Abstract
Transmembrane fluxes are major determinants of several enzyme activities localized in the luminal compartment of the endoplasmic reticulum (ER). Although a large number of metabolites were shown to be transported across the ER membrane, only a few transporters have been identified so far. It can be assumed that the basal permeability of ER membrane vesicles (microsomes) to a variety of small molecules is due to the presence of a low-selectivity channel or pore rather than many strictly specific transporters. The translocon complex is a possible candidate for this role because it transitionally forms an open channel in the ER membrane and an increasing amount of evidence shows the permeation of small compounds through this channel. It seems plausible that the translocon pore is not only responsible for inward and outward peptide translocation but also contributes to basal Ca2+ leakage from the ER and ensures the substrate supply for certain luminal ER enzymes.
Introduction
The endoplasmic reticulum (ER) membrane has been frequently reported to be generally permeable to small compounds, and hence its barrier function has been repeatedly questioned. ER-derived microsomal vesicles, indeed, exhibit a slow basal permeability to a variety of structurally unrelated compounds, yet only a few specific transporters have been identified so far. Nevertheless, the reported size- and charge-dependence of the basal permeability (Nilsson et al. [Citation1973], Meissner & Allen [Citation1981]) contradicts the passive diffusion through the lipid bilayer and indicates the existence of one or more pores/channels in the ER membrane. Recent observations reviewed here strongly suggest that translocon protein channel can be one of the possible candidates to fill this role.
The features of the translocon peptide channel
In eukaryotic cells, secretory and membrane proteins are synthesized on rough ER (RER) surface and transported across or integrated into the membrane by the protein conducting channel translocon (for a review see Osborne et al. [Citation2005]). This aqueous pore is a heterotrimeric protein complex composed of Sec61αβγ subunits. Several associated proteins such as SR (signal recognition particle receptor), TRAM (translocation-associated membrane protein) and TRAP (translocon-associated protein) have also been reported to take part in the transport process. Besides the typical cotranslational protein translocation into the ER lumen, this pore can also participate in posttranslational translocation and retrotranslocation.
The existence of a membrane spanning aqueous channel in the ER membrane was first demonstrated by conductivity measurements. Addition of puromycin – a tRNA analogue antibiotic which arrests translation and releases the nascent chain from the ribosome – caused a large increase in the membrane conductance (see A). The ion flow could then be abolished by the removal of the ribosomes from the membrane surface (see C). It was, therefore, concluded that an ion-conducting channel remains after the translocon is freed of the nascent polypeptide chain and it persists in open conformation as long as ribosomes are attached (Simon & Blobel [Citation1991]).
Figure 1. The three states of ribosome-translocon complex. (A) The ribosome-translocon channel is occupied by the polypeptide chain being translocated. (B) After the completion of translocation, the polypeptide leaves the channel empty. In this state, the complex allows the passage of small molecules. (C) The pore is closed again once the ribosome has been released from the translocon.
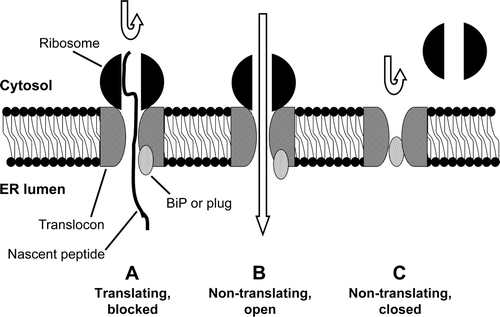
The aqueous environment of secretory proteins undergoing translocation across the ER membrane was also confirmed by incorporating water-sensitive fluorescent probes into nascent chains during translation. The probes were in an aqueous milieu as shown both by their fluorescence lifetimes and by collisional quenching of their fluorescence by iodide ions introduced into the ER lumen (Crowley et al. [Citation1994]). Fluorescent quenchers were also applied to determine the size of the pore, and it was shown that I- or even NAD can enter the channel during the translocation of the polypeptide (Hamman et al. [Citation1997]). Therefore, it can be concluded that translocon is the largest pore in the ER membrane, with an estimated diameter of 40–60 Å in the ribosome bound state and a smaller diameter of 9–15 Å in the ribosome free state (Johnson & van Waes [Citation1999]). The structure of the Sec61 pore revealed recently by X-ray crystallography suggests a smaller size; the maximum dimensions measured this way are 15×20 Å (van den Berg et al. [Citation2004]). Recent reports demonstrated the persistent binding of ribosomes after termination of translocation. It has been reported that approximately two-thirds of the ribosomal subunits remain bound to the translocons after the normal completion of protein translation (Potter & Nicchitta [Citation2002]). Snapp et al. examined the fate of translocon during the transportation cycle, using a method based on fluorescence resonance energy transfer (FRET) to probe the relative proximities of the channel components. These studies revealed that the constituents remain assembled, and have a similar organization both during and after translocating the nascent chain (Snapp et al. [Citation2004]).
Recent data demonstrate the association of ribosomes to oligomeric assemblies of Sec61 protein. Electron cryo-microscopy showed that membrane-bound ribosomes were anchored to Sec61-tetramers (Menetret et al. [Citation2005]). A free Sec61 population was also found in a lower oligomeric state linked weakly to non-translating ribosomes (Schaletzky & Rapoport [Citation2006]), and there are other data about the connection of Sec61 complex and ribosomes lacking nascent peptide chain (Kalies et al. [Citation1994]). Similarly to eukaryotic Sec61, bacterial-archaeal SecY complexes can be present in oligomeric form. Although the translocation pore is formed by a single copy of the SecY complex, the translocation has been recently shown to be mediated by its oligomers (Osborne & Rapoport [Citation2007]). The role of oligomerization underneath the ribosome can be the facilitation of interactions with channel partners (ribosome, SecA etc.); oligomers may provide more linkages (Osborne et al. [Citation2005]). It can be supposed that oligomerization affects the permeability barrier either in translocating or non-translocating complexes; however, the oligomerization state was not investigated in the studies focusing on the role of translocon in non-specific transport. These data indicate that the ER membrane might contain a large number of ribosome-bound translocon with their pores unoccupied by polypeptides after the completed translocation. In this state, when the channel is kept open by the ribosome but its lumen is empty, the complex seems to allow the passage of small molecules (B).
Maintaining the permeability barrier
The large diameter of ribosome-translocon complexes raises the question how the membrane barrier is maintained during translocation. Two models have been proposed to explain this problem. The first theory was based on the results of functional studies. When fluorescent molecules were incorporated into the polypeptide chain, the quencher I− ions could not reach them during translocation. It has been concluded that the tight ribosome-translocon junction can prevent the permeation of small molecules from the cytoplasmic side. It was also demonstrated that BiP, a prominent ER chaperone blocks the luminal side of the pore in the case of either translocating or non-translocating translocons (Hamman et al. [Citation1998]). When the length of the nascent chain reaches ∼70 amino acids, BiP is released and the luminal end of the pore is opened (Crowley et al. [Citation1994]). The sealing process requires ADP-bound conformation of this chaperone, and the reopening of the pore requires a conformational change induced by ATP binding. Pore closure involves functional interactions between both the substrate-binding region and the J domain-binding region of BiP and membrane proteins (Alder et al. [Citation2005]). The role of the ribosome as an outer and BiP as an inner gatekeeper does not explain how the membrane barrier is maintained during posttranslational translocation (in the absence of the ribosome) or in bacteria (in the absence of BiP) (Rapoport et al. [Citation2004]).
An alternative explanation about the barrier was suggested by structural studies. The image of the protein-conducting channel was provided by the 3,2Å resolution X-ray structure of the detergent solubilized SecY complex from the archaebacterium Methanococcus jannaschii (van den Berg et al. [Citation2004]). Regarding the significant sequence conservation, this structure is likely representative of all species. It was observed that the channel has an hourglass-shape with waist formed by a ring of hydrophobic residues, which is hypothesized to make a seal around the translocating nascent chain. In the closed state, a short helical segment acts as an apparent plug, which is removed by the binding of ribosomes. In this model, the membrane barrier can be maintained by the plug and the nascent chain in all modes of translocation (Osborne et al. [Citation2005]). However, it was observed that the deletion of the plug domain did not affect the viability of yeast (Junne et al. [Citation2006]) or bacteria (Maillard et al. [Citation2007], Li et al. [Citation2007]), which led to the conclusion that plug domain is not essential for sealing the pore, but might have a stabilizing role during translocon formation. The observation revealing that a new helical plug is formed by conformational rearrangements and seals the pore of the plug-deleted translocon explains how the permeability barrier was maintained in the mutant cells (Li et al. [Citation2007]). Investigation of the efficacy of gating showed that SecY channel is impermeable to small molecules such as water and ions. The participation of both the pore ring and the plug was evidenced in maintenance of the barrier (Saparov et al. [Citation2007]).
Both models agree in that the translocon channel together with the ribosome provides a linked aqueous channel and that the polypeptide chain being translocated is the obstacle to the traffic of small molecules.
The role of translocons in Ca2 + leakage
The ER is the largest calcium store in all types of cells, and plays a major role in Ca2 + signalling. Tight regulation of ER Ca2 + concentration is also essential for protein folding and maturation. In resting state, the Ca2 + content of the ER lumen is the result of a balance between the active uptake by the SERCA (sarcoplasmic and ER calcium ATP-ase) pumps and passive efflux or basal leakage (Camello et al. [Citation2002]). This passive calcium leakage can be demonstrated by inhibiting SERCA pumps with thapsigargin (Treiman et al. [Citation1998]), which leads to calcium release from the ER. The mechanism responsible for this phenomenon and the type of the calcium leak channel has been unclear for a long time (Camello et al. [Citation2002]). Several proteins with channel-forming properties have been suggested as potential calcium leak channels. For example, Bcl2 oncogene product have been claimed to be involved in the ER Ca2 + leak (Foyouzi-Youssefi et al. [Citation2000]). However, the experimental results are often contradictory, and the Bcl2 proteins are not constitutively expressed in every cell type, whilst the Ca2 + leak appears to be a general phenomenon (Distelhorst & Shore, [Citation2004]). Very recently, it has been also suggested that pannexin-1 (PanX1) may form Ca2 + -permeable channels in the ER and contribute to the ER Ca2 + leak (Vanden Abeele et al. [Citation2006], Tu et al. [Citation2006]). Pannexin-1, however, is poorly expressed in certain tissues, including the liver, where the phenomenon of calcium leakage is present (Baranova et al. [Citation2004]). There are also conflicting results about presenilin holoproteins as presumable Ca2 + leak channels, and the expression of these proteins in different cell types is also unclear (Zatti et al. [Citation2006]). In skeletal and cardiac muscle sarcoplasmic reticulum, basal leak may occur through the ryanodine receptor (Pessah et al. [Citation1997]). A similar role of other second messenger activated channels (e.g. IP3 receptor) was hypothesized in non-muscle cells (Hofer et al. [Citation1996]).
A growing amount of data indicates that the translocon peptide channel has a remarkable role in the non-specific Ca2 + leakage. Direct luminal Ca2 + measurement in the ER of pancreatic acinar cells demonstrated that the basal Ca2 + leak could be enhanced by puromycin, while it could not be blocked by the antagonists of either the IP3 receptor or the ryanodine receptor or the NAADP receptor (Lomax et al. [Citation2002]). In accordance with this observation, the thapsigargin-induced calcium release from the ER to the cytosol of LNCaP cells could be reduced by puromycin pre-treatment (Van Coppenolle et al. [Citation2004]), which suggests that an increased leakage depleted the ER calcium stores. This effect was not influenced by the inhibition of IP3 or ryanodine receptor channels but could be counteracted by the peptidyl transferase inhibitor anisomycin, which prevents puromycin to release nascent chains from the translocon. It was also found that the thapsigargin- or EGTA-induced loss of ER calcium is dependent on the presence of opened translocon complexes in the ER membrane (Flourakis et al. [Citation2006]). The assumption that translocon complex is a major calcium leak channel in the ER membrane is further supported by microsomal experiments. The passive Ca2 + efflux was shown to be heterogenous in rat liver microsomes: one component required counter ion influx and was inhibited by Gd3 + and La3 + , the other component was stimulated by puromycin (Giunti et al. [Citation2007]). Collectively, the remarkable contribution of translocon to Ca2 + leakage is clearly demonstrated; nevertheless the involvement of a putative cation channel can also be assumed.
SOCE (store-operated calcium entry) was shown to be triggered by both EGTA-evoked and thapsigargin-evoked Ca2 + leakage through the translocon complex (Flourakis et al. [Citation2006]). Moreover, SOCE could be initiated by increasing the number of opened translocon complexes with pactamycin (Ong et al. [Citation2007]). This antibiotic, which blocks the initiation of translation can represent what happens when a stop codon is reached and peptide synthesis is terminated physiologically, in contrast to the effect of puromycin, which causes the premature release of the translocated nascent peptides. The Ca2 + leakage and the induction of SOCE by either thapsigargin or pactamycin were prevented by emetine, which increases the number of closed ribosome-translocon complexes by the stabilization of ribosome-nascent chain complexes (Ong et al. [Citation2007]). Beyond the demonstration of passive calcium efflux through the translocon, these data also suggest a functional coupling between SOCE and protein synthesis to maintain ER Ca2 + within the normal range.
Permeation of small, neutral molecules through the peptide channel
The hydrolysis of 4-methylumbelliferyl-α-D-glucopyranoside is catalyzed by an ER resident α-glucosidase, hence is dependent on its entry into the ER. The reaction was found to be affected by antibiotics modulating protein synthesis in broken Chinese hamster ovary-S cells. The hydrolysis was enhanced when the number of empty ribosomes was increased either by the premature release of nascent proteins with puromycin or by the inhibition of translation initiation with pactamycin (Heritage & Wonderlin [Citation2001], Roy & Wonderlin, [Citation2003]). The effect of these antibiotics was abolished by high-salt treatment, which releases ribosomes from the ER membrane (Heritage & Wonderlin [Citation2001]), and by cycloheximide an elongation and release inhibitor antibiotic (Roy & Wonderlin [Citation2003]), respectively. These findings led to the conclusion that translocons are permeable to this neutral molecule as long as empty ribosomes remain bound to them.
Transport measurements in subfractions of rat liver microsomes demonstrated the permeability of translocon channel to sucrose. Sucrose is not metabolized in the lumen of the ER, so it presumably does not have specific transporter and its transmembrane traffic can represent non-specific transport processes. A significant sucrose efflux was demonstrated in rough but not in smooth microsomal subfraction (Giunti et al. [Citation2007]). Moreover, puromycin increased the permeability of non-fractionated microsomal vesicles to sucrose, while it was ineffective in smooth microsomes (Lizák et al. [Citation2006]), which strongly supports the involvement of the translocon.
Permeation of charged molecules through the translocon
Electrophysiological studies demonstrated that charged molecules like glutamate and HEPES can permeate the translocon channel. Pancreatic rough microsomes were fused to one side of a planar bilayer and the membrane conductance was found to be largely increased by the addition of puromycin (Simon & Blobel [Citation1991]). Contribution of translocon peptide channel to the permeation of low molecular mass anions was further investigated in rat liver microsomes. Puromycin increased both UDP-glucuronosyltransferase and mannose-6-phosphatase activities in intact microsomes, which was likely due to the better access of the substrates UDP-glucuronic acid and mannose-6-phosphate, respectively, to the luminal enzymes (Lizák et al. [Citation2006]). It should be noted that mannose-6-phosphate does not have a specific transporter in the ER. This effect of puromycin could be prevented by anisomycin pretreatment or by an antibody against Sec61 translocon component. In accordance with the enzyme activity measurements, direct transport assays showed that puromycin stimulates the uptake of UDP-glucuronic acid (but not glutathione and glucose-6-phosphate) in microsomal vesicles, while it is ineffective in the smooth-ER-derived microsomal vesicles, which are poor in translocon peptide channels (Lizák et al. [Citation2006]). These findings indicate that translocons can contribute to the substrate supply of certain intraluminal enzymes.
The role of translocon was hypothesized (but not proved) in the transport of various xenobiotics, FAD (Tu & Weissman, [Citation2002]) and exogenous biotin derivatives (Le Gall et al. [Citation2004]).
Conclusions
A unique property of the ER membrane is the relative permeability to various low molecular weight compounds. This could be explained by the relatively less ordered structure of the ER membrane due to its remarkably lower cholesterol and higher protein content in comparison to the plasma membrane (Papahadjopoulos et al. [Citation1972]). However, the permeability of the ER membrane is definitely selective with respect to the size and, to a lesser extent, to the charge of the transported solutes, which corresponds with the transport through channels/pores rather than with simple diffusion. The repeatedly observed difference between the permeability of rough and smooth ER membranes (Meissner & Allen, [Citation1981], Lizák et al. [Citation2006], Giunti et al. [Citation2007]) suggests the role of translocon complex. The more pronounced leakiness of the RER membrane is in accordance with the abundance of translocon protein channels having an average diameter of approximately 20 Å, which is sufficiently wide to allow the traffic of Ca2 + and small water-soluble molecules. Indeed, the passage of ions and small molecular weight compounds through the translocon was demonstrated.
The increased permeability of the ER membrane due to the presence of translocons cannot be simply regarded as imperfection. The main metabolic functions of the ER are tightly linked to the transmembrane fluxes of substrates and products. Although the number of identified and functionally characterized proteins is increasing, the conspicuous discrepancy between the abundance of functionally characterized transport processes and the handful transporters identified at molecular level has remained. This circumstance might suggest that translocon (and perhaps other ER channels) can be involved in the substrate supply of at least some luminal enzymes. Additionally, it can ensure the supply of recyclable cofactors of intraluminal enzymes (e.g. pyridine nucleotides, FAD, etc.). Several transport processes across the ER membrane were proven or hypothesized to be based on antiport mechanism (for a review see Csala et al. [Citation2007]), which require the appropriate counter-molecules, potentially provided by the slow flux through the translocon. The large amount of evidence supporting the role of translocon in transmembrane fluxes is partially provided by studies in which puromycin or related agents were applied to open the channel. The effectiveness of these compounds has been demonstrated in both microsomal and cellular systems. Although other sites of action can be also hypothesized, presently there are no other experimentally well-grounded suggestions for the mechanism of the increased permeability evoked by puromycin than the opening of the translocon. However, the in vivo contribution of the translocon to the ER permeability is still arguable. The pore is occupied during translation by the peptide chain being polymerized and is blocked by BiP, a prominent intraluminal chaperone when no ribosome is associated to the complex. Nevertheless, recent findings show that the dissociation of ribosomes from translocon complexes is delayed after the termination of protein synthesis. There is overwhelming evidence that the rough ER contains at least two classes of ribosomes: the ones undergoing co-translational translocation in form of polysomes and the ones that have finished protein synthesis (Neuhof et al. [Citation1998], Potter et al. [Citation2001]). It was also observed that during the ER stress dependent inhibition of translation ribosomes remained bound to the ER (Stephens et al. [Citation2005]). Moreover, in vitro experiments showed that non-translating ribosomes devoid of nascent peptides can be attached to microsomal vesicles, which indicates that an intrinsic affinity between the ribosome and the ER membrane is strong enough to keep the structure of the rough ER intact even in case of inactive translation (see Wu et al. [Citation2007] and references therein). It is not known, however, to what extent and how long the ribosomes remain on the ER after translation has been terminated. It is also a matter of debate whether the non-translating and functioning ribosomes occupy the same position on the ER surface. The questions related to the physiological situation are also valid in case of puromycin-terminated translation.
In conclusion, several data indicate that the presence of the translocon is a major determinant of the permeability properties of the ER membrane. When a non-translating ribosome is associated to the translocon complex, they form a transitional low-selectivity channel between the cytosol and the ER lumen. Non-translating ER-attached ribosomes therefore may form a structural basis for the transport of small molecules with limited specificity in the ER.
Acknowledgements
OTKA (National Scientific Research Fund) F46740, T48939 and TS 049851, Ministry of Education (NKFP 1A/056/2004), Ministry of Welfare (ETT 182 and 183/2006), Szentágothai János Knowledge Center (RET) and The Italian Ministry of University and Research (Grant No. RBAU014PJA) provided support. The two research groups have a Bilateral Hungarian–Italian Intergovernmental S&T Cooperation Grant.
References
- Alder NN, Shen Y, Brodsky JL, Hendershot LM, Johnson AE. The molecular mechanisms underlying BiP-mediated gating of the Sec61 translocon of the endoplasmic reticulum. J Cell Biol 2005; 168: 389–399
- Baranova A, Ivanov D, Petrash N, Pestova A, Skoblov M, Kelmanson I, Shagin D, Nazarenko S, Geraymovych E, Litvin O, Tiunova A, Born TL, Usman N, Staroverov D, Lukyanov S, Panchin Y. The mammalian pannexin family is homologous to the invertebrate innexin gap junction proteins. Genomics 2004; 83: 706–716
- Camello C, Lomax R, Petersen OH, Tepikin AV. Calcium leak from intracellular stores – the enigma of calcium signalling. Cell Calcium 2002; 32: 355–361
- Crowley KS, Liao S, Worrell VE, Reinhart GD, Johnson AE. Secretory proteins move through the endoplasmic reticulum membrane via an aqueous, gated pore. Cell 1994; 78: 461–471
- Csala M, Marcolongo P, Lizák B, Senesi S, Margittai É, Fulceri R, Magyar JÉ, Benedetti A, Bánhegyi G. Transport and transporters in the endoplasmic reticulum. Biochim Biophys Acta 2007; 1768: 1325–1341
- Distelhorst CW, Shore GC. Bcl-2 and calcium: controversy beneath the surface. Oncogene 2004; 23: 2875–2880
- Flourakis M, Van Coppenolle F, Lehen'kyi V, Beck B, Skryma R, Prevarskaya N. Passive calcium leak via translocon is a first step for iPLA2-pathway regulated store operated channels activation. FASEB J 2006; 20: 1215–1217
- Foyouzi-Youssefi R, Arnaudeau S, Borner C, Kelley WL, Tschopp J, Lew DP, Demaurex N, Krause KH. Free in PMC Bcl-2 decreases the free Ca2 + concentration within the endoplasmic reticulum. Proc Natl Acad Sci USA 2000; 97: 5723–5728
- Giunti R, Gamberucci A, Fulceri R, Bánhegyi G, Benedetti A. Both translocon and a cation channel are involved in the passive Ca2 + leak from the endoplasmic reticulum: a mechanistic study on rat liver microsomes. Arch Biochem Biophys 2007; 462: 115–121
- Hamman BD, Chen JC, Johnson EE, Johnson AE. The aqueous pore through the translocon has a diameter of 40–60 A during cotranslational protein translocation at the ER membrane. Cell 1997; 89: 535–544
- Hamman BD, Hendershot LM, Johnson AE. BiP maintains the permeability barrier of the ER membrane by sealing the lumenal end of the translocon pore before and early in translocation. Cell 1998; 92: 747–758
- Heritage D, Wonderlin WF. Translocon pores in the endoplasmic reticulum are permeable to a neutral, polar molecule. J Biol Chem 2001; 276: 22655–22662
- Hofer AM, Curci S, Machen TE, Schulz I. ATP regulates calcium leak from agonist-sensitive internal calcium stores. FASEB J 1996; 10: 302–308
- Johnson AE, van Waes MA. The translocon: a dynamic gateway at the ER membrane. Annu Rev Cell Dev Biol 1999; 15: 799–842
- Junne T, Schwede T, Goder V, Spiess M. The plug domain of yeast Sec61p is important for efficient protein translocation, but is not essential for cell viability. Mol Biol Cell 2006; 17: 4063–4068
- Kalies KU, Gorlich D, Rapoport TA. Binding of ribosomes to the rough endoplasmic reticulum mediated by the Sec61p-complex. J Cell Biol 1994; 126: 925–934
- Le Gall S, Neuhof A, Rapoport T. The endoplasmic reticulum membrane is permeable to small molecules. Mol Biol Cell 2004; 15: 447–455
- Li W, Schulman S, Boyd D, Erlandson K, Beckwith J, Rapoport TA. The plug domain of the SecY protein stabilizes the closed state of the translocation channel and maintains a membrane seal. Mol Cell 2007; 26: 511–521
- Lizák B, Czegle I, Csala M, Benedetti A, Mandl J, Bánhegyi G. Translocon pores in the endoplasmic reticulum are permeable to small anions. Am J Physiol Cell Physiol 2006; 291: C511–C517
- Lomax RB, Camello C, Van Coppenolle F, Petersen OH, Tepikin AV. Basal and physiological Ca2 + leak from the endoplasmic reticulum of pancreatic acinar cells. Second messenger-activated channels and translocons. J Biol Chem 2002; 277: 26479–26485
- Maillard AP, Lalani S, Silva F, Belin D, Duong F. Deregulation of the SecYEG translocation channel upon removal of the plug domain. J Biol Chem 2007; 282: 1281–1287
- Meissner G, Allen R. Evidence for two types of rat liver microsomes with differing permeability to glucose and other small molecules. J Biol Chem 1981; 256: 6413–6422
- Menetret JF, Hegde RS, Heinrich SU, Chandramouli P, Ludtke SJ, Rapoport TA, Akey CW. Architecture of the ribosome-channel complex derived from native membranes. J Mol Biol 2005; 348: 445–457
- Neuhof A, Rolls MM, Jungnickel B, Kalies KU, Rapoport TA. Binding of signal recognition particle gives ribosome/nascent chain complexes a competitive advantage in endoplasmic reticulum membrane interaction. Mol Biol Cell 1998; 9: 103–115
- Nilsson R, Peterson E, Dallner G. Permeability of microsomal membranes isolated from rat liver. J Cell Biol 1973; 56: 762–776
- Ong HL, Liu X, Sharma A, Hegde RS, Ambudkar IS. Intracellular Ca2 + release via the ER translocon activates store-operated calcium entry. Pflugers Arch 2007; 453: 797–808
- Osborne AR, Rapoport TA, van den Berg B. Protein translocation by the Sec61/SecY channel. Annu Rev Cell Dev Biol 2005; 21: 529–550
- Osborne AR, Rapoport TA. Protein translocation is mediated by oligomers of the SecY complex with one SecY copy forming the channel. Cell 2007; 129: 97–110
- Papahadjopoulos D, Nir S, Oki S. Permeability properties of phospholipid membranes: effect of cholesterol and temperature. Biochim Biophys Acta 1972; 266: 561–583
- Pessah IN, Molinski TF, Meloy TD, Wong P, Buck ED, Allen PD, Mohr FC, Mack MM. Bastadins relate ryanodine-sensitive and -insensitive Ca2 + efflux pathways in skeletal SR and BC3H1 cells. Am J Physiol 1997; 272: C601–C614
- Potter MD, Nicchitta CV. Endoplasmic reticulum-bound ribosomes reside in stable association with the translocon following termination of protein synthesis. J Biol Chem 2002; 277: 23314–23320
- Potter MD, Seiser RM, Nicchitta CV. Ribosome exchange revisited: a mechanism for translation-coupled ribosome detachment from the ER membrane. Trends Cell Biol 2001; 11: 112–115
- Rapoport TA, Goder V, Heinrich SU, Matlack KE. Membrane-protein integration and the role of the translocation channel. Trends Cell Biol 2004; 14: 568–575
- Roy A, Wonderlin WF. The permeability of the endoplasmic reticulum is dynamically coupled to protein synthesis. J Biol Chem 2003; 278: 4397–4403
- Saparov SM, Erlandson K, Cannon K, Schaletzky J, Schulman S, Rapoport TA, Pohl P. Determining the conductance of the SecY protein translocation channel for small molecules. Mol Cell 2007; 26: 501–509
- Schaletzky J, Rapoport TA. Ribosome binding to and dissociation from translocation sites of the endoplasmic reticulum membrane. Mol Biol Cell 2006; 17: 3860–3869
- Simon SM, Blobel G. A protein-conducting channel in the endoplasmic reticulum. Cell 1991; 65: 371–380
- Snapp EL, Reinhart GA, Bogert BA, Lippincott-Schwartz J, Hegde RS. The organization of engaged and quiescent translocons in the endoplasmic reticulum of mammalian cells. J Cell Biol 2004; 164: 997–1007
- Stephens SB, Dodd RD, Brewer JW, Lager PJ, Keene JD, Nicchitta CV. Stable ribosome binding to the endoplasmic reticulum enables compartment-specific regulation of mRNA translation. Mol Biol Cell 2005; 16: 5819–5831
- Treiman M, Caspersen C, Christensen SB. A tool coming of age: thapsigargin as an inhibitor of sarco-endoplasmic reticulum Ca(2 + )-ATPases. Trends Pharmacol Sci 1998; 19: 131–135
- Tu BP, Weissman JS. The FAD- and O2-dependent reaction cycle of Ero1-mediated oxidative protein folding in the endoplasmic reticulum. Mol Cell 2002; 10: 983–994
- Tu H, Nelson O, Bezprozvanny A, Wang Z, Lee SF, Hao YH, Serneels L, De Strooper B, Yu G, Bezprozvanny I. Presenilins form ER Ca2 + leak channels, a function disrupted by familial Alzheimer's disease-linked mutations. Cell 2006; 126: 981–993
- Van Coppenolle F, Vanden Abeele F, Slomianny C, Flourakis M, Hesketh J, Dewailly E, Prevarskaya N. Ribosome-translocon complex mediates calcium leakage from endoplasmic reticulum stores. J Cell Sci 2004; 117: 4135–4142
- Van den Berg B, Clemons WM, Jr, Collinson I, Modis Y, Hartmann E, Harrison SC, Rapoport TA. X-ray structure of a protein-conducting channel. Nature 2004; 427: 36–44
- Vanden Abeele F, Bidaux G, Gordienko D, Beck B, Panchin YV, Baranova AV, Ivanov DV, Skryma R, Prevarskaya N. Functional implications of calcium permeability of the channel formed by pannexin 1. J Cell Biol 2006; 174: 535–546
- Wu WC, Liu HW, Lin A. Human ribosomal protein L7 displays an ER binding property and is involved in ribosome-ER association. FEBS Lett 2007; 581: 651–657
- Zatti G, Burgo A, Giacomello M, Barbiero L, Ghidoni R, Sinigaglia G, Florean C, Bagnoli S, Binetti G, Sorbi S, Pizzo P, Fasolato C. Presenilin mutations linked to familial Alzheimer's disease reduce endoplasmic reticulum and Golgi apparatus calcium levels. Cell Calcium 2006; 39: 539–550