Abstract
Associations between the 140 amino acid protein α-synuclein (asyn) and presynaptic vesicles may play a role in maintaining synaptic plasticity and neurotransmitter release. These physiological processes may involve disruption and fusion of vesicles, arising from interactions between specific regions of asyn, including the highly basic N-terminal domain, and the surface of vesicles. This work investigates whether asyn affects the integrity of model unilamellar vesicles of varying size and phospholipid composition, by monitoring paramagnetic Mn2+-induced broadening of peaks in the 31P nuclear magnetic resonance spectrum of the lipid head groups. It is shown that asyn increases the permeability to Mn2+ of both large (200nm diameter) and small (50nm diameter) vesicles composed of zwitterionic phosphatidylcholine and anionic phosphatidylglycerol at protein/lipid molar ratios as low as 1:2000. Further experiments on peptides corresponding to sequences in the N-terminal (10–48), C-terminal (120–140) and central hydrophobic (71–82) regions of asyn suggest that single regions of the protein are capable of permeabilizing the vesicles to varying extents. Electron micrographs of the vesicles after addition of asyn indicate that the enhanced permeability is coupled to large-scale disruption or fusion of the vesicles. These results indicate that asyn is able to permeabilize phospholipid vesicles at low relative concentrations, dependent upon the properties of the vesicles. This could have implications for asyn playing a role in vesicle synthesis, maintenance and fusion within synapses.
Abbreviations | ||
asyn | = | α-synuclein |
asyn(10–48) | = | a peptide comprising residues 10–48 from α-synuclein |
asyn(71–82) | = | a peptide comprising residues 71–82 from α-synuclein |
asyn(120–140) | = | a peptide comprising residues 120–140 from α-synuclein |
EM | = | Electron Microscopy |
DMPC | = | L-α-dimyristoylphosphatidylcholine |
DOPC | = | L-α-dioleoylphosphatidylcholine |
DOPG | = | L-α-dioleoylphosphatidylglycerol |
HPLC | = | high-pressure liquid chromatography |
LUVs | = | large unilamellar vesicles |
NMR | = | nuclear magnetic resonance |
MAS | = | magic-angle spinning |
PC | = | phosphatidylcholine |
SUVs | = | small unilamellar vesicles |
Introduction
α-Synuclein (asyn) is a 140 amino acid protein that is concentrated at the presynaptic termini of primary neurons within the central nervous system. The protein has been implicated in several neurodegenerative diseases owing to the identification of insoluble deposits of asyn in neuronal inclusions known as Lewy bodies, which are found in diseased brain tissue Citation[1]. Neurodegenerative disease progression is associated with the conversion of natively unfolded asyn to a β-sheet rich conformation, followed by aggregation into filamentous inclusions. Interactions between asyn and presynaptic vesicles are believed to underpin the physiological role of the protein in maintaining synaptic plasticity and neurotransmitter release Citation[2–4], and may also be implicated in the aggregation process Citation[5], Citation[6].
Consistent with the role of asyn in mediating neurotransmitter release, protofibrillar asyn has been shown to be capable of vesicle permeabilization at high protein concentrations Citation[7] with soluble asyn observed using atomic force microscopy to disrupt lipid bilayers and form holes at specific defect sites Citation[8]. There are also suggestions that the pathogenic form of asyn may be soluble oligomers produced as intermediates along the aggregation pathway that form channels in cell membranes and cause increased permeability leading to cell death Citation[9–11]. Previous work has shown that asyn associates with the surface of phospholipid vesicles to an extent that depends on the phospholipid head group composition and vesicle size Citation[8], Citation[12], Citation[13]. Our earlier work found that the lysine-rich N-terminus of asyn interacts preferentially with acidic phospholipid head groups, whereas the glutamate-rich C-terminus associates weakly with zwitterionic head groups such as phosphatidylcholine (PC) Citation[12]. The same study also provided evidence that the membrane association of asyn promotes the lateral separation of anionic and neutral lipid species. There is limited information about the relationship between membrane affinity of asyn and vesicle destabilization, with Zhu et al., proposing a correlation between the ability of asyn to disrupt membranes and lipid binding affinity Citation[14].
This work investigates the destabilizing effects of soluble asyn on unilamellar phospholipid vesicles of defined composition and size. Vesicle destabilization was measured as the increase in permeability to the paramagnetic nuclear magnetic resonance (NMR) relaxation agent Mn2+, by exploiting the 31P NMR signals from the phospholipid headgroups, and by electron microscopy (EM). Experiments were conducted to study how the charge carried by the lipid headgroups and the hydrocarbon chain composition influences vesicle stability in the presence of asyn. For this purpose large and small unilamellar vesicles were prepared containing the lipids L-α-dimyristoylphosphatidylcholine (DMPC) or L-α-dioleoylphosphatidylcholine (DOPC), alone or mixed with the anionic lipid L-α-dioleoylphosphatidylglycerol (DOPG). The lipids differ in their hydrocarbon chain length (C14 for myristoyl and C18 for oleoyl) and saturation. DOPG does not occur in eukaryotic organisms but has convenient NMR properties, giving a signature peak in the 31P spectrum that is well resolved from that of phosphatidylcholine Citation[12]. Synaptic membranes in eukaryotes are mainly composed of predominantly neutral PC (∼40%), phosphatidylethanolamine (∼25%), plasmenylethanolamine (∼15%) and sphingomyelin (∼15%), with acidic phosphatidylserine (∼10%) and phosphatidylinositol (∼4%) distributed throughout Citation[15], Citation[16]. The distribution of phospholipids within neuronal vesicles is asymmetric, with most of the anionic lipids found in the cytosol-facing leaflet of the membrane bilayer. The results suggest that vesicle destabilization by asyn is highly dependent on the physicochemical properties of the lipid components, but that vesicle size is less critical. Electron microscopy (EM) reveals that vesicle permeabilization is concomitant with considerable changes in morphology and, in certain cases, promotes vesicle fusion. These results highlight the sensitivity of 31P NMR to vesicle disruption and support further the role of asyn as a fusogenic protein, possibly functioning in the fusion of synaptic vesicles involved in synapse regulation.
Materials and methods
Materials
DMPC, DOPG and DOPC were all purchased from Sigma Chemicals Limited. Full-length asyn was purchased from rPeptide (USA). The peptide asyn(10–48) was synthesized in pure form by Peptide Protein Research Ltd (UK). The peptides asyn(71–82) and asyn(120–140) were synthesized in University of Manchester using solid phase fmoc (N-(9-fluorenyl)methoxycarbonyl) L-amino acid chemistry, on an Applied Biosystems 433A peptide synthesizer. Cleavage from the resin was achieved using 95% trifluoroacetic acid/2.5% water/2.5% triisopropylsilane and the peptide precipitated and cleaned by ether washing. Purification was achieved using high-pressure liquid chromatography (HPLC) with a C18 column and the product identity was confirmed by MALDI mass spectrometry. Both peptides were over 95% pure, as confirmed by HPLC. All peptides were prepared without terminal acetylation or amidation to improve solubility.
Preparation of lipid vesicles
Small unilamellar vesicles (SUVs) were prepared by tip sonication of aqueous lipid suspensions Citation[17]. Lipids (DMPC or DOPC alone, and mixed with DOPG with PC at two-fold molar excess) were co-dissolved in chloroform, the solvent was removed under a stream of argon and dried under high vacuum overnight. The lipid film was resuspended in double-distilled H2O to the concentration required for the assay and vortexed until mixed homogeneously. The suspension was sonicated for three cycles (1min on/1min off) on ice. The formation of SUVs was assumed to occur when the suspensions became clear. Large unilamellar vesicles (LUVs) were prepared by resuspending the lipid film without sonication and extruding through a 200nm syringe filter 10times.
NMR methods
SUVs containing 20 mg lipid were prepared in 500 µl ddH2O, as described above. The volume was reduced to 200 µl using a centrifugal filter and 50 µl of the remaining solution (containing 5 mg lipids) was transferred to a 4 mm zirconia rotor. Magic-angle spinning (MAS) 31P NMR spectra were recorded at 30°C on a Bruker Avance 400 MHz spectrometer using a double tuned probe operating at frequencies of 400.13 MHz for 1H and 162.12 MHz for 31P, with sample rotation at 4 kHz. Spectra were recorded as a result of accumulating 10,000–20,000 transients with a 1-s recycle delay. A single crystal of MnCl (Mn2+) was added directly to the rotor and vortexed to ensure thorough mixing and a further spectrum obtained. Peptide/protein was added in 10 µl ddH2O to the required molar ratio and 31P MAS NMR spectra obtained. In control experiments the detergent Triton-X100 was added to the vesicle suspension in a 10 µl volume to a final concentration of 2 mM.
Electron microscopy
Morphologies of phospholipid vesicles alone and following addition of asyn to a lipid/peptide molar ratio of 1 000:1, with/without Mn2 + and EDTA (1 mM) were analysed by EM using negative staining methods (2% uranyl acetate). Lipid and peptide suspensions (10 µl) were loaded onto carbon coated nickel grids and visualized on a Tecnai EM at 100 kV with digital images produced. Sizes were measured using methods within the operating system.
Results
31P NMR-asyn
An assay was developed in which 31P NMR spectroscopy was used to test the effects of full-length and peptide fragments of asyn on the integrity and permeability of phospholipid vesicles. All phospholipids have an intrinsic NMR signal arising from 31P in their head groups and changes in 31P NMR peak widths or intensities after addition of paramagnetic ions to vesicles can be exploited to report on the permeability of vesicles. Previous studies using the phospholipids employed in this work have shown that DMPC/DOPG vesicles are found in liquid-crystalline fluid phase suitable for the analysis performed Citation[12], Citation[18], Citation[19]. The principle of the NMR assay is demonstrated below.
The 31P NMR spectrum of a DMPC/DOPG SUV suspension in the absence of Mn2 + (, top) shows two major peaks, one for DMPC at ∼0.2 ppm and the other from DOPG at ∼ −1 ppm. Each peak is split into two further components representing the head-groups of DMPC and DOPG from the inner and outer leaflets of the bilayer. The two components reflect non-degeneracy of the chemical shifts for the two bilayer leaflets arising from differences in membrane curvature and pH on the vesicle exterior and interior Citation[20]. The addition of paramagnetic Mn2 + ions to the intact vesicles selectively broadens out the signals from the exposed outer leaflet of the bilayer leaving peaks only from the inner bilayer leaflet (, middle). Disruption of the vesicles, achieved in the example here by the addition of the detergent Triton-X100, completely abolishes the NMR signal because now both leaflets of the bilayer are now exposed to Mn2 + (, bottom). Hence, the peak intensities observed before and after the addition of asyn are diagnostic of any increase in permeability to Mn2 + that is induced by the protein, which can be taken as an indication of vesicle disruption.
Figure 1. An assay using 31P MAS NMR to investigate the effects of asyn on the permeability of phospholipid vesicles. The spectrum of SUVs of DMPC/DOPG at a 2:1 molar ratio shows peaks from the two lipid components on the inside (PCi, PGi) and outside (PCo, PGo) of the vesicles (top). Addition of Mn2 + to the vesicles selectively broadens the peaks from the exposed lipids on the vesicle exterior leaving visible only the peaks from the interior lipids (middle). Subsequent addition of Triton-X100 breaks open the vesicles, which exposes both leaflets of the bilayer to Mn2 + and no signal is observed (bottom).
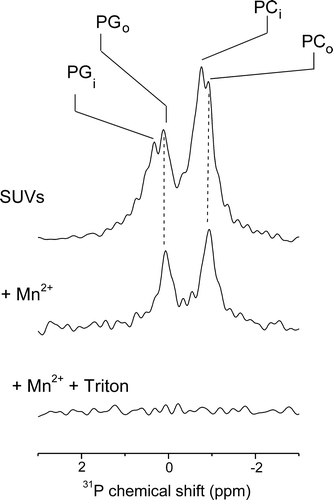
a shows 31P NMR spectra of phospholipid vesicles in the presence of Mn2 + (i.e. peaks present from the inner bilayer leaflet only) before and after the addition of asyn to a lipid/protein molar ratio of 1 000:1. The signal observed after the addition of protein is a measure of the amount of intact vesicles remaining. The top spectrum, corresponding to 20-nm diameter SUVs composed of DOPC and DOPG in a 2:1 molar ratio, exhibit a small (∼8%) reduction in intensity upon addition of asyn, suggesting that asyn has little effect on vesicle integrity at this protein concentration. In contrast, spectra corresponding to SUVs and LUVs composed of DMPC/DOPG show a larger reduction in peak intensity (∼80%) following the addition of asyn, consistent with significant permeabilization of the vesicles. The spectrum corresponding to SUVs composed of DOPC alone shows a reduction in peak intensity by ∼60% following the addition of asyn. Interestingly, addition of asyn to SUVs composed of DMPC alone completely abolished the NMR signal, suggesting that asyn completely permeabilizes PC vesicles composed of saturated myristoyl chains. To investigate the effect of vesicle size on the permeabilizing effect of asyn the experiment was repeated using 200-nm diameter LUVs of DMPC/DOPG (a, bottom). The individual phospholipid peaks from LUVs cannot be defined due to reduced membrane tumbling and lateral diffusion, preventing efficient averaging of anisotropic motion within the vesicle suspension Citation[21]. The spectrum nevertheless showed a reduction in overall intensity of ∼80% after adding asyn to a lipid/protein molar ratio of 1 000:1, which is similar to the reduction observed for the SUVs of the same lipid composition. The 31P NMR experiment thus suggests that, all other parameters being equal, vesicle size does not influence the permeabilizing effect of asyn.
Figure 2. Results of 31P MAS NMR experiments to investigate the effects of asyn on the permeability of phospholipid vesicles. Spectra of initially intact SUVs composed of DOPC/DOPG, DMPC/DOPG, DOPC, DMPC and LUVs of DMPC/DOPG, after addition of Mn2 + are shown before (left) and after (right) the addition of asyn to a lipid/protein molar ratio of 1 000:1 (a). The signal observed after the addition of protein is a measure of the amount of intact vesicles remaining. Figure b, summarizes the 31P signal intensity remaining after the addition of Mn2 + and asyn to lipid/protein molar ratios 2 000:1, 1 000:1, 900:1 and 800:1 of various phospholipid compositions as shown. Intensity values are scaled with lipid alone equal to 1. All experiments were performed at 30°C.
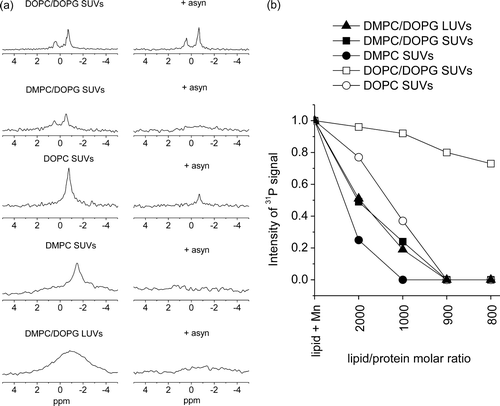
b shows a plot of 31P NMR peak intensities for SUVs of different lipid compositions (DMPC/DOPG, DMPC, DOPC/DOPG and DOPC) and LUVs of DMPC/DOPG at various lipid/asyn ratios. The results indicate that vesicles of PC, alone or mixed with DOPG, are more prone to permeabilization by asyn when PC has saturated myristoyl chains than when the lipid has unsaturated (and longer) oleoyl chains. The measurement temperature of 30°C is only slightly higher than the melting temperature (Tm) of pure DMPC myristoyl chains (23°C), and asyn could induce phase changes or lipid domain formation which disrupt the vesicles. Previous 31P NMR spectra of DMPC/DOPC vesicles at 4°C were consistent with asyn organizing the lipids into DMPC-rich clusters with gel-like properties Citation[12]. It is also possible that DMPC, having a shorter hydrocarbon chain than DOPC, imposes a higher internal potential energy strain on the lipid bilayer which is relieved when asyn associates with the surface and breaks open the vesicles. Both SUVs and LUVs of DMPC/DOPG are affected by asyn to the same extent, suggesting that curvature strain is not important. The peak intensity plot (b) also shows that SUVs composed of DOPC or DMPC alone are more prone to permeabilization by asyn than are DMPC/DOPG or DOPC/DOPG vesicles, respectively. This observation is rather surprising because asyn has previously been shown to interact preferentially with SUVs containing anionic lipids Citation[12], Citation[22], showing weak association with zwitterionic SUVs.
31P NMR – peptide fragments of asyn
To examine which regions of asyn could contribute to destabilizing the vesicles, the 31P NMR experiments were repeated on SUVs of DMPC/DOPG before and after adding peptides representing residues from the N-terminal (10–48), central (71–82) and C-terminal (120–140) segments of the protein. Previous work has shown that the basic asyn(10–48) interacts with the negatively charged surface of DMPC/DOPG multilamellar vesicles and orders the lipids into clusters that can be resolved by 31P NMR at 4°C Citation[12]. Sub-microscopic reorganization of the lipid components in this way, whilst not affecting the lamellar structure of the membrane, may have consequences for vesicle permeability and integrity. In 31P NMR spectra of SUVs containing DMPC/DOPG in the presence of Mn2 + are shown before and after the addition of asyn(10–48), asyn(71–82) and asyn(120–140) at a lipid/peptide molar ratio of 25:1. The addition of asyn(10–48) was accompanied by an approximate 20% reduction in the peak intensities, indicating that a fraction of the vesicles were fractured or permeabilized by the peptide. The addition of asyn(71–82) had a larger effect upon vesicle integrity, reducing the peak intensities by ∼45%. By contrast, asyn(120–140) had no effect upon vesicle integrity, which is consistent with previous observations that asyn(120–140) does not associate with multilamellar vesicles of DMPC/DOPG Citation[12]. The results of these experiments on the peptide fragments substantiate our previous observations that the N-terminal region of asyn interacts with DMPC/DOPG more strongly than does the C-terminal region. The peptide asyn(71–82) was also examined with DMPC SUVs at the same lipid/peptide molar ratio of 25:1 (, bottom). Here, complete loss of signal is observed suggesting a greater effect of asyn(71–82) on the integrity of zwitterionic DMPC vesicles than anionic DMPC/DOPG vesicles despite containing a positively charged lysine residue. Insufficient quantities of asyn(10–48) and asyn(120–140) were available to examine their effects on the DMPC vesicles.
Figure 3. Investigation of the association of asyn peptide fragments with phospholipid vesicles. Spectra of initially intact vesicles after addition of Mn2 + are shown before (left) and after (right) the addition of asyn(10–48), asyn(71–82) or asyn(120–140) at a lipid/peptide molar ratio of 25:1. As above, the signal observed after the addition of peptide is a measure of the amount of intact vesicles remaining.
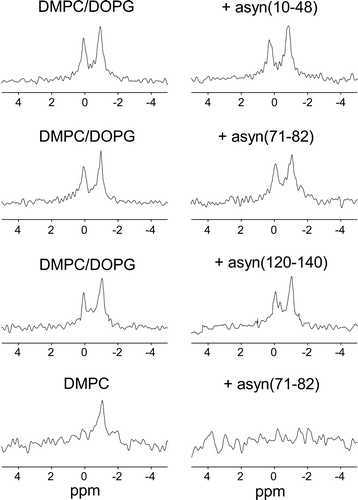
Morphological analysis
EM was used to examine how vesicle permeabilization by asyn relates to the morphology of the vesicles. In the absence of protein all the vesicle suspensions contained uniform, spherical lipid structures (a and a) with the SUVs having a diameter of ∼50–100 nm and the LUVs having a diameter of ∼200–250 nm.
Figure 4. EM images of mixed phospholipid vesicles alone (a), upon addition of Mn2 + (b), in the presence of asyn and Mn2 + (c), and in the presence of asyn with 1 mM EDTA (d). Lipid/protein molar ratios; 1000:1. Scale bars 200 nm.
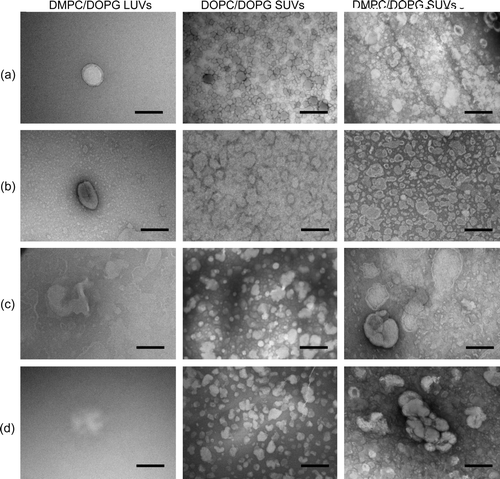
Figure 5. EM images of zwitterionic phospholipid vesicles alone (a), upon addition of Mn2 + (b), in the presence of asyn and Mn2 + (c), and in the presence of asyn with 1 mM EDTA (d). Lipid/protein molar ratios; 1 000:1. Scale bars 200 nm.
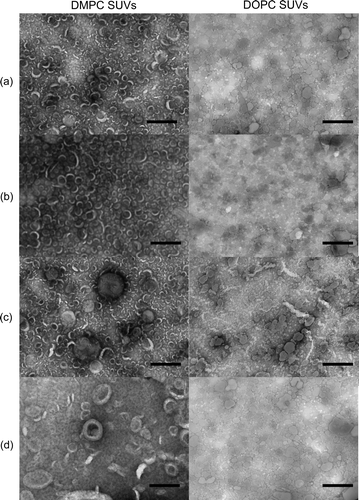
Manganese ions have been shown to induce fusion and fragmentation of vesicles Citation[23], Citation[24]. Vesicle suspensions were examined by EM in the presence of Mn2 + under the same conditions as used for the NMR experiments to eliminate the possibility that Mn2 + may be causing morphological changes in the vesicles that result in the observed changes in vesicle permeability (b and b). The morphology of the vesicles was not affected by the presence of Mn2 + alone. Further EM images were produced for the various vesicle compositions studied above following the addition of asyn to a lipid/protein molar ratio of 1 000:1 (c and c). The morphology of the DOPC/DOPG SUVs was not affected by asyn, consistent with the low permeabilizing effect of the protein on these vesicles. In contrast, addition of asyn to DMPC/DOPG SUVs and LUVs appears to induce pronounced changes in vesicle morphology. SUVs appear to undergo vesicle fusion to give a variety of vesicle sizes; ranging from individual vesicles (∼100–200 nm) to multi-vesicle structures with size ∼500 nm. However, LUVs appear to rupture rather than fuse upon addition of asyn, possibly due to a greater distance between individual vesicles impeding the fusion process. Morphological analysis of the zwitterionionic phosphatidylcholine SUVs were also consistent with NMR data, indicating significant fusion of DMPC vesicles and less morphological disruption of the DOPC vesicles. Similar effects were observed for vesicles upon addition of EDTA (d and d), confirming that fusion was mediated by the protein alone and not in conjunction with divalent cations (e.g. Ca2 + ), which have been shown to be involved in promoting vesicle association Citation[25]. Taken together the EM images confirm speculations drawn from NMR data suggesting that asyn is capable of altering vesicle integrity and permeabilizing phospholipid vesicles composed of DMPC alone or mixed with DOPG, with a small effect on DOPC SUVs, but no effect on DOPC/DOPG vesicles.
Discussion and conclusion
The effects of asyn and associated peptide fragments on the membrane integrity of lipid vesicles varying in composition and size have been studied here. SUVs have dimensions similar to those of presynaptic vesicles, making them a useful model system to provide insight into how asyn might interact with vesicles within the brain. The membrane binding affinity of asyn has previously been shown to vary according to lipid composition, which may help to direct the protein to where it is required Citation[22]. Membrane interactions of asyn appear to be tightly regulated within neurons, with hydrophobic and electrostatic interactions acting together to maintain association Citation[14]. In the neuronal environment, presynaptic vesicle destabilization by asyn could have implications for vesicle synthesis, membrane fusion and for neurotransmitter release.
Studies of the membrane binding properties of asyn have shown that the protein binds strongly to SUVs of acidic phospholipids Citation[26], but not to SUVs of phosphatidylcholine Citation[22]. By contrast, asyn has been shown to bind to LUVs of phosphatidylcholine with similar affinity as for LUVs of acidic lipids Citation[27]. Our previous work Citation[12] showed that asyn associates with multilamellar phospholipid vesicles containing lipids with anionic head-groups and more weakly with membranes of zwitterionic phosphatidylcholine. The lysine-rich peptide asyn(10–48) was found to associate with lipid head–groups with a preference for a negative membrane surface charge, whereas the acidic peptide asyn(120–140) has a slightly higher affinity for membranes with no net surface charge. From these findings it was speculated that the N-terminal and C-terminal domains of asyn may contribute to the affinity and selectivity with which the protein interacts with lipid membranes. Our earlier work focused on multilamellar vesicles, which, although convenient models of cellular membranes, are not representative of presynaptic vesicles and are unsuitable for monitoring the effects of asyn on vesicle permeability.
Here we show that the association of asyn with PC and PC/DOPG SUVs can increase vesicle permeability at protein/lipid molar ratios as low as 1:2 000. The results presented suggest that lipid vesicles are permeabilized by asyn to an extent that depends upon the lipid composition, with vesicle size being less critical. Surprisingly, the extent of vesicle permeabilization is greater for pure phosphatidylcholine vesicles than for vesicles of the same lipids (DOPC or DMPC) mixed with DOPG. Anionic lipids therefore appear to moderate the disruptive effect of the protein under the conditions studied here. This suggests that the effect is not related simply to the affinity of asyn for the membrane surface, because it is generally accepted that the protein associates preferentially with anionic lipids and more weakly with zwitterionic lipids. The results also imply that the lysine-rich N-terminal region is less likely to contribute to vesicle permeabilization than is the more acidic C-terminus, which prefers neutral membrane surfaces. In fact, the three peptides asyn(10–48), asyn(71–82) and asyn(120–140) have only a weak permeabilizing effect (< 50%) on DMPC/DOPG vesicles at the relatively high protein to lipid ratio of 1:25 (), suggesting that in full length asyn different regions must act in concert to achieve the larger effects observed on the DMPC/DOPG vesicles at much lower protein/lipid ratios (). By contrast, the peptide asyn(71–82), comprising residues VTGVTAVAQKTV from the core amyloidogenic region of asyn, completely permeabilizes DMPC vesicles when the peptide added in an initial monomeric form. The peptide has a formal charge of +1 and so would be expected to have a slight preference for anionic lipid headgroups over zwitterionic headgroups. The larger effect of asyn(71–82) on DMPC vesicles indicates that permeabilization by this peptide, and perhaps also by full-length asyn, may not be mediated by headgroup interactions but instead by destabilizing hydrophobic contacts between the nonpolar side groups and the bilayer interior. Modelling of a peptide representing residues 67–78 of asyn, which also induces vesicle leakage, predicts a tilted orientation within lipid bilayers, with the bulk of the peptide penetrating the bilayer interior Citation[28]. A similar orientation can be envisaged for asyn(71–82), with the polar Glu and Lys side groups oriented toward the bilayer surface and the nonpolar residues exerting a destabilizing effect deeper within the bilayer. The presence of a negative surface charge in PC/DOPG vesicles may present an electrostatic barrier that hinders the insertion of the nonpolar portion of the peptide into the bilayer. Similarly the central hydrophobic region of full-length asyn may penetrate more deeply into the bilayer when the surface charge is low. The effects of asyn on membranes containing DMPC were generally greater than on membranes containing DOPC, which indicates that the disruptive properties of asyn may be influenced by differences in the fluidity, surface area or thickness of phospholipid vesicles imposed by the chain composition. In the case of the DMPC/DOPG vesicles, asyn interactions may relieve additional strain energy imposed by the mixture of C14 and C18 hydrocarbon chains, which must adapt their segmental order parameters to maintain a uniform bilayer thickness. The importance of the chain composition again points to hydrophobic protein-lipid interactions as being important for membrane disruption.
Analysis of the vesicles by EM reveals that the extent of permeabilization by asyn broadly reflects the changes in morphology of the vesicles. The vesicles disrupted to the largest extent by asyn appear to fuse together to produce larger, asymmetric structures (DMPC and DMPC/DOPG SUVs) or rupture (DMPC/DOPG LUVs). Differences observed by EM between LUVs and SUVs could be explained by SUVs being closer together within vesicle suspensions compared with LUVs, enabling fusion to occur more readily. This effect occurs due to the filtering process used to prepare the LUVs. Formation of LUVs is usually achieved by filtering using a polycarbonate filter, but the concentrations required here are unsuitable for these filters. Cellulose acetate filters were used to obtain approximate size vesicles which were adequate for the experiments carried out. However, this filtration method causes loss of lipid reducing the number of lipid molecules, resulting in vesicles being further apart within solution, as observed by EM.
Studies on peptides from viral fusion proteins indicate that many of the key properties of fusion peptides are similar to the characteristics of asyn. Fusion peptides generally have a destabilizing and membrane perturbing mode of action with the key regions being hydrophobic and rich in gly residues Citation[29]. Fusion proteins are found to be structured often with α-helical conformations required for insertion into the membrane due to unfavourable insertion of non-hydrogen bonded amide groups Citation[30]. It is well established that the N-terminus of asyn preferentially forms an α-helix when associated with lipid membranes Citation[22]. Other studies suggest that fusion peptides are able to associate to form multimeric structures on membrane surfaces in a β-sheet dependent manner Citation[31]. This is also a characteristic that can be applied to observations that asyn aggregates with β-sheet conformation at membrane surfaces Citation[5], Citation[32]. In order to act as a fusion peptide asyn must be able to insert within the membrane to exert its influence within the membrane core. Paramagnetic line broadening experiments have been used to probe penetration of asyn into membrane regions, and indicate that the regions around residues 42 and 68–79 are found within the membrane interior Citation[33]. These residues are found within the peptides asyn(10–48) and asyn(71–82), consistent with indications within this study that these peptide fragments are capable of altering phospholipid membrane integrity of DMPC/DOPG SUVs. The so-called tilted peptide corresponding to residues 67–78 of asyn also induces fusion and leakage of both neutral and anionic SUVs Citation[28]. Taken together this suggests that the N-terminal and central regions may act within full–length asyn to initiate destabilization of phospholipid vesicles. Another identified property of fusion peptides is that small mutations in sequence can alter the membrane interaction of the peptide. Properties of asyn can again be applied to this criteria due to known disease-associated mutations, e.g. A30P and A53T, changing membrane associative ability Citation[8].
It is noted that the mixture of lipids used in the model vesicles does not reflect the physiological composition of presynaptic vesicles. These results do, however, demonstrate that for certain vesicle compositions asyn has permeabilizing and/or fusogenic properties, whereas for other compositions the effect is less pronounced. Here, the effect can be attributed to lipid chain mismatch or to domain formation, both events that can impose substantial strain on the lipid bilayer that can be relieved by vesicle rupture or fusion. This property of asyn to selectively disrupt vesicles may be involved within the proposed role maintaining synaptic vesicle pools and neurotransmitter release.
The ability of asyn to function as a fusion protein is consistent with a role for asyn in presynaptic vesicle cycling involving vesicle maintenance, fusion and transport upon synaptic stimulation. Asyn knockout mice show impairment of vesicle cycling, observed as a reduced response to prolonged synaptic stimulation Citation[34]. Biochemical analysis of cytoplasmic protein extracted from rat brains estimates that asyn is present in vivo at a concentration less than 30–60 µM Citation[35], representing 0.5–1% of total cytoplasmic protein. Due to the high levels of presynaptic vesicles relative to asyn concentration in vivo, it may be necessary for destabilizing interactions between asyn and lipid membranes to occur at low protein/lipid ratios to have a physiological effect. Our results show that the surface charge density of the vesicles may also modulate the disruptive effect of asyn.
The novel method of measuring membrane permeability presented here using 31P NMR can be employed to study how peptide-lipid interactions affect vesicle integrity for a variety of uses; including drug development and understanding biophysical properties depicting how molecules interact with lipids.
Acknowledgements
This work was supported financially with a PhD studentship, equipment grant and fellowship to JM from the Alzheimer's Research Trust. The BBSRC is acknowledged for funding in support of the NMR facility. Thanks to the Biomedical EM-unit at the University of Liverpool for assistance with instrument operation. Declaration of interest: The authors report no conflicts of interest. The authors alone are responsible for the content and writing of the paper.
References
- Spillantini LC, Schmidt ML, Lee VMY, Trojanowski JQ, Jakes R, Goedert M. α-Synuclein in Lewy bodies. Nature 1997; 388: 839–840
- Murphy DD, Rueter SM, Trojanowski JQ, Lee VMY. Synucleins are developmentally expressed, and α-synuclein regulates the size of the presynaptic vesicular pool in primary hippocampal neurons. J Neurosci 2000; 20: 3214–3220
- George JM, Jin H, Woods WS, Clayton DF. Characterization of a novel protein regulated during the critical period for song learning in the zebra finch. Neuron 1995; 15: 361–372
- Lotharius J, Brundin P. Impaired dopamine storage resulting from α-synuclein mutations may contribute to the pathogenesis of Parkinson's disease. Human Molec Genetics 2002; 11: 2395–2407
- Jo E, Darabie AA, Han K, Tandon A, Fraser PE, McLaurin J. α-Synuclein-synaptosomal membrane interactions. Eur J Biochem 2004; 271: 3180–3189
- Zhu M, Fink AL. Lipid binding inhibits α-synuclein fibril formation. J Biological Chem 2003; 278: 16873–16877
- Volles MJ, Lansbury PTJ. Vesicle permeabilization by protofibrillar α-synuclein is sensitive to Parkinson's disease-linked mutations and occurs by a pore-like mechanism. Biochemistry 2002; 41: 4595–4602
- Jo E, McLaurin J, Yip CM, George-Hyslop P St, Fraser PE. 2000. α-Synuclein membrane interactions and lipid specificity. J Biological Chem 275:34328–34334.
- Lashuel HA, Petre BM, Wall J, Simon M, Nowark RJ, Walz T, Lansbury PT Jnr. 2002. α-Synuclein, especially the Parkinson's disease-associated mutants, forms pore-like annular and tubular protofibrils. J Molec Biol 322:1089–1102.
- Ding TT, Lee SJ, Rochet JC, Lansbury PT Jnr. 2002. Annular α-synuclein protofibrils are produced when spherical protofibrils are incubated in solution or bound to brain-derived membranes. Biochemistry 41:10209–10217.
- Volles MJ, Lee S-J, Rochet J-C, Shtilerman MD, Ding TT, Kessler JC, Lansbury PT Jnr. 2001. Vesicle permeabilisation by protofibrillar α-synuclein: implications for the pathogenesis and treatment of Parkinson's disease. Biochemistry 40:812–7819.
- Madine J, Doig AJ, Middleton DA. A study of the regional effects of α-synuclein on the organisation and stability of phospholipid bilayers. Biochemistry 2006; 45: 5783–5792
- Ramakrishnan M, Jensen PH, Marsh D. α-Synuclein association with phosphatidylglycerol probed by lipid spin labels. Biochemistry 2003; 42: 12919–12926
- Zhu M, Li J, Fink AL. The association of α-synuclein with membranes affects bilayer structure, stability, and fibril formation. J Biological Chem 2003; 278: 40186–40197
- Michaelson DM, Barkai G, Brenholz Y. Asymmetry of lipid organization in cholinergic synaptic vesicle membranes. Biochem J 1983; 211: 155–162
- Takamori S, Holt M, Stenius K, Lemke EA, Gronborg M, Riedel D, Urlaub H, Schenck S, Brugger B, Ringler P, Muller SA, Rammner B, Grater F, Hub JS, De Groot BL, Mieskes G, Moriyama Y, Klingauf J, Grubmuller H, Heuser J, Wieland F, Jahn R. Molecular anatomy of a trafficking organelle. Cell 2006; 127: 831–846
- Barenholz Y, Gibbes D, Litman BJ, Goll J, Thompson TE, Carlson FD. A simple method for the preparation of homogeneous phospholipid vesicles. Biochemistry 1977; 16: 2806–2810
- Jack ER, Madine J, Lian LY, Middleton DA. Membrane interactions of peptides representing the polybasic regions of three Rho GTPases are sensitive to the distribution of arginine and lysine residues. Molec Membrane Biol 2008; 25: 14–22
- Clayton JC, Hughes E, Middleton DA. The cytoplasmic domains of phospholamban and phospholemman associate with phospholipid membrane surfaces. 2005; 44: 17016–17026
- Swairjo MA, Seaton BA, Roberts MF. Effect of vesicle composition and curvature on the dissociation of phosphatidic acid in small unilamellar vesicles – a 31P NMR study. Biochim Biophys Acta 1191:354–361.
- Burnell EE, Cullis PR, De Kruijff B. Effects of tumbling and lateral diffusion on phosphatidylcholine model membarne 31P-NMR lineshapes. Biochim Biophys Acta 1980; 603: 63–69
- Davidson WS, Jonas A, Clayton DF, George JM. Stabilization of a-synuclein secondary structure upon binding to synthetic membranes. J Biological Chem 1998; 273: 9443–9449
- Hemakanthi G, Dhathathreyan A. Fusion of vesicles in manganese complex of a single-chain schiff base amphiphile-3-cyano-N-benzylidene hexacylamine. J Colloid Interface Sci 2002; 253: 393–396
- Towler MC, Prescott AR, James J, Lucocq JM, Ponnambalam S. The manganese cation disrupts membrane dynamics along the secretory pathway. Experim Cell Res 2000; 259: 167–179
- Jeremic A, Kelly M, Cho JA, Cho S-J, Horber H, Jena BP. Calcium drives fusion of SNARE-apposed bilayers. Cell Biol Int 2004; 28: 19–31
- Chandra S, Chen X, Rizo J, Jahn R, Sudhof TC. A broken α-helix in folded α-synuclein. J Biological Chem 2003; 278: 15313–15318
- Narayanan V, Scarlata S. Membrane binding and self-association of α-synuclein. Biochemistry 2001; 40: 9927–9934
- Crowet J-M, Lins L, Dupiereux I, Elmoualija B, Lorin A, Charloteaux B, Stroobant V, Heinen E, Brasseur R. In silico tilted properties of the 67–78 fragment of α-synuclein are responsible for membrane destabilisation and neurotoxicity. Proteins 2007; 68: 936–947
- Epand RM. Fusion peptides and the mechanism of viral fusion. Biochim Biophys Acta 2003; 1614: 116–121
- Wimley WC, White SH. Experimentally determined hydrophobicity scale for proteins at membrane interfaces. Nature Struct Biol 2001; 3: 842–848
- Yang J, Gabrys CM, Weliky DP. Solid-state nuclear magentic resonance evidence for an extended β strand conformation of the membrane-bound HIV-1 fusion peptide. Biochemistry 2001; 40: 8126–8137
- Cole NB, Murphy DD, Grider T, Rueter S, Brasaemle D, Nussbaum RL. Lipid droplet binding and oligomerization properties of the Parkinson's disease protein α-synuclein. J Biological Chem 2002; 277: 6344–6352
- Bussell R, Ramlall TFJnr, Eliezer D. Helix periodicity, topology and dynamics of membrane-associated α-Synuclein. Protein Sci 2005; 14: 862–872
- Abeliovich A, Schmitz Y, Farinas I, Choi-Lundberg D, Ho W-H, Castillo PE, Shinsky N, Manuel Garcia Verdugo J, Armanini M, Ryan A, Hynes M, Philips H, Sulzer D, Rosenthal A. Mice lacking α-synuclein display functional deficits in the nigrostriatal dopamine system. Neuron 2000; 25: 239–252
- Iwai A, Masliah E, Yoshimoto M, Ge N, Flanagan L, Rohan de Silva HA, Kittel A, Saitoh T. The precursor protein of non-Aβ Component of Alzheimer's disease amyloid is a presynaptic protein of the central nervous system. Neuron 1995; 14: 467–475