ABSTRACT
A molecular and morphological taxonomic study of Corallina (Corallinales, Rhodophyta) from Tristan da Cunha and the Falkland Islands revealed Corallina chamberlainiae J.Brodie & R.Mrowicki sp. nov. from both South Atlantic archipelagos, and Corallina cf. caespitosa only in the Falkland Islands. Analysis of mitochondrial COI-5P and plastid psbA resolved C. chamberlainiae as a distinct clade composed of specimens from Tristan and the Falkland Islands (COI-5P), in addition to two matching New Zealand samples (psbA). In psbA analyses, C. cf. caespitosa was close to two species from Japan (as C. pilulifera and C. melobesioides) but was separate from C. caespitosa sensu stricto from Britain and C. ferreyrae (isotype) from Peru. In COI-5P analyses, C. cf. caespitosa was in a clade with C. caespitosa (holotype) and close to, but distinct from, C. ferreyrae and an unidentified South African Corallina species. Application of the Automatic Barcode Gap Discovery (ABGD) and Poisson tree processes (bPTP) for COI-5P lumped C. chamberlainiae into one clade, whereas the Generalized Mixed Yule Coalescent (GMYC) model split it into two well-supported groups, one of which only contained Falkland Island specimens. All three models lumped C. cf. caespitosa with C. caespitosa. C. chamberlainiae closely resembles C. officinalis, the generitype, but is distinguished by smaller size and a more compressed thallus towards the apex. Corallina cf. caespitosa resembles C. caespitosa but is smaller and has fused, palm-like upper fronds. Neither of these taxa is conspecific with C. ferreyrae although C. caespitosa was recently synonymized with C. ferreyrae. C. chamberlainiae and C. cf. caespitosa occupy similar intertidal habitats to their respective, closely related counterparts C. officinalis and C. caespitosa. The results indicate cryptic diversity and suggest that there are many misidentified Corallina species. The paucity of South Atlantic studies of the Corallinales points to the need for a much greater taxonomic effort in this biogeographic region.
Introduction
Corallina is a genus of geniculate calcified red algae that occur on shores and in the shallow subtidal in temperate regions of the world (Brodie et al., Citation2013). The genus is a member of the Corallinales, in the Corallinophycidae, a large subclass of calcified red algae whose taxa are particularly important as autogenic ecosystem engineers in shallow water of temperate regions (Johansen, Citation1981; Jones et al., Citation1994; Nelson, Citation2009). The physical structure of Corallina species provides a habitat for many small invertebrates (Williamson et al., Citation2014), making these species a valuable component for diversity in shallow marine environments.
Identification of geniculate coralline algae is highly problematic and it is well-established that there is considerable cryptic diversity within Corallina (e.g. Hind & Saunders, Citation2013; Williamson et al., Citation2015), with many species misidentified or different species going under the same name in different parts of the world. As with many other groups of red algae, a complex picture of genetic diversity and cryptic species is emerging using molecular taxonomic approaches (e.g. Walker et al., Citation2009). The genus Corallina is well-defined, the type C. officinalis Linnaeus having been epitypified and redescribed by Brodie et al. (Citation2013). There are 204 species names and 68 infraspecific names listed for Corallina, of which 28 are currently accepted (Guiry & Guiry, Citation2020). There has been an increasing drive in recent years to revise the taxonomy of this genus, notably in the north-eastern Atlantic (Walker et al., Citation2009; Brodie et al., Citation2013; Pardo et al., Citation2015; Williamson et al., Citation2015), north-western Atlantic (Hind & Saunders Citation2013), north-eastern Pacific and New Zealand (Harvey et al., Citation2005; Broom et al., Citation2008; Farr et al., Citation2009; Nelson et al., 2015; Twist et al., Citation2019). One geographic area where the coralline algae have received little or no attention is the South Atlantic. In this study, we focus on two island archipelagos, Tristan da Cunha and the Falkland Islands.
Tristan da Cunha is a group of volcanic islands consisting of three ‘top’ islands, Tristan da Cunha, Nightingale and Inaccessible, and Gough Island, 380 km away to the south-southeast. These are the only isolated islands in temperate waters in the South Atlantic (Scott, Citation2017). Between November 1955 and May 1956, Nigel M. Wace made a collection of marine algae from Gough Island as part of the Gough Island Scientific Survey (Chamberlain, Citation1965). This collection, deposited in the Natural History Museum algal herbarium (BM), included material of geniculate coralline algae that was studied by Yvonne Chamberlain (Citation1965). She assigned two species to Corallina, one of which she identified as C. officinalis, although she noted that there were morphologically similar specimens in the BM herbarium identified as C. officinalis, C. cuvieri and C. chilensis, and that the distinction between these and species such as C. vancouveriensis was not clear. She decided that the other species was new and described it as C. goughensis Y.M.Chamberlain (Citation1965, p. 2013). In 1972, Bill Johansen examined the type material of C. goughensis (BM000569792) and concluded that it was a species of Arthrocardia (the type herbarium sheet is annotated ‘= Arthrocardia sp. HWJ VII 72’). We have recently obtained psbA sequence data from the type material of C. goughensis which confirms that it is a species of Arthrocardia. The sequence is similar but not identical to A. corymbosa from South Africa (GenBank code JQ917408.1).
Between 2005 and 2014, new collections of marine algae were made from the Tristan da Cunha archipelago as part of projects on the underwater life of Gough Island (Scott & Holt, Citation2016) and the Tristan da Cunha archipelago (Scott, Citation2017). The coralline algae were subsequently sent to the BM for molecular analysis and the resulting Corallina data incorporated into this study.
Despite almost 200 years of seaweed collections from the Falkland Islands (the earliest specimen in the BM is from 1822), until this study, there were only seven specimens of Corallinaceae from these islands in the BM herbarium, and no research on Falklands coralline algae has been published. The first major collections of coralline algae were made during expeditions in 2018 and 2019 by Brodie and Mrowicki. The aim of this study was to undertake a molecular taxonomic study of the genus Corallina from Tristan da Cunha and the Falklands.
Materials and methods
Collections
Specimens were collected from the intertidal and shallow sublittoral zones at sites throughout the Falkland Islands (January–February 2018 and February 2019) and the Tristan da Cunha archipelago (between 2004 and 2014; Supplementary table 1). Field-collected samples were air-dried or pressed and dried onto herbarium sheets. Additional specimens from the BM algal herbarium were included in analyses and all material is now housed in this herbarium.
DNA extraction, amplification and sequencing
Extractions were performed on the BioSprint® 96 platform using the BioSprint® 96 DNA Plant Kit (Qiagen, Hilden, Germany) following the manufacturer’s instructions. Each sample (~0.1 cm3 with no visible epiphytes) was first disrupted with a 3 mm tungsten carbide ball using a TissueLyser II (Qiagen) at 30 Hz. Two markers were amplified: (1) the 664 bp 5’ barcode region of the mitochondrial cytochrome c oxidase subunit 1 (COI-5P), using primers GazF1 and GazR1 (Saunders, Citation2005); and (2) a ~950 bp segment of the plastid Photosystem II thylakoid membrane protein D1 (psbA), using primers psbAF1 and psbAR2 (Yoon et al., Citation2002).
Each PCR amplification (total volume 25 μl) contained 2.5 μl 10× NH4 reaction buffer, 1.5 μl 50 mM MgCl2, 0.5 μl BIOTAQTM DNA polymerase (all from BIOTAQTM DNA Polymerase kit, Bioline Reagents Ltd, UK), 0.5 μl 100 mM dNTP, 1 μl each of forward and reverse primer (10 μM), 17 μl nuclease free water and 1 μl template DNA. Amplification profiles for COI-5P and psbA followed Melbourne et al. (Citation2017) and were achieved using a Techne Prime Elite thermal cycler (Cole-Parmer Ltd, UK). Samples for which amplification was initially unsuccessful were purified using the Illustra GFX DNA PCR and Gel Band Purification Kit (GE Healthcare, UK) following the manufacturer’s protocol.
Prior to sequencing, samples were cleaned using Axygen PCR purification beads (ThermoFisher, UK). Dideoxy cycle sequencing was performed on the samples using Big Dye v1.1 (Life Technologies, UK) containing 2 ng 100 bp–1 of amplicon size and 1 μM primer in 10 μl volume. Amplification was performed using the Techne Thermo cycler (28 cycles; 10 s at 96°C, 5 s at 50°C and 4 min at 60°C). Excess dye labelled nucleotides from the sequencing reaction were removed with ethanol-sodium acetate precipitation and sequenced products were dried, resuspended and run on a 96-capillary 3730xl DNA Analyzer (Applied Biosystems, Fischer Scientific, UK).
Data analyses
Forward and reverse sequences were aligned, edited and trimmed using Unipro UGENE (v1.32; Okonechnikov et al., Citation2012). Additional sequences were obtained from GenBank and added to COI-5P and psbA datasets, including related sequences retrieved via BLAST and two outgroup sequences per dataset. Both outgroup taxa were selected from the order Corallinales: Lithothamnion glaciale, a maerl-forming species in the family Lithothamniaceae, and Ellisolandia elongata, a geniculate species in the same tribe (Corallineae) as the genus Corallina, within the Corallinaceae. COI-5P (n = 40) and psbA (n = 46) sequences were aligned separately using MAFFT (v7.427; Katoh & Standley, Citation2013) via the L-INS-i algorithm. Prior to phylogenetic analyses, regions of poor alignment and sites containing gaps were removed from each alignment using Gblocks (v0.91b; Castresana, Citation2000), resulting in 533 and 787 sites for COI-5P and psbA, respectively. Analyses were performed on both alignments separately, in addition to a concatenated alignment (COI-5P+psbA) including 32 sequences (total 1320 sites). Best-fit partitioning schemes and corresponding nucleotide substitution models, based on the corrected Akaike Information Criterion (AICc), were determined for all alignments using PartitionFinder (v2.1.1; Lanfear et al., Citation2016; Supplementary table 2).
Phylogenetic relationships were inferred based on Bayesian and Maximum likelihood optimality criteria, using MrBayes (v3.2.6; Ronquist et al., Citation2012) and RAxML-NG (v0.9.0; Kozlov et al., Citation2019), respectively. Bayesian analysis was performed using two runs of four Markov chains over 5 million generations, sampling every 100 generations, with the first 25% of trees discarded as burn-in. Convergence was diagnosed using the average standard deviation of split frequencies (ASDSF; <0.01) in addition to potential scale reduction factors (PSRF; ≈1) and estimated sample sizes (ESS; >200) for all parameters, and by examining parameter log-likelihood traces using Tracer (v1.7.1; Rambaut et al., Citation2018). Maximum likelihood analysis involved 100 tree searches using 50 random and 50 parsimony-based starting trees, followed by non-parametric bootstrapping with 5000 replicates to generate branch support values for the best-scoring tree.
Species delimitation
Given the conservative nature of the psbA gene (Nelson et al., Citation2015), species boundaries were assessed using the single-gene COI-5P sequence alignment only. Three species delimitation tools were applied: Automatic Barcode Gap Discovery (ABGD; Puillandre et al., Citation2012), which sorts individuals into hypothetical species based on the ‘barcode gap’ between intraspecific and interspecific pairwise differences; the Generalized Mixed Yule Coalescent method (GMYC; Fujisawa & Barraclough, Citation2013), which defines the transition between inter- and intraspecific processes using a time-calibrated, ultrametric phylogenetic tree; and the Poisson tree processes model (PTP; Zhang et al., Citation2013), which also delimits putative species based on a phylogenetic tree, but relies on the number of substitutions rather than time to identify transitions in branching rate.
ABGD was performed on pairwise COI-5P distances, calculated using the Kimura two-parameter (K2P) model (Kimura, Citation1980), via the ABGD website (https://bioinfo.mnhn.fr/abi/public/abgd/) with default parameters, including relative gap width (X) set to 1.5.
Prior to GMYC, a COI-5P ultrametric Bayesian tree was constructed in BEAST2 (v2.6.2; Bouckaert et al., Citation2019) under a strict clock model based on a constant Yule speciation tree prior, following Melbourne et al. (Citation2017), with TIM+I+G site models applied to both partitions separately (Supplementary table 2) via the package ‘SSM’ (v1.1.0). Using the package ‘CoupledMCMC’ (v1.0.1; Müller & Bouckaert, Citation2020), Metropolis coupled Markov chain Monte Carlo analyses were performed for two runs of four chains over 10 million generations, sampling every 100 generations, with 25% burn-in. Convergence was diagnosed by examining parameter trace plots and ESS values in Tracer v1.7.1, in addition to ASDSF and PSRF values calculated using the packages ‘RWTY’ (v1.0.2; Warren et al., Citation2017) and ‘coda’ (v0.19.3; Plummer et al., Citation2006) in R (v3.6.1; R Core Team, Citation2019), following the same criteria for the main Bayesian phylogenetic analyses. Post-burn-in trees were summarized using TreeAnnotator v2.6.2, targeting the maximum clade credibility tree and keeping target node heights, with a posterior probability limit of zero, and a single-threshold GMYC analysis was performed on the resulting tree using the R package ‘splits’ (v1.0-19; Ezard et al., Citation2017).
The bPTP server (https://species.h-its.org/ptp/) was used to run a Bayesian implementation of the PTP analysis on the COI-5P consensus Bayesian tree, with 500 000 generations and 25% burn-in, and convergence was diagnosed by examining the resulting likelihood trace plot.
Morphology
Sporangial conceptacles and overall cell morphology were observed in three histologically prepared specimens that had been decalcified in 10% nitric acid. The small sample size was due to the paucity of material with reproductive structures. All images (conceptacles and genicula) were made using the Zeiss Axioskop2 using Axio Vs40 V 4.8.2.4. Measurements were recorded using ImageJ analysis software (Rasband, Citation1997–Citation2018).
Results
Molecular phylogenetic analysis
Analysis of COI-5P and psbA sequence data of specimens from Tristan da Cunha and the Falkland Islands revealed two Corallina species, Corallina chamberlainiae J.Brodie & R.Mrowicki sp. nov. and C. cf. caespitosa. C. chamberlainiae formed a well-supported clade in both gene analyses, including samples from Tristan, Nightingale and Gough Islands, and from both East and West Falkland (). C. cf. caespitosa formed a discrete clade in analyses of both gene regions and was only found in the Falkland Islands
Fig. 1. Distribution maps of Corallina chamberlainiae and C. cf. caespitosa specimens collected from Tristan da Cunha (a–e) and the Falkland Islands (e). Points on the maps only include those for which there are specific locations or precise coordinates. Less localized distribution data are given in Supplementary table 1. The dashed line represents the boundary of the Tristan da Cunha Exclusive Economic Zone
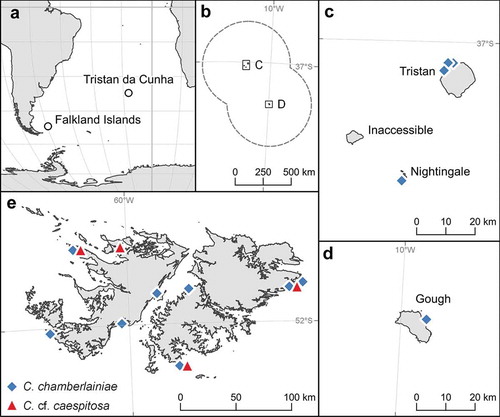
Fig. 2. Bayesian phylogenetic tree inferred for COI-5P sequence data. Node values indicate Maximum likelihood bootstrap support values/Bayesian posterior probabilities (‘−’ denotes < 50% support). Alternating shades correspond to species groups inferred by delimitation analyses (ABGD, Automatic Barcode Gap Discovery; GMYC, Generalized Mixed Yule Coalescent; bPTP, Bayesian implementation of Poisson tree processes). Scalebar represents number of substitutions per site
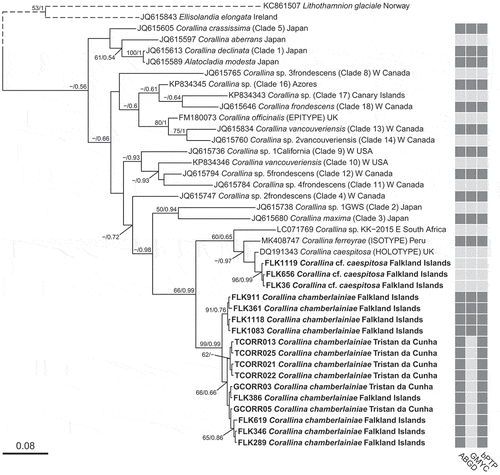
Fig. 3. Bayesian phylogenetic tree inferred for psbA sequence data. Node values indicate Maximum likelihood bootstrap support values/Bayesian posterior probabilities (‘−’ denotes < 50% support). Scalebar represents number of substitutions per site
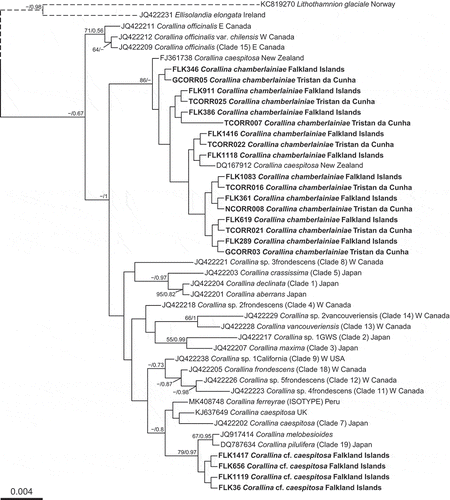
Analysis of COI-5P showed very little sequence variation for C. chamberlainiae (maximum pairwise difference 6 bp), and although there appeared to be some substructuring, this was not supported, with the possible exception of a weak clade of four Falkland Island specimens (FLK911, FLK361, FLK1118 and FLK1083; ) which were from slightly less exposed shores than the other FLK samples. The COI-5P sequences did not match any other samples in GenBank. The C. chamberlainiae clade was sister to C. cf. caespitosa from the Falkland Islands. The psbA tree () revealed a well-resolved clade (maximum within-clade pairwise difference 5 bp) containing C. chamberlainiae samples from Tristan, Gough and Nightingale in addition to sequences of two specimens from New Zealand identified as C. caespitosa, one from the North Island (GenBank sequence FJ361738), and the other from the Chatham Islands (DQ167912). The clade was sister to C. officinalis. The divergence between C. chamberlainiae and C. cf. caespitosa clades, in terms of absolute bp difference, was much lower for psbA (8–12 bp) than for COI-5P (27–30 bp).
Analysis of COI-5P () revealed a well-supported clade for C. cf. caespitosa that was closely related to the holotype of C. caespitosa (UK BM000804549), the isotype of C. ferreyrae (Peru) and an unidentified specimen from South Africa. In the psbA results, C. cf. caespitosa was resolved in a discrete subclade in a clade with specimens from Japan identified as C. melobesioides and C. pilulifera (the latter clade 19 of Williamson et al., Citation2015). The clade was related to C. caespitosa (UK), the isotype of C. ferreyrae (Peru) and C. caespitosa Japan (Clade 7 of Williamson et al., Citation2015). It also matched a sequence of Corallina pilulifera from Japan.
Species delimitation
For COI-5P, the three species delimitation models produced almost identical results, although ABGD (prior intraspecific divergence 0.002–0.007) and bPTP were slightly more conservative than GMYC for the two species. For C. chamberlainiae, ABGD and bPTP lumped all sequences into one species whereas GMYC split it into two well-resolved sub-clades, whereby the four specimens from slightly less exposed shores in the Falkland Islands were split from the remaining specimens (Supplementary fig. 1). For C. cf. caespitosa, all delimitation models lumped this species with the holotype of C. caespitosa.
Corallina chamberlainiae J.Brodie & R.Mrowicki sp. nov. ()
Diagnosis: Base crustose; stiff unbranched stipe; branching dense, simple to compound pinnate, more complex towards the tip; intergenicula generally longer than broad, cylindrical at frond base, becoming compressed and wedge-shaped towards the apex, lateral branches of intergenicula more cylindrical in shape; terminal intergenicula with 3 tips (occasionally 4 or more), often flattened and fused. Tetrasporangial conceptacles axial, 400–500 µm in length and 350–450 µm width. Bisporangial conceptacles axial, 400–450 µm in length and 200–300 µm width. Spermatangial and carposporangial conceptacles were not observed. COI-5P/psbA GenBank sequences (MT592005/MT592006).
HOLOTYPE: Gough Island, Tristan da Cunha, coll. Sue Scott, 28 Jan 2014, GCORR03, BM013844023, deposited in the Natural History Museum, London, UK (BM) ().
Figs 4–6. Corallina chamberlainiae from Tristan da Cunha and the Falkland Islands. Fig. 4. Holotype, GCORR03, from Gough Island, Tristan da Cunha, collected by Sue Scott, 28.1.2014. Fig. 5. Specimen of C. chamberlainiae from the Falkland Islands, FLK386, collected from Cape Orford, West Falkland, 291.2018, J. Brodie and R. Mrowicki. Fig. 6. Specimen of C. chamberlainiae from the Falkland Islands, FLK289, collected from Shag Cove, West Falkland, 27.i.2018, J. Brodie and R. Mrowicki
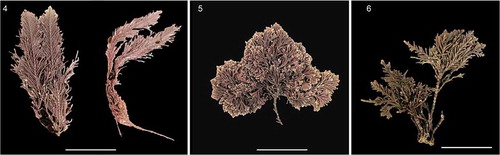
Figs 7–11. Corallina chamberlainiae vegetative and reproductive morphology. Figs 7-10. Holotype material, Fig. 11. BMTCORR025 from top islands (unspecified location), Tristan da Cunha. Fig. 7. Part of holotype decalcified for morphology. Fig. 8. Decalcified upper thallus of holotype to show arrangement of growing tips; Fig. 9. Reproductive conceptacle with putative sporangia. Fig. 10. Close up of developing sporangia in conceptacle. Fig. 11. Inset to show broad, flattened appearance of growing tips common in some specimens
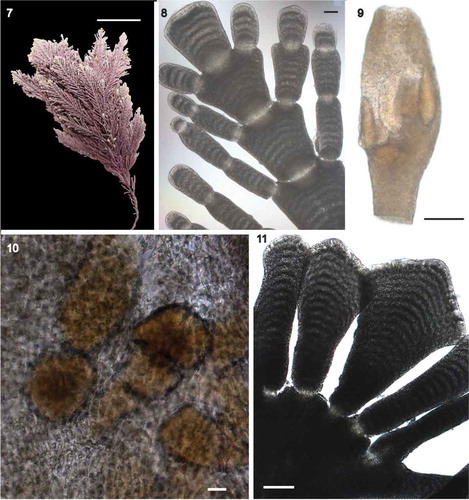
TYPE LOCALITY: Gough Island, Tristan da Cunha: site GL04, Penguin Island Shore.
ETYMOLOGY: The specific epithet is named after Yvonne Chamberlain (1933–2017), a specialist in the taxonomy of the Corallinales, who studied the seaweeds collected from Gough Island by Nigel M. Wace (Chamberlain, Citation1965).
HABITAT: Marine, growing attached to rock or other crustose coralline algae on the lower shore and sublittoral fringe, on vertical rocks, along the edge of channels and runnels, in rock pools just below the water line, and on upward-facing rock in the shallow subtidal.
DISTRIBUTION: South Atlantic, recorded from Tristan da Cunha (including the main island of Tristan, Nightingale Island and Gough Island), the Falkland Islands (East and West Falkland) and New Zealand (Ahipara, North Island and Wharekauri, Chatham Islands).
Description
Vegetative and reproductive morphology. Thallus with a firmly attached crustose basal disc bearing tufts of branched stiff, usually erect fronds. Unbranched or rarely branched below to give a distinctive ‘stalked’ appearance, densely branched above; simple to compound pinnate, more complex towards tip; lateral intergenicula branches more cylindrical in shape; intergenicula generally longer than broad, cylindrical at frond base, becoming compressed, and wedge-shaped towards the frond apex; terminal intergenicula with 3 tips (occasionally 4 or more), often fused. Only tetrasporangial and bisporangial conceptacles observed. For morphological dimensions, see .
Comparison of Corallina chamberlainiae sp. nov. with C. officinalis Linnaeus, the type of the genus and C. officinalis from Gough Island, Tristan da Cunha
Corallina chamberlainiae superficially resembles C. officinalis Linnaeus but all vegetative dimensions are smaller () and intergenicula are typically much more compressed towards the apex. Branching is in one plane, often giving a fan-shaped appearance, whereas C. officinalis typically grows in more than one plane and can become very irregular and straggly. Tetrasporangia in C. chamberlainiae are similar or slightly longer and wider than in C. officinalis. Colour and habitat type are similar in both species. C. chamberlainiae compares well to the vegetative description of C. officinalis from Gough Island ().
Comparison of Corallina cf. caespitosa with C. caespitosa R.H.Walker, J.Brodie & L.M.Irvine and C. ferreyrae from Peru
Corallina cf. caespitosa () has shorter fronds than C. caespitosa, although denser branching towards the apex gives a flabellate (fan-shaped) appearance in both species ()
Table 1. Comparison of morphological, reproductive characters and habitat between Corallina chamberlainiae sp. nov. from Tristan da Cunha and the Falkland Islands (this study), C. officinalis Linnaeus (Brodie et al. Citation2013), C. officinalis Gough Island, Tristan da Cunha (Chamberlain, Citation1965), C. caespitosa as described from Britain (modified from Walker et al. (Citation2009), C. ferreyrae from Peru (as ferreyrai, Dawson et al., Citation1964) and C. cf. caespitosa from the Falkland Islands (this study)
Figs 12–16. Corallina cf. caespitosa vegetative morphology. Fig. 12. Gross morphology, FLK36 Sealer Cove, Saunders Island, Falkland Islands, collected on 20.i.2018; Fig 13–16. FLK656 Penguin Point (beach), West Falkland, 01.ii.2018. Fig. 13. Gross morphology; Figs 14–16. Decalcified upper thallus to show variation and compressed nature
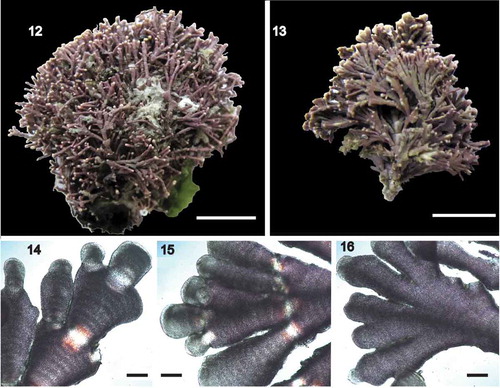
Discussion
This is the first detailed study of geniculate coralline algae from the Falkland Islands, in combination with samples from Tristan da Cunha. The use of robust molecular-assisted methods has revealed the presence of a new species, Corallina chamberlainiae J.Brodie & R.Mrowicki, which is widespread throughout both the Falkland Islands and Tristan da Cunha, where it is a habitat-forming species on rocky shores. The results confirm Chamberlain’s (Citation1965) suspicions that C. officinalis was the incorrect identification for this species in Tristan da Cunha. The results also reveal the presence of a second species, C. cf. caespitosa from the Falklands which, although it is closely related to several other species (including C. caespitosa), on further investigation may represent a second new species.
Although there were no matches of our sequence data with other species of Corallina in GenBank, given that there are 204 species names for Corallina (Guiry & Guiry, Citation2020) and a large proportion have not been studied using molecular techniques, there is the possibility that an earlier name exists for our new species C. chamberlainiae. Two other names, Corallina berteroi and C. hombronii, have been used for coralline species documented during expeditions in the Falkland Islands. However, there is no evidence that C. berteroi was actually collected from the Falkland Islands. Samples under this name collected during the Challenger Expedition (1872–1876) are labelled in the BM ‘Valparaiso and Falklands’ but these are distinct localities and there is no evidence which specific material was collected from the Falkland Islands during the expedition. The other specimens of C. berteroi in the BM were collected from Antofagasta, Valparaiso, and the Juan Fernandez Islands in the Pacific. This material does not resemble C. chamberlainiae and its morphology appears to resemble Arthrocardia. The second species, C. hombronii, is listed from East Falkland but does not resemble C. chamberlainiae and it was a suspected Haliptilon species according to Garbary & Johansen (Citation1982).
These two examples illustrate the problem of the misapplication of names that is apparent for both C. chamberlainiae and C. cf. caespitosa. Whilst Scott & Holt (Citation2016) were more cautious using?Corallina sp., C. chamberlainiae was previously recorded as C. officinalis both in Tristan da Cunha (Chamberlain, Citation1965) and in the Falklands (specimens in the BM), and recorded as C. caespitosa in New Zealand (North Island and the Chatham Islands). C. officinalis sensu stricto is more or less confined to the North Atlantic (Brodie et al., Citation2013; Williamson et al., Citation2015), with a few reports of this species in the north-eastern Pacific (Hind & Saunders, Citation2013, as Pachyarthron; Magill et al., Citation2019).
Whether C. cf. caespitosa should be erected as a new species is less clear. The similarity of its COI-5P sequence to that of the holotype of C. caespitosa indicates that it is conspecific with this species, and this is supported by all species delimitation models used in this study. Although the COI-5P results might be an indication of recent divergence, the recognition of morphological differences between the two taxa, and separate resolution of C. cf. caespitosa with psbA, suggests that data from more samples and gene regions might provide a definitive answer. The close psbA match of C. cf. caespitosa with samples identified as C. pilulifera and C. melobesioides, both from Japan, hints that this taxon could be widespread beyond the Falkland Islands. C. pilulifera Postels & Ruprecht was originally described from ‘Siberia transbaicalensis’ (Postels & Ruprecht, Citation1840) which has been interpreted to be the Sea of Okhotsk (Silva et al., Citation1996: 232) between Russia and Japan. This would therefore represent an earlier name, but the Japanese sample from which the sequence was obtained is not considered to be C. pilulifera (Paul Gabrielson, personal communication). The synonymy of C. caespitosa with C. ferreyrae (Bustamante et al., Citation2019) is not supported in our study. We therefore retain C. caespitosa as a separate species.
The possible biogeographic signal revealed by the GMYC species delimitation model in C. chamberlainiae in the Falkland Islands may be evidence of speciation through isolation, although more data are required to confirm this. The results support the role of islands in driving speciation, juxtaposed with concepts of connectivity. During the Last Glacial Maximum (~23 to 18 ka), the Falkland Islands probably provided a refugia for species between the glaciated regions of Patagonia and South Georgia (Leese et al., Citation2008; González-Wevar et al., Citation2012). Recolonization events to these areas may have originated from the stable ‘founder’ populations in the Falkland Islands. Anecdotal evidence supports this hypothesis, suggesting that shallow marine species diversity of the Islands is higher than that of mainland southern South America, for both fauna and flora (Paul Brickle and Emma Beaton, unpublished data).
The presence of C. chamberlainiae on both the Falklands Islands and Tristan da Cunha provides evidence of connectivity between the two island groups; indeed, the biogeography of the temperate and sub-Antarctic South Atlantic is revealing some interesting patterns. Fraser et al. (Citation2010) used mitochondrial (COI-5P) and chloroplast (rbcL) markers to reveal genetic diversity within Durvillaea antarctica across temperate and sub-Antarctic regions. Although the widespread D. antarctica was a genetically diverse species, the vast majority of this variation was restricted to temperate latitudes. Within New Zealand and Chile, for example, substantial genetic differentiation was detected between populations separated by relatively small geographic distances (e.g. tens of kilometres). In higher sub-Antarctic latitudes, genetic homogeneity was detected over vast geographic distances. For example, samples from South Georgia, Marion, Crozet, Kerguelen, Macquarie and the Falkland Islands were essentially indistinguishable for both COI-5P and rbcL markers. Gough Island, further north and warmer than the aforementioned islands, also shared a common haplotype with the sub-Antarctic islands and had an additional haplotype. It is likely that there are further examples of historical and contemporary connectivity in marine plants and animals. At contemporary scales, rafting of buoyant kelps is likely to be an important ecological mechanism enabling kelp, associated flora (including epiphytes) and invertebrates to reach other biogeographic regions (Donald et al., Citation2005; Thiel & Gutow, Citation2005). Macrocystis pyrifera is also known to potentially remain alive and reproductively viable for long periods adrift at sea (Macaya et al., Citation2005; Hernández-Carmona et al., Citation2006) and therefore likely to facilitate species connectivity over large geographic regions.
This study highlights the fact that there is still much to discover about macroalgal biodiversity of the Falkland Islands, Tristan da Cunha, and other remote archipelagos in the South Atlantic. A global revision to the taxonomy of Corallina is needed, potentially through examination of multiple molecular markers for better species resolution and phylogenetic relationships. It is well known that the COI-5P marker potentially masks different species, and while psbA is recognized as a useful marker for screening diversity in coralline algae (Broom et al., Citation2008; Nelson et al., Citation2015), it may be of limited use in distinguishing species within the genus Corallina owing to low intraspecific sequence variation (Nelson et al., Citation2015). To better resolve the relationship between the seaweeds of Tristan da Cunha and the Falklands, we need more investigations in Chile, South Africa, South Georgia as well as New Zealand. Resolving species identities, concepts and factors such as dispersal and connectivity is important because this provides the baseline from which to measure future change in marine communities. It also may inform potential routes for non-native, potentially invasive species, to travel to new locations. Such knowledge can be invaluable in conservation and marine management in a time of rapid environmental change.
Supplementary information
The following supplementary material is accessible via the Supplementary Content tab on the article’s online page at https://doi.org/10.1080/09670262.2020.1780635
Supplementary table 1. Samples used for molecular analysis from Tristan da Cunha and the Falkland Islands.
Supplementary table 2. Summary of best-fit partitioning schemes and nucleotide substitution models for sequence alignments.
Supplementary fig. 1. Bayesian phylogenetic tree inferred for concatenated COI-5P+psbA sequence data.
Author contributions
J. Brodie conceived the study, collected the Falklands material and drafted the manuscript; S. Russell performed lab work; R. Mrowicki collected the Falklands material, performed lab work and molecular analysis, prepared the distribution maps and contributed to manuscript writing; L. Melbourne performed molecular and morphological analysis, drafted methods and prepared the morphological plates; P. Brickle provided connectivity insight and contributed to manuscript writing; S. Scott collected the material from Tristan da Cunha and edited the manuscript.
TEJP-2020-0002-File010.pdf
Download PDF (69.2 KB)TEJP-2020-0002-File009.docx
Download MS Word (22.4 KB)Acknowledgements
We are extremely grateful to the people of Tristan da Cunha and the Falkland Islands for their hospitality and support for this work. We particularly thank the staff at SAERI for their assistance with logistics for Juliet and Rob during their time in the Falkland Islands. We thank the two anonymous reviewers also for their helpful comments.
Disclosure statement
No potential conflict of interest was reported by the authors.
Supplementary Material
The following supplementary material is accessible via the Supplementary Content tab on the article’s online page at https://doi.org/10.1080/09670262.2020.1780635.
Additional information
Funding
References
- Bouckaert, R., Vaughan, T.G., Barido-Sottani, J., Duchêne, S., Fourment, M., Gavryushkina, A., Heled, J., Jones, G., Kühnert, D., De Maio, N., Matschiner, M., Mendes, F. K., Müller, N.F., Ogilvie, H.A., du Plessis, L., Popinga, A., Rambaut, A., Rasmussen, D., Siveroni, I., Suchard, M.A., Wu, C.-H., Xie, D., Zhang, C., Stadler, T. & Drummond., A.J. (2019). BEAST 2.5: an advanced software platform for Bayesian evolutionary analysis. PLoS Computational Biology, 15: e1006650.
- Brodie, J., Walker, R., Williamson, C. & Irvine, L.M. (2013). Epitypification and redescription of Corallina officinalis L., the type of the genus, and C. elongata Ellis & Solander (Corallinales, Rhodophyta). Cryptogamie, Algologie, 34: 49–56.
- Broom, J., Hart, D.R., Farr, T.J., Nelson, W.A., Neill, K.F., Harvey, A.S. & Woelkerling, W.J. (2008). Utility of psbA and nSSU for phylogenetic reconstruction in the Corallinales based on New Zealand taxa. Molecular Phylogenetics and Evolution, 46: 958–973.
- Bustamante, D.E., Calderon, M.S. & Hughey, J.R. (2019). Conspecificity of the Peruvian Corallina ferreyrae with C. caespitosa (Corallinaceae, Rhodophyta) inferred from genomic analysis of the type specimen. Mitochondrial DNA Part B Resources 4(1): 1285–1286.
- Castresana, J. (2000). Selection of conserved blocks from multiple alignments for their use in phylogenetic analysis. Molecular Biology and Evolution, 17: 540–552.
- Chamberlain, Y.M. (1965). Marine Algae of Gough Island. Bulletin of the British Museum (Natural History) Botany series, 3: 175–232.
- Dawson, E.Y., Acleto, O.C. & Foldvik, N. (1964). The seaweeds of Peru. Beihefte zur Nova Hedwigia 13: 1–111, 81 pls.
- Donald, K.M., Kennedy, M. & Spencer, H.G. (2005). Cladogenesis as the result of long-distance rafting events in Pacific topshells (Gastropoda: Trochidae). Evolution, 59: 1701–1711.
- Ezard, T., Fujisawa, T. & Barraclough, T. (2017). splits: SPecies’ Limits by Threshold Statistics. https://R-Forge.R-project.org/projects/splits/.
- Farr, T., Broom, J., Hart, D., Neill, K. & Nelson, W. (2009). Common Coralline Algae of Northern New Zealand. NIWA Information Series No. 70.
- Fraser, C.I., Winter, D.J., Spencer, G.H. & Waters, J.M. (2010). Multigene phylogeny of the southern bull-kelp genus Durvillaea (Phaeophyceae: Fucales). Molecular Phylogenetics and Ecology, 57: 1301–1311.
- Fujisawa, T. & Barraclough, T.G. (2013). Delimiting species using single-locus data and the Generalized Mixed Yule Coalescent approach: a revised method and evaluation on simulated data sets. Systematic Biology, 62: 707–724.
- Garbary, D.J. & Johansen, H.W. (1982). Scanning electron microscopy of Corallina and Haliptilon (Corallinaceae, Rhodophyta): surface features and their taxonomic implications. Journal of Phycology, 18: 211–219.
- González-Wevar, C.A., Hüne, M., Cañete, J.I., Mansilla, A., Nakano, T. & Poulin, E. (2012). Towards a model of postglacial biogeography in shallow marine species along the Patagonian Province: lessons from the limpet Nacella magellanica (Gmelin, 1791). BMC Evolutionary Biology, 12: 139. doi: 10.1186/1471-2148-12-139.
- Guiry, M.D. & Guiry, G.M. (2020). AlgaeBase. World-wide electronic publication, National University of Ireland, Galway. https://www.algaebase.org; searched on 21 April 2020.
- Harvey, A., Woelkerling, W., Farr, T., Neill, K. & Nelson, W. (2005). Coralline Algae of Central New Zealand: An Identification Guide to Common Crustose Species. NIWA Information Series No. 57.
- Hernández-Carmona, G., Hughes, B. & Graham, M.H. (2006). Reproductive longevity of drifting kelp Macrocystis pyrifera (Phaeophyceae) in Monterey Bay, USA. Journal of Phycology, 42: 119–1207.
- Hind, K.R. & Saunders, G.W. (2013). A molecular phylogenetic study of the tribe Corallineae (Corallinales, Rhodophyta) with an assessment of genus‐level taxonomic features and descriptions of novel genera. Journal of Phycology, 49: 103–114.
- Irvine, L.M. & Johansen, H.W. (1994). Corallinoideae. In Seaweeds of the British Isles Volume 1 Rhodophyta. Part 2B Corallinales, Hildenbrandiales (Irvine, L.M. & Chamberlain, Y.M., editors), pp. 37–57. HMSO, London.
- Johansen, H.W. (1981). Coralline Algae, A First Synthesis. CRC Press, Boca Raton, FL.
- Katoh, K. & Standley, D.M. (2013). MAFFT multiple sequence alignment software version 7: improvements in performance and usability. Molecular Biology and Evolution, 30: 772–780.
- Kimura, M. (1980). A simple method for estimating evolutionary rates of base substitutions through comparative studies of nucleotide sequences. Journal of Molecular Evolution, 16: 111–120.
- Kozlov, A.M., Darriba, D., Flouri, T., Morel, B. & Stamatakis, A. (2019). RAxML-NG: a fast, scalable and user-friendly tool for maximum likelihood phylogenetic inference. Bioinformatics, 35: 4453–4455.
- Lanfear, R., Frandsen, P.B., Wright, A.M., Senfeld, T. & Calcott, B. (2016).
- Leese, F., Kop, A., Wägele, J. & Held, C. (2008). Cryptic speciation in a benthic isopod from Patagonian and Falkland Island waters and the impact of glaciations on its population structure. Frontiers in Zoology, 5: 19. doi:10.1186/1742-9994-5-19.
- Macaya, E.C., Boltaña, S., Hinojosa, I.A., Macchiavello, J.E., Valdivia, N.A., Vásquez, N.R., Buschmann, A.H., Vásquez, J.A., Alonson Vega, J.M. & Thiel, M. (2005). Presence of sporophylls in floating kelp rafts of Macrocystis spp. (Phaeophyceae) along the Chilean Pacific coast. Journal of Phycology, 41: 913–922.
- Magill, C.L., Maggs, C.A., Johnson, M.P. & O’Connor, N. (2019). Sustainable harvesting of the ecosystem engineer Corallina officinalis for biomaterials. Frontiers in Marine Science, https://doi.org/10.3389/fmars.2019.00285.
- Melbourne, L.A., Hernández-Kantún, J.J., Russell, S. & Brodie, J. (2017). There is more to maerl than meets the eye: DNA barcoding reveals a new species in Britain, Lithothamnion erinaceum sp. nov. (Hapalidiales, Rhodophyta). European Journal of Phycology, 52: 166–178.
- Monaghan, M.T., Wild, R., Elliot, M., Fujisawa, T., Balke, M., Inward, D. J., Lees, D.C., Ranaivosolo, R., Eggleton, P., Barraclough, T.G. & Vogler, A.P. (2009). Acceleratedspecies inventory on Madagascar using coalescent-based models of species delineation. Systematic Biology, 58: 298–311.
- Müller, N.F. & Bouckaert, R.R. (2020). Adaptive parallel tempering for BEAST 2. bioRxiv doi: 10.1101/603514.
- Nelson, W., Sutherland, J.E., Farr, T.J., Hart, D.R., Neill, K.F., Kim, H.J. & Yoon, H.S. (2015). Multi‐gene phylogenetic analyses of New Zealand coralline algae: Corallinapetra novaezelandiae gen. et sp. nov. and recognition of the Hapalidiales ord. nov., Journal of Phycology, 51: 454−468.
- Nelson, W.A. (2009). Calcified macroalgae – critical to coastal ecosystems and vulnerable to change: a review. Marine & Freshwater Research, 60: 787−801.
- Okonechnikov, K., Golosova, O., Fursov, M. & the UGENE team. (2012). Unipro UGENE: a unified bioinformatics toolkit. Bioinformatics, 28: 1166–1167.
- Pardo, C., Peña, V., Barreiro, R. & Bárbara, I. (2015). A molecular and morphological study of Corallina sensu lato (Corallinales, Rhodophyta) in the Atlantic Iberian Peninsula. Cryptogamie, Algologie, 36: 31–54.
- Plummer, M., Best, N., Cowles, K. & Vines, K. (2006). CODA: Convergence Diagnosis and Output Analysis for MCMC. R News, 6: 7–11.
- Postels, A. & Ruprecht, F. (1840). Illustrationes algarum in itinere circum orbem jussu imperatoris Nicolai I. Atque auspiciis navarchi Friderici Lütke annis 1826, 1827, 1828 et 1829 celoce Seniavin exsecuto in Oceano pacifico, inprimis septemtrionale ad littora rossica asiatico-americana collectarum. pp. [i-vi], [i]- iv, 1-28 [1-2, index], [Latin:] [-iv], [1]-22, [1-2, index], 40 pls. Typis Eduardi Pratz, Petropoli [St. Petersburg].
- Puillandre, N., Lambert, A., Brouillet, S. & Achaz, G. (2012). ABGD, Automatic Barcode Gap Discovery for primary species delimitation. Molecular Ecology, 21: 1864–1877.
- R Core Team (2019). R: A Language and Environment for Statistical Computing. R Foundation for Statistical Computing, Vienna.
- Rambaut, A., Drummond, A.J., Xie, D., Baele, G. & Suchard, M.A. (2018). Posterior summarization in Bayesian phylogenetics using Tracer 1.7. Systematic Biology, 67: 901–904.
- Rasband, W.S. (1997– 2018). ImageJ. U.S. National Institutes of Health, Bethesda, Maryland. http://imagej.nih.gov/ij/.
- Ronquist, F., Teslenko, M., van der Mark, P., Ayres, D.L., Darling, A., Höhna, S., Larget, B., Liu, L., Suchard, M.A. & Huelsenbeck, J.P. (2012). MrBayes 3.2: efficient Bayesian phylogenetic inference and model choice across a large model space. Systematic Biology, 61: 539–542.
- Saunders, G.W. (2005). Applying DNA barcoding to red macroalgae: a preliminary appraisal holds promise for future applications. Philosophical Transactions of the Royal Society of London, B, Biological Sciences, 360: 1879–1888.
- Scott, S. (2017). A Biophysical Profile of the Tristan da Cunha Archipelago. Commissioned and reviewed by the Pew Charitable Trusts. https://www.pewtrusts.org/-/media/assets/2019/07/cp_on_a_remote_archipelago_rich_biodiversity_faces_threats.pdf.
- Scott, S. & Holt, R. 2016. Underwater life of Gough Island, South Atlantic Ocean. Marine
- Silva, P.C., Basson, P.W. & Moe, R.L. (1996). Catalogue of the benthic marine algae of the Indian Ocean. University of California Publications in Botany, 79: 1–1259.
- Thiel, M. & Gutow, L. (2005). The ecology of rafting in the marine environment. II. The rafting organisms and community. Oceanography and Marine Biology, an Annual Review, 42: 181–263.
- Twist, B.A., Neill, K.F., Bilewitch, J., Jeong, S.Y., Sutherland, J.E. & Nelson, W.A. (2019). High diversity of coralline algae in New Zealand revealed: Knowledge gaps and implications for future research. PLoS ONE, 14(12): e0225645.
- Walker, R.H., Brodie, J., Russell, S. & Irvine, L.M. (2009). The taxonomy and biodiversity of geniculate coralline algae in the northeastern Atlantic: Corallina caespitosa sp. nov. (Corallinoideae, Rhodophyta). Journal of Phycology, 45: 287–297.
- Warren, D.L., Geneva, A.J. & Lanfear, R. (2017). RWTY (R We There Yet): an R package for examining convergence of Bayesian phylogenetic analyses. Molecular Biology and Evolution, 34: 1016–1020.
- Williamson, C.J., Njorka, J., Perkins, R., Yallop, M.L. & Brodie, J. (2014). Skeletal mineralogy of geniculate corallines: providing context for climate change and ocean acidification. Marine Ecology Progress Series, 531: 71–84.
- Williamson, C.J., Walker, R.H., Robba, L., Yesson, C., Russell, S., Irvine, L.M. & Brodie, J. (2015). Towards resolution of diversity in Corallina (Tribe Corallineae, Rhodophyta) and related genera. Phycologia, 54: 2–11.
- Yoon, H.S., Hackett, J.D. & Bhattacharya, D. (2002). A single origin of the peridinin- and fucoxanthin-containing plastids in dinoflagellates through tertiary endosymbiosis. Proceedings of the National Academy of Sciences USA, 99: 11724–11729.
- Zhang, J., Kapli, P., Pavlidis, P. & Stamatakis, A. (2013). A general species delimitation method with applications to phylogenetic placements. Bioinformatics, 29: 2869–2876.