Abstract
P2X receptors are cation selective ion channels gated by the binding of extracellular ATP. Seven subtypes have been identified and they have widespread and overlapping distributions throughout the body. They form homo- and heterotrimeric complexes that differ in their functional properties and subcellular localization. They form part of larger signalling complexes, interacting with unrelated ion channels and other membrane and cytosolic proteins. Up- or down-regulation of their expression is associated with several disease states. This review aims to summarize recent work on the assembly and trafficking of this family of receptors.
Introduction
Extracellular ATP is an important signalling molecule that controls numerous biological processes in the nervous, immune, endocrine, respiratory and cardiovascular systems Citation[1–4]. Its effects are mediated via cell surface P2 receptors of which there are two types: the G protein coupled P2Y receptors and the P2X receptors, which possess an integral cation channel that opens in response to ATP. The P2X receptors represent important therapeutic targets in pain, inflammation and stroke Citation[4], Citation[5]. Seven members of this family, sharing 35–50% amino acid identity, have been identified in vertebrates Citation[6]. These subunits show a widespread and overlapping distribution and are able to coassemble as heteromeric receptors with differing pharmacological properties Citation[7]. Unrelated proteins such as nicotinic and 5-HT receptors and pannexin hemichannels also appear capable of interacting with P2X receptor subunits. The response of P2X receptors is further regulated by controlling the trafficking of receptors to the plasma membrane and intracellular compartments, by the interaction between motifs in specific subtypes and additional accessory proteins.
Physiological roles of P2X receptors
The physiological roles of these receptors have been recently reviewed Citation[1–3] and a summary of what has been confirmed using mice with P2X receptor genes either knocked out or knocked down is provided in in Supplementary data (online version only).
Figure 1. Trafficking of P2X receptors to and from the plasma membrane. The P2X receptor subtypes are broadly classified into four groups based upon their trafficking behaviour. Motifs that have been shown to be determinants of receptor trafficking are indicated. Plasma membrane (1–2), P2X1-7 receptors stabilized by YXXXK motif. Deletions within LPS-binding motif of P2X7, inhibit plasma membrane expression. Endosomes (4–6), P2X4 constitutively internalized from the plasma membrane via dynamin and AP-2 dependent process involving IL and YXXGL motifs. Receptors can be recycled but are predominantly targeted to lysosomes. P2X1,5 and 7 are internalized in response to chronic agonist application. They recycle back to the plasma membrane. Lysosomes (3, 7–9), P2X4 is targeted to lysosomes, protected from degradation and released via exocytosis. P2X4/6 and P2X4/5 assemblies are also targeted here. Delivery to lysosomes may be via a direct pathway from the TGN as well as an indirect pathway via the plasma membrane. ER, P2X1,2,3,5 exported as homo- and heterotrimers. P2X4 rapidly exported as homo- and hetero-trimers. P2X6 retained as a monomer or exported as a heterotrimer. P2X7 exported as a trimer or retained incorrectly assembled.
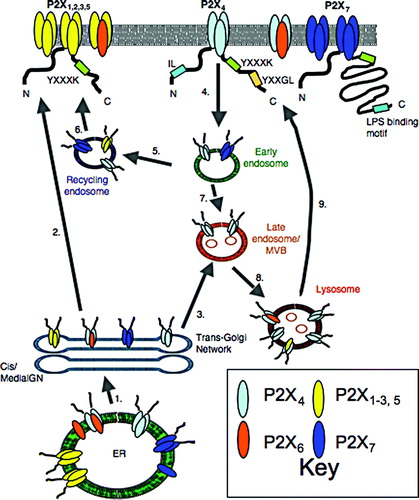
Molecular nature of P2X receptors
The P2X receptors belong to a different structural class to the Cys-loop and glutamate receptor families of ligand gated cation channels Citation[8–10]. Each subunit is between 379–595 amino acids in length, contains two transmembrane (TM) segments, and between 50–70% is contributed by a large extracellular loop that contains 10 disulfide bonds and several N-linked glycosylation sites. The N- and C-termini are cytoplasmic and, in the case of P2X1, 3, 4, 5 and 6, they are both short. P2X2 has a longer C-terminus (120 amino acids) that is involved in interactions with other ligand gated ion channels of the Cys-loop family. P2X7 has a 240 amino acid C-terminal domain that is similarly involved in interactions with other proteins, suggesting that this receptor forms part of a multi-protein signaling complex. As yet, no high-resolution structural information is available for P2X receptors, however this information has recently been obtained for an acid sensing ion channel (ASIC) that shares a similar trimeric architecture with members of the P2X family Citation[11]. Although there is no obvious sequence similarity between these families, in both cases the subunits possess two TM regions, a large extracellular domain of similar length containing multiple disulphide bonds and a pore primarily lined by residues from TM2. The ASIC structure might therefore prove to be a useful model to make predictions about P2X receptor structure-function relationships.
Evidence for trimeric complexes
P2X receptors are multimeric proteins. The first direct evidence for this was the demonstration that P2X2 and P2X3 can coassemble to produce heteromeric channels with functional properties that distinguish them from the parent homomeric receptors Citation[12]. For the majority of multimeric integral membrane proteins, assembly takes place at the endoplasmic reticulum (ER) membrane, and a quality control machinery ensures that only the correctly folded and assembled complexes are exported from the ER. A key role in this process is played by the processing of core glycans that are added at consensus Asn-X-Ser/Thr motifs as soon as the nascent polypeptide chains enter the ER Citation[13].
Several different approaches have been used to determine the stoichiometry of P2X receptors; there is now a consensus that these receptors are trimeric. For many years, however, the issue of stoichiometry was controversial because of a report that when the large extracellular domain of the P2X2 subunit was expressed in Escherichia coli in isolation from the rest of the protein, it assembled to form stable tetramers Citation[14]. These assemblies bound agonist (ATP) and antagonist (suramin), suggesting that they closely resembled the arrangement of the extracellular domains of the intact receptor complex. This now seems unlikely to be the case, and there is evidence to support a role for the transmembrane segments as important determinants of subunit coassembly Citation[15].
The first study to provide evidence for the trimeric structure of recombinant P2X receptors was by Nicke et al. Citation[16]. They purified His-tagged P2X1 and P2X3 receptors expressed in Xenopus oocytes, cross-linked the complexes using bifunctional analogues of the antagonist pyridoxal-phosphate-6-azophenyl-2′,4′-disulfonic acid (PPADS), and analyzed their size by SDS-PAGE. For both receptors, this revealed a single protein band corresponding to a homotrimer and similar results were obtained when the receptor complexes were resolved under non-denaturing conditions. A later study from the same group demonstrated that P2X2, P2X4 and P2X5 similarly form stable homotrimers Citation[17]. In addition to trimers, higher order complexes were also resolved with molecular weights consistent with hexameric and nonameric structures. The stability of these structures was dependent upon the detergent used for solubilization and, unlike the trimers, they were not cross-linked using the short-arm linker glutaraldehyde. This suggests that trimeric assemblies are able to form stable clusters, but subunits belonging to different trimers are further apart within the cluster than subunits within a trimer.
Visualization of individual P2X2 receptors by atomic force microscopy (AFM) has also provided direct evidence for the trimeric nature of these complexes. Barrera et al. Citation[18] imaged receptors adsorbed to poly-L-lysine coated mica and from the particle dimensions they calculated the molecular volume to be 409 nm3, close to that expected for a receptor trimer (473 nm3). In addition, they decorated particles with bound monoclonal anti-His IgG and measured the angle separating two bound antibodies as 123°, very close to the value of 120° predicted for a trimeric receptor. AFM was also used to image purified P2X2 receptors in the aqueous phase Citation[19] and low resolution images of P2X2 receptors obtained using electron microscopy showed structures with a triangular shape Citation[20].
Unlike other members of the P2X receptor family, P2X6 appears not to form stable homotrimers and as a result it is retained in the ER by the quality control machinery Citation[17], Citation[21], Citation[22]. A comparison of P2X6 and P2X2 receptor volumes by AFM showed that the volume of P2X6 particles (145 nm3) was a third of that of P2X2 particles, consistent with it being a monomer Citation[18]. Deleting the uncharged first 14 amino acids of P2X6 or substituting positive or negatively charged residues for serines at positions 3 and 11 was sufficient to increase surface expression of the receptor Citation[23]. The double S3K, S11K mutation was also shown to stabilize the formation of P2X6 homotrimers as measured by the appearance of a second peak in the frequency distribution of molecular volumes. This result suggests that the N-terminus of P2X6 is normally engaged in a hydrophobic interaction which destabilizes assembly of homomeric complexes.
Mechanisms of assembly
P2X receptors assemble in a subunit specific manner and yet little is known about the mechanisms controlling this specificity. No single domain has been identified as the determinant for the compatibility of interactions between subunits. The high resolution structure of the ASIC channel shows extensive subunit-subunit interactions within the trimeric complex, particularly involving a β-sheet region within the ectodomains and both TM domains. The picture that emerges from analysis of deletion mutants of P2X receptors is consistent with this; these studies point to the involvement of TM and extracellular regions but provide no evidence to support a crucial role for N- and C-terminal cytoplasmic regions Citation[21]. There may be differences between the subtypes, for example for P2X2, a mutant lacking the entire TM1 and N-terminal regions co-immunoprecipiated with the full length subunit whereas a mutant that terminated 25 amino acids upstream of TM2 did not, suggesting that TM2 plays a critical role in assembly Citation[15]. In contrast a P2X7 receptor variant lacking the entire C-terminus, TM2 and a distal part of the ectodomain, was able to co-immuonoprecipitate (co-IP) with the full length P2X7 subunit and also to act in a dominant negative manner to inhibit P2X7-receptor mediated responses Citation[24]. These deletion mutants are, however, likely to be imperfectly folded making it difficult to raise firm conclusions on the molecular determinants of subunit assembly.
A splice variant of P2X5 which lacks the C-terminal end of the ectodomain and the outer half of TM2 has a severe assembly defect and is retained within the ER Citation[25]. Insertion of a polyalanine region at this position was sufficient to complement the missing outer portion of TM2 and restore homotrimeric formation and the export of complexes to the cell surface. This argues against the outer portion of TM2 being an important determinant of subunit compatibility and suggests that TM2 is primarily important for tethering the end of the ectodomain to the membrane and restricting its mobility, thus enabling the ER luminal region to engage in intersubunit interactions. Systematic mutagenesis of TM2 indicates only one position, a conserved asparate at position 355, where hydrophobic substitutions are not well tolerated with regards to assembly and ER export of the receptor. This side chain might engage in specific salt-bridge interactions and a possible partner is arginine at position 34 in TM1, which is also conserved in all subtypes except P2X7.
Heteromeric P2X receptor assemblies
The formation of heteromeric P2X receptor complexes alters receptor function, pharmacology and trafficking, thereby increasing the diversity of channels expressed. This has important implications for understanding the physiological roles and the design of therapeutics targeting these receptors. There are many examples in the literature where multiple P2X receptor subtypes have been shown to be endogenously coexpressed and it therefore seems likely that heteromers are of physiological importance. The vast majority of studies that have analysed the properties of heteromeric receptors have, however, been carried out on receptors overexpressed in either Xenopus oocytes or mammalian cell lines. Thus although there are many examples where structural and functional interactions between different subtypes have been shown, it is not known whether the heteromers form predominantly under physiological settings. Below we have summarized the evidence in support of different heteromeric receptors and grouped them according to the nature of the evidence. We have placed the P2X2/3 heteromer in a group of its own because this is the only combination that has clearly been shown to be physiologically relevant. The second group is where there is direct evidence that the two subunits can coassemble around a common conduction pore but this evidence exists only for heterologously over-expressed receptors and this includes P2X2/6, P2X1/2 and P2X1/4. Finally we have subunit combinations for which there is co-IP and functional evidence for an interaction but the formation of heterotrimeric complexes has not been shown directly. For these it is possible that the interaction is between homotrimers rather than within heterotrimers. This includes P2X4/5, P2X4/6, P2X4/7 and P2X1/5 and of these, only for P2X4/7 has an interaction been demonstrated for native receptors.
Group 1
P2X2/3 heteromers
The P2X2/3 heteromeric receptor currents are easily distinguishable, by their pharmacology and kinetics, from the parent homomeric receptor currents, and this property has facilitated the identification of a native correlate Citation[12]. P2X2 receptors are slowly desensitizing and insensitive to the agonist α,β,methylene ATP, whereas P2X3 are rapidly desensitizing and activated by α,β,methylene ATP. When coexpressed, they produce a slowly desensitizing current activated by α,β,methylene ATP which cannot be attributed to the sum of the homomeric receptor currents. P2X3 has the most restricted distribution of any of the P2X subtypes, but is found in a subpopulation of sensory neurones together with P2X2 Citation[26]. Currents with similar properties to those of the heteromeric receptor have been recorded from nodose, coeliac, superior cervical and dorsal root ganglia neurones and these responses are altered in the P2X2, P2X3 and P2X2/3 double knock out mice Citation[27–29]. Further evidence supporting the existence of P2X2/3 heteromers comes from the co-IP of the heterologously expressed subunits and the use of site directed mutagenesis combined with functional and biochemical analyses to show that coexpression of wild type P2X3 can rescue non-functional and trafficking impaired P2X2 mutants Citation[30], Citation[31]. The arrangement of subunits within the heteromeric complex was probed by introducing cysteines at positions where they can form disulphide bonds between neighbouring subunits; this approach revealed that heterotrimers have the composition P2X2(P2X3)2 Citation[32].
Group 2
(1) P2X2/6 heteromers
P2X2, P2X4 and P2X6 are coexpressed in several regions within the central nervous system and all have been shown, by immunogold labeling, to be targeted to a similar location on dendritic spines at the periphery of the postsynaptic specializations Citation[33]. The mechanism involved in the specific targeting of these complexes is unknown; however their colocalization suggests that the native receptors in the brain are likely to be heteromeric assemblies of two or more of these subunits. P2X6 co-IPs with P2X2 when they are heterologously coexpressed Citation[21] and is no longer retained in the ER but traffics to the plasma membrane Citation[23]. Functional analysis of coexpressed receptors in Xenopus oocytes suggests that the heteromeric P2X2/6 receptor currents are difficult to distinguish from homomeric P2X2 receptor currents, although the incorporation of P2X6 increases the calcium permeability of P2X2 receptors by ∼50%, an effect that might be important for a role in synaptic plasticity Citation[34–36]. The subunit arrangement of P2X2/6 heteromers was determined by AFM imaging of antibody-decorated receptors purified from HEK 293 cells. Unlike P2X2/3 receptors, both P2X2(P2X6)2 and (P2X2)2P2X6 complexes were observed at frequencies dependent upon the relative levels of expression of the two subunits Citation[37].
(2) P2X1/2 heteromers
Evidence in support of this combination has been obtained using the Xenopus oocytes expression system. An additional phenotype was described after their coexpression, with novel pH sensitivity that could not be attributed to the homomeric receptor currents Citation[38]. Blue native (BN)-PAGE analysis of purified plasma membrane receptors identified P2X1/2 heterotrimers but not P2X1 homotrimers suggesting that heteromer formation is preferred Citation[17]. A physiological role for these heteromers is unknown and there is little overlap in their tissue distribution.
(3) P2X1/4 heteromers
P2X4 is the most widely expressed subtype and is present in most tissues that have so far been analyzed. It overlaps with P2X1 in smooth muscle, microglia and peripheral immune cells Citation[39], and although these two subtypes were originally shown not to co-IP when coexpressed in HEK 293 cells Citation[21], a more recent study using Xenopus oocytes, showed that when heterologously coexpressed they form part of the same trimeric complex and that currents with a novel functional phenotype are produced. P2X4 produces almost no response to 10 µM α,β,methylene ATP, but with P2X1, mediates a current in response to this agonist that desensitizes more slowly than the P2X1 homomers Citation[40].
Group 3
(1) P2X1/5 heteromers
P2X1 and P2X5 are coexpressed in the ventral horn of the spinal cord and co-IP when coexpressed in HEK 293 cells Citation[41], Citation[42]. P2X1 shares similar functional properties to P2X3 in that it produces rapidly desensitizing currents in response to α,β,methylene ATP, whereas P2X5 is similar to P2X2 and is insensitive to this agonist. When coexpressed, the currents show biphasic desensitization to α,β, methylene ATP and most notably, resensitize much more rapidly than the P2X1 homomers Citation[41], Citation[43].
(2) P2X4/5 heteromers
There has been no functional characterization of this combination, however they have been shown to co-IP when coexpressed in HEK 293 cells Citation[21] and the trafficking of P2X5 is altered by coexpression with P2X4, such that P2X5 colocalizes with P2X4 in lysosomes (RML unpublished results).
(3) P2X4/6 heteromers
P2X4 is reported to overlap with P2X6 in the CNS and the periphery, including endothelial cells. When heterologously coexpressed they co-IP and the distribution of P2X6 changes such that it is no longer retained within the ER but follows P2X4 to endolysosomes Citation[21], Citation[22]. Novel pharmacological properties of the heteromeric receptor currents have been reported in Xenopus oocytes Citation[34], and this mainly involves an increase in the sensitivity of the current to antagonists such as suramin and Reactive Blue 2.
(4) P2X4/7 heteromers
Until recently P2X7 was thought to be unique in not forming functional heteromeric receptors with any other member of the P2X family. It is, however, coexpressed with P2X4 in immune, endothelial and epithelial cells, and shares greater sequence similarity with P2X4 compared with the other subtypes. P2X7 was recently shown to co-IP with P2X4 when heterologously coexpressed, and the endogenous receptors also co-IP from mouse bone marrow derived macrophages Citation[44]. Coexpression of a dominant negative mutant of P2X4 with P2X7 reduced P2X7 receptor mediated currents, whereas coexpression of a non-functional but non-dominant negative P2X4 mutant introduced P2X4-like pharmacological properties to the whole cell current. When heterologously coexpressed, the extent of co-localization of P2X4 and P2X7 is low suggesting that the favoured assembly pathway is the formation of homotrimers. Native receptor currents with P2X4/7-like properties have, however, been recorded from airway epithelial cells Citation[45].
Although the electrophysiological properties of many homomeric and heteromeric receptors have been described, there are examples of P2X receptor currents recorded from native tissue with properties that do not match the recombinant receptors. These include ATP-evoked currents recorded from rat primary sensory neurons and from trigeminal mesencepthalic nucleus neurons Citation[46], Citation[47]. Either there are heteromeric assemblies that have not yet been characterized or other proteins interact with the receptors in native tissue to modify their properties.
Formation of multi-protein signaling complexes
P2X receptors functionally interact with other neighbouring P2X receptors and also with ion channels that belong to different structural classes including members of the Cys loop family and gap junction family. Underlying these interactions is a close spatial arrangement of the ion channel complexes within the plasma membrane. For P2X2 receptors, the formation of stable clusters of homotrimers, as resolved by BN-PAGE analysis Citation[17], correlates with the demonstration that the receptors within a patch of membrane open in a non-independent manner, displaying positive cooperativity Citation[48–50]. P2X2 receptors also interact with members of the Cys-loop family of ligand gated ion channels, including GABAA, nicotinic and 5HT3 receptors, all of which are involved in fast synaptic transmission Citation[51–56]. In this case the effect of co-activation is cross-inhibition, as demonstrated initially in sympathetic neurones, where inward currents evoked by the simultaneous application of ATP and ACh were shown to be considerably smaller than the sum of the responses produced by individually applying the two agonists Citation[57]. FRET analysis of heterologously coexpressed P2X2 and nicotinic receptors indicates that they are located within 100 Å of each other suggesting that they form oligomers Citation[58]. The mechanism underlying the reciprocol inhibitory cross-talk is poorly understood although there is evidence to suggest that it involves the C-terminal region of P2X2 receptors Citation[55], Citation[59], Citation[60]. This region is very divergent between the different subtypes and yet functional coupling with the Cys-loop channels is not restricted to P2X2 receptors. It has also been shown for P2X3 and GABAA receptors in nociceptive DRG neurones and to require a QST motif within the C-terminus of P2X3 Citation[61]. The interaction between these two classes of receptors affects their trafficking as well as their function. The coexpression of P2X2 with rho1/GABA receptors was shown to recruit the mainly intracellular rho1/GABA receptors to the plasma membrane suggesting that coassembly of these channels occurs within the ER such that they are targeted to synapses together Citation[60].
P2X7 receptors have a very different tissue distribution from P2X2 and P2X3 receptors, being predominantly expressed in immune and epithelial cells. They interact with another ion channel, pannexin-1 (panx1) Citation[62] which belongs to a recently identified family of proteins (panx1-3) that share homology with invertebrate gap junction proteins innexins Citation[63]. Despite this homology, recent evidence suggests that panx1 does not form intercellular gap junctions but instead functions as a hemichannel Citation[64–66]. Panx1 is expressed in a variety of immune cells and also cell lines that have been used to characterize P2X7 receptor function. P2X7 receptor activation induces responses that are unique to this subtype, such as an increase in membrane permeability to large dyes and cytoskeletal rearrangements that result in plasma membrane blebbing. Knocking down panx1 expression was shown to inhibit P2X7 receptor-mediated dye uptake but not membrane blebbing Citation[62]. In immune cells, inhibiting panx1 reduces P2X7 receptor stimulated release of IL-1β. The nature of the structural and functional association between P2X7 and panx1 remains to be elucidated.
Ion channels are not the only proteins that are known to associate with P2X receptors. P2X2 interacts with a neuronal scaffolding protein Fe65 and they co-localize at excitatory synapses in the CNS Citation[67]. In addition a proline rich region within the C-terminus of P2X2 binds βIII tubulin, suggesting that microtubules are involved in regulating this receptor Citation[68]. A proteomic analysis of P2X7-containing complexes purified from HEK293 cells identified 11 different proteins within the complex including β-actin, α-actinin, heat shock proteins and a receptor protein tyrosine phosphatase Citation[69]. Many of these interactions are thought to involve the unique, long C-terminal region of P2X7 but the functional significance of these interactions is not fully understood.
Trafficking of P2X receptors
The secretory pathway
P2X receptors are delivered to the plasma membrane along the secretory pathway via the ER and the Golgi complex. There are mechanisms along this pathway capable of either increasing or decreasing delivery of receptors to the plasma membrane and this is one way in which the responsiveness of the cell to extracellular ATP can be regulated. The efficiency with which P2X receptors assemble and traffic along the secretory pathway varies between the different subtypes and is also cell type-dependent.
As discussed earlier, P2X6 receptors are retained in the ER as monomers but do traffic to the surface as heteromers with P2X2 and P2X4. P2X5 is believed to have an ER retention motif which is at least partly responsible for its low functional expression as a homomer Citation[70]. For P2X3 receptors, increased trafficking along the secretory pathway appears to be an important mechanism for enhancing its activity in sensory neurones. Potentiation of P2X3 receptor function is associated both with nerve injury induced allodynia and the algogenic action of calcitonin-gene-related peptide (CGRP) Citation[71], Citation[72]. In trigeminal neurones, pretreatment with CGRP was shown to stimulate trafficking of P2X3 receptors to the cell surface via a brefeldin A sensitive pathway and this caused a strong and prolonged enhancement of receptor function that was dependent upon protein kinase (PK) A and PKC activation. The Ca2 + /calmodulin dependent PK II has also been identified as being involved in regulating the trafficking of P2X3 receptors; it becomes activated by increased electrical activity and promotes the surface expression of P2X3 receptors in DRG neurones without changing their overall expression Citation[73]. Confocal images showing the subcellular distribution of neuronal P2X3 receptors are consistent with their redistribution from the ER to the cell surface, however the assembly state of the receptor has not been examined and so it is unknown whether the receptor is retained in monomeric or trimeric form.
For P2X7 receptors, assembly and trafficking to the plasma membrane appears to be cell type dependent. In monocytes and lymphocytes the receptors are predominantly intracellular Citation[74] whereas when monocytes differentiate into macrophages there is a large increase in their surface expression and function Citation[75]. This change occurs without a significant increase in receptor mRNA levels, suggesting that the upregulation of surface P2X7 involves a redistribution of intracellular receptors to the plasma membrane. In the brain, western blot analysis of P2X7 receptors suggested they were predominantly in monomeric form and incompletely glycosylated, whereas in peritoneal macrophages, they were identified only as glycosylated multimeric complexes Citation[76]. A later study from the same group, however, cast doubt on the specificity of the antibody when used for brain tissue Citation[77]. Unlike P2X6, homomeric P2X7 receptors are functional and do not to require coassembly with other members of the P2X family in order to be exported from the ER. There are however several truncated splice variants of P2X7 receptors, one of which was recently shown to assemble with the full-length variant and antagonize its functional expression Citation[78], Citation[79]. Another was shown to be expressed at high levels in the brain Citation[79]. The expression of truncated splice variant could be a mechanism regulating the correct assembly and trafficking of P2X7 receptors. In addition, P2X7 receptors interact with many other proteins, including heat shock protein 90 (HSP90), which is a molecular chaperone able to prevent protein aggregation and to promote refolding of denatured proteins. This chaperone becomes tyrosine phosphorylated when associated with P2X7, but whether or not it plays a role in assembly and targeting of the receptor is unknown Citation[80]. The long C-terminus of the P2X7 receptor is thought to be involved in many of the interactions with other proteins and within the distal C-terminus is a region with homology to lipopolysaccharide binding proteins Citation[81]. Partial deletions and point mutations within this region, including a mutation linked to chronic lymphocytic leukemia Citation[82], inhibit the plasma membrane expression of P2X7 receptors and promote ER retention, whereas larger deletions restore both the expression and some of the functional properties of receptors at the plasma membrane Citation[83], Citation[84]. This suggests that neighbouring regions within the distal C-terminus are either directly or indirectly involved in regulating the exposure of a retention motif.
The endocytic pathway
For many ligand-gated ion channels, retrieval from and recyling to the plasma membrane is an important mechanism for rapidly modulating their function Citation[85]. Alternatively receptors can be targeted to lysosomes for degradation leading to a reduced response to a ligand. The stabilization of P2X receptors at the plasma membrane requires a motif, YXXXK, present within the proximal C-terminal region of all P2X receptor subunits Citation[31]. P2X2 receptors are normally stably expressed at the cell surface, however, mutations at Y362 or K366, enhance their retrieval without affecting their trafficking through the secretory pathway. These mutations also disrupted the axonal targeting of P2X2 receptors heterologously expressed in hippocampal neurones, suggesting that selective stabilization might be responsible for determining the location of the receptors. Despite the conservation of this motif throughout the P2X family, sustained exposure to agonist has been reported to cause the internalization of P2X1, P2X5 and P2X7 receptors Citation[86–91].
The role of endocytic pathways in regulating the surface expression of P2X receptors has been principally studied for the P2X4 receptor. They undergo constitutive endocytosis, which proceeds rapidly via a dynamin and AP-2 dependent mechanism, and a tyrosine-based motif within the C-terminus and a dileucine-like motif within the N-terminus act independently to mediate this process and target the receptors to lysosomes Citation[22], Citation[92–94]. The tyrosine based motif takes the form of a novel YXXGL motif which can be recognized by AP-2 and binds to the same pocket on the µ2 subunit as YXXφ motifs. The endogenous P2X4 receptors in immune and vascular endothelial cells are predominantly localized to lysosomes, and proteomic studies of lysosome resident membrane protein have identified this subtype but no other members of the family Citation[95]. Similar to other lysosome-targeted proteins, P2X4 resists degradation within this proteolytic environment by virtue of N-linked glycans and it is up-regulated at the plasma membrane following stimulation of lysosome exocytosis. The enhancement of P2X4 receptor mediated currents indicates that lysosome resident receptors retain their functional integrity Citation[94]. Why P2X4 receptors are targeted to lysosomes is unclear. It is also unclear whether they always traffic via the plasma membrane or can take a direct route from the TGN to late endosomes as suggested for other proteins with a dileucine-targeting motif. Lysosomal targeting ensures low surface expression of the receptor in non-activated immune cells and this is likely to be important because an up-regulation of P2X4 receptors in microglia following nerve injury contributes to neuropathic pain. Activation of P2X7 receptors triggers lysosome exocytosis and this could potentially recruit P2X4 receptors to the plasma membrane Citation[96]. Lysosome exocytosis also occurs in response to membrane injury, when ATP will be released from the damaged cell which could lead to P2X4 receptor activation Citation[97], Citation[99].
It is possible that P2X4 receptors have a functional role within endo-lysosomes. Lysosomes have been shown to be physiologically important calcium stores Citation[98] and the highly calcium permeable P2X4 receptors may play a role in calcium flux between the lumen and the cytosol. P2X4 receptors are also delivered to phagosomes and it is tempting to speculate they could form a sensor for ATP released from a phagocytosed bacterium or apoptotic cell Citation[94]. A recent report on a P2X-like receptor from Dictyostelium was the first to show an intracellular role for these channels; these receptors are targeted to contractile vacuoles and are important for osmoregulation Citation[99]. A mechanism for regulating the activity of intracellular P2X receptors has not been reported, although lysosomes are known to contain high levels of ATP Citation[100].
Future perspectives
Studies into the assembly of P2X receptors have revealed surprising complexity in their interactions with each other, with structurally unrelated ion channels and with non-integral membrane proteins. Further analyses of these complexes with both low and high-resolution structural techniques will be able to more definitively address the composition of these heteromeric assemblies. Site directed mutagenesis combined with functional analyses should provide a means of uncoupling these interactions thereby revealing their molecular basis and functional importance.
Understanding the physiological roles of these assemblies is required because several of the receptors are important therapeutic targets and their coassembly is likely to alter their pharmacological properties, which may provide an opportunity for more specific drug design. Studies using mice with genes knocked out for multiple P2X subunits may further reveal the contribution of the heteromeric receptors towards ATP-mediated responses
The targeting of P2X receptors to their destinations within the cell also provides insight into their physiological roles. Elucidation of the mechanisms that control assembly and trafficking of the receptor complexes will provide a greater understanding of the importance of regulating plasma membrane in physiological and pathological processes.
Supplementary Material
Supplementary Table I. Physiological and pathological role of P2X receptors confirmed by knocking out or knocking down their expression.
Acknowledgements
We would like to thank Drs J. M. Edwardson and I. Greger for providing helpful comments on this manuscript. Declaration of interest: The authors report no conflicts of interest. The authors alone are responsible for the content and writing of the paper.
References
- Roberts JA, Vial C, Digby HR, Agboh KC, Wen H, Atterbury-Thomas A, Evans RJ. Molecular properties of P2X receptors. Pflugers Arch 2006; 452: 486–500
- North RA. Molecular physiology of P2X receptors. Physiol Rev 2002; 82: 1013–1067
- North RA, Verkhratsky A. Purinergic transmission in the central nervous system. Pflugers Arch 2006; 452: 479–485
- Khakh BS, North RA. P2X receptors as cell-surface ATP sensors in health and disease. Nature 2006; 442: 527–532
- Inoue K. The function of microglia through purinergic receptors: neuropathic pain and cytokine release. Pharmacol Ther 2006; 109: 210–226
- Khakh BS, Burnstock G, Kennedy C, King BF, North RA, Seguela P, Voigt M, Humphrey PP. International union of pharmacology. XXIV. Current status of the nomenclature and properties of P2X receptors and their subunits. Pharmacol Rev 2001; 53: 107–118
- North RA, Surprenant A. Pharmacology of cloned P2X receptors. Annu Rev Pharmacol Toxicol 2000; 40: 563–580
- Brake AJ, Wagenbach MJ, Julius D. New structural motif for ligand-gated ion channels defined by an ionotropic ATP receptor. Nature 1994; 371: 519–523
- Valera S, Hussy N, Evans RJ, Adami N, North RA, Surprenant A, Buell G. A new class of ligand-gated ion channel defined by P2X receptor for extracellular ATP. Nature 1994; 371: 516–519
- Surprenant A, Buell G, North RA. P2X receptors bring new structure to ligand-gated ion channels. Trends Neurosci 1995; 18: 224–229
- Jasti J, Furukawa H, Gonzales EB, Gouaux E. Structure of acid-sensing ion channel 1 at 1.9 A resolution and low pH. Nature 2007; 449: 316–323
- Lewis C, Neidhart S, Holy C, North RA, Buell G, Surprenant A. Coexpression of P2X2 and P2X3 receptor subunits can account for ATP-gated currents in sensory neurons. Nature 1995; 377: 432–435
- Ruddock LW, Molinari M. N-glycan processing in ER quality control. J Cell Sci 2006; 119: 4373–4380
- Kim M, Yoo OJ, Choe S. Molecular assembly of the extracellular domain of P2X2, an ATP-gated ion channel. Biochem Biophys Res Commun 1997; 240: 618–622
- Torres GE, Egan TM, Voigt MM. Identification of a domain involved in ATP-gated ionotropic receptor subunit assembly. J Biol Chem 1999; 274: 22359–22365
- Nicke A, Baumert HG, Rettinger J, Eichele A, Lambrecht G, Mutschler E, Schmalzing G. P2X1 and P2X3 receptors form stable trimers: a novel structural motif of ligand-gated ion channels. Embo J 1998; 17: 3016–3028
- Aschrafi A, Sadtler S, Niculescu C, Rettinger J, Schmalzing G. Trimeric architecture of homomeric P2X2 and heteromeric P2X1 + 2 receptor subtypes. J Mol Biol 2004; 342: 333–343
- Barrera NP, Ormond SJ, Henderson RM, Murrell-Lagnado RD, Edwardson JM. Atomic force microscopy imaging demonstrates that P2X2 receptors are trimers but that P2X6 receptor subunits do not oligomerize. J Biol Chem 2005; 280: 10759–10765
- Nakazawa K, Yamakoshi Y, Tsuchiya T, Ohno Y. Purification and aqueous phase atomic force microscopic observation of recombinant P2X2 receptor. Eur J Pharmacol 2005; 518: 107–110
- Mio K, Kubo Y, Ogura T, Yamamoto T, Sato C. Visualization of the trimeric P2X2 receptor with a crown-capped extracellular domain. Biochem Biophys Res Commun 2005; 337: 998–1005
- Torres GE, Egan TM, Voigt MM. Hetero-oligomeric assembly of P2X receptor subunits. Specificities exist with regard to possible partners. J Biol Chem 1999; 274: 6653–6659
- Bobanovic LK, Royle SJ, Murrell-Lagnado RD. P2X receptor trafficking in neurons is subunit specific. J Neurosci 2002; 22: 4814–4824
- Ormond SJ, Barrera NP, Qureshi OS, Henderson RM, Edwardson JM, Murrell-Lagnado RD. An uncharged region within the N terminus of the P2X6 receptor inhibits its assembly and exit from the endoplasmic reticulum. Mol Pharmacol 2006; 69: 1692–1700
- Feng YH, Li X, Wang L, Zhou L, Gorodeski GI. A truncated P2X7 receptor variant (P2X7-j) endogenously expressed in cervical cancer cells antagonizes the full-length P2X7 receptor through hetero-oligomerization. J Biol Chem 2006; 281: 17228–17237
- Duckwitz W, Hausmann R, Aschrafi A, Schmalzing G. P2X5 subunit assembly requires scaffolding by the second transmembrane domain and a conserved aspartate. J Biol Chem 2006; 281: 39561–39572
- Kobayashi K, Fukuoka T, Yamanaka H, Dai Y, Obata K, Tokunaga A, Noguchi K. Differential expression patterns of mRNAs for P2X receptor subunits in neurochemically characterized dorsal root ganglion neurons in the rat. J Comp Neurol 2005; 481: 377–390
- Khakh BS, Humphrey PP, Surprenant A. Electrophysiological properties of P2X-purinoceptors in rat superior cervical, nodose and guinea-pig coeliac neurones. J Physiol 1995; 484(Pt 2)385–395
- Cockayne DA, Dunn PM, Zhong Y, Rong W, Hamilton SG, Knight GE, Ruan HZ, Ma B, Yip P, Nunn P, McMahon SB, Burnstock G, Ford AP. P2X2 knockout mice and P2X2/P2X3 double knockout mice reveal a role for the P2X2 receptor subunit in mediating multiple sensory effects of ATP. J Physiol 2005; 567: 621–639
- Zhong Y, Dunn PM, Bardini M, Ford AP, Cockayne DA, Burnstock G. Changes in P2X receptor responses of sensory neurons from P2X3-deficient mice. Eur J Neurosci 2001; 14: 1784–1792
- Wilkinson WJ, Jiang LH, Surprenant A, North RA. Role of ectodomain lysines in the subunits of the heteromeric P2X2/3 receptor. Mol Pharmacol 2006; 70: 1159–1163
- Chaumont S, Jiang LH, Penna A, North RA, Rassendren F. Identification of a trafficking motif involved in the stabilization and polarization of P2X receptors. J Biol Chem 2004; 279: 29628–29638
- Jiang LH, Kim M, Spelta V, Bo X, Surprenant A, North RA. Subunit arrangement in P2X receptors. J Neurosci 2003; 23: 8903–8910
- Rubio ME, Soto F. Distinct localization of P2X receptors at excitatory postsynaptic specializations. J Neurosci 2001; 21: 641–653
- Le KT, Babinski K, Seguela P. Central P2X4 and P2X6 channel subunits coassemble into a novel heteromeric ATP receptor. J Neurosci 1998; 18: 7152–7159
- King BF, Townsend-Nicholson A, Wildman SS, Thomas T, Spyer KM, Burnstock G. Coexpression of rat P2X2 and P2X6 subunits in Xenopus oocytes. J Neurosci 2000; 20: 4871–4877
- Egan TM, Khakh BS. Contribution of calcium ions to P2X channel responses. J Neurosci 2004; 24: 3413–3420
- Barrera NP, Henderson RM, Murrell-Lagnado RD, Edwardson JM. The stoichiometry of P2X2/6 receptor heteromers depends on relative subunit expression levels. Biophys J 2007; 93: 505–512
- Brown SG, Townsend-Nicholson A, Jacobson KA, Burnstock G, King BF. Heteromultimeric P2X(1/2) receptors show a novel sensitivity to extracellular pH. J Pharmacol Exp Ther 2002; 300: 673–680
- Xiang Z, Burnstock G. Expression of P2X receptors on rat microglial cells during early development. Glia 2005; 52: 119–126
- Nicke A, Kerschensteiner D, Soto F. Biochemical and functional evidence for heteromeric assembly of P2X1 and P2X4 subunits. J Neurochem 2005; 92: 925–933
- Torres GE, Haines WR, Egan TM, Voigt MM. Co-expression of P2X1 and P2X5 receptor subunits reveals a novel ATP-gated ion channel. Mol Pharmacol 1998; 54: 989–993
- Collo G, North RA, Kawashima E, Merlo-Pich E, Neidhart S, Surprenant A, Buell G. Cloning of P2X5 and P2X6 receptors and the distribution and properties of an extended family of ATP-gated ion channels. J Neurosci 1996; 16: 2495–2507
- Le KT, Boue-Grabot E, Archambault V, Seguela P. Functional and biochemical evidence for heteromeric ATP-gated channels composed of P2X1 and P2X5 subunits. J Biol Chem 1999; 274: 15415–15419
- Guo C, Masin M, Qureshi OS, Murrell-Lagnado RD. Evidence for functional P2X4/P2X7 heteromeric receptors. Mol Pharmacol 2007; 72: 1447–1456
- Ma W, Korngreen A, Weil S, Cohen EB, Priel A, Kuzin L, Silberberg SD. Pore properties and pharmacological features of the P2X receptor channel in airway ciliated cells. J Physiol 2006; 571: 503–517
- Patel MK, Khakh BS, Henderson G. Properties of native P2X receptors in rat trigeminal mesencephalic nucleus neurones: lack of correlation with known, heterologously expressed P2X receptors. Neuropharmacology 2001; 40: 96–105
- Tsuzuki K, Ase A, Seguela P, Nakatsuka T, Wang CY, She JX, Gu JG. TNP-ATP-resistant P2X ionic current on the central terminals and somata of rat primary sensory neurons. J Neurophysiol 2003; 89: 3235–3242
- Clyne JD, Brown TC, Hume RI. Expression level dependent changes in the properties of P2X2 receptors. Neuropharmacology 2003; 44: 403–412
- Ding S, Sachs F. Evidence for non-independent gating of P2X2 receptors expressed in Xenopus oocytes. BMC Neurosci 2002; 3: 17
- Fujiwara Y, Kubo Y. Density-dependent changes of the pore properties of the P2X2 receptor channel. J Physiol 2004; 558: 31–43
- Zhou X, Galligan JJ. Non-additive interaction between nicotinic cholinergic and P2X purine receptors in guinea-pig enteric neurons in culture. J Physiol 1998; 513(Pt 3)685–697
- Barajas-Lopez C, Espinosa-Luna R, Zhu Y. Functional interactions between nicotinic and P2X channels in short-term cultures of guinea-pig submucosal neurons. J Physiol 1998; 513(Pt 3)671–683
- Khakh BS, Zhou X, Sydes J, Galligan JJ, Lester HA. State-dependent cross-inhibition between transmitter-gated cation channels. Nature 2000; 406: 405–410
- Barajas-Lopez C, Montano LM, Espinosa-Luna R. Inhibitory interactions between 5-HT3 and P2X channels in submucosal neurons. Am J Physiol Gastrointest Liver Physiol 2002; 283: G1238–1248
- Boue-Grabot E, Barajas-Lopez C, Chakfe Y, Blais D, Belanger D, Emerit MB, Seguela P. Intracellular cross talk and physical interaction between two classes of neurotransmitter-gated channels. J Neurosci 2003; 23: 1246–1253
- Sokolova E, Nistri A, Giniatullin R. Negative cross talk between anionic GABAA and cationic P2X ionotropic receptors of rat dorsal root ganglion neurons. J Neurosci 2001; 21: 4958–4968
- Searl TJ, Redman RS, Silinsky EM. Mutual occlusion of P2X ATP receptors and nicotinic receptors on sympathetic neurons of the guinea-pig. J Physiol 1998; 510(Pt 3)783–791
- Khakh BS, Fisher JA, Nashmi R, Bowser DN, Lester HA. An angstrom scale interaction between plasma membrane ATP-gated P2X2 and alpha4beta2 nicotinic channels measured with fluorescence resonance energy transfer and total internal reflection fluorescence microscopy. J Neurosci 2005; 25: 6911–6920
- Boue-Grabot E, Toulme E, Emerit MB, Garret M. Subunit-specific coupling between gamma-aminobutyric acid type A and P2X2 receptor channels. J Biol Chem 2004; 279: 52517–52525
- Boue-Grabot E, Emerit MB, Toulme E, Seguela P, Garret M. Cross-talk and co-trafficking between rho1/GABA receptors and ATP-gated channels. J Biol Chem 2004; 279: 6967–6975
- Toulme E, Blais D, Leger C, Landry M, Garret M, Seguela P, Boue-Grabot E. An intracellular motif of P2X(3) receptors is required for functional cross-talk with GABA(A) receptors in nociceptive DRG neurons. J Neurochem 2007; 102: 1357–1368
- Pelegrin P, Surprenant A. Pannexin-1 mediates large pore formation and interleukin-1beta release by the ATP-gated P2X7 receptor. Embo J 2006; 25: 5071–5082
- Panchin YV. Evolution of gap junction proteins – the pannexin alternative. J Exp Biol 2005; 208: 1415–1419
- Bruzzone R, Barbe MT, Jakob NJ, Monyer H. Pharmacological properties of homomeric and heteromeric pannexin hemichannels expressed in Xenopus oocytes. J Neurochem 2005; 92: 1033–1043
- Boassa D, Ambrosi C, Qiu F, Dahl G, Gaietta G, Sosinsky G. Pannexin1 channels contain a glycosylation site that targets the hexamer to the plasma membrane. J Biol Chem 2007; 282: 31733–31743
- Penuela S, Bhalla R, Gong XQ, Cowan KN, Celetti SJ, Cowan BJ, Bai D, Shao Q, Laird DW. Pannexin 1 and pannexin 3 are glycoproteins that exhibit many distinct characteristics from the connexin family of gap junction proteins. J Cell Sci 2007; 120: 3772–3783
- Masin M, Kerschensteiner D, Dumke K, Rubio ME, Soto F. Fe65 interacts with P2X2 subunits at excitatory synapses and modulates receptor function. J Biol Chem 2006; 281: 4100–4108
- Gendreau S, Schirmer J, Schmalzing G. Identification of a tubulin binding motif on the P2X2 receptor. J Chromatogr B Analyt Technol Biomed Life Sci 2003; 786: 311–318
- Kim M, Jiang LH, Wilson HL, North RA, Surprenant A. Proteomic and functional evidence for a P2X7 receptor signalling complex. Embo J 2001; 20: 6347–6358
- Bo X, Jiang LH, Wilson HL, Kim M, Burnstock G, Surprenant A, North RA. Pharmacological and biophysical properties of the human P2X5 receptor. Mol Pharmacol 2003; 63: 1407–1416
- Chen Y, Li GW, Wang C, Gu Y, Huang LY. Mechanisms underlying enhanced P2X receptor-mediated responses in the neuropathic pain state. Pain 2005; 119: 38–48
- Fabbretti E, D'Arco M, Fabbro A, Simonetti M, Nistri A, Giniatullin R. Delayed upregulation of ATP P2X3 receptors of trigeminal sensory neurons by calcitonin gene-related peptide. J Neurosci 2006; 26: 6163–6171
- Xu GY, Huang LY. Ca2 + /calmodulin-dependent protein kinase II potentiates ATP responses by promoting trafficking of P2X receptors. Proc Natl Acad Sci USA 2004; 101: 11868–11873
- Gu BJ, Zhang WY, Bendall LJ, Chessell IP, Buell GN, Wiley JS. Expression of P2X(7) purinoceptors on human lymphocytes and monocytes: evidence for nonfunctional P2X(7) receptors. Am J Physiol Cell Physiol 2000; 279: C1189–1197
- Gudipaty L, Humphreys BD, Buell G, Dubyak GR. Regulation of P2X(7) nucleotide receptor function in human monocytes by extracellular ions and receptor density. Am J Physiol Cell Physiol 2001; 280: C943–953
- Kim M, Spelta V, Sim J, North RA, Surprenant A. Differential assembly of rat purinergic P2X7 receptor in immune cells of the brain and periphery. J Biol Chem 2001; 276: 23262–23267
- Sim JA, Young MT, Sung HY, North RA, Surprenant A. Reanalysis of P2X7 receptor expression in rodent brain. J Neurosci 2004; 24: 6307–6314
- Feng YH, Li X, Zeng R, Gorodeski GI. Endogenously expressed truncated P2X7 receptor lacking the C-terminus is preferentially upregulated in epithelial cancer cells and fails to mediate ligand-induced pore formation and apoptosis. Nucleosides Nucleotides Nucleic Acids 2006; 25: 1271–1276
- Cheewatrakoolpong B, Gilchrest H, Anthes JC, Greenfeder S. Identification and characterization of splice variants of the human P2X7 ATP channel. Biochem Biophys Res Commun 2005; 332: 17–27
- Adinolfi E, Kim M, Young MT, Di Virgilio F, Surprenant A. Tyrosine phosphorylation of HSP90 within the P2X7 receptor complex negatively regulates P2X7 receptors. J Biol Chem 2003; 278: 37344–37351
- Denlinger LC, Fisette PL, Sommer JA, Watters JJ, Prabhu U, Dubyak GR, Proctor RA, Bertics PJ. Cutting edge: the nucleotide receptor P2X7 contains multiple protein- and lipid-interaction motifs including a potential binding site for bacterial lipopolysaccharide. J Immunol 2001; 167: 1871–1876
- Wiley JS, Dao-Ung LP, Li C, Shemon AN, Gu BJ, Smart ML, Fuller SJ, Barden JA, Petrou S, Sluyter R. An Ile-568 to Asn polymorphism prevents normal trafficking and function of the human P2X7 receptor. J Biol Chem 2003; 278: 17108–17113
- Smart ML, Gu B, Panchal RG, Wiley J, Cromer B, Williams DA, Petrou S. P2X7 receptor cell surface expression and cytolytic pore formation are regulated by a distal C-terminal region. J Biol Chem 2003; 278: 8853–8860
- Denlinger LC, Sommer JA, Parker K, Gudipaty L, Fisette PL, Watters JW, Proctor RA, Dubyak GR, Bertics PJ. Mutation of a dibasic amino acid motif within the C terminus of the P2X7 nucleotide receptor results in trafficking defects and impaired function. J Immunol 2003; 171: 1304–1311
- Royle SJ, Murrell-Lagnado RD. Constitutive cycling: a general mechanism to regulate cell surface proteins. Bioessays 2003; 25: 39–46
- Jensik P, Cox T. ATP-induced internalization of amphibian epithelial P2X receptors is linked to channel opening. Pflugers Arch 2002; 444: 795–800
- Dutton JL, Poronnik P, Li GH, Holding CA, Worthington RA, Vandenberg RJ, Cook DI, Barden JA, Bennett MR. P2X(1) receptor membrane redistribution and down-regulation visualized by using receptor-coupled green fluorescent protein chimeras. Neuropharmacology 2000; 39: 2054–2066
- Ennion SJ, Evans RJ. Agonist-stimulated internalisation of the ligand-gated ion channel P2X(1) in rat vas deferens. FEBS Lett 2001; 489: 154–158
- Hiken JF, Steinberg TH. ATP downregulates P2X7 and inhibits osteoclast formation in RAW cells. Am J Physiol Cell Physiol 2004; 287: C403–412
- Feng YH, Wang L, Wang Q, Li X, Zeng R, Gorodeski GI. ATP stimulates GRK-3 phosphorylation and beta-arrestin-2-dependent internalization of P2X7 receptor. Am J Physiol Cell Physiol 2005; 288: C1342–1356
- Li GH, Lee EM, Blair D, Holding C, Poronnik P, Cook DI, Barden JA, Bennett MR. The distribution of P2X receptor clusters on individual neurons in sympathetic ganglia and their redistribution on agonist activation. J Biol Chem 2000; 275: 29107–29112
- Royle SJ, Bobanovic LK, Murrell-Lagnado RD. Identification of a non-canonical tyrosine-based endocytic motif in an ionotropic receptor. J Biol Chem 2002; 277: 35378–35385
- Royle SJ, Qureshi OS, Bobanovic LK, Evans PR, Owen DJ, Murrell-Lagnado RD. Non-canonical YXXGPhi endocytic motifs: recognition by AP2 and preferential utilization in P2X4 receptors. J Cell Sci 2005; 118: 3073–3080
- Qureshi OS, Paramasivam A, Yu JC, Murrell-Lagnado RD. Regulation of P2X4 receptors by lysosomal targeting, glycan protection and exocytosis. J Cell Sci 2007; 120: 3838–3849
- Schroder B, Wrocklage C, Pan C, Jager R, Kosters B, Schafer H, Elsasser HP, Mann M, Hasilik A. Integral and associated lysosomal membrane proteins. Traffic 2007; 8: 1676–1686
- Andrei C, Margiocco P, Poggi A, Lotti LV, Torrisi MR, Rubartelli A. Phospholipases C and A2 control lysosome-mediated IL-1 beta secretion: implications for inflammatory processes. Proc Natl Acad Sci USA 2004; 101: 9745–9750
- Reddy A, Caler EV, Andrews NW. Plasma membrane repair is mediated by Ca(2 + )-regulated exocytosis of lysosomes. Cell 2001; 106: 157–169
- Churchill GC, Okada Y, Thomas JM, Genazzani AA, Patel S, Gallione A. NAADP mobilizes ca2 + from reserve granules, lysosome-related organelles, in sea urchin eggs. Cell 2002; 111: 703–708
- Fountain SJ, Parkinson K, Young MT, Cao L, Thompson CR, North RA. An intracellular P2X receptor required for osmoregulation in Dictyostelium discoideum. Nature 2007; 448: 200–203
- Zhang Z, Chen G, Zhou W, Song A, Xu T, Luo Q, Wang W, Gu XS, Duan S. Regulated ATP release from astrocytes through lysosome exocytosis. Nat Cell Biol 2007; 9: 945–953
Supplementary References
- Mulryan K, Gitterman DP, Lewis CJ, Vial C, Leckie BJ, Cobb AL, Brown JE, Conley EC, Buell G, Pritchard CA, Evans RJ. Reduced vas deferens contraction and male infertility in mice lacking P2X1 receptors. Nature 2000; 403: 86–89
- Vial C, Evans RJ. P2X(1) receptor-deficient mice establish the native P2X receptor and a P2Y6-like receptor in arteries. Mol Pharmacol 2002; 62: 1438–1445
- Vial C, Evans RJ. P2X receptor expression in mouse urinary bladder and the requirement of P2X(1) receptors for functional P2X receptor responses in the mouse urinary bladder smooth muscle. Br J Pharmacol 2000; 131: 1489–1495
- Rong W, Gourine AV, Cockayne DA, Xiang Z, Ford AP, Spyer KM, Burnstock G. Pivotal role of nucleotide P2X2 receptor subunit of the ATP-gated ion channel mediating ventilatory responses to hypoxia. J Neurosci 2003; 23: 11315–11321
- Souslova V, Cesare P, Ding Y, Akopian AN, Stanfa L, Suzuki R, Carpenter K, Dickenson A, Boyce S, Hill R, Nebenuis-Oosthuizen D, Smith AJ, Kidd EJ, Wood JN. Warm-coding deficits and aberrant inflammatory pain in mice lacking P2X3 receptors. Nature 2000; 407: 1015–1017
- Dorn G, Patel S, Wotherspoon G, Hemmings-Mieszczak M, Barclay J, Natt FJ, Martin P, Bevan S, Fox A, Ganju P, Wishart W, Hall J. siRNA relieves chronic neuropathic pain. Nucleic Acids Res 2004; 32: e49
- Cockayne DA, Hamilton SG, Zhu QM, Dunn PM, Zhong Y, Novakovic S, Malmberg AB, Cain G, Berson A, Kassotakis L, Hedley L, Lachnit WG, Burnstock G, McMahon SB, Ford AP. Urinary bladder hyporeflexia and reduced pain-related behaviour in P2X3-deficient mice. Nature 2000; 407: 1011–1015
- Vlaskovska M, Kasakov L, Rong W, Bodin P, Bardini M, Cockayne DA, Ford AP, Burnstock G. P2X3 knock-out mice reveal a major sensory role for urothelially released ATP. J Neurosci 2001; 21: 5670–5677
- Bian X, Ren J, DeVries M, Schnegelsberg B, Cockayne DA, Ford AP, Galligan JJ. Peristalsis is impaired in the small intestine of mice lacking the P2X3 subunit. J Physiol 2003; 551: 309–322
- Finger TE, Danilova V, Barrows J, Bartel DL, Vigers AJ, Stone L, Hellekant G, Kinnamon SC. ATP signaling is crucial for communication from taste buds to gustatory nerves. Science 2005; 310: 1495–1499
- Yamamoto K, Korenaga R, Kamiya A, Ando J. Fluid shear stress activates Ca(2 + ) influx into human endothelial cells via P2X4 purinoceptors. Circ Res 2000; 87: 385–391
- Yamamoto K, Korenaga R, Kamiya A, Qi Z, Sokabe M, Ando J. P2X(4) receptors mediate ATP-induced calcium influx in human vascular endothelial cells. Am J Physiol Heart Circ Physiol 2000; 279: H285–292
- Yamamoto K, Sokabe T, Matsumoto T, Yoshimura K, Shibata M, Ohura N, Fukuda T, Sato T, Sekine K, Kato S, Isshiki M, Fujita T, Kobayashi M, Kawamura K, Masuda H, Kamiya A, Ando J. Impaired flow-dependent control of vascular tone and remodeling in P2X4-deficient mice. Nat Med 2006; 12: 133–137
- Liang L, Zsembery A, Schwiebert EM. RNA interference targeted to multiple P2X receptor subtypes attenuates zinc-induced calcium entry. Am J Physiol Cell Physiol 2005; 289: C388–396
- Zsembery A, Boyce AT, Liang L, Peti-Peterdi J, Bell PD, Schwiebert EM. Sustained calcium entry through P2X nucleotide receptor channels in human airway epithelial cells. J Biol Chem 2003; 278: 13398–13408
- Zsembery A, Fortenberry JA, Liang L, Bebok Z, Tucker TA, Boyce AT, Braunstein GM, Welty E, Bell PD, Sorscher EJ, Clancy JP, Schwiebert EM. Extracellular zinc and ATP restore chloride secretion across cystic fibrosis airway epithelia by triggering calcium entry. J Biol Chem 2004; 279: 10720–10729
- Tsuda M, Shigemoto-Mogami Y, Koizumi S, Mizokoshi A, Kohsaka S, Salter MW, Inoue K. P2X4 receptors induced in spinal microglia gate tactile allodynia after nerve injury. Nature 2003; 424: 778–783
- Sim JA, Chaumont S, Jo J, Ulmann L, Young MT, Cho K, Buell G, North RA, Rassendren F. Altered hippocampal synaptic potentiation in P2X4 knock-out mice. J Neurosci 2006; 26: 9006–9009
- Le Feuvre RA, Brough D, Iwakura Y, Takeda K, Rothwell NJ. Priming of macrophages with lipopolysaccharide potentiates P2X7-mediated cell death via a caspase-1-dependent mechanism, independently of cytokine production. J Biol Chem 2002; 277: 3210–3218
- Labasi JM, Petrushova N, Donovan C, McCurdy S, Lira P, Payette MM, Brissette W, Wicks JR, Audoly L, Gabel CA. Absence of the P2X7 receptor alters leukocyte function and attenuates an inflammatory response. J Immunol 2002; 168: 6436–6445
- Ke HZ, Qi H, Weidema AF, Zhang Q, Panupinthu N, Crawford DT, Grasser WA, Paralkar VM, Li M, Audoly LP, Gabel CA, Jee WS, Dixon SJ, Sims SM, Thompson DD. Deletion of the P2X7 nucleotide receptor reveals its regulatory roles in bone formation and resorption. Mol Endocrinol 2003; 17: 1356–1367
- Chessell IP, Hatcher JP, Bountra C, Michel AD, Hughes JP, Green P, Egerton J, Murfin M, Richardson J, Peck WL, Grahames CB, Casula MA, Yiangou Y, Birch R, Anand P, Buell GN. Disruption of the P2X7 purinoceptor gene abolishes chronic inflammatory and neuropathic pain. Pain 2005; 114: 386–396
- Feng YH, Li X, Zeng R, Gorodeski GI. Endogenously expressed truncated P2X7 receptor lacking the C-terminus is preferentially upregulated in epithelial cancer cells and fails to mediate ligand-induced pore formation and apoptosis. Nucleosides Nucleotides Nucleic Acids 2006; 25: 1271–1276
- Wiley JS, Dao-Ung LP, Gu BJ, Sluyter R, Shemon AN, Li C, Taper J, Gallo J, Manoharan A. A loss-of-function polymorphic mutation in the cytolytic P2X7 receptor gene and chronic lymphocytic leukaemia: a molecular study. Lancet 2002; 359: 1114–1119