Abstract
A new species Temnogametum iztacalense sp. nov. (Zygnemataceae) was described using an integrative taxonomic approach. Material was collected between 2014–2019 from purple mats at the bottom of La Luna Lake, which is a shallow, well-oxygenated, acidic and ultraoligotrophic high mountain lake exposed to high levels of ultraviolet radiation. Morphological analysis revealed that the material belonged to the Zygnemataceae and contained mature zygospores of oblate-sphaeroidal shape; smooth and yellow exospores, finely punctate and brown mesospores, and thin hyaline endospores. Transmission electron microscopy showed vacuolization, wavy thylakoids and a large number of starch granules and plastoglobules. The topology of the concatenated 18S-rbcL tree using the Bayesian method separated T. iztacalense into an independent branch within the Zygnematales. The chromatographic profile of T. iztacalense revealed 20 compounds, the major ones being lutein (596.9 ± 20.9 mg g–1 algae) and β-carotene (171.4 ± 3.3 mg g–1 algae). This is the first reported phylogeny of Temnogametum isolated from this kind of lake based on 18S-rbcL sequences.
Introduction
High mountain lakes are generally exposed to conditions that largely result in oligotrophic environments, such as extremely fluctuating, transparent, well oxygenated water and poorly mineralized status which results in limited phytoplankton growth. These conditions allow the development of specific communities that are adapted to the physiological stress caused by ultraviolet radiation (UVR). It is already known that one of the most important features of the biota to reduce UVR damage is the synthesis of protoprotective compounds, including carotenoids; these metabolites are efficient at transforming the energy of UVR into heat without causing negative effects (Alcocer et al., Citation2020). The massive growth of phytobenthos is related to the quantity of nutrients, in particular phosphates, which are known to help protect against UVR damage (Carrillo et al., Citation2007). The knowledge of such ecosystems has been developed mainly in central and northern Europe and North America (Sommaruga, Citation2001; Catalán et al., Citation2006).
Species of Zygnemataceae are particularly abundant in the northern hemisphere, where they form bright green floating masses in many temperate environments in spring (Randhawa, Citation1959). Tapolczai et al. (Citation2015) noted that growth of these algae in the lakes of Lugano (Switzerland) and Kinneret (Israel) affected fishing and contaminated the drinking water supply. The formation and development of blooms of Mougeotia have also been recorded over several years in Lake Kinneret (Zohary et al., Citation2019).
Traditional taxonomy of the Zygnemataceae (Transeau, Citation1932; Randhawa, Citation1959; Gauthier-Lièvre, Citation1965; Kadlubowska, Citation1984) mainly considers morphological and reproductive species characteristics. However, molecular taxonomy of the Zygnemataceae has been advanced by contributions from McCourt et al. (Citation2000), Gontcharov et al. (Citation2004), Gontcharov (Citation2008), Kim et al. (Citation2012) and Stancheva et al. (Citation2012, Citation2014) who provide the sequences of several molecular markers for Zygnema, Spirogyra, Mougeotia and Zygogonium, among other taxa. However, the relationships within the family Zygnemataceae remain unresolved due to the limited scope of taxa analysed. The class Zygnematophyceae includes the order Zygnematales and two families: the Mesotaeniaceae and Zygnemataceae. The Zygnemataceae includes 16 genera with a broad global distribution, the most diverse genera include Spirogyra with 533 species, Zygnema with 210 species and Mougeotia with 174 species (Guiry & Guiry, Citation2019). On the other hand, the genus Temnogametum, established by West & West (Citation1897), is known to be rare and have a high morphological resemblance to Mougeotia in the vegetative state, but the number of zygospore wall layers is diagnostic (Temnogametum has three while Mougeotia has two; Transeau, Citation1932).
To date, 19 species of Temnogametum have been described. Gauthier-Lièvre (Citation1965) reported five species from Africa: T. africanum Gauthier-Liévre, T. congolense Gauthier-Liévre, T. gracilescens Gauthier-Liévre, T. heterosporum West & G.S.West (type) and T. rayssiae Bourrelly. Randhawa (Citation1959), Yamagishi (Citation1963), Chen & Zhou (Citation1980), Kadlubowska (Citation1984) and Panikkar & Ampili (Citation1988, Citation1991) provided data on the distribution, morphology and habitat of 10 species from Asia (India, Pakistan, China, Bangladesh and Japan): T. boreale Yamagishi, T. cylindrosporum M.O.P.Iyengar, T. fujianense Chen & Zhou, T. indicum M.O.P.Iyengar, T. keralense Panikkar & Ampili, T. malabaricum Panikkar & Ampili, T. mayyanadense Erady & Rajappan, T. prescottii R.J.Patel, T. tirupatiense M.O.P.Iyengar and T. subtropicum A.K.Islam.
Likewise, Brook & Johnson (Citation2002) described T. sinense C.C.Jao & H.J.Hu from the British Isles. Four species are known for the American continents; Transeau (Citation1932) described T. thaxteri Transeau from Trinidad and Tobago, Randhawa (Citation1959) reported T. transeaui Prescott from Ecuador and T. uleanum (Möbius) Wille from Brazil, while Wei & Ying-Kit (Citation2000) presented records of T. tirupatiense from Ontario lakes, Canada. Other records have included Temnogametum sp. from locations in the USA and the wetlands of Colombia (Hoshaw, Citation1983; Lasso et al., Citation2014).
Transeau (Citation1932), Randhawa (Citation1959), Gauthier-Lièvre (Citation1965), Chen & Zhou (Citation1980) and Panikkar & Ampili (Citation1988, Citation1991) reported the presence of purple cell sap in Temnogametum africanum, T. fujianense, T. indicum, T. keralense, T. mayyanadense, T. thaxteri, T. tirupatiense and T. uleanum, as well as in Zygogonium ericetorum Kützing and Zygnema terrestre Randhawa due to phycoporphyrin compounds. Ralph (Citation1962) reported that Mougeotia capucina C.Agardh and other Zygnemataceae had iron compounds and tannins that were responsible for forming the blue-purple and red-purple pigments.
Green filamentous algae of the class Zygnematophy-ceae belong to the most common primary producers in polar and temperate environments such as lakes, shallow pools and meltwater streamlets. Some of them proliferate in littoral areas of acidic lakes, where their composition is dominated by the Zygnematales including species of Zygnema, Spirogyra and Mougeotia (Randhawa, Citation1959; Wei et al., Citation1989; Graham et al., Citation1996; Pichrtová et al., Citation2018). Despite taxonomic revisions of the order Zygnematales from different parts of the world, many taxa have not been determined at the species level, because information on the reproductive structures is lacking and/or the number of taxa with molecular sequence data is small (Poulicková et al., Citation2007). In Mexico, the phycoflora was documented by Ortega (Citation1984) and Novelo & Tavera (Citation2011), but, there are no records for Temnogametum. Phytobenthos grow in the extreme conditions of the acid lake La Luna situated in the high mountain Nevado de Toluca located in the Trans-Mexican Volcanic Belt (Armienta et al., Citation2000; Alcocer et al., Citation2004; Alcocer, Citation2008). Consequently, this lake has served as a model to investigate climate change through the presence/absence of diatoms and chrysophytes (Caballero-Miranda, Citation1996; Vilaclara et al., Citation2005; Cuna et al., Citation2014) and the structure of zooplankton assemblages (Dimas-Flores et al., Citation2008) but investigations never included the Zygnematales. Furthermore, this genus has not been the subject of a molecular study in any part of the world. In the present study, we analysed the phosphates in sediment and inside Temnogametum iztacalense cells, ultrastructure, molecular phylogeny, morphology and pigments of the purple morphs of phytobenthos in La Luna Lake at Nevado de Toluca.
Materials and methods
This study was conducted on a natural population of the unique purple morph of T. iztacalense collected from La Luna (19°06′30′′N, 99°45′09′′W), at 4240 m above sea level. The lake, located inside the crater of the Nevado de Toluca volcano, Mexico, has a maximum length of 197 m in the WSW-ENE direction, a maximum width of 189 m, a volume of 159 865 m3, an area of 2.6 ha, a perimeter of 0.61 km with a maximum depth of 10 m. Records from 1982 to 2002 indicated that the maximum water temperature was 12.5°C in June and July, and the minimum was 4.8°C in January and February. This lake is ultraoligotrophic with low levels of chlorophyll (0.33 µg l–1, a transparency of 9.4 m) and UVR records of 5418 ± 2528 J m–2 day–1 (Banderas-Tarabay et al., Citation1991; Alcocer et al., Citation2004, Citation2020). The lake contains well-oxygenated water throughout the entire water column (close to 6.1 mg l–1), has a conductivity of 0.8–9.1 µS cm–1, an acidic pH (3.8–5.8) and low mineralization (Garduño et al., this study).
Sampling
Temnogametum iztacalense was repeatedly collected along the shoreline of La Luna using a random sampling technique (Bojorge-García et al., Citation2014) in 2014 (April and September), 2015 (October), 2016 (March and October), 2018 (August and September) and 2019 (January). Field material was transported immediately to the laboratory in 1 l jars containing 700 ml water from the site. The green filaments of Klebsormidium sp. and Zygnema sp. which grow between the purple mats of T. iztacalense (~0.1% of collections), were removed by hand using a stereoscopic microscope. Subsequently, the mat sample was divided equally into three parts: one part each for microscopic observations, DNA extraction and extraction for pigment profiles by HPLC-PDA.
Phosphate determination in sediment and Temnogametum iztacalense
The Mehlich 3 technique (Mehlich, Citation1984) was used to determine the concentration of phosphate in the sediment from La Luna Lake and T. iztacalense. In biological material, wet digestion in nitric acid and hydrochloric acid were used (AOAC, Citation1990).
Light microscopy (LM) and confocal laser scanning microscopy (CM)
Microscopic observations were made with an Olympus BX50 microscope equipped with a digital camera. The chloroplast, aplanospore and zygosporangium morphology images were acquired using a Leica TCS SP8 (Leica Microsystems, Mannheim, Germany) with a HP PL APO CS2 oil 63×/1.4 objective using the LAS X software in a 1024 × 1024 format at 400 Hz scan speed, equipped with an argon-Krypton laser (excitation wavelength 443 nm, emission wavelength 470 nm) and HyD detectors (Stancheva et al., Citation2016; Pichrtová et al., Citation2018). Measurements corresponded to at least 215 cells.
Staining methods were used to identify pectin, sporopollenin and cellulose. Pectin was stained (red) using ruthenium red 1%. Sporopollenin was stained (red) on the addition of a drop of concentrated HNO3 and toluidine blue 1%. Zygospore wall layers were successfully distinguished using a few drops of 10% KOH. The cellulose was stained (blue) using Calcofluor (Sigma-Aldrich) and a few drops of 10% KOH (Southworth, Citation1973; Poulicková et al., Citation2007; Stancheva et al., Citation2014).
Taxonomic determination followed the criteria of Transeau (Citation1932), Randhawa (Citation1959), Gauthier-Lièvre (Citation1965), Kadlubowska (Citation1984) and Brook & Johnson (Citation2002). All material was deposited in the herbarium (IZTA), Universidad Nacional Autónoma de México (UNAM), Iztacala herbarium (Thiers, Citation2020).
Transmission electron microscopy
The purple morph of T. iztacalense was preserved with 2% glutaraldehyde for 4 h, then post-fixed in 1% OsO4 for 30 min, followed by dehydration in 30–100% ethanol at 4°C. The samples were then transferred to 100% propylene oxide and infiltrated into epon-propylene oxide (1–1) for 48 h, followed by embedding in epon (Burke & Geiselman, Citation1971) and polymerization at 60°C for 48 h (Trenty et al., Citation1992; Holzinger et al., Citation2010). Cross sections were performed with a Leica ultramicrotome (Leica, Wetzlar, Germany) and observed with the JEOL 1200-EX II TEM at the Institute of Cellular Physiology, UNAM.
DNA extraction and sequencing
Whole genomic DNA of the purple T. iztacalense was extracted using the UltraClean Plant DNA Isolation Kit (MoBio Laboratories Inc., Carlsbad, California, USA) according to the manufacturer’s instructions. Genomic DNA was examined on a 1% agarose gel run at 80 volts for 20 min. For amplification of the 18S rRNA and rbcL genes, previously published primer pairs were used (). Samples were amplified (Biometra themocycler, Jena Company) using a 20 μl PCR reaction with NZYTaqII2 X Green Master Mix Kit (Nzytech, Portugal) as follows: Initial denaturation at 94°C for 1 min, followed by 35 cycles of 94°C for 1 min, 55°C for 45 s, 72°C for 1 min, and a final 10 min extension at 72°C. PCR products were separated in a 1.5% agarose gel in 1× TAE Buffer and photographed under UV transillumination. The samples were then extracted from gels and purified with NZYTech Gelpure Kit (Nzytech, Portugal). Sequences were obtained by direct Sanger sequencing of the purified amplicons at GATC Biotech (Germany).
Table 1. Oligonucleotides used for PCR and sequencing of 18S and rbcL for Temnogametum iztacalense
Sequence analysis and phylogenetic tree construction
Geneious 9.1 (Biomatters Ltd) was used to analyse and edit all sequence data. Our 18S and rbcL partial sequences were aligned with 29 Zygnematophyceae sequences from GenBank (). Alignments were constructed either individually or concatenated and were inspected by eye and submitted to Find-Model (http://www.hiv.lanl.gov/content/sequence/findmodel/findmodel.html) in order to obtain the best substitution model. GTR+ gamma model was used in all phylogenetic analysis. Phylogenetic trees were inferred with MrBayes 3.2.6 (Ronquist & Huelsenbeck, Citation2003), using Maximum likelihood (ML) and Maximum parsimony (MP) criteria plugins developed for Geneious, with a nodal support calculated with 100 000 bootstrap replicates. Bayesian analysis were run for 1 000 000 generations, sampling every 200 generations with a 10% burn-in. 18S rDNA (1660 aligned bp including gaps) and rbcL (1286 bp) were analysed separately and concatenated (2946 bp).
Table 2. Taxa used in the phylogenetic analysis, designation of strains or voucher/GenBank accession numbers of SSU and rbcL. Mexican material in bold
Pigment extraction
Three methods of sampling fresh T. iztacalense were used. Pigment sample (PS): algae were frozen with liquid nitrogen in the study area and lyophilized. Filamentous sample (FS): algae were washed with water and filtered through Whatman® GF/C (Merck), then frozen at −70°C and lyophilized. Lyophilized sample (LS): algae were washed with water and passed through a fine mesh (10 μm), then frozen at −70°C and lyophilized. All samples were reduced to a fine powder using a Precellys®24 homogenizer (Bertin Technologies) and kept in the dark until extraction.
Pigments were extracted from T. iztacalense dry biomass with solvents of different polarities including an acetone extraction, and a sequential extraction with hexane, ethyl acetate and methanol. First, 150 mg of powdered T. iztacalense (PS, FS and LS each) was suspended in 10 ml of acetone and disrupted by sonication (VibraECell, USA) at a frequency of 70–80 Hz for 3 min. Cell debris was removed by centrifugation (15 000 g, 10 min, 4ºC; Gyrozen 2236R, South Korea). The acetone extraction process was repeated five times, and the supernatants resulting from each extraction were combined and evaporated under reduced pressure (Centrivap Vacuum Concentrator, Labconco, USA). The dry extracts were kept at −20ºC until pigment analysis could be completed. In the sequential extraction 500 mg of powdered T. iztacalense (PS, FS and LS each) was suspended in 20 ml of hexane, and disrupted by sonication (VibraECell, USA) at a frequency of 70–80 Hz for 3 min. Cell debris was removed by centrifugation (15 000 g, 10 min, 4ºC; Gyrozen 2236R, South Korea), and the supernatant was collected and evaporated under reduced pressure (Centrivap Vacuum Concentrator, Labconco, USA). The cell debris was subsequently extracted with 20 ml of ethyl acetate, and finally with 20 ml of methanol. The supernatants were evaporated under reduced pressure and kept at −20ºC until analysis. In separated HPLC-PAD analysis, the dry extracts were resuspended in methanol (HPLC grade) to a final concentration of 10 mg ml–1 and filtered through a 0.22 μm pore membrane.
Determination of carotenoid and chlorophyll profile using HPLC-PDA
The pigment profiles of T. iztacalense resulting from the three drying and two extraction methods were achieved using HPLC-PDA following the methods of Amaro et al. (Citation2015). The extracts were analysed in a HPLC system coupled with a PDA, Waters Alliance 2695 (Waters, USA), in a C18 100A column (250–4.6 mm; Phenomenex) as stationary phase. A column heater (Waters, USA) was used to maintain the constant temperature, at 25ºC, during the analyses. The mobile phase consisted of ethyl acetate (A) and acetonitrile:water 9:1 (v/v) (B) (VWR Prolabo), starting with 100% B and installing a gradient to obtain 40% B at 31 min, 40% B at 36 min, 0% B at 38 min, 0% B at 43 min and 100% B from 50–55 min. The flow rate was 1 ml min–1 and the injection volume was 20 μl. All the HPLC-grade solvents used in the analysis were filtered with GH Polypro (GHP) Membrane Disk Filters (0.2 μm diameter, Pall, NY, USA). Data were processed using Empower chromatography software (Waters, USA). Spectra data from all compounds were collected in the range 250–750 nm.
Compounds were identified by comparing their retention times and UV-Vis spectra with those of authentic standards (Extrasynthese, Genay, France and Sigma Aldrich, St. Louis, USA). Carotenoid quantification was achieved by measuring the absorbance recorded on the chromatograms relative to external standards, at 450 nm. Lutein, chlorophyll-a and β-carotene were quantified with the authentic standards; unidentified carotenoids were quantified as lutein, chlorophyll derivatives as chlorophyll-a, violaxanthin derivatives as violaxanthin and Ɛ-carotene derivatives as Ɛ-carotene. Calibration curves were obtained with standard solutions corresponding to five different concentrations, selected as representative of the range of compounds concentrations in the samples. The calibration plots, r2 values, the limit of detection (LOD = 3So/b) and the limit of quantification (LOQ = 10So/b) (where So is the standard deviation of signal-to-noise ratio and b is the slope of the calibration plot) for the analysed carotenoids and chlorophyll-a are shown in . Pigment quantity in each sample was calculated as μg g–1 of lyophilized algal biomass for each drying, extraction method.
Table 3. Calibration curves of authentic standards used for quantification of different carotenoids and chlorophyll-a
Statistical analysis
Statistical analysis was performed using IBM SPSS STATISTICS software, version 25.0 (IBM Corporation, New York, USA, 2011). Data were analysed for normality and homogeneity of variances by Kolmogorov–Smirnov and Levene’s tests, and then a one-way ANOVA, using a Tukey’s HSD (honest significant difference) as post-hoc test, was used to identify significant variation across extraction methodologies.
Results
Phosphate in sediment and T. iztacalense
The phosphate levels in the sediment ranged from 119–166 ppm, while values were 890 ppm PO4 inside Temnogametum. This indicates a higher concentration (7-fold) inside Temnogametum cells with respect to the sediment.
Transmission electron microscopy
In the purple morph of T. iztacalense (–), polymorphic vacuoles of 0.94–6.6 μm in diameter were observed that were electron-translucent in the periphery and centre of the cell. The cell wall was 0.43–0.86 μm thick and smooth (). An ovoid nucleus with a spherical nucleolus was also observed (). The chloroplast contained thylakoid membranes and electron-dense pyrenoids surrounded by large translucent starch granules. The chloroplast showed thylakoids organized in sacs, some of which penetrated into the interior of the pyrenoid ().
Figs 1–6. Transmission electron micrographs of T. iztacalense collected in the field. Fig. 1. Cross-section of the cell with electron-dense masses accompanied by numerous vacuoles; Fig. 2. Nucleus near the chloroplast; thylakoid membranes can be distinguished in the chloroplast; pyrenoid intraplastidal; Fig. 3. Pyrenoid with invaginations of the thylakoid membrane (arrow) with starch granules around it and numerous plastoglobules of different sizes on the thylakoid membrane; Fig. 4. Wavy thylakoids; Fig. 5. Mitochondria with different electron densities due to the crystals present; Fig. 6. Golgi apparatus surrounded by an electron-dense mass. Abbreviations: Cl, chloroplast; G, Golgi apparatus; M, mitochondria; N, nucleus; P, pyrenoid; CW, cell wall; PG, plastoglobules; S, starch granules; and V, vacuoles. Scale bars: Fig. 1= 2 μm; Fig. 2 = 5 μm; Figs 3 and 5 = 0.5 μm; Figs 4 and 6 = 200 nm
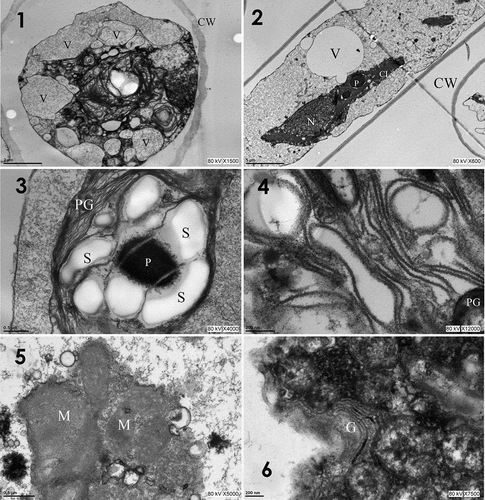
The chloroplast of T. iztacalense has corrugated thylakoid membranes in a parallel orientation and abundant electron-dense plastoglobules of 0.083–0.416 μm in diameter (). The mitochondria were present as electron-opaque crystals in the lumen (), and the Golgi apparatus was surrounded by electron-dense materials ().
Phylogenetic analyses
Sequences of 18S rDNA and rbcL were obtained from DNA isolated from T. iztacalense at La Luna Lake (). By combining the 18S and rbcL data sets (2946 nt) in a concatenated model, all Zygnematophyceae lineages were resolved, and two main clade clusters corresponding to the orders Desmidiales and Zygnematales were revealed (). The first clade comprised Cosmarium (DESM), Closterium (CL), Gonatozygon and Genicularia (GON) and Netrium (N). The order Zygnematales presented as a polytomy comprising six branches included in the ‘crown Zygnematales’, including long-branches of Spirogyra (SPI), Mougeotia-Mesotaenium (MOUG), Mesotaenium, Zygnemopsis, Cylindrocystis (MZC) and Zygnema (ZYG). Between both clade clusters, T. iztacalense sp. nov. formed an independent branch inside the order Zygnematales. The topology of the concatenated 18S-rbcL tree inferred with the Bayesian method, separated the material from Mexico into an independent branch (Supplementary figs 1 and 2 respectively). The information provided by these markers supports the differences at a molecular level between taxa whose genetic distance was 10.5%.
Fig. 7. Zygnematophyceae phylogeny based on 29 concatenated sequences (18S rDNA with rbcL, 2946 aligned positions), using the GTR + gamma substitution model. Bootstrap posterior probability values are indicated on each node (Bayesian/Maximum likelihood/Maximum parsimony). The new species described in this study is indicated in bold. Clade clusters were designated according to Gontcharov et al. (Citation2004); Gontcharov & Melkonian (Citation2010). Branch was reduced 50% (//). Phylogram was inferred using the Bayesian approach
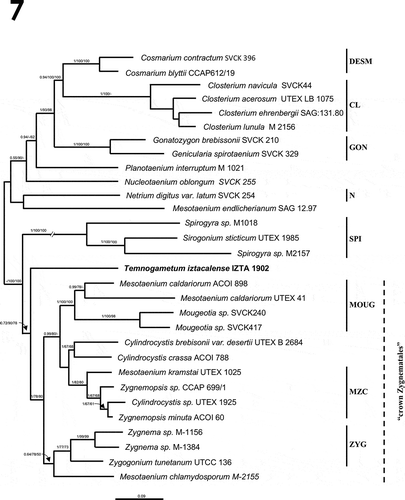
Description of the new species
Temnogametum iztacalense Garduño & Martínez sp. nov. (Class Zygnematophyceae, Order Zygnematales, Family Zygnemataceae)
Habitat: Phytobenthos of purple mats grown in the La Luna Lake, Mexico (–).
Fig. 8-12. Fig. 8 Area of study, La Luna Lake, Estado de Mexico, Mexico; Fig. 9. Detail of Temnogametum iztacalense forming dark coloured bottom mats; Fig. 10. Young purple filament vegetative cell with ribbon-shaped chloroplast containing the row of pyrenoids and central nucleus (LM; n, nucleus); Fig. 11. Imaging fluorescence of one chloroplast and pyrenoids in T. iztacalense (CM; Cl Chloroplast; p, pyrenoids; s, septum); Fig. 12. Maturing grey-purple filament (LM). Figs 10–12 Scale bar = 20 μm
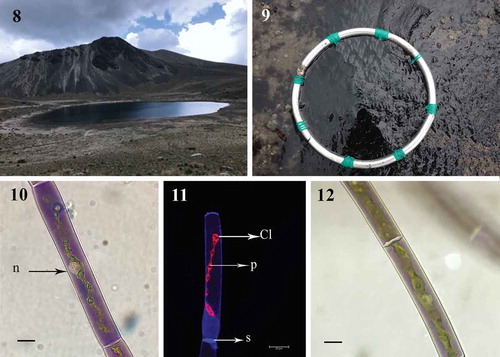
Diagnosis: The mean values of width and length for vegetative cells were 17 (12–28) μm and 254 (71–387) μm respectively. The cells were cylindrical, with one ribbon-shaped chloroplast occupying more than half of the cell, with 3–16 pyrenoids in a single row (). Cell sap has a deep purple colour in young filaments and central nucleus (), but in most cells the filaments in reproduction were grey-violet or green (). Irregular and self-coiled filaments (), either lateral and scalariform conjugation (–); gametangia 17–23 μm diameter × 13–19 μm long; zygospore formed in the conjugation tubes, space between the zygospore and the zygosporangium is filled of homogeneous pectin (). The wall of zygosporangium of cellulose (). With two or more zygospores in series (). The young zygospore was irregularly square with rounded angles and concave sides (–). Mature zygospore in surface view, has oblate-sphaeroidal shape, 30–60 μm wide × 30–57 μm long × 12–20 μm height (, ), while in side view they are triangular to ellipsoidal (). The zygospore has a three-layered wall (–): exospore thin, smooth and yellow; mesospore finely punctate of brown colour (); and a thin hyaline endospore, with a sigmoid line (). Zygospores by lateral conjugation were obliquely ovoid, 35 μm × 43 μm. Sexual reproductive structures were observed in September (2014, 2018), October (2015, 2016) and January (2019). Aplanospores of 10–20 μm wide and 19–40 μm long (–).
Fig. 13-17. Fig. 13 Filament in early stages of scalariform conjugation with dark purple colour (LM); Figs 14–15. Scalariform conjugation, detail of short cells and different colour and conjugation tube (LM); Figs 16–17. Young zygospores in surface view of quadrangular shape and quadrangular with rounded angles (LM). Figs 13–17 Scale bar = 50 μm
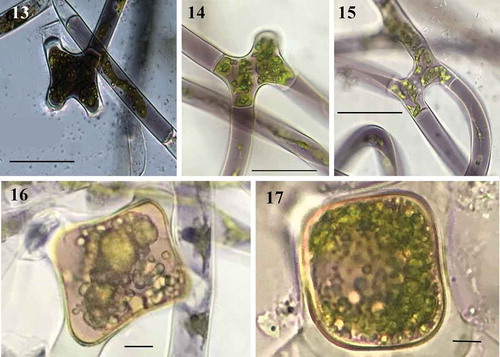
Fig. 18-20. Fig. 18 Conjugation between cells of the same filament forming a loop (LM); Fig. 19. Two young zygospores in a filament (LM); Fig. 20 Side view triangular of zygospores (LM). Figs 18, 20 Scale bar = 20 μm, Fig. 19. Scale bar = 50 μm
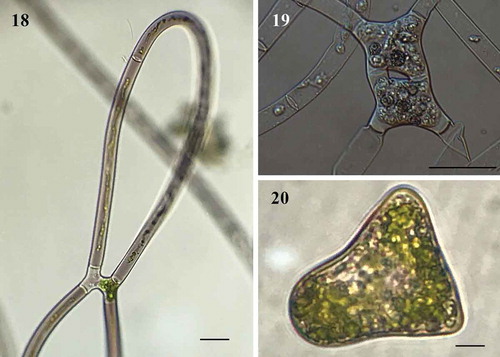
Fig. 21-24. M Fig. 21 Mature zygospores in surface view with natural brown colour (LM); Fig. 22. Mature zygospores in surface view, stained with toluidine blue (LM); Fig. 23. Space between the zygospore and the zygosporangium, stained red with ruthenium red (LM); Fig. 24. Sporangium strain with calcofluor (CM). Figs 21-24 Scale bar = 20 μm
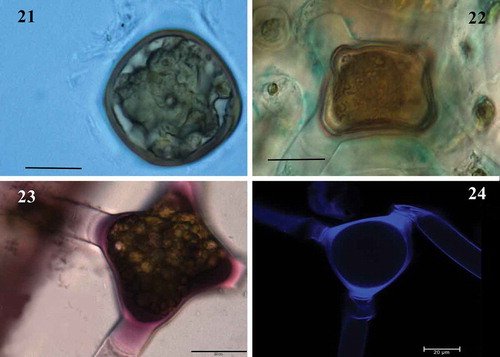
Fig. 25-28. Fig. 25 Zygospore with three-layered wall, mesospore finely punctate and brown colour (LM); Fig. 26. Detail of mesospore finely punctate (LM); Fig. 27. Mature zygospore showing the ‘riss-linie’ sigmoid (LM); Fig. 28. Detail of mesospore finely punctate and ‘riss-linie’ sigmoid (LM). Fig. 25. Scale bar = 20 μm. Figs 26-28 Scale bar = 10 μm
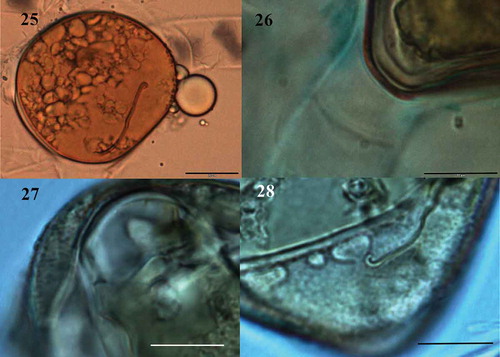
Fig. 29-30. Fig. 29 Aplanospore strain with calcofluor (CM); Fig. 30. Aplanospore (LM); Fig 29. Scale bar = 10 μm. Fig. 30. Scale bar = 20 μm
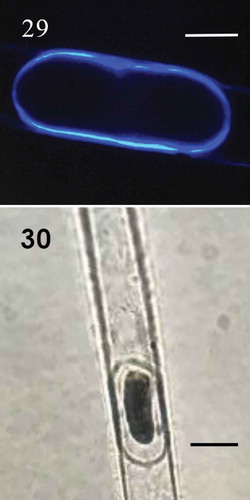
Holotype: IZTA-1902. Facultad de Estudios Superiores Iztacala, UNAM. IZTA Herbarium. Collected by: Carlos López Salas and Saúl Aldair Fuentes Zuno 26/09/2018. La Luna Lake, Nevado de Toluca, Estado de México. Mexico.
ISOTYPE: IZTA-1894. Facultad de Estudios Superiores Iztacala, UNAM. IZTA Herbarium. Collected by: Carlos López Salas and Gloria Garduño Solórzano, 01/08/2018. La Luna Lake, Nevado de Toluca, Estado de México. Mexico.
Etymology: From the Nahuatl language for ‘Place of White Houses’. Dedicated to the place of work and academic development of the first author.
GenBank accession number sequences: MK684164 (18S rDNA partial) and MK688381 (rbcL partial).
The main morphological and ecological characteristics of T. africanum, T. boreale, T. fujianense, T. gracilescens and T. tirupatiense were compared because these species are characterized as having a chloroplast with pyrenoids in a row, as well as scalariform and lateral reproduction ().
Table 4. Morphological and ecological features of Temnogametum iztacalense in comparison with other species having pyrenoids in a row. Differences are in bold, nd = no data
Pigments
The purple morph of T. iztacalense showed a chromatographic profile with 20 compounds, corresponding to 10 carotenoids and 10 chlorophylls (–). shows the pigments profile obtained with the different extracts. Acetone is known for its high efficiency in carotenoid extraction, hence it was chosen to compare different methodologies of biomass treatment upon collection to select the most efficient method for carotenoid conservation.
Table 5. Carotenoid and chlorophyll content in different Temnogametum iztacalense extracts (μg g–1 dry biomass) determined by HPLC-PDA1,2,3
Figs 31–32. Chromatograms of photosynthetic pigments of the different Temnogametum iztacalense extracts (μg g−1 dry biomass) determined by HPLC-PDA; which showed 20 compounds, with lutein and β-carotene being the main components; Fig. 31. Pigment sample PS-acetone, material was frozen with liquid nitrogen in the study area and lyophilized; Fig. 32. Lyophilized sample LS-ethyl acetate
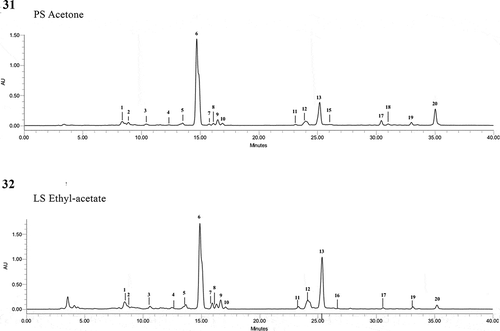
The sample subjected to freezing with liquid nitrogen upon collection (PS) had the highest total pigments content (10241.951 µg g–1 dry biomass), followed by LS (6376.277 µg g–1 dry biomass) and FS (2213.516 µg g–1 dry biomass) (p < 0.05, ANOVA, Tukey’s HSD). These last two treatments were shown to be less effective, beyond the loss of pigments ().
The same trend was also observed in all samples, for total carotenoids and chlorophylls, with the latter being present in higher amounts. Lutein (–; peak 6, ) was the most abundant carotenoid, with amounts ranging from 222.388 (FS) to 596.940 (PS) µg g–1 of dry biomass, followed by β-carotene (–; peak 20, ) (56.298–171.422 µg g–1 dry biomass). Violaxanthin and Ɛ-carotene derivatives were also tentatively identified in all samples.
In order to better clarify the effect of the extraction solvent on the pigment profile, LS and FS, the samples present in higher amounts were also subjected to extraction with hexane, ethyl acetate and methanol. As can be observed through the analysis of , ethyl acetate was the most efficient solvent for pigment extraction, followed by hexane and methanol (p < 0.05, ANOVA, Tukey’s HSD). Except for chlorophyll-a (–, peak 16) which was not detected in acetone extracts, the pigment qualitative profile was similar for all the solvent systems explored herein. However, from a quantitative point of view, acetone was by far the most effective solvent for pigments extraction ().
Discussion
During the fieldwork the purple mats of T. iztacalense were dominant in the phytobenthos of the acid lake studied and this is the first report of blooms; this habitat is different from those reported by Randhawa (Citation1959) and Gauthier-Lièvre (Citation1965) which included marshes, streams, channels, bogs, ponds and shallow water in paddy field in tropical and subtropical areas from Africa and Asia. The concentration of phosphorus in sediment of the study area and inside T. iztacalense cells was high; in contrast, the lake water column has been classified as an ultraoligotrophic environment with phosphates of 0.13 ± 0.15 PO4 μM (Alcocer et al., Citation2004, Citation2020). This allows us to explain the macroscopic mats of phytobenthos, and our results were consistent with Carrillo et al. (Citation2007), who recognized that phosphorus inputs diminish the negative effects of ultraviolet radiation on algae in high mountain lakes; it also explains how these filamentous algae obtain their nutrients and can bloom, as has been reported in Lake Ontario, Canada (Geider & La Roche, Citation2002). These data reveal the intrinsic variations of the natural limnological and geochemical processes that occur between the water column and the sediments of this extreme environment.
Molecular data
Molecular results in this study support the finding that the morphology of material obtained from La Luna Lake, Mexico, was consistent with the description of the genus Temnogametum and distinct from the description of the molecular results of the genus Mougeotia despite the morphological similarity between these two genera. Molecular studies on Temnogametum have not been previously carried out, and those of Mougeotia are scarce (Kenneth et al., Citation2001).
Therefore, we considered the rbcL and 18S data from representatives of the order Zygnematales: Zygnemataceae () and analysed their phylogenetic positions. The use of a range of molecular markers has allowed resolution of some nodes that were previously unresolved (McCourt et al., Citation2000; Gontcharov et al., Citation2004; Gontcharov, Citation2008; Gontcharov & Melkonian, Citation2010) and hence allowed the phylogeny in this class to be partially elucidated. Likewise, the topology of the tree reported in this work in relation to the orders Desmidiales and Zygnematales was consistent with published data (Gontcharov et al., Citation2004; Gontcharov & Melkonian, Citation2010). It also indicates that Temnogametum is a paraphyletic taxon and confirms its location in the family Zygnemataceae.
Since the early reports of Temnogametum spp. in the American continent (Transeau, Citation1932), the discovery of T. iztacalense in Mexico is only the fifth reporting of species of this genus in the region (Randhawa, Citation1959; Wei & Ying-Kit, Citation2000). The extreme environments where species tend to occur, such as high mountains in various countries of the continent, for example Prescott (Citation1947) reported T. transeauii of bog from Ecuador at 5997 m, suggest Temnogametum’s diversity is underestimated. This is compounded due to the frequent lack of defining morphological and reproductive characters, such as mature zygospores (Wei & Ying-Kit, Citation2000) and the need for molecular analysis.
The results indicate that T. iztacalense is related to T. africanum, T. boreale, T. fujianense, T. gracilescens and T. tirupatiense. These species are characterized by a chloroplast with pyrenoids in one row and reproduction by zygospores (Yamagishi, Citation1963; Gauthier-Lièvre, Citation1965; Wei & Ying-Kit, Citation2000). Conversely, mesospore ornamentation in the new species differs, being smooth in T. africanum, T. boreale and T. fujianense, while it is finely scrobiculate in T. gracilescens and finely punctate in T. iztacalense. The occurrence of T. iztacalense in an acid lake at 4240 m above sea level differs from the others that occur in lakes, rivers, streams and marshes in the range of 8–2160 m above sea level ().
The species that is most similar to T. iztacalense is T. tirupatiense; T. iztacalense has a shorter cell length (71–387 μm versus 132–540 μm), a different number of pyrenoids (3–16 versus 10–15), and space between the zygospore and the zygosporangium (homogeneous versus lamellated), based on the surface view of the mature zygospore (oblato-sphaeroidal versus quadrangular with concave sides) (Randhawa, Citation1959; Wei et al., Citation1989; Wei & Ying-Kit, Citation2000). The brown colour of the zygospores in T. iztacalense is reported for this structure which can be hyaline, yellow, pink, red, orange and brown (Randhawa, Citation1959). This colour might relate to the phenolic compounds recognized in these structures in the genus Zygnema (Pichrtová et al., Citation2013).
Ultrastructure
The ultrastructure findings show that the purple mats of T. iztacalense had wavy thylakoids, many plastoglobules and large vacuoles similar to those reported in green algal chloroplasts subjected to excess UVR, including in Prasiola crispa (Ulvales) and Zygnema spp. in the polar regions (Antarctica), where cells were exposed to physiological stress (Holzinger & Lütz, Citation2006; Pichrtová et al., Citation2013).
Moreover, our biological material showed pyrenoids with some thylakoid membranes that cross them, surrounded by many starch granules which is similar to what has been recorded in the order Zygnematales by Randhawa (Citation1959) and Herburger et al. (Citation2015). The vacuoles of T. iztacalense were up to three times larger (6.6 μm) than those of Zygogonium ericetorum with vacuoles 1–2 μm in diameter as reported by Holzinger et al. (Citation2010). In addition, the vacuoles were located near the periphery of the cell, perhaps to protect from the extreme environmental conditions, similar to what was observed for Zygnema spp. in polar regions (Pichrtová et al., Citation2013). In addition, the presence of numerous mitochondria and dense Golgi apparatus in T. iztacalense cells suggests significant cellular activity as Remias et al. (Citation2011) observed in the ultrastructure of Ancylonema nordenskiöldii from glaciers.
Pigments
This is the first report of the pigment profile of T. iztacalense, as well as the comparison of different methodologies, and where lutein and β-carotene were two carotenoids with high concentrations, as found in culture of Mougeotia spp. (Muntean et al., Citation2007). Sommaruga (Citation2001) indicated that xanthophyll pigments such as lutein and zeaxanthin protect the photosynthetic system under conditions of photooxidative stress; in this mechanism, most absorbed sunlight is removed and dissipated as heat.
Xanthophylls, zeaxanthin and antheraxanthin are necessary not only for immediate thermal dissipation but also to dissipate persistent thermal energy in plants under long-term environmental stress, a process by which the xanthophyll cycle accumulates zeaxanthin and antheraxanthin. As a whole, these pigments might contribute to the purple colour of T. iztacalense. In contrast, Aigner et al. (Citation2013) reported that the purple colouration of Zygogonium ericetorum was related to the presence of soluble compounds such as polyphenolic and hydrolysable tannins which were not investigated in this study.
Other pigment studies identified (Othman et al., Citation2018) found carotenoid compounds in six green algae, of which Chlorella fusca was the one that had the highest amount of lutein with 69.5 µg g–1. Meanwhile, β-carotene was present in C. vulgaris with 18.4 µg g–1. In contrast, for T. iztacalense, we identified nine times more lutein and β-carotene at concentrations of 596.9 µg g–1and 171.0 µg g–1, respectively. Meanwhile, Figueroa et al. (Citation2009), Remias & Lutz (Citation2007) and Remias et al. (Citation2011) reported that the carotenoids of Zygnemopsis decussata, Chlamydomonas nivalis, Haemotococcus pluvialis and Ancylonema nordenskiöldii provided protection against excessive irradiation on glaciers in the Arctic, indicating that the high amount of carotenoids in the material from Mexico is a possible mechanism of protection against UV radiation. This work recognizes a new species with a polyphasic approach of the Temnogametum genus and the high lutein and β-carotene content in T. iztacalense could be a promising source of antioxidants, and worthy of further study.
Author contributions
G. Garduño-Solórzano: original concept, preparation of T. iztacalense extracts, species diagnosis, sampling, electron microscopy, manuscript drafting and editing; M. Martínez-García: DNA isolation, PCR, preparation of T. iztacalense extracts diagnosis, manuscript drafting and editing; G. Scotta Hentschke: analysis of molecular data, manuscript drafting and editing; G. Lopes: determination of carotenoids and chlorophylls profile by HPLC-PDA, manuscript drafting and editing; R. Castelo Branco: PCR, sequencing, and manuscript editing; V.M.O. Vasconcelos: coordinator of the experimental phase of molecular biology, profile by HPLC-PDA, and manuscript editing; J.E. Campos: sequences analysis and phylogenetic tree construction, manuscript drafting and editing; R. López Cano: sampling, phosphate determination in soil and T. iztacalense, and manuscript editing; R.E. Quintanar-Zúñiga: microscopy and manuscript editing.
TEJP-2019-0087-File017.tiff
Download TIFF Image (19.3 MB)TEJP-2019-0087-File016.tiff
Download TIFF Image (19.3 MB)Acknowledgements
We acknowledge the Program for the Recovery and Sustainable Management of the Nevado de Toluca by Ignacio Pichardo Pagaza. We thank Sofía Manzur García Maass and the Direction of the Area of Protection of Flora and Fauna Nevado de Toluca by Luis Miguel Mandujano. We thank the Director General of ICAMEX, Pedro Mijares Oviedo and Guadalupe González Castorena for the analysis of sediment, and Rodolfo Paredes of the Institute of Cellular Physiology, University of Mexico, for the support of MET. We would like to thank all colleagues who helped us to collect field samples, mainly Carlos Lopez Salas, Monserrat Flores Calzada and Daniel Sanchez Avila. We acknowledge the DGAPA by the Programa de Apoyo para la Superación del Personal Académico, UNAM (PASPA) for financial support for the Research Stay in CIIMAR, Oporto, Portugal and at the Laboratory of Algología from Facultad de Biología and Microscopy Service of Murcia University, Spain; Marina Aboal and María Teresa Coronado Parra. We thank the anonymous reviewers who added their valuable comments to the final writing.
Disclosure statement
No potential conflict of interest was reported by the authors.
Supplementary Information
The following supplementary material is accessible via the Supplementary Content tab on the article’s online page at https://doi.org/10.1080/09670262.2019.1789226.
Supplemental figure 1 Zygnematophyceae phylogeny based on 29 18S rDNA sequences (1660 aligned positions), using the GTR + gamma substitution model. Bootstrap posterior probability values are indicated on each node (Bayesian/Maximum likelihood/Maximum parsimony). The new species described in this study is indicated in bold. Clade clusters were designated according to Gontcharov et al. (Citation2004) and Gontcharov & Melkonian (Citation2010). Phylogram was inferred using the Bayesian approach and the tree was constructed with the Neighbour-joining method.
Supplemental figure 2 Zygnematophyceae phylogeny based on 30 rbcL sequences (1286 aligned positions), using the GTR + gamma substitution model. Bootstrap posterior probability values are indicated on each node (Bayesian/Maximum likelihood/Maximum parsimony). The new species described in this study is indicated in bold. Clade clusters were designated according to Gontcharov et al. (Citation2004) and Gontcharov & Melkonian (Citation2010). Phylogram was inferred using the Bayesian approach and the tree was constructed with the Neighbour-Joining method.
Additional information
Funding
References
- Aigner, S., Remias, D., Karsten, U. & Holzinger, A. (2013). Unusual phenolic compounds contribute to ecophysiological performance in the purple-colored green Zygogonium ericetorum (Zygnematophyceae, Streptophyta) from a high-alpine habitat. Journal of Phycology, 49: 648–660.
- Alcocer, J. (2008). Limnology. In The Heavenly Waters of Nevado de Toluca (Luna, P., Montero, A. & Junco, R., editors), 10–12. INAH, México.
- Alcocer, J., Oseguera, L.A., Escobar, E., Peralta, L. & Lugo, A. (2004). Phytoplankton biomass and water chemistry in two high-mountains tropical lakes in central Mexico. Arctic Antarctic and Alpine Research, 36: 342–346.
- Alcocer, J., Delgado, C.L. & Sommaruga, R. (2020). Photoprotective compounds in zooplankton of two adjacent tropical high mountain lakes with contrasting underwater light climate and fish occurrence. Journal of Plankton Research, 1: 1–14.
- Amaro, H.M., Fernandes, F., Valentao, P., Andrade, P.B., Sousa-Pinto, I., Malcata, F.X. & Guedes, A. (2015). Effect of solvent system on extractability of lipidic components of Scenedesmus obliquus (M2-1) and Gloeothece sp. on antioxidant scavenging capacity thereof. Marine Drugs, 13: 6453–6471.
- AOAC. (1990). Association of Official Analytical Chemicals, Official Methods of Analysis, 15th edition. AOAC, Washington, DC.
- Armienta, M.A., De la Cruz-Reyna, S. & Macías, J.L. (2000). Chemical characteristics of the crater lakes of Popocatepetl, El Chichon and Nevado de Toluca volcanoes, Mexico. Journal of Volcanology and Geothermal Research, 97: 105–125.
- Banderas-Tarabay, A., González Villela, R. & Lanza Espino, G. (1991). Limnological of a high-mountain lake in Mexico. Hydrobiología, 224: 1–10.
- Bojorge-García, M., Carmona, J. & Ramírez, R. (2014). Species richness and diversity of benthic diatom communities in tropical mountain streams of Mexico. Inland Waters, 4: 279–292.
- Brook, A.J. & Johnson, L.R. (2002). Order Zygnematales. In The Freshwater Algal Flora of the British Isles: An Identification Guide to Freshwater and Terrestrial Algae (John, D.M., Whitton, B.A. & Brook, A.J., editors), 479–593. Cambridge University Press, Cambridge.
- Burke, C.N. & Geiselman, C.W. (1971). Extract anhydride epoxy percentages for electron microscopy embedding (Epon). Journal of Ultrastructure Research, 36: 119–126.
- Caballero-Miranda, M. (1996). The diatom flora of two acid lakes in central México. Diatom Research, 11: 227–240.
- Carrillo, P., Delgado-Molina, J.A., Medina-Sánchez, J.M., Bullejos, F.J. & Villar-Argaiz, M. (2007). Phosphorus inputs unmask negative effects of ultraviolet radiation on algae in a high mountain lake. Global Change Biology, 14: 423–439.
- Catalán, J., Camarero, L., Felip, M., Pla, S., Ventura, M., Buchaca, T., Bartumeus F., Miró, A., Casamayor, E.O., Medina-Sánchez, J.M., Bacardit, M., Altuna, M., Bartons, M. & Díaz de Quijano, D. (2006). High mountain lakes: extreme habitats and witnesses of environmental changes. Limnetica, 25: 551–584.
- Chen, Z. & Zhou, Z. (1980). Studies on the Zygnemataceae I. Oceanologia et Limnologia Sínica, 11: 285–286, 291–292.
- Cuna, E., Zawisza, E., Caballero, M., Ruiz-Fernández, A.C., Lozano-García, S. & Alcocer, J. (2014). Environment impacts of Little Ice Age cooling in central Mexico recorded in the sediments of a tropical alpine lake. Journal of Paleolimnology, 51: 1–14.
- Dimas-Flores, D.N., Alcocer, J. & Ciros, P.J. (2008). The structure of the zooplankton assemblages from two neighboring tropical high mountain lakes. Journal of Freshwater Ecology, 23: 21–31.
- Figueroa, F.L., Korbee, N., Carrillo, P., Medina-Sánchez, J.M., Mata, M., Bonomi, J. & Sánchez-Castillo, P.M. (2009). The effects of UV radiation on photosynthesis estimated as chlorophyll fluorescence in Zygnemopsis decussata (Chlorophyta) growing in a high mountain lake (Sierra Nevada, Southern Spain). Journal of Limnology, 68: 206–216.
- Gauthier-Lièvre, L. (1965). Zygnémacées africaines. Beiheft zur Nova Hedwigia, 20: 1–210.
- Geider, R.J. & La Roche, J. (2002). Redfield revisited: variability of C:N:P in marine microalgae and its biochemical basis. European Journal of Phycology, 37: 1–17.
- Gontcharov, A.A. (2008). Phylogeny and classification of Zygnematophyceae (Streptophyta): current state of affairs. Fottea, 8: 87–104.
- Gontcharov, A.A. & Melkonian, M. (2010). Molecular phylogeny and revision of the genus Netrium (Zygnematophyceae, Streptophyta): Nucleotaenium gen. nov. Journal of Phycology, 46: 346–362.
- Gontcharov, A.A., Marin, B. & Melkonian, M. (2004). Are combined analyses better than single gene phylogenies? A case study using SSU rDNA and rbcL sequence comparisons in the Zygnematophyceae (Streptophyta). Molecular Biology and Evolution, 21: 612–624.
- Graham, J.M., Arancibia-Avila, P. & Graham, L.E. (1996). Effects of pH and selected metal on growth of the filamentous green algae Mougeotia under acidic conditions. Limnology and Oceanography, 41: 263–270.
- Guiry, M.D. & Guiry, G.M. (2019). Algaebase. World-wide electronic publication, National University of Ireland, Galway. Available at htpp://www.algasebase.org.
- Herburger, K., Lewis, L.A. & Holzinger, A. (2015). Photosynthetic efficiency, desiccation tolerance and ultrastructure in two phylogenetically distinct strains of alpine Zygnema sp. (Zygnematophyceae, Streptophyta): role of pre-akinete formation. Protoplasma, 252: 571–589.
- Holzinger, A. & Lütz, C. (2006). Algae and UV irradiation: effects on ultrastructure and related metabolic functions. Micron, 37: 190–207.
- Holzinger, A., Tschaikner, A. & Remias, D. (2010). Cytoarchitecture or the desiccation-tolerant green alga Zygogonium ericetorum. Protoplasma, 243: 15–24.
- Hoshaw, R.W. (1983). Systematics of the Zygnemataceae (Chlorophyceae). III. Transeau Collection of the Zygnemataceae: An Old Collection with Modern Uses. American Journal of Botany, 70: 145–153.
- Kadlubowska, J.Z. (1984). Chlorophyta 8. Conjugatophyceae I: Zygnemales. In Süsswasserflora von Mitteleuropa (Ettl, H., Gerloff, J., Heynig, H. & Mollenhauer, D., editors), 11–137. Gustav Fischer Verlag, Jena.
- Katana, A., Kwiatowski, J., Spalik, K., Zakrys, B., Szalacha, E., Szymanska, H. (2001). Phylogenetic position of Koliella (Chlorophyta) as inferred from nuclear and chloroplast small subunit rDNA. Journal of Phycology, 37: 443–451.
- Kenneth, G.K., McCourt, R.M., Cimino, M.T. & Delwiche, C.F. (2001). The closest living relatives of land plants. Science, 294: 2351–2353.
- Kim, J.H., Boo, S.M. & Kim, Y.H. (2012). Morphology and plastid psbA phylogeny of Zygnema (Zygnemataceae, Chlorophyta) from Korea: Z. insigne and Z. leoispermum. Algae 27: 225–234.
- Lasso, C.A., Gutiérrez, F. de P. & Morales, B.D. (Editors) (2014). X. Humedales interiores de Colombia: identificaciÓn, caracterización y establecimiento de límites según criterios biológicos y ecológicos. Serie Editorial Recursos Hidrobiológicos y Pesqueros Continentales de Colombia. Instituto de Investigación de Recursos Biológicos Alexander von Humboldt (IAvH), Bogotá, D.C.
- McCourt, R.M., Karol, K.G., Bell, J., Helm-Bychowski, K.M., Grajewska, A., Wojciechowski, M.F. & Hoshaw. R.W. (2000). Phylogeny of the conjugating green algae (Zygnemophyceae) based on rbcL sequences. Journal of Phycology, 36: 747–758.
- Mehlich, A. (1984). Mehlich-3 soil test extractant: a modification of Mehlich-2 extractant. Communications in Science Plant Analysis, 15: 1409–1416.
- Muntean, E., Bercea, V., Dragos, N. & Muntean, N. (2007). Potential use of Mougeotia sp. algae in food production, based on its carotenoid content. Journal of Agroalimentary Processes and Technologies, 13: 143–148.
- Novelo, E. & Tavera, R.L. (2011). A graphic panorama of the freshwater algae from Mexico. Hidrobiologica, 21: 333–341.
- Othman, R., Hidayah, N.N., Mohd Hatta, F.A. & Jamaludin, M.A. (2018). Natural carotenoid pigments of six Chlorophyta green algae species. Journal of Pharmacy and Nutrition Sciences, 8: 1–15.
- Ortega, M.M. (1984). Catalog of Recent Continental Algae from Mexico. UNAM, Mexico.
- Panikkar, M.V.N. & Ampili, P. (1988). Temnogametum keralense, a new species from South India. Journal of Economic and Taxonomic Botany, 12: 397–400.
- Panikkar, M.V.N. & Ampili, P. (1991). Species of Temnogametum W. & G.S. West from Kerala, South India. Geobios New Reposts, 10: 117–120.
- Pichrtová, M., Remias, D., Lewis, L.A. & Holzinger, A. (2013). Changes in phenolic compounds and cellular ultrastructure of Arctic and Antarctic strains of Zygnema (Zygnematophyceae, Streptophyta) after exposure to experimentally enhanced UV to PAR ratio. Microbial Ecology, 65: 68–83.
- Pichrtová, M., Holzinger, A., Kulichová, J., Ryšánek, D., Šoljaková, T., Trumhová, K. & Nemcova, Y. (2018). Molecular and morphological diversity of Zygnema and Zygnemopsis (Zygnematophyceae, Streptophyta) from Svalbard (High Arctic). European Journal of Phycology, 53: 492–508.
- Poulicková, A., Žižka, Z., Hašler, P. & Benada, O. (2007). Zygnematalean zygospores: morphological features and use in species identification. Folia Microbiology, 52: 135–145.
- Prescott, G.W. (1947). New Zygnemataceae from Ecuador. Ohio Science, 47: 130–132.
- Ralph, A.L. (1962). Physiology and Biochemistry of Algae. Academic Press, New York.
- Randhawa, M.S. (1959). Zygnemataceae. Indian Council of Agricultural Research, New Delhi.
- Remias, D. & Lutz, C. (2007). Characterization of esterified secondary carotenoids and of their isomers in green algae: a HPLC approach. Algological Studies, 124: 85–94.
- Remias, D., Holzinger, A., Aigner, S. & Lütz, C. (2011). Ecophysiology and ultrastructure of Ancylonema nordenskiöldii (Zygnematales, Streptophyta), causing brown ice on glaciers in Svalbard (high Arctic). Polar Biology, 35: 899–908.
- Ronquist, F. & Huelsenbeck, J.P. (2003). MrBayes 3.0: Bayesian phylogenetic inference under mixed models. Bioinformatics, 19: 1572–1574.
- Sommaruga, R. (2001). The role of solar UV radiation in the ecology of alpine lakes. Journal of Photochemistry and Photobiology B: Biology, 62: 35–42.
- Southworth, D. (1973). Cytochemical reactivity of pollen walls. Journal of Histochemistry and Cytochemistry, 21: 73–80.
- Staay, S.Y.M.V., De Wachter, R. & Vaulot, D. (2001). Oceanic 18S rDNA sequences from picoplankton reveal unsuspected eukaryotic diversity. Nature, 409: 607–610.
- Stancheva, R., Sheath, R.G. & Hall, J.D. (2012). Systematics of the genus Zygnema (Zygnematophyceae, Charophyta) from Californian watersheds. Journal of Phycology, 48: 409–422.
- Stancheva, R., Hall, J.D., Herburger, K., Lewis, L.A., McCourt, R.M., Sheath, R.G. & Holzinger, A. (2014). Phylogenetic position of Zygogonium ericetorum (Zygnemataceae, Charophyta) from a high alpine habitat and ultrastructural characterization of unusual aplanospores. Journal of Phycology, 50: 790–803.
- Stancheva, R., Herburger, K., Sheath, R.G. & Holzinger, A. (2016). Conjugation morphology of Zygogonium ericetorum (Zygnematophyceae, Charophyta) from a high alpine habitat. Journal of Phycology, 52: 131–134.
- Tapolczai, K., Anneville, O., Padisák, J., Salmaso, N., Morabito, G., Zohary, T., Tadonléké, R.D. & Rimet, F. (2015). Occurrence and mass development of Mougeotia spp. (Zygnemataceae) in large, deep lakes. Hydrobiologia, 745: 17–29.
- Thiers, B. (2020). Index Herbariorum. A Global Directory of Public Herbaria and Associated Staff. New York Botanical Garden’s Virtual Herbarium. Available at: http://sweetgum.nybg.org/science/ih.
- Transeau, E.N. (1932). The genus Temnogametum. Ohio Journal of Science, 32: 487–491.
- Trenty, A., Groling, F., Magdowski, G. & Wagner, G. (1992). Electron microscopic characterization of calcium-biding physodes in the green alga Mougeotia scalaris. Histochemistry, 97: 487–492.
- Vilaclara, G., Cuna, E. & Zeeb, B. (2005). Subfossil Chrysophyte morphotypes from two tropical high mountain lakes in Nevado de Toluca volcano, central Mexico. Beihefte zur Nova Hedwigia, 128: 309–335.
- Wei, Y.X. & Ying-Kit, Y. (2000). Reproduction of five species of Zygnemataceae (Chlorophyta) in Ontario lakes, Canada. Algological Studies, 98: 91–108.
- Wei, Y.X., Yung-Kit, Y., Jackson, M.B. & Sawa, T. (1989). Some Zygnemataceae (Chlorophyta) of Ontario, Canada including descriptions of two new species. Canadian Journal of Botany, 67: 3233–3247.
- West, W. & West, G.S. (1897). Welwitsch’s African freshwater algae. Journal of Botany, British and Foreign, 35: 1–7, 33–42, 77–89, 113–122, 172–183, 235–243, 264–272, 297–304.
- Yamagishi, T. (1963). New species of the Zygnemataceae collected in Japan. Botany Magazine Tokyo, 76: 215–218.
- Zohary, T., Alster, A., Hadas, O. & Obertegger, U. (2019). There to stay: invasive filamentous green alga Mougeotia in Lake Kinneret, Israel. Hydrobiologia, 831: 87–100.