiology, 75: 2375–2381.
- Tomaru, Y., Fujii, N., Oda, S., Toyoda, K. & Nagasaki, K. (2011a). Dynamics of diatom viruses on the western coast of Japan. Aquatic Microbial Ecology, 63: 223–230.
- Tomaru, Y., Shirai, Y., Toyoda, K. & Nagasaki, K. (2011b). Isolation and characterisation of a single- stranded DNA virus infecting the marine planktonic diatom Chaetoceros tenuissimus. Aquatic Microbial Ecology, 64: 175–184.
- Tomaru, Y., Takao, Y., Suzuki, H., Nagumo, T., Koike, K. & Nagasaki, K. (2011c). Isolation and characterization of a single-stranded DNA virus infecting Chaetoceros lorenzianus Grunow. Applied and Environmental Microbiology, 77: 5285–5293.
- Tomaru, Y., Toyoda, K., Kimura, K., Hata, N., Yoshida, M. & Nagasaki, K. (2012). First evidence for the existence of pennate diatom viruses. ISME Journal, 6: 1445–1448.
- Tomaru, Y., Toyoda, K., Kimura, K., Takao, Y., Sakurada, K., Nakayama, N. & Nagasaki, K. (2013). Isolation and characterization of a single-stranded RNA virus that infects the marine planktonic diatom Chaetoceros sp. (SS08-C03). Phycological Research, 61: 27–36.
- Toyoda, K., Kimura, K., Hata, N., Nakayama, N., Nagasaki, K. & Tomaru, Y. (2012). Isolation and characterization of a single-stranded DNA virus infecting the marine planktonic diatom Chaetoceros sp. (strain TG07-C28). Plankton and Benthos Research, 7: 20–28.
- Turcotte, F., Mouget, J.-L., Genard, B., Lemarchand, K., Deschênes, J.-S. & Tremblay, R. (2016). Prophylactic effect of Haslea ostrearia culture supernatant containing the pigment marennine to stabilize bivalve hatchery production. Aquatic Living Resources, 29: 401–409.