Abstract
For many types of cells, an increase in cell density leads to characteristic changes in intracellular signalling and cell function. It is unknown, however, whether cell density affects the function of T lymphocytes. It is presented here that aggregation of Jurkat T cells, murine thymocytes or human peripheral blood T cells, results in gradual modification of the Lck tyrosine kinase. Within one hour of aggregation, Lck in the detergent-insoluble lipid raft fraction is dephosphorylated mainly at the carboxy-terminal tyrosine. Further aggregation leads to gradual loss of Lck protein from both lipid raft and non-raft fractions which is accompanied by increased protein ubiquitination, a process that is more evident in the detergent-soluble fraction. In contrast, the expression of LAT, which like Lck distributes to raft and non-raft membrane, or Csk, a kinase with a structure similar to Lck, is not affected by cell aggregation. Dephosphorylation of lipid raft-associated Lck, albeit with reduced kinetics, is observed in aggregated Jurkat CD45-deficient cells as well, suggesting involvement of additional tyrosine phosphatases. Changes in Lck structure and expression correlate with reduced ability of aggregated cells to fully activate protein tyrosine phosphorylation after stimulation of the TCR, and with changes in the activation of down-stream signalling cascades.
Abbreviations | ||
pY | = | phospho-tyrosine |
TCR | = | T cell receptor |
Ub | = | ubiquitin |
mAb | = | monoclonal antibody |
MβCD | = | methyl-β-cyclodextrin |
CTB | = | cholera toxin B subunit; PHA, phytohaemagglutinin |
Introduction
Lck is a member of the Src family of tyrosine kinases that couples the T cell antigen receptor (TCR) to intracellular signalling cascades Citation[1]. Upon receptor stimulation, Lck initiates signalling by phosphorylating the chains of the TCR resulting in recruitment of the cytosolic tyrosine kinase ZAP-70 and subsequent phosphorylation of other downstream protein targets Citation[2]. One such substrate is the adapter molecule LAT (linker for activation of T cells), which when phosphorylated it promotes the assembly of signalling molecules at the plasma membrane Citation[3]. The generation of such signalling complexes controls downstream events including stimulation of mitogen-activated protein kinase pathways (MAPK) and production of second messengers Citation[4].
Dual amino-terminal acylation directs localization of Lck to the inner leaflet of the plasma membrane Citation[5–7]. The same modification controls partitioning of Lck into lipid raft microdomains Citation[5], Citation[7]. Lipids rafts are specialized plasma membrane domains that have distinct lipid composition and are considered to participate in various biological processes including signal transduction Citation[8]. They promote lateral compartmentalization at the plasma membrane by attracting certain proteins while excluding others. This segmentation has an important role in maintaining signalling homeostasis, but also, upon receptor stimulation, lipid rafts support signalling by facilitating the transient assembly of protein complexes. In T cells, numerous studies have suggested a role for lipid rafts during TCR-mediated signalling Citation[7], Citation[9–11].
The catalytic activity of Lck, and of the other members of the Src family of kinases, is regulated by tyrosine phosphorylation (reviewed in Citation[12]). A ‘closed’, low-activity configuration of the enzyme is induced by the phosphorylation of a conserved carboxy-terminal (C-terminal) tyrosine (Y505 in mouse Lck protein) Citation[13], while auto-phosphorylation of a second tyrosine (Y394) within the ‘activation loop’ of the kinase domain, forces the molecule to adopt an ‘open’ conformation Citation[14]. Lck that co-purifies with detergent-resistant lipid rafts was found predominantly in the ‘closed’ configuration while in the detergent-soluble fraction Lck was mainly in the ‘open’ structure Citation[15–17]. This difference between the two pools of Lck could be due to different Lck-regulating proteins present in the two fractions. For example, the tyrosine phosphatase CD45, which dephosphorylates Y505, is found primarily in the detergent-soluble fraction Citation[9], while lipid rafts contain the adapter molecule Protein Associated with GEMs/Csk binding protein (PAG/Cbp) which recruits Csk to phosphorylate Y505 on Lck Citation[18], Citation[19].
Src family kinases are also regulated by ubiquitination. In T cells, co-stimulation of the TCR and CD4 receptors induced the association of the ubiquitin ligase c-Cbl with Lck, which was accompanied by Lck poly-ubiquitination and degradation Citation[20]. Interestingly, in another study it was shown that Lck interaction with c-Cbl negatively regulates partitioning of Lck into lipid raft domains Citation[21].
Culturing cells at high density generates signals which modulate the activity of intracellular signalling pathways Citation[22], Citation[23]. This event has been mostly studied in endothelial cells, which when cultured at high density, stop proliferating and respond poorly to growth factor stimulation Citation[24], Citation[25]. To our knowledge, there is no information on whether high cell density can regulate T cell functions. In the course of our studies, we noticed changes in Lck tyrosine phosphorylation when T cells were aggregated. This prompted us to further study the effects of cell density on T cell function and here we show that aggregation results initially in the dephosphorylation of raft-associated Lck and subsequently in its ubiquitination and loss of protein expression. These changes have functional consequences on signal transduction via the TCR.
Materials and methods
Cells and animals
The E6-1 clone of the Jurkat T cell line and its CD45-deficient derivative J45.01 were grown in RPMI-1640 supplemented with 5% foetal calf serum (FCS) (Invitrogen, Carlsbad, CA), 2 mM L-glutamine, 100 U/ml penicillin and 100 µg/ml streptomycin. Human peripheral blood T lymphocytes were obtained from healthy volunteers. Briefly, mononuclear cells were separated from heparinized blood by centrifugation over Ficoll-Hypaque (Pharmacia Biotech, Bucks, UK) and T cells were positively selected following incubation of the mononuclear cells with anti-CD3 coated magnetic beads (Dynal, Oslo, Norway). Purified cells were placed in culture for 24 h before an aggregation experiment. FACS analysis showed that >90% of the cells were T lymphocytes. For experiments with murine thymocytes, single cell suspension was obtained from thymi from 8–12-week-old DBA/1 mice (Harlan Olac, Bicester, UK) by passing the specimens through a cell strainer.
Antibodies and other reagents
Anti-Lck monoclonal (mAb), 3A5, and polyclonal antibodies to Lck, Fyn, Csk and ubiquitin were purchased from Santa Cruz Biotechnology (Santa Cruz, CA). Polyclonal antibodies specific for the phosphorylated forms of Lck Tyr505 (pY505Lck), ERK1/2 and p38 were from Cell Signalling Technology (Beverly, MA), while anti-LAT and the anti-phosphotyrosine (pY) mAb 4G10 were purchased from Upstate Biotechnology (Lake Placid, NY). The anti-CD3 (OKT3) and anti-CD28 (CD28.2) mAbs used for cell stimulation were from BD Biosciences Pharmingen (San Diego, CA).
Cell aggregation
Jurkat cells were maintained in 175 cm2 flasks and harvested for experiments when at the logarithmic phase of growth (2×105−1×106 cells/ml). Aggregation was performed by resuspending 20–40×106 cells in 2 ml of RPMI 1640/5% FCS medium and then placed in a 30 ml universal, conical-bottom tube and left to precipitate to the bottom of the tube in a tissue culture incubator adjusted to 5% CO2 atmosphere. At the time points shown in , cells were collected and either disrupted in lysis buffer or were further treated as indicated in Figure legends.
Figure 1. Changes in protein tyrosine phosphorylation induced by high cell density in lipid rafts. (A) Lipid rafts from Jurkat cells aggregated for the times indicated, were blotted with antibodies to phosphotyrosine (pY), Lck and LAT. (B) Lipid raft (LR) and detergent-soluble (DS) fractions were probed with antibodies to phosphorylated 505 tyrosine (p505) or Lck. Densitometric values of the protein bands are expressed as a ratio of p505/Lck, setting as reference (value of 1) the ratio in the DS fraction. (C) Confocal images of non-aggregated and 2-h aggregated cells doubly stained with anti-p505 (green) and CTB (red) or anti-Lck (green) and CTB (red). Co-localization is determined by the development of yellow colour. (D) Jurkat cells were incubated for 2 h in different culture dishes and probed with anti-pY, anti-Lck and anti-LAT antibodies.
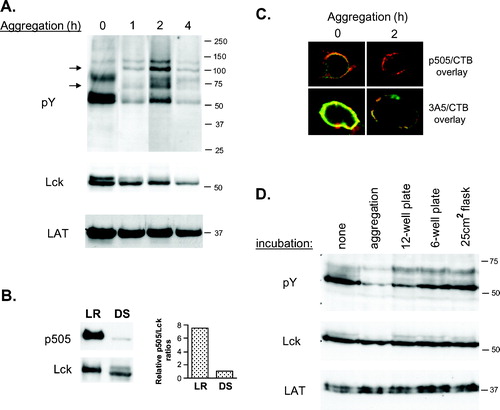
Stimulation of T cells with anti-CD3 and anti-CD28 mAbs
Cells (1×107 cells/ml) were aggregated for 0–4 h and then half of the cells were incubated with 2 µg/ml each of anti-CD3 and anti-CD28 mAbs on ice for 15 min, followed by a further 5 min incubation at 37°C after addition of 2 µg/ml of goat anti-mouse immunoglobulin (Ig) (BD Biosciences) to cross-link the TCR and co-receptor. The other half of the culture representing unstimulated cells received only goat anti-mouse Ig. Cells were then lysed in RIPA buffer (150 mM NaCl, 50 mM Tris pH 8.0, 1% NP-40, 0.5% deoxycholic acid and 0.1% SDS) containing protease inhibitors (5 µg/ml of each Chymostatin, Pepstatin A, Leupeptin, Aprotinin and 1 mM of AEBSF hydrochloride, all from Calbiochem, La Jolla, CA) and 10 mM of the phosphatase inhibitor, sodium orthovanadate (Sigma-Aldrich, Dorset, UK) and cell lysates were used for western analysis or immunoprecipitations.
Preparation of detergent-insoluble lipid rafts
To isolate detergent-resistant lipid raft membranes (LR) and detergent-soluble lysate (DS), cells were lysed in ice-cold MNE buffer (25 mM Mes pH 6.5, 150 mM NaCl, and 5 mM EDTA) containing 1% TritonX-100 plus protease and phosphatase inhibitors. Lysates were adjusted to 40% sucrose and overlaid with an equal volume of 30% sucrose followed by 1 ml of 5% sucrose solution and centrifuged overnight at 200,000 g at 4°C. Lipid raft membranes were collected from the 5–30% interface, the sucrose content was diluted with MNE buffer and lipid rafts were pelleted in a microcentrifuge at 14,000 g for 20 min. For subsequent experiments, the lipid raft pellets were resuspended in RIPA buffer containing 20 mM MβCD which extracts cholesterol and facilitates their complete disruption. The 40% heavy sucrose lysate was collected from the bottom of the tube.
Confocal microscopy
Briefly, cells were stained for lipid rafts with PE-conjugated cholera toxin fragment B (CTB) (2 µg/ml, Molecular Probes Inc, Eugene, OR, USA) for 15 min on ice and were subsequently, washed and fixed with 4% p-formaldehyde (PFA). Fixed cells were allowed to settle on microscopy slides pre-treated with poly-L-lysine, permeabilized with 0.2% Triton X-100 and blocked with 0.5% fish skin gelatine. Cells were incubated with the indicated primary antibodies (5 µg/ml) for 2 h at 4°C followed by secondary FITC-conjugated reagents. Single optical sections of stained cells were acquired using a Zeiss LSM510META confocal microscope (Jena, Germany) with images adjusted to the same output intensities.
Cell fractionation
Cells were disrupted in hypotonic buffer (10 mM Tris, pH 7.4, 2 mM EDTA) containing protease inhibitors and subjected to two freeze-thaw cycles after which the salt concentration was adjusted to 150 mM NaCl. Intact cells and nuclei were eliminated by centrifugation at 480× g for 5 min, and soluble and particulate fractions were generated by centrifugation at 100,000× g for 30 min. Equivalent portions from the two fractions were analysed by western blotting.
Results
Cell aggregation induces changes in the pattern of tyrosine phosphorylated proteins in T cell lipid raft
Changes were noticed in the level of tyrosine phosphorylation of proteins when Jurkat T cells were incubated at high cell density and in the absence of any exogenous stimulus. High cell density was achieved by resuspending 20–40×106 Jurkat T cells in 2 ml of culture medium and then allowing the cells to settle to the bottom of a universal tube. Cells were collected following incubation for 1, 2 and 4 h, detergent-insoluble lipid rafts were purified and tyrosine phosphorylated proteins were analysed by western blotting. Non-aggregated cells (0 h) are freshly harvested from a 175 cm2, tissue culture flask.
Two phosphoproteins were clearly visible in T cell lipid rafts migrating at approximately 55 and 70–80 kDa. Within 1 h of aggregation, substantial dephosphorylation of both bands was noticed which was maintained for up to 4 h, the longest time point of cell aggregation in our experiments (see A, top panel). In parallel to the dephosphorylation of these two bands, there was increased tyrosine phosphorylation of two other bands migrating at approximately 65 kDa and 110 kDa (arrows, A, top panel) that was first visible after 1 h of cell aggregation and for the duration of the experiment. Anti-Lck western analysis showed a gradual reduction of Lck protein expression over time (A, middle panel). In contrast, LAT expression was not significantly reduced (A, lower panel). Aggregation of cells for less than 1 h had no effect on the pattern of tyrosine phosphorylated proteins in lipid rafts (data not shown).
The 55 kDa phosphoprotein was previously shown to represent Lck that is phosphorylated primarily at the C-terminal tyrosine Citation[16], Citation[17]. To confirm this observation, comparable amounts of Lck from the LR and DS fractions were analysed with antibodies reactive to phosphorylated Y505 (p505). Densitometric analysis of the western blot in B showed approximately eight-fold higher Y505 phosphorylation in the lipid raft-associated Lck pool compared to Lck in the DS fraction (B). Furthermore, confocal images of cells revealed extensive co-localization of phospho-Y505 (green colour) with the lipid raft marker GM1, visualized with CTB (red colour) (C). This co-localization was strongly reduced in cells aggregated for 2 h due to the dephosphorylation of Y505 (C). Staining with the 3A5 anti-Lck mAb showed strong co-localization of Lck with lipid rafts in untreated and aggregated cells, however, the intensity of 3A5 stain was somewhat reduced in cells aggregated for 2 h probably due to degradation of Lck protein (see C).
To establish a relationship between cell density and reduction in Lck phosphorylation, Jurkat cells were cultured for 2 h in a universal tube as above, 12-well plate, 6-well plate or in a 25 cm2 flask, thus providing variable surface area for the cells to spread. As before, aggregation in a universal tube resulted in significant reduction in Lck phosphorylation (D). Substantial Lck dephosphorylation was also observed in cells placed in a 12-well dish, while no changes were evident in cells cultured in a 6-well plate or a 25 cm2 flask (D). Blotting of the membrane with specific antibodies showed decreased expression of Lck in aggregating cells, while there was no detectable reduction in LAT expression (D, middle and lower panels respectively).
Cell aggregation induces protein ubiquitination
One mechanism, via which expression levels of targeted proteins are regulated, is protein ubiquitination. To see if increased cell density induces protein ubiquitination, LR and DS aliquots representing 5×106 and 0.5×106 cells respectively from cells aggregated for 0–4 h were analysed with anti-ubiquitin (Ub) antibodies. Increased protein ubiquitination was seen within 1 h of cell aggregation in both fractions and was further elevated at later time points (see A). Western blotting of the same membrane with anti-Lck antibodies showed that the levels of Lck in lipid rafts were gradually decreased at longer cell incubations (A, Lck short exposure) and the same was true for non-raft lysates (A, Lck long exposure). There were no significant changes in LAT expression (A).
Figure 2. Induction of protein ubiquitination by cell aggregation. (A) LR and DS fractions from cells aggregated for the times shown were blotted with antibodies to ubiquitin (Ub), Lck and LAT. (B) Lck was immunoprecipitated from LR and DS fractions from cells aggregated for the times shown and western blotted with anti-Ub and anti-Lck antibodies. (C) Cells aggregated for 0, 1 and 2 h were disrupted in hypotonic buffer and the cytosolic (S100) and particulate (P100) fractions were probed with anti-Ub and anti-JNK1 antibodies. (D) Confocal sections of cells aggregated for 2 h, and double stained with anti-Ub antibodies (green) and CTB (red). Co-localization is determined by overlay of the images acquired in the two different channels. The bar indicates 5 µm.
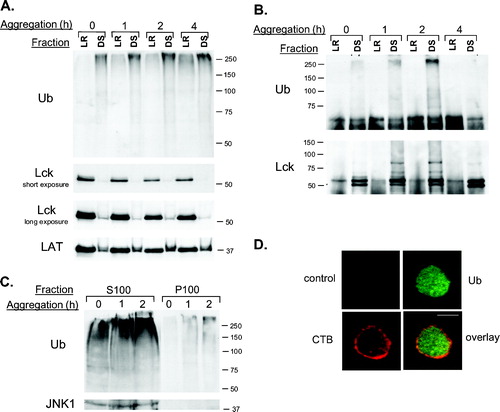
To see if one of the proteins that is ubiquitinated following cell aggregation is Lck, aliquots representing 30×106 and 39×106 cells from the LR and DS fractions respectively were used for Lck immunoprecipitations (see B). There was some Lck ubiquitination in the soluble lysate pool and in the absence of cell aggregation most likely reflecting physiological turnover of the protein in these cells. Lck ubiquitination increased in the DS fraction after 1 h of cell aggregation and there was further increase after 2 h, but surprisingly, there was a reduction after 4 h of cell aggregation (B, top panel). This result might suggest that aggregation-induced ubiquitination of Lck is transient, peaking at around 2 h. This is supported by our observation that Lck ubiquitination was visible in the DS fraction after 4 h of aggregation when the experiment was done in the presence of a proteasome inhibitor (data not shown). In contrast, there was no detectable ubiquitination of Lck co-purifying with lipid rafts (B) even at long exposures of the blot. The level of immunoprecipitated Lck in each sample was determined by probing the same membrane with anti-Lck antibodies (B, bottom panel). Less Lck was immunoprecipitated from LR samples because the raft-associated Lck pool is smaller compared to the pool in the DS fraction Citation[17]. In other experiments, up to 5×108 cells were used, however, again we were unable to detect Lck ubiquitination in lipid rafts (data not shown). Despite these efforts, we cannot exclude the possibility that Lck ubiquitination takes place in these domains but at levels that cannot be detected with the immunoprecipitation/western blotting method used here.
To further explore the sub-cellular compartmentalization of ubiquitination, cytosolic and particulate, fractions of cells aggregated for 0, 1 and 2 h were western blotted with anti-ubiquitin antibodies. As seen in C, the vast majority of ubiquitinated proteins were detected in the cytosolic (S100) fraction. However, a small but clearly visible signal was detectable in the particulate (P100) fraction as well especially after 2 h of aggregation (see C). Western blotting of the membrane for JNK1 shows equivalent protein loading. In addition, confocal images of cells aggregated for 2 h and doubly stained with anti-ubiquitin antibodies (green) and CTB (red), shows broad distribution of ubiquitinated proteins in the cell, and some small but detectable co-localization with lipid rafts (yellow) (see D).
Protein ubiquitination induced by high cell density in thymocytes and normal T cells
To establish that changes induced by aggregation is a physiologic response of T cells to high cell density, aggregation experiments were repeated with murine thymocytes and human peripheral blood T cells. LR and DS fractions representing 20×106 and 1×106 cells respectively from thymocytes aggregated for 0–4 h, were probed sequentially with antibodies to pY, Ub, Lck and LAT. Dephosphorylation of Lck in lipid rafts was visible as early as 2 h after the initiation of aggregation (see A, top panel), while in the DS fraction there was an increase in ubiquitination (A, second panel). Protein ubiquitination was detectable in the lipid rafts as well after 4 h of thymocyte aggregation (A, second panel). Western blotting with anti-Lck antibodies showed a gradual reduction in the levels Lck protein expressed in both fractions, which is similar to the results obtained with Jurkat cells (A, third panel). Over the course of three experiments, no significant reduction in LAT protein expression was noted after cell aggregation (A, bottom panel). Similarly, a 2-h aggregation of human T cells resulted in increased protein ubiquitination as well as a small but detectable reduction in Lck expression (see B).
Figure 3. Aggregation induced changes in murine thymocytes and human peripheral blood T cells. (A) The LR and DS fractions from thymocytes aggregated for 0–4 h were sequentially probed with antibodies to pY, Ub, Lck and LAT. (B) Purified human peripheral blood T cells were aggregated for 0 or 2 h and lysates were western blotted with anti-Ub and anti-Lck antibodies.
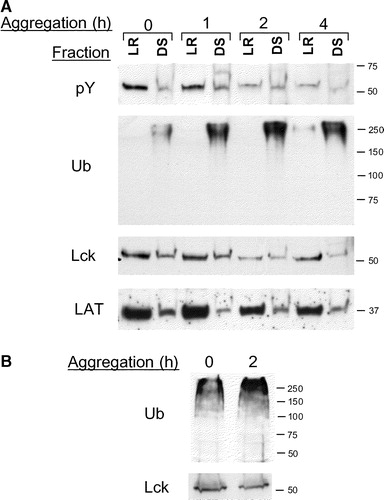
Reduced kinetics of tyrosine phosphorylation and protein ubiquitination in CD45-deficient cells
Since CD45 can dephosphorylate the C-terminal tyrosine of Lck, the CD45-deficient Jurkat line, J45.01, was used to investigate the potential role of this phosphatase in the aggregation-induced dephosphorylation of Lck. When lipid raft membranes were probed with the anti-pY mAb, a gradual reduction of Lck phosphorylation over a period of 4 h was noted, which however was not as rapid as that seen in the parental Jurkat cells (A). In addition, the kinetics of Lck protein reduction was slower, albeit visible after 4 h of aggregation (A, middle panel). In Jurkat cells high cell density induces the appearance of two phosphoproteins in lipid rafts, which were also visible in aggregated J45.01 cells but only after over-exposure of the blot (arrowheads, B).
Figure 4. Lck dephosphorylation and ubiquitination in CD45-deficient T cells. (A) Cells were aggregated for the times shown and lipid rafts were analysed for tyrosine phsphorylated proteins (pY), Lck and LAT expression. (B) Lipid rafts from J45.01 cells aggregated for 0 or 2 h were blotted with the anti-pY mAb and the western blot was intentionally over-exposed to detect weakly phosphorylated proteins. The arrowheads to the right of the first panel show two phosphoproteins that appear after aggregation of the cells. (C) LR and DS fractions from aggregated cells were analysed for protein ubiquitination (Ub) and LAT expression. (D) Lck immunoprecipitates from cells treated as in (C) were blotted with anti-Ub and anti-Lck antibodies.
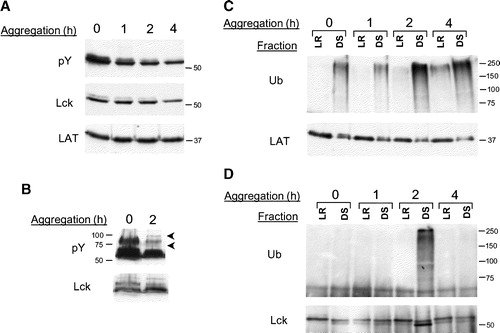
Probing the LR and DS fractions with anti-Ub antibodies showed that induced protein ubiquitination was taking place in CD45-deficient cells both in raft and non-raft fractions and was initially visible after 2 h of aggregation (C). Likewise, anti-Ub probing of Lck immunoprecipitations showed poly-ubiquitination of Lck in detergent-soluble lysates after 2 h of aggregation, however, as with the parental Jurkat cells, we were unable to detect any ubiquitination of Lck in lipid rafts (see D).
Cell density-induced changes are not due to extensive apoptosis
To see if there is a link between apoptosis and the changes observed in aggregated cells, we assayed for early (annexin V binding) and late (DNA fragmentation) apoptotic markers in aggregated cells. For comparison, we included cells treated with hydrogen peroxide (H2O2), which is shown to activate Lck Citation[26] or with PHA overnight, a treatment known to induce massive apoptosis of Jurkat cells. Approximately 4% of Jurkat cells collected at the logarithmic phase of growth stained positive with annexin V (see A). This percentage did not change significantly after 1 h of aggregation, although at this time point Lck dephosphorylation was visible in cell lysates (see C). Binding of annexin V increased gradually in cells aggregated for 2 and 4 h (approximately 8% and 14% respectively), however, there was never extensive apoptosis in aggregated cells in contrast to PHA treatment where 73% of the cells stained positive (A). Furthermore, no DNA laddering was seen in aggregated cells, although this was clearly visible in PHA-treated cells (B). Dephosphorylation of Lck was visible within 1 h of cell aggregation, and was stronger at later time points (C, top panel). H2O2 treatment did not have any appreciable effect, while PHA induced strong increase of the slower migrating form of Lck (C, top panel). As before, aggregation induced protein ubiquitination as did H2O2 and PHA treatment (C, second panel). After 4 h of aggregation there was significant reduction of Lck expression, whilst cells treated with PHA produced a diffused Lck band probably owing to its extensive phosphorylation on serine and threonine residues (C, third panel). Collectively, these results suggest that cell density-induced dephosphorylation and possibly protein ubiquitination, are not due to extensive apoptosis.
Figure 5. Aggregation-induced Lck dephosphorylation and ubiquitination is not linked to cell apoptosis. Jurkat T cells were aggregated for 0–4 h or treated with H2O2 for 4 h or PHA overnight. (A) Percentage of cells stained with annexin V. Actual numbers are shown in parenthesis above the bars. (B) Genomic DNA extracted from the cell cultures was analysed for fragmentation. (C) Cell lysates from the above treated cultures were sequentially blotted with antibodies to pY, Ub, Lck and LAT.
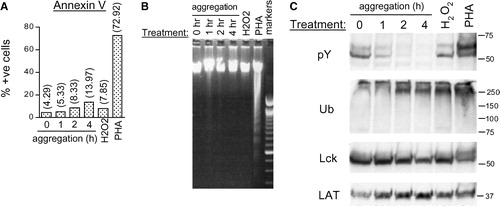
Changes in TCR signalling in aggregated T cells
To assess possible functional consequences of aggregation on TCR signalling, Jurkat cells were aggregated as before and then stimulated with a combination of anti-CD3 and anti-CD28 antibodies. One hour of aggregation did not change the pattern and intensity of the TCR-inducible phosphoproteins, however, after 2 and 4 h, there was a clear reduction in the intensity of these bands, which was more pronounced in cells aggregated for 4 h (A, top panel). TCR/CD28 stimulation resulted in the appearance of slower migrating Lck bands as reported previously Citation[27], which were reduced in intensity in cells aggregated for 2 h and were undetectable in cells aggregated for 4 h (see A, second panel). Moreover, loss of Lck protein expression was noticeable at 2 and 4 h, whilst Y505 dephosphorylation was detectable within 1 h of cell aggregation, as described earlier (A). There was no noticeable reduction in expression of Csk and Fyn proteins in aggregated cells. (A).
Figure 6. Reduced TCR signalling in aggregated cells. (A) Jurkat T cells were aggregated for the times shown and then left unstimulated or were stimulated with a combination of anti-CD3 and anti-CD28 antibodies. Whole cell lysates were sequentially probed with antibodies to pY, Lck, phospho-Y505, Csk and Fyn. (B) Cells were treated as in (A) and lysates were analysed for activation-specific phosphorylation of p38 and ERK1/2 proteins. Equivalent protein loading was determined by anti-Fyn blotting of the membrane. ns; non-specific.
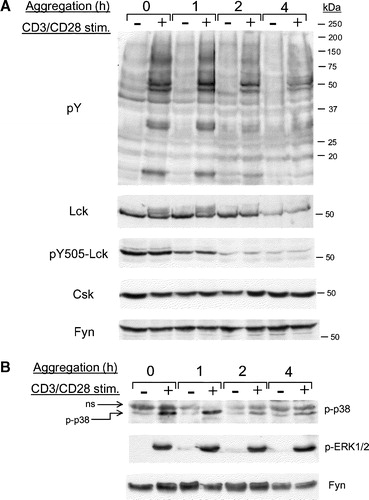
TCR stimulation activates the MAP kinase pathways ERK1/2 and p38. To investigate how these pathways are modulated by aggregation, cell lysates were probed with antibodies specific to the phosphorylated/activated forms of ERK1/2 and p38. Parallel with the reduced ability of aggregated cells to fully induce protein tyrosine phosphorylation after TCR stimulation, activation of the p38 pathway was strongly reduced, although not completely inhibited, in cells aggregated for 2 and 4 h (see B). Surprisingly, activation of the ERK1/2 was unaffected and even after 4 h of aggregation cells were fully capable of inducing this MAPK cascade (see B). This may suggest that residual tyrosine phosphorylation in cells aggregated for 2 and 4 h is sufficient to induce full ERK activation or that other TCR-regulated signalling events trigger ERK activation.
Discussion
Several cell types respond poorly to stimulation by growth factors when they reach confluency. Here we show that high cell density also regulates aspects of T cell function both in a T cell line and in primary T cells. A critical signalling molecule found to be modified by cell aggregation was the tyrosine kinase Lck, which is localized to both detergent-insoluble lipid raft and detergent-soluble membrane. Lck associated with the raft fraction is predominantly phosphorylated at the C-terminal tyrosine (Y505) assuming a ‘closed’ structure Citation[15–17] (see B). In contrast, the Lck pool in the detergent-soluble lysate seems to be primarily in the Y505-dephosphorylated, ‘open’ configuration Citation[15], Citation[17]. Within 1 h of aggregation, Y505 was extensively dephosphorylated in lipid rafts, while phosphorylation of two other proteins was increased (see A). The identity of these inducible phospho-proteins is unknown at present. Aggregation of CD45-deficient cells resulted in Lck dephosphorylation in lipid rafts, although with slower kinetics, suggesting that CD45 plays a partial role in density-induced dephosphorylation of Y505. It is also possible that the slower kinetics of Lck dephosphorylation could be due to the small amounts of CD45 expressed in J45.01 cells (about 10% of the levels expressed in parental Jurkat cells, T. Magee, personal communication). However, additional tyrosine phosphatases could be involved in this process. A possible candidate could be RPTPα, which like CD45, can dephosphorylate the C-terminal regulatory tyrosine of Src-family kinases, as demonstrated for Src and Fyn Citation[28].
Fyn is also localized to lipid rafts and could be susceptible to a similar kind of regulation as Lck. However, in our previous studies we found that this is not the case and that raft-associated Fyn, in contrast to Lck, had substantial enzymatic activity approximately equal to the activity of Fyn residing in non-raft membrane Citation[16], Citation[17]. Although the reason for this differential regulation of these two structurally similar kinases is unclear, this study did not address in detail whether high cell density modulates Fyn function. The experiment in , however, suggests that Fyn is not degraded as readily as Lck in aggregated T cells.
Following aggregation of T cells for two or more hours, a gradual loss of Lck protein from both the lipid raft and the non-raft fractions was noticed ( and ). This could be due to inducible ubiquitination and degradation of Lck levels in densely cultured cells. Although aggregation induced global protein ubiquitination in both fractions, specific Lck ubiquitination was detected only in the detergent-soluble fraction (). Therefore, loss of Lck protein from lipid rafts may not be due to its in situ ubiquitination and degradation, but as a result of its translocation to non-raft membrane, possibly after dephosphorylation of Y505 and mitigation of certain structural constrains imposed on the molecule by the ‘closed’ structure. For example, when Lck assumes its ‘open’ form, domains of the protein become available to interact with other protein partners, as has been demonstrated for the SH3 domain which interacts with c-Cbl, an interaction that negatively regulates Lck partition into lipid rafts Citation[21]. If this is the case, then Lck is a target for ubiquitination only when it is in the ‘open’ configuration. Such interpretation will corroborate published work from other labs showing that the activated form of Src family kinases is preferentially targeted for ubiquitination Citation[20], Citation[29], Citation[30]. We cannot exclude the possibility that some Lck ubiquitination does take place in lipid rafts but was not detected with the methodology employed here. Finally, it is possible that in addition to proteasome, Lck degradation in aggregating T cells involves endocytosis and lysosomal targeting.
A major question that needs to be addressed in follow-up work is the characterization at the molecular level of the stimulus that is generated when T cells are densely packed. It is reasonable to assume that under the conditions described in this report as aggregation, there is substantial and possibly sustained homotypic cell contact enabling activation of surface receptors and signalling. Such receptors could be adhesion molecules and a report has implicated Lck in signalling via β2 integrins in T cells Citation[31]. However, we cannot exclude the possibility that it is not homotypic cell contact that triggers Lck ubiquitination, but rather changes in the immediate cell environment arising from our experimental conditions, such as nutrient depletion or other stress signals. Although the experiment shown in D suggests that it is cell proximity rather than changes in the composition of the culture medium. Nevertheless, the present report describes for the first time a new mechanism for the regulation of Lck in T cells, which justifies further study.
Changes in the structure and expression of Lck may have profound effects in T cell function. It is shown here that cell aggregation for two or more hours compromises the strength of proximal TCR signalling as measured by inducible tyrosine phosphorylation. Activation of the downstream p38 MAPK cascade is also inhibited, surprisingly however, the ERK MAPK pathway is not affected. This may indicate that either residual protein tyrosine phosphorylation occurring in aggregated cells is sufficient to induce full activation of ERK-1/2, or that activation of this pathway is uncoupled from early tyrosine phosphorylation that takes place proximal to the TCR. Therefore, high cell density could determine not only the strength but also the end composition of the TCR signal, and as such may control the quality of T cell responses. Changes in the TCR-generated signal could lead to anergy instead of activation. It was demonstrated recently that Ca2 + -induced anergy is a multistep process which is mediated, at least in part, by the proteolytic degradation of critical signalling proteins such as protein kinase C theta (PKC-θ) and phospholipase C gamma 1 (PLC-γ1) Citation[32]. Interestingly, degradation of PLC-γ1 and PKC-θ was more pronounced after stimulating Ca2 + -treated cells with anti-CD3 antibodies or by simply culturing the cells at high cell density for 1–2 h Citation[32].
In conclusion, it is presented here for the first time that high cell density plays a critical role in T cell function. Culturing cells in vitro at high density induced profound alterations in the structure and expression of Lck, which were accompanied by changes in TCR signalling. In vivo, T cells may reside in close contact, resembling condition of in vitro aggregation, in tissues such as the thymus and lymph nodes. In this case, signals that are generated by closely-packed cells would have a regulatory role during thymocyte development possibly by fine-tuning the processes of positive and negative selection in the thymus, but also they may determine the magnitude of an immune response in the periphery. Future studies that expand on this initial observation will help us understand how homotypic cell contact regulates T cell functions by unravelling the molecular mechanisms that are involved in this process. This paper was first published online on prEview on 19 July 2005.
This work was supported by the Joint Research Board of the Special Trustees of St Bartholomew's Hospital, a Wellcome Trust Career Development Award, and the Arthritis Research Campaign (UK). We also thank Fabian Flores-Borja for help with the purification of human T cells and confocal microscopy.
References
- Straus DB, Weiss A. Genetic evidence for the involvement of the lck tyrosine kinase in signal transduction through the T cell antigen receptor. Cell 1992; 70: 585–593
- Latour S, Veillette A. Proximal protein tyrosine kinases in immunoreceptor signalling. Curr Opin Immunol 2001; 13: 299–306
- Samelson LA. Signal transduction mediated by the T cell antigen receptor: The role of adapter proteins. Ann Rev Immunol 2002; 20: 371–394
- Cantrell DA. GTPases and T cell activation. Immunol Rev 2003; 192: 122–130
- Shenoy-Scaria AM, Gauen LKT, Kwong J, Shaw AS, Lublin DM. Palmitylation of an amino-terminal cysteine motif of protein tyrosine kinases p56lck and p59fyn mediates interaction with glycosyl-phosphatidylinositol-anchored proteins. Mol Cell Biol 1993; 13: 6385–6392
- Koegl M, Zlatkine P, Ley SC, Courtneidge SA, Magee AI. Palmitoylation of multiple Src-family kinases at a homologous N-terminal motif. Biochem J 1994; 303: 749–753
- Kabouridis PS, Magee AI, Ley SC. S-acylation of LCK protein tyrosine kinase is essential for its signalling function in T lymphocytes. EMBO J 1997; 16: 4983–4998
- Simons K, Ikonen E. Functional rafts in cell membranes. Nature 1997; 387: 569–572
- Janes PW, Ley SC, Magee AI, Kabouridis PS. The role of lipid rafts in T cell antigen receptor (TCR) signalling. Sem Immunol 2000; 12: 23–34
- Xavier R, Seed B. Membrane compartmentation and the response to antigen. Curr Opin Immunol 1999; 11: 265–269
- Langlet C, Bernard AM, Drevot P, He HT. Membrane rafts and signalling by the multichain immune recognition receptors. Curr Opin Immunol 2000; 12: 250–253
- Thomas SM, Brugge JS. Cellular functions regulated by Src family kinases. Ann Rev Cell Dev Biol 1997; 13: 513–609
- Xu W, Doshi A, Lei M, Eck MJ, Harrison SC. Crystal structures of c-Src reveal features of its autoinhibitory mechanism. Molec Cell 1999; 3: 629–638
- Yamaguchi H, Hendrickson WA. Structural basis for activation of human lymphocyte kinase Lck upon tyrosine phosphorylation. Nature 1996; 384: 484–489
- Rodgers W, Rose JK. Exclusion of CD45 inhibits activity of p56lck associated with glycolipid-enriched membrane domains. J Cell Biol 1996; 135: 1515–1523
- Kabouridis PS, Janzen J, Magee AL, Ley SC. Cholesterol depletion disrupts lipid rafts and modulates the activity of multiple signalling pathways in T lymphocytes. Eur J Immunol 2000; 30: 954–963
- Kabouridis PS. Selective interaction of LAT (linker of activated T cells) with the open-active form of Lck in lipid rafts reveals a new mechanism for the regulation of Lck in T cells. Biochem J 2003; 371: 907–915
- Brdicka T, Pavlistova D, Leo A, Bruyns E, Korinek V, Angelisova P, Scherer J, Shevchenko A, Hilgert I, Cerny J, Drbal K, Kuramitsu Y, Kornacker B, Horejsi V, Schraven B. Phosphoprotein associated with glycosphingolipid-enriched microdomains (PAG), a novel ubiquitously expressed transmembrane adaptor protein, binds the protein tyrosine kinase csk and is involved in regulation of T cell activation. J Exp Med 2000; 191: 1591–1604
- Kawabuchi M, Satomi Y, Takao T, Shimonishi Y, Nada S, Nagai K, Tarakhovsky A, Okada M. Transmembrane phosphoprotein Cbp regulates the activities of Src-family tyrosine kinases. Nature 2000; 404: 999–1003
- Rao N, Miyake S, Reddi AL, Douillard P, Ghosh AK, Dodge IL, Zhou P, Fernandes ND, Band H. Negative regulation of Lck by Cbl ubiquitin ligase. Proc Natl Acad Sci USA 2002; 99: 3794–3799
- Hawash IY, Kesavan KP, Magee AI, Geahlen RL, Harrison ML. The Lck SH3 domain negatively regulates localization to lipid rafts through an interaction with c-Cbl. J Biol Chem 2002; 277: 5683–5691
- Vinals F, Pouyssegur J. Confluence of vascular endothelial cells induces cell cycle exit by inhibiting p42/p44 mitogen-activated protein kinase activity. Mol Cell Biol 1999; 19: 2763–2772
- Batt DB, Roberts TM. Cell density modulates protein-tyrosine phosphorylation. J Biol Chem 1998; 273: 3408–3414
- Lampugnani MG, Corada M, Andriopoulou P, Esser S, Risau W, Dejana E. Cell confluence regulates tyrosine phosphorylation of adherens junction components in endothelial cells. J Cell Sci 1997; 110: 2065–2077
- Grazia Lampugnani M, Zanetti A, Corada M, Takahashi T, Balconi G, Breviario F, Orsenigo F, Cattelino A, Kemler R, Daniel TO, Dejana E. Contact inhibition of VEGF-induced proliferation requires vascular endothelial cadherin, beta-catenin, and the phosphatase DEP-1/CD148. J Cell Biol 2003; 161: 793–804
- Hardwick JS, Sefton BM. Activation of the Lck tyrosine protein kinase by hydrogen peroxide requires the phosphorylation of Tyr-394. Proc Natl Acad Sci USA 1995; 92: 4527–4531
- Watts JD, Sanghera JS, Pelech SL, Aebersold R. Phosphorylation of serine 59 of p56lck in activated T cells. J Biol Chem 1993; 268: 23275–23282
- Ponniah S, Wang DZ, Lim KL, Pallen CJ. Targeted disruption of the tyrosine phosphatase PTPalpha leads to constitutive downregulation of the kinases Src and Fyn. Curr Biol 1999; 9: 535–538
- Hakak Y, Martin GS. Ubiquitin-dependent degradation of active Src. Curr Biol 1999; 9: 1039–1042
- Harris KF, Shoji I, Cooper EM, Kumar S, Oda H, Howley PM. Ubiquitin-mediated degradation of active Src tyrosine kinase. Proc Natl Acad Sci USA 1999; 96: 13738–13743
- Fagerholm S, Hilden TJ, Gahmberg CG. Lck tyrosine kinase is important for activation of the CD11a/CD18-integrins in human T lymphocytes. Eur J Immunol 2002; 32: 1670–1678
- Heissmeyer V, Macian F, Im SH, Varma R, Feske S, Venuprasad K, Gu H, Liu YC, Dustin ML, Rao A. Calcineurin imposes T cell unresponsiveness through targeted proteolysis of signalling proteins. Nat Immunol 2004; 5: 255–265