Abstract
The human immunodeficiency virus type 1 (HIV-1) is an enveloped virus with a lipid bilayer that contains several glycoproteins that are anchored in, or closely associated with, the membrane surface. The envelope proteins have complex interactions with the lipids both on the host cells and on the target cells. The processes of budding from host cells and entry into target cells occur at sites on the plasma membrane, known as lipid rafts, that represent specialized regions that are rich in cholesterol and sphingolipids. Although the envelope glycoproteins are antigenic molecules that potentially might be used for development of broadly neutralizing antibodies in a vaccine to HIV-1, the development of such antibodies that have broad specificities against primary field isolates of virus has been largely thwarted to date by the ability of the envelope proteins to evade the immune system through various mechanisms. In this review, the interactions of HIV-1 with membrane lipids are summarized. Liposomes are commonly used as models for understanding interactions of proteins with membrane lipids; and liposomes have also been used both as carriers for vaccines, and as antigens for induction of antibodies to liposomal lipids. The possibility is proposed that liposomal lipids, or liposome-protein combinations, could be useful as antigens for inducing broadly neutralizing antibodies to HIV-1.
Acronyms | ||
CL | = | cardiolipin |
CTH | = | ceramide trihexoside (also known as globotriosyl ceramide, or Gb3) |
GalCer | = | galactosyl ceramide (also known as galactocerebroside) |
GM1 | = | ganglioside GM1 |
GM3 | = | ganglioside GM3 |
LacCer | = | lactosylceramide (also known as ceramide dihexoside, or CDH), MPR: the external membrane proximal region of the HIV-1 envelope protein gp41 |
PC | = | phosphatidylcholine |
PE | = | phosphatidylethanolamine |
PE | = | phosphatidylgycerol |
PI | = | phosphatidylinositol |
PIP | = | phosphatidylinositol-4-phosphate |
PIP2 | = | phosphatidylinositol-4,5-bis-phosphate |
PS | = | phosphatidylserine |
SgalCer | = | galactosyl ceramide-3-sulfate (also known as sulfatide) |
SM | = | sphingomyelin |
Introduction
One of the major barriers that has emerged in the development of an effective HIV-1 vaccine is the difficulty in obtaining neutralizing antibodies that block infection by field isolates derived from a wide cross-section of clades (subtypes) (Moore et al. [Citation1995], McMichael & Hanke [Citation2003]). In order to obtain broadly neutralizing or protective antibodies to HIV-1 it will be necessary for antibodies to utilize antigenic epitopes (i.e., molecular recognition sites for binding of antibodies) that are conserved in the virus or that are present in the host or target cell in the regions in which the virus either buds or where binding or fusion with the virus occurs (McMichael & Hanke [Citation2003], Burton et al. [Citation2004]).
Most mammalian cells have a relatively conserved repertoire of lipids in the lipid bilayer of the plasma membrane, including glyceryl phospholipids, sphingosyl phospholipids (mainly sphingomyelin), lysophospholipids, glycosphingolipids, and cholesterol. In order to understand the role of lipids in HIV-pathogenesis, it is important to understand the interactions of HIV-1 with host cells and target cells. As discussed below, it is believed that patterns of plasma membrane lipids, known as lipid rafts, might serve as binding sites not only for viral interactions with host and target cells, but also as lipids that might be incorporated into HIV-1 to comprise the lipid bilayer of the virus envelope. Certain types of liposomes might be viewed as synthetic models of stabilized lipid raft-like structures and immunological studies with liposomes may provide insights about interactions of HIV-1 with lipid bilayers. The possibility exists that HIV-1 lipid structures, or liposomes, might be useful either as antigens, or as auxiliary lipids in combination with proteins and peptides, that could be exploited for immunological approaches to HIV-1.
This review has three goals; (i) To review current knowledge of HIV-1 lipid-protein interactions, particularly with respect to lipid rafts; (ii) To review the field of antibodies to liposomal phospholipids and cholesterol; and (iii) To explore the idea that the lipid bilayer might be an important factor in HIV-1 immunology, and to introduce the concept that antibodies to liposomal phospholipids and cholesterol might play a role in the development of neutralizing antibodies to HIV-1.
Lipid rafts
In contrast to the classical fluid mosaic hypothesis of homogeneous membrane lipid bilayer structure promulgated by Singer and Nicolson ([Citation1972]), the plasma membrane is now thought to comprise a heterogeneous array of laterally distributed islands of self-associated lipids, known as lipid rafts, caveolae, and other structures (Simons & Ikonen [Citation1997], Pike [Citation2003], Simons & Vaz [Citation2004]). Lipid rafts are specialized organized subdomains of the plasma membrane that have high concentrations of cholesterol and high concentrations of sphingolipids, including SM and glycosphingolipids, highly saturated phospholipids, and proteins. Under certain circumstances other types of phospholipids, such as PS, PE, and inositol phosphatides (especially PIP2), have been reported to be preferentially associated with lipid rafts, particularly on the inner lamella of the lipid bilayer. Certain glycosylphosphatidylinositol-anchored proteins are also raft associated (Simons & Ikonen [Citation1997]).
Because of differential packing characteristics of glyceryl phospholipids compared to sphingolipids and cholesterol, the glyceryl phospholipids of bilayer membranes have a tendency to form liquid disordered domains, while the sphingolipids and cholesterol tend to self-associate and phase separate as laterally distributed highly ordered tightly packed semi-solid lipid islands in the liquid disordered domains (Rietveld & Simons [Citation1998]). Although the ordered and disordered domains are thermodynamically distinct regions (Almeida et al. [Citation2005]), lipid rafts are highly dynamic transient structures that can be differentiated into different types that have characteristic degrees of stability (Subczynski & Kusumi [Citation2003]).
Sindbis virus is one example of an enveloped virus that binds to lipid rafts (Bollinger et al. [Citation2005]). Previous research using liposomal model membranes containing PC, PE, SM, and cholesterol has revealed that interactions of Sindbis virus with liposomes can emulate some, but not necessarily all, functional interactions of the virus with cells (Mooney et al. [Citation1975]). Liposomes containing such lipids might therefore reasonably be considered to represent a model of stable lipid rafts.
The concept of lipid rafts has engendered enormous interest because of the insight that they represent the localized site of numerous important receptor, signaling, trafficking, and endocytosis and fusion mechanisms (Simons & Ikonen [Citation1997], Pike [Citation2003], Rajendran & Simons [Citation2005]). Particularly important, lipid rafts serve as the specific or nonspecific entry points for a wide range of bacterial, protozoan, and many types of viral pathogens, including HIV-1 (Mañes et al. [Citation2003]).
Human immunodeficiency virus type 1 (HIV-1)
HIV-1 is a spherical, enveloped RNA retrovirus, a lentivirus, that fuses with the plasma membrane of a host cell to insert its genomic RNA. The envelope of HIV-1 contains a lipid bilayer that is associated with two loosely bound glycoproteins, gp120 and gp41. These proteins are created during intracellular virus assembly when a precursor protein, gp160, is cleaved to form gp120 and gp41 (A). The gp41 is a trimeric transmembrane protein that is anchored in the lipid bilayer, and during viral maturation and budding the intraviral end of gp41 is bound to sites located on an N-terminal myristoylated matrix protein (p17) (Yu et al. [Citation1992], Freed & Martin [Citation1995], Hill et al. [Citation1996]). As with other enveloped viruses, the lipid composition of the virus is largely reflective of the composition of lipid rafts present in the plasma membrane of the host cell (Aloia et al. [Citation1988], [Citation1993]).
Figure 1. Interactions of HIV-1 envelope proteins with plasma membrane lipids during target cell binding (B) and fusion steps (C). See text for further details.
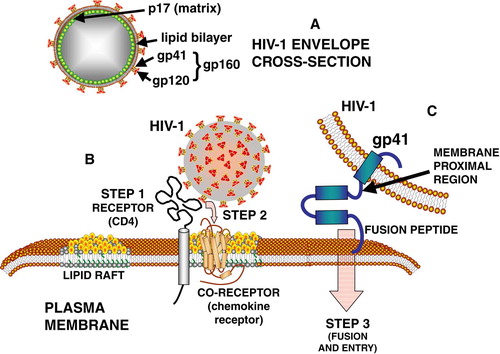
Cytopathology and apoptosis induced by HIV-1
Cells that become infected with HIV-1 undergo cytopathological effects leading to apoptosis. This may initially occur because arginine residues on the C terminal end of gp41 (fusion peptide) electrostatically bind to PS or PG or other charged phospholipids on the inner lamella of the plasma membrane of the target cell. The interactions of the C terminus of gp41 with PS or PG results in the formation of a lipidic pore, or causes other lipid membrane changes, leading to cellular permeability, cytopathology, apopotosis with movement of PS from the inner lamella of the lipid bilayer to the outer lamella, and death (Chernomordik et al. [Citation1994], Trommeshauser et al. [Citation2000]). After the subsequent acquisition of viral phospholipids from the host cell plasma membrane by the budding virus, the resultant viral lipid bilayer resembles that of apoptotic cells in that PS is present on the outer lamella of the budded viral bilayer (Callahan et al. [Citation2003]).
Intracellular viral maturation and budding: Roles of inositol phosphates and phosphatides
During the intracellular maturation phase of the virus in the host cell, an interaction of p17 with inositol pentaphosphate is important in the formation of the virion (Campbell et al. [Citation2001]). Other inositol phosphates or sulfates cannot substitute for inositol pentaphosphate; for example, inositol hexaphosphate, causes the generation of virus-like particles that have the wrong shape and size (Campbell et al. [Citation2001]). The intracellular trafficking patterns of the maturing virion appear to be dependent on the participation of PIP2 (Ono et al. [Citation2004]). Inositol phosphatides thus appear to serve an important role in the formation of the virion. It therefore seems likely that inositol phosphatides are incorporated into the mature virion, and are probably located at the interface of the p17 matrix with the inner lamella of the envelope bilayer.
Interactions of HIV-1 with lipid rafts and raft lipids
It is now widely accepted that HIV-1 buds out of (Nguyen & Hildreth [Citation2000], Ono & Freed [Citation2001]; Jolly & Sattentau [Citation2005], Brügger et al. [Citation2006]), and enters into (Mañes et al. [Citation2000], Nisole et al. [Citation2002]), the lipid raft areas of the plasma membrane (). HIV-1 also uses lipid raft-colocalized CD4 and chemokine receptors for productive entry into CD4+ cells (Popik et al. [Citation2002]). Lipid raft glycosphingolipids that have relevance to HIV-1 budding or entry include, among others, gangliosides GM1 and GM3, GalCer, SGalCer, LacCer, and CTH (see Supplementary , online only, for detailed structures).
Supplementary Figure 1. Structures of ceramide and selected glycosphingolipids that bind to and interact with HIV-1 envelope proteins, or with CD4 or chemokine receptors, during binding and fusion of HIV-1 with target cells. As shown, ceramide consists of a sphingosine that contains an amide-linked fatty acid.
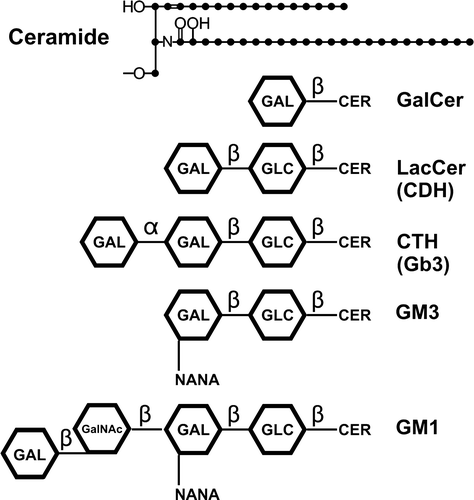
Fusion of HIV-1 with target cells
After budding from host cells, the HIV-1 virus exhibits a strong tendency to infect T lymphocytes as target cells, using CD4 as a receptor (Piguet & Sattentau [Citation2004]). The binding and fusion of HIV with the target cell involves a choreographed ballet between the proteins of the free virus and the entry site of the target cell (B, 1C). HIV entry into a cell is a multistep process initially involving the interactions of viral envelope protein gp120 and gp41 with several binding sites on the cell surface. The envelope proteins exist as a trimer consisting of 3 gp120 molecules and 3 gp41 molecules. The binding of gp120 to CD4 is followed by conformational changes in the gp120 protein that expose binding sites to chemokine receptors, CXCR4 or CCR5, that serve as co-receptor binding sites for interactions of the virus with the target cell (Berger et al. [Citation1999], Doms [Citation2000]; Huang et al. [Citation2005]). The binding of gp120 to the chemokine co-receptor in turn induces conformational changes that allow the binding of the gp41 anchor protein to the cell, and this is followed by fusion of the viral lipid bilayer with the plasma membrane bilayer, and entry of the virion RNA into the target cell (Colman & Lawrence [Citation2003]) (C). The binding and entry processes entail numerous types of interactions between proteins and lipids of the virus and specific lipids of the target cell (Fantini et al. [Citation2002]).
Gp41 contains 3 distinct domains, the ectodomain, transmembrane domain, and cytoplasmic domain (). Of these, only the ectodomain will be reviewed in this article. The ectodomain contains several distinct regions. The hydrophobic N-terminus contains the fusion peptide that initiates the binding of gp41 by inserting into hydrophobic region of the target cell lipid bilayer (Kliger et al. [Citation1997]). There are two helical-like regions called the leucine/isoleucine zipper region [N-helical heptad repeat region and the C-terminal heptad repeat region (C-helical region)], which are separated by a hairpin loop (McGaughey et al. [Citation2004]). After the C-helix is the membrane proximal region (MPR) which contains a large number of hydrophobic amino acids, namely DKWASLWNWFDITNWLWYIK.
Roles of glycosphingolipids in HIV-1 interactions with host cells
Several glycosphingolipids, including GalCer and SgalCer, have been proposed as alternate receptors or binding sites for HIV-1 (Bhat et al. [Citation1991], [Citation1993], Harouse et al. [Citation1991], Fantini et al. [Citation1993], [Citation1998], Alfsen & Bomsel [Citation2002]). Bhat et al. ([Citation1993]), using a truncated form of gp120, proposed that the binding site for GalCer/SGalCer is located between amino acids 206–275. In contrast, a binding site of HIV-1 to GalCer has also been located to residues 650–685 of gp41, and more specifically to residues 662–667 (comprising ELDKWA) on gp41 which is proposed to serve as a lectin for galactose-specific binding of HIV-1 to epithelial cells (Alfsen & Bomsel [Citation2002]). GM3 and CTH have also been indicated as binding sites for HIV-1 envelope (Hammache et al. [Citation1998a],Citation[b], [Citation1999], Puri et al. [Citation1998], Hug et al. [Citation2000]). Interestingly, the V3 loop on gp120 has been reported to have a binding site for GalCer (Fantini et al. [Citation1997]), and gp120 also binds GM3 and CTH (Hammache et al. [Citation1998a],Citation[b], [Citation1999]). This therefore indicates that gp120 and gp41 might both bind to GalCer, SGalCer, GM3, and CTH. It is conceivable that this could represent a sequential interaction of gp120 with the lipid bilayer, followed by interaction of gp41 with the lipid bilayer during a fusion process. This is suggested by the binding of GM3 to both the V3 loop and to CD4 (Hammache et al. [Citation1998a]). The GM3 binding to V3 involves the sequence GPGRAF, while GM3 binds CD4 through a different sequence (Hammache et al. [Citation1998a]). Thus, it has been proposed that GM3 facilitates the interaction of the V3 loop with CD4 (Hammache et al. [Citation1998a]). It should also be noted that the precise gp120 binding site for every glycolipid has not necessarily been delineated, and it is possible that sites other than GPGRAF might be involved in the binding of certain glycolipid headgroups.
The purported galactose specificity of HIV-1 for binding to the above glycosphingolipids is somewhat surprising to a glycolipid immunologist for two reasons. First, although GalCer, CTH, and GM1 all have a terminal galactose, the anomeric conformations of the terminal galactoses of both GM1 and GalCer are beta galactose, and the CTH has a terminal alpha galactose. From an immunogenic standpoint, beta-linked and alpha-linked galactose are immunologically distinguishable in that specific antibodies to GalCer cross-react with LacCer but not with CTH, and antibodies to CTH do not cross-react with GalCer or LacCer (Alving & Richards [Citation1977]). One might expect that anomeric binding distinctions could similarly be important for a galactose-specific binding site on HIV-1 envelope proteins. The second reason this is surprising to a glycolipid immunologist is that SGalCer has a terminal sulfate group and GM3 has a terminal sialic acid attached to galactose and neither molecule has a terminal galactose. If the galactose of SGalCer or GM3 is being recognized by HIV-1 it is achieved despite the presence of a bulky highly charged moiety attached to the galactose that blocks the binding of anti-galactose antibodies. However, regardless of the presence or absence of an exact specificity of the antibodies for glycolipid headgroups, antibodies to ELDKWA do inhibit gp41 binding to epithelial GalCer (Alfsen et al. [Citation2001]). In addition, the antibodies also block HIV transcytosis across a tight epithelial barrier (Bomsel et al. [Citation1998]), and they neutralize infection by a large array of HIV-1 primary isolates both in vitro and in vivo (Baba et al. [Citation2000], Mascola et al. [Citation2000]).
The huge diversity of the headgroups of GalCer, SGalCer, CTH, GM1, and GM3, and the fact that the lipid moieties of all of these glycolipids are essentially identical (i.e., ceramide), would seem to point to the lipid portions of the glycolipids as being particularly important for interaction with HIV-1.
The critical role of the fatty acid composition of GM3 has been emphasized further by the binding of human CD4 to human GM3 but not to bovine GM3 (Hammache et al. [Citation1998a]). Human and bovine GM3 presumably differ in their fatty acid compositions, and it was proposed that different conformations of human and bovine GM3 oligosaccharide headgroups were caused by the different fatty acids of the two types of GM3 as they interacted with the lipid bilayer, resulting in different binding affinities to CD4.
Roles of cholesterol and sphingomyelin (SM) in HIV-1 envelope binding and fusion with target cells
Host membrane cholesterol is critical for HIV-1 co-receptor function of chemokine receptors and is required for fusion and infection of cells (Liao et al. [Citation2001], Guyader et al. [Citation2002]). The highly conserved MPR region of gp41 has considerable hydrophobicity, in keeping with its proximity to the hydrophobic lipid structures of the membrane, and in keeping with its role in viral spike fusion processes (C, 2). Li and Papadopoulos ([Citation1998]) identified a common cholesterol recognition amino acid consensus pattern, L/V-(X)(1-5)-Y-(X)(1-5)-R/K, which can be found in the membrane proximal region of gp41 distal to the adjacent 2F5 and 4E10 recognition sites. The domain of gp41 responsible for binding to cholesterol was then localized within the residues 678–683 (LWYIK) at the C-terminus of MPR, immediately adjacent to the membrane spanning domain (Vincent et al. [Citation2002]).
It has been demonstrated that the MPR region of gp41, in combination with cholesterol and SM, promotes the intervesicular mixing of lipids in liposomes. Cholesterol and SM also promoted self-aggregation of the peptide into an ordered structure, a prerequisite that would be required for fusion of gp41 with plasma membranes of target cells (Sáez-Cirión et al. [Citation2002]). All of this points to cholesterol as an essential element for interaction of the sequence immediately above the membrane spanning sequence of gp41 with target cells as being required for fusion of HIV-1. The role of SM in the process of interaction with cholesterol is likely due to facilitation of interaction with cholesterol binding by the lipid ceramide portion of SM. It is noteworthy that the lipid ceramide composition of SM is essentially identical to the lipid ceramide moiety of GalCer, SGalCer, CTH, GM1, and GM3, differing only in fatty acid chain lengths of the various lipid molecules.
Role of PE in HIV-1 envelope binding and fusion with target cells
PE is likely to be a vital element in the process of fusion of HIV-1 with the lipid raft. Many biological processes that involve fusion of membranes have a nonlamellar (i.e., non-lipid bilayer) stage that occurs during the process. A primary mechanism for overcoming the energy barrier during the membrane destabilization step leading to fusion of membranes is to utilize the natural tendency of PE to participate in the formation of inverted nonlamellar hexagonal phase II (HII) structures that leads to pore formation and membrane fusion (Seddon [Citation1990]). Increasing concentrations of membrane cholesterol, such as are found in lipid rafts, also have a tendency to promote an increased formation of nonlamellar HII phase transitions of PE (Seddon [Citation1990]). PE is the naturally occurring mammalian lipid that is most prone to induce the non-lamellar HII phase. It is thus perhaps not coincidental that PE comprises a major component (ca. 25%) of the lipid bilayer composition of both the HIV-1 organism and the target cells (Aloia et al. [Citation1993]). Non-lamellar structures involving gp41 are thought to occur during the fusion process involving peptides in the loop region (amino acids 579–613) comprising some of the conserved immunodominant region of the ectodomain and the C-terminal region of the N-terminal heptad repeats region of gp41 (Pascual et al. [Citation2005]).
The role of PE in the fusion reaction of gp41 is strongly suggested by the observation that the 16 N-terminal amino acids of gp41 induces the mixing of lipids in the lipid bilayer of liposomes in vitro, but fusion of the liposomes only occurs when the liposomes contain PE (Martin et al. [Citation1993]). Furthermore, in the absence of lipids, the peptide has a beta-sheet conformation, but this changes partly into an alpha-helix conformation after interactions with the liposomes. In addition, the helix shifts from being parallel to the lipid-water interface in the absence of PE to being parallel to the lipid fatty acid chains in the presence of PE (Martin et al. [Citation1993]). Computer modeling suggested that 3 or 4 of the alpha-helices could self-associate to form a transmembrane structure into the bilayer (Martin et al. [Citation1993]). All of this is consistent with a crucial role of PE in the induction of non-lamellar HII phase structures leading to fusion of the virus with the host cell.
Role of PS in uptake of HIV-1 by macrophages
As discussed earlier, infection of target cells with HIV-1 leads to apoptosis of the cell, with transfer of PS from the inner lamella to the outer lamella of the lipid raft and expression of PS on the outer lamella of the budded virus. It is interesting to note that the PS of the HIV-1 lipid bilayer is quantitatively elevated by 40% when compared with the plasma membrane of the infected host cell (Aloia et al. [Citation1993]). The PS on the outer lamella of the lipid bilayer of the budded virus may serve as a cofactor for infection of monocytic cells by binding to a PS receptor on the monocytic cells (Callahan et al. [Citation2003]). Blocking of PS by annexin, a protein that specifically binds PS, inhibits HIV-1 infection of primary macrophages, but does not inhibit CD4-mediated infection of T cells (Callahan et al. [Citation2003]).
Possible role of lipid raft and HIV-1 lipids as antigens for influencing HIV-1 infectivity
Based on the above complex interactions of HIV-1 with lipids, we conclude that it is likely that the lipid moieties of the sphingolipids in lipid rafts are important. However, the headgroups of the glycosphingolipids also participate, sometimes with great specificity, in the functional interactions of HIV-1 with plasma membranes. Based on this further, we speculate that it might be possible to interfere globally with the interactions of HIV-1 with plasma membrane rafts by using antibodies directed against any of the phospholipids, cholesterol, or glycosphingolipids of the lipid rafts, including antibodies to SM, PE, charged phospholipids such as PS, GalCer, SGalCer, CTH, GM1, or GM3, cholesterol, or any other lipids in the adjoining environment of these lipids.
Antibodies to liposomal lipids
As shown below, methods are available for producing specific antibodies to lipid bilayer lipids that might be used to probe the antigenic structures of HIV-1 envelope bilayer lipids. In previous research from our laboratory polyclonal and monoclonal antibodies to protein-free and glycolipid-free liposomes were obtained by immunizing rabbits or mice with synthetic liposomes containing phospholipids, cholesterol, and lipid A imbedded in the lipid bilayer (Schuster et al. [Citation1979], Banerji & Alving [Citation1981], Banerji et al. [Citation1982], Wassef et al. [Citation1984], reviewed by Alving [Citation1986]).
The antibodies developed in this laboratory were initially examined for their ability to cause complement-dependent damage to liposomes, resulting in breakdown of the permeability barrier and release of encapsulated glucose. Adsorption of serum of immunized rabbits with liposomes lacking lipid A, and also lacking individual phospholipids or cholesterol, demonstrated that maximum adsorption occurred with liposomes containing the original pattern of lipids (Schuster et al. [Citation1979]). Because of this, these types of antibodies were generally referred to generically as anti-liposome antibodies, rather than as antibodies having only a single lipid specificity. However, different individual phospholipid specificities could be identified in various rabbit antisera, including specificity to phosphatidylcholine that was differentiated from phosphatidylsulfocholine (Wassef et al. [Citation1990]), specificities to phosphatidic acid, and to PS (Banerji & Alving [Citation1990]).
To determine whether the above types of absorption patterns were due merely to a multiplicity of antibodies having different specificities, anti-liposome monoclonal antibodies (mAbs) were produced by selection of hybridomas for the ability to react with liposomes lacking lipid A (Banerji et al. [Citation1982]). The mAbs were found to be highly specific for the particular patterns of phospholipids and cholesterol that were present in the liposomes. In order to probe the antigen binding sites of the antibodies, small soluble inhibitors of antibody activities were also examined. A prominent subsite in the antigen binding site that recognized phosphate was easily identified by the inhibitory ability of phosphocholine, but not choline, and even sodium phosphate, and inositol hexaphosphate, but not inositol (reviewed by Alving [Citation1986]). An apparent subsite for serine was also found in rabbit antisera to PS (Banerji & Alving [Citation1990]).
Presumably because of the above phosphate-binding subsites in the antigen binding sites of the antibodies, considerable cross-reactivities of polyclonal antibodies and mAbs were observed with related phospholipids. However, mAbs to PIP were identified that could cross-react with PI but not with PIP2, or vice versa (Wassef et al. [Citation1984], Alving [Citation1986]). Thus, striking specificity to individual liposomal lipids, such as inositol phosphatides, could be obtained.
Mabs were even created that recognized liposomes containing 71 mol% cholesterol but that did not recognize 43 mol% cholesterol (Swartz et al. [Citation1988]). These latter antibodies also recognized highly purified crystalline cholesterol by ELISA (Swartz et al. [Citation1988]), and did not have a prominent phosphate-binding subsite (Stollar et al. [Citation1989]). A series of mAbs was also created to squalene, a precursor in synthesis of cholesterol (Matyas et al. [Citation2000]). This was quite remarkable in that squalene is an alkene hydrocarbon that lacks a polar group. Although considerable cross-reactivity did occur with squalane, the hydrogenated form of squalene, mAbs could be identified that did not cross-react with squalane.
Other laboratories have developed polyclonal antibodies and mAbs to lipids by various methods, including mAb specificities to PIP2 (Matuoka et al. [Citation1988]); liposomal PIP2 (Miyazawa et al. [Citation1988]); PI (Bate & Kwiatkowski [Citation1994]), and PS (Umeda et al. [Citation1989], Ran et al. [Citation2005]). The latter anti-PS antibody (called 3G4) (Ran et al. [Citation2005]) actually has a specificity that requires the presence of a phospholipid-binding protein, beta-2-glycoprotein I, and is therefore possibly an antibody to the protein rather than an antibody to the lipid.
Influence of glycosphingolipids on binding of antibodies to liposomal lipids: Implications for HIV binding and fusion with host cells
A commonplace and striking observation that was made about the above antibodies to liposomal phospholipids and cholesterol is that enormously increased binding activities were often observed when the test liposomes also contained glycosphingolipids, such as GalCer, CTH, or GM1 in the lipid bilayer (Banerji et al. [Citation1982]). Since these glycolipids had not been present in the immunizing liposomes, it was believed that the glycolipids altered the conformation of the phospholipids and cholesterol in the liposomes, causing these latter moieties to be better exposed to the binding of the specific antibodies. It is our hypothesis that this same phenomenon could be in play in the studies (see above) that suggest that GalCer and CTH are binding sites for the HIV-1. Based on the liposome studies, it is possible that the binding activities for HIV-1 are actually targeted to the high concentrations of cholesterol or to the phospholipids (such as PE) that are present in the lipid rafts, and that the lipid (ceramide) portions of GalCer and/or CTH simply enhance that binding by promoting better binding of HIV to the cholesterol or PE or other phospholipids.
Potential of using antibodies to lipids to neutralize HIV-1
The MPR region of gp41 (C, 2) contains the binding epitopes for two human IgG monoclonal antibodies that are known to be broadly neutralizing antibodies, known as 2F5 and 4E10 Zwick et al. [Citation2001], Burton et al. [Citation2004]. 2F5 binds to ELDKWA (the MPR starts at D) and 4E10 binds to NWFDIT (Parker et al. [Citation2001], Zwick et al. [Citation2001]). The 2F5 epitope, ELDKWA, is the same sequence identified as the above binding site for GalCer (Alfsen & Bomsel [Citation2002]). The cholesterol binding site LWYIK is at the end of the MPR (Vincent et al. [Citation2002]). The overall series of interactions of HIV-1 involving budding, binding and fusion with host and target cells exposes lipid-associated proteins (Wyatt & Sodroski [Citation1998]), and even lipids themselves (Haynes et al. [Citation2005], Sanchez-Martinez et al. [Citation2006]), as potential antigenic targets for virus neutralization.
The easy availability of numerous types of antibodies to lipids raises the possibility that direct binding of antibodies to certain types of lipids might be examined to try to neutralize HIV-1 during its interactions with lipid rafts of host or target cells. The antibodies might also directly kill the virus through complement activation, or virus-infected cells might be eliminated by antibody-dependent cellular cytoxicity (Silvestris et al. [Citation1996]). As noted earlier, because of apoptosis of the host cell, PS which is normally present on the inner lamella of the plasma membrane of the cell becomes visible on the outer lamella of the viral lipid bilayer. It would not be unreasonable to suspect that other phospholipids, such as PE and inositol phosphatides, that are normally present in the inner lamella of host cell plasma membranes might also be present on the outer lamella of the HIV-1 lipid bilayer after budding from the apoptotic host cell. Such a transition of inner lamella plasma membrane phospholipids to the outer lamella of the viral lipid bilayer might serve to make these phospholipids available for antibody binding and might differentiate the viral membrane from the target cell membrane.
The possible antigenic visibility of viral phospholipids was highlighted by the observation that two broadly neutralizing human mAbs, 2F5 and 4E10, both have binding specificities that include the binding to a variety of phospholipids (Haynes et al. [Citation2005], Sanchez-Martinez et al. [Citation2006]). For example, 4E10 apparently bound both to neutral phospholipids (PC, SM, and PE) and anionic phospholipids (CL and PS). Both of these antibodies therefore have specificities that recognize both amino acid sequences in gp41 and lipid compositions in the lipid bilayer. Attempts to produce broadly neutralizing antibodies solely against the amino acid sequences have not been highly successful (McGaughey et al. [Citation2004]) and it is possible that lipid recognition might be useful, or even required, for full neutralizing activity of this type of antibody. Our laboratory is currently engaged in a program to examine the potential that antibodies described above to liposomal phospholipids, cholesterol, and squalene might have direct neutralizing activities to HIV-1.
Antibodies to cholesterol
It might be possible to interfere with the binding or fusion of HIV-1 directly by utilizing antibodies to cholesterol. As noted earlier, cholesterol is highly immunogenic in mice when present in high concentrations in liposomes containing lipid A (Swartz et al. [Citation1988], Alving & Swartz [Citation1991]). Naturally-occurring antibodies to cholesterol, both IgM and IgG, are present in sera from essentially 100% of humans (Alving et al. [Citation1989], Alving & Wassef [Citation1999], Horvath et al. [Citation2001], Fust et al. [Citation2005]). Interestingly, the titers of antibodies to cholesterol were markedly higher in the sera of HIV infected patients when compared to HIV seronegative controls (Horvath et al. [Citation2001], Fust et al. [Citation2005]). Introduction of highly active antiretroviral therapy (HAART) resulted in a significant and gradual drop in the anti-cholesterol antibody titers in parallel with a decrease in viral load and increase in CD4+ cell counts (Horvath et al. [Citation2001]). However, it is not clear at present whether the increased titers of antibodies to cholesterol were an epiphenomenon associated with HIV-1 infection, or whether the antibodies actually had some beneficial effects against HIV-1.
Antibodies to PE
Another area of vulnerability of HIV-1 could involve PE. It is our hypothesis that antibodies to one or more of the lipids in the environment of the PE, including antibodies to PE itself, or to glycosphingolipids, or to cholesterol could interfere with the fusion process involving PE. For example, it has been reported that the HII phase is immunogenic in mice, and murine antibodies to HII are strongly reactive with both PE and with cardiolipin (CL) but do not react with lamellar phase lipids (Rauch & Janoff [Citation1990]). This may be significant in view of the recent report that broadly reacting neutralizing antibodies derived from B cells of HIV-1 infected humans (2F5 and 4E10), antibodies that react with conserved epitopes in gp41, also react with both CL and PE (Haynes et al. [Citation2005]). It should be noted that CL is normally present only on the inner membrane of mitochondria and has not been reported to be present on plasma membranes or HIV-1 (Aloia et al. [Citation1988], [Citation1993]). Furthermore, PE is normally present only on the inner lamella of plasma membranes, but in view of the transposition of PS to the outer leaflet of HIV-1, it is possible that PE might also be present on the outer surface of HIV-1
Antibodies to liposomal inositol phosphatides
Antibodies to PIP represent another interesting neutralizing antibody possibility that could be tested. PIP, an intermediate in the synthesis of PIP2 from PI (Downes et al. [Citation2005]), is synthesized by a PI-4-kinase that is located in the lipid rafts and caveolae-like vesicles of the plasma membrane of eukaryotic cells, and PIP2 is involved in membrane-associated intracellular signaling (Waugh et al. [Citation1998]). Although PIP is thought to be present in low concentrations and is located primarily in the cytoplasmic face of rafts in the plasma membrane, is not clear whether, like PS and PE, it might be present on the outer lamella of the viral lipid bilayer. As noted earlier, PIP2 is strongly associated with the P17 matrix protein of HIV-1 (Hill et al. [Citation1996]). P17 is known to interact with the intraviral and cytoplasmic C-terminal tail of gp41 (Freed & Martin [Citation1995], Bhattacharya et al. [Citation2006]), and one could speculate that PIP2 might become transiently available to antibody binding during the fusion process of gp41 with target cells.
Certain GPI-anchored proteins, such as Thy-1 and CD59, are incorporated into the HIV-1 virion during budding of the virus from lipid rafts (Nguyen & Hildreth [Citation2000]). GPI-anchored proteins were originally discovered on the surface of Trypanosoma brucei (Ferguson et al. [Citation1985]), and antibodies to PI and PIP were induced in rabbits infected with Trypanosoma rhodesiense (Richards et al. [Citation1983]). Thus, PI and PIP in an infectious organism can be immunogenic, and it appears possible that PI or PIP in the GPI-anchored proteins or in the lipid bilayer of the HIV-1 virion, or even in the host cell, could represent a theoretical target for neutralizing antibodies to HIV-1.
Naturally-occurring antibodies to lipids: binding of anti-lipid antibodies to lipid bilayers and normal cells
Naturally-occurring antibodies to many lipids, including phospholipids (Alving [Citation1984], Cabiedes et al. [Citation1998]), cholesterol (Alving et al. [Citation1989], Avila et al. [Citation1996], Alving & Wassef [Citation1999], Horvath et al. [Citation2001], Horvath & Biro [Citation2003]), and squalene (Matyas et al. [Citation2004]) are widespread in normal human blood. Antibodies to phospholipids are commonly transiently induced as an epiphenomenon during many infectious diseases (Vaarala et al. [Citation1986]), including HIV-1 (Silvestris et al. [Citation1996]), and in conjunction with certain drugs (Canoso & Sise [Citation1982]). If this is so, why do these antibodies not protect against HIV-1 or against other enveloped viruses having a lipid bilayer? Moreover, why are there no adverse effects on normal cells?
The answer to these questions is two-fold. First, lipids on normal cells are strongly shielded from binding of antibodies due to adjacent larger molecules, including large or charged glycolipids or overlying proteins (Alving & Wassef [Citation1999], Alving [Citation2006]). This was experimentally demonstrated by Fogler et al. ([Citation1987]) in a study in which two monoclonal antibodies to liposomal phospholipids (including a monoclonal antibody to PIP) did not react with freshly explanted, nonadherent macrophages, but did react with the cells after they became adherent to the tissue culture plastic surface. The binding of the antibodies was enhanced by treatment of the cells with trypsin, but was inhibited by treatment of the cells with phospholipase C or D (Fogler et al. [Citation1987]). Thus, it is quite possible, during the intricate interactions of HIV-1 envelope proteins during the budding or entry process, that lipid substances on the viral envelope, or on the cell itself, might become transiently available to antibody binding. Thus, the differential binding of antibodies to infected cells, but not to normal cells, could occur, and this might serve as a mechanism for targeting infected cells for elimination by anti-lipid antibodies.
Second, it is likely that naturally-occurring antibodies to lipids have normal functions in the circulation, and they probably mainly exist as immune complexes. For example, monoclonal and polyclonal antibodies to cholesterol bind to low density lipoproteins but, because of overlying apoprotein, they do not bind to high density lipoproteins (Dijkstra et al. [Citation1996]), and naturally-occurring antibodies to cholesterol have been proposed to modulate low density protein levels in the blood (Alving & Wassef [Citation1999]). Naturally-occurring antibodies to numerous phospholipids have also been shown to occur as circulating immune complexes in all normal human sera (Cabiedes et al. [Citation1998]). It has been proposed that such antibodies serve as scavengers for removal of debris from apoptotic cells or from inflammatory foci (reviewed by Alving [Citation2006]).
In view of the above, in order for antibodies to lipids to be effective against HIV-1 it might be necessary either to have a high titer of free circulating antibodies to lipids, or, as in the case of the neutralizing antibodies 4E10 and 2F5 (Haynes et al. [Citation2005], Sanchez-Martinez et al. [Citation2006]), to have antibodies that simultaneously recognize both the HIV-1 envelope and the lipids that are adjacent to the amino acid sequences in the HIV-1 envelope.
Safety of antibodies to lipids
It should be clear from the above discussion, that antibodies to lipids are not likely to be dangerous. Normal cells and tissues are protected from adverse effects of antibodies to lipids through microheterogeneity at the surface of cell membranes in which large membrane molecules prevent the binding of antibodies to small antigenic groups of lipids at surfaces of plasma membranes, and through the existence of circulating immune complexes that prevent binding of the complexed antibodies to cells. An illustration of the safety of antibodies to lipids is exhibited by the results of passive administration of high concentrations of cocktails of monoclonal antibodies that included 4E10 and 2F5, both of which bind phospholipids, in phase I trials in patients infected with HIV-1 (Armbruster et al. [Citation2002], [Citation2004], Trkola et al. [Citation2005]). A complete discussion of the relative safety of antibodies to lipids has also been given elsewhere (Alving [Citation2006]).
A dangerous autoimmune disease (so-called “anti-phospholipid” syndrome) has been described that is associated with recurring episodes of thrombosis (Miyakis et al. [Citation2006], Alving [Citation2006]). The name of this disease entity is probably a misnomer because the specificity of the antibodies is thought to be directed mainly or exclusively to phospholipid-binding proteins (beta-2-glycoprotein I, or thrombin) rather than to the lipids themselves (McNeil et al. [Citation1990], Roubey et al. [Citation1995]). Antibodies to CL, PS, or both, commonly occur in HIV-1-infected patients, but thrombophilic events are not associated with such antibodies (Silvestris et al. [Citation1996], Falco et al. [Citation1993]). An assay that distinguished antibodies that bound to CL alone from antibodies that required beta-2-glycoprotein I and phospholipid, demonstrated that the anti-CL antibodies from HIV-1 patients did not require beta-2-glycoprotein I, and beta-2-glycoprotein I actually competed with the anti-CL antibodies for binding to CL (Falco et al. [Citation1993]).
Antibodies that recognize beta-2-glycoprotein I, such as 3G4, a mouse IgG3 monoclonal antibody specific for PS-beta-2-glycoprotein (Beck et al. [Citation2006]), might have a potential for thrombotic effects. However, antibodies to liposomal lipids do not require beta-2-glycoprotein I for binding and would not be expected to exhibit thrombotic phenomena. Although the theoretical possibility does exist that high titers of antibodies to lipids that do not require beta-2-glycoprotein I might have detrimental effects, to date the preponderance of evidence suggests that the antibodies do not induce autoimmune pathological effects.
Disclaimer
The views and opinions expressed herein are the private opinions of the authors and do not necessarily reflect the views of the U.S. Army or the US Department of Defense.
References
- Alfsen A, Iniguez P, Bouguyon E, Bomsel M. Secretory IgA specific for a conserved epitope on gp41 envelope glycoprotein inhibits epithelial transcytosis of HIV-1. J Immunol 2001; 166: 6257–6265
- Alfsen A, Bomsel M. HIV-1 gp41 envelope residues 650–685 exposed on native virus act as a lectin to bind epithelial cell galactosyl ceramide. J Biol Chem 2002; 277: 25649–25659
- Almeida PF, Pokorny A, Hinderliter A. Thermodynamics of membrane domains. Biochim Biophys Acta 2005; 1720: 1–13
- Aloia RC, Jensen FC, Curtain CC, Mobley PW, Gordon LM. Lipid composition and fluidity of the human immunodeficiency virus. Proc Natl Acad Sci USA 1988; 85: 900–904
- Aloia RC, Tian H, Jensen FC. Lipid composition and fluidity of the human immunodeficiency virus envelope and host cell plasma membranes. Proc Natl Acad Sci USA 1993; 90: 5181–5185
- Alving CR. Natural antibodies against phospholipids and liposomes in humans. Biochem Soc Trans 1984; 12: 342–344
- Alving CR. Antibodies to liposomes, phospholipids and phosphate esters. Chem Phys Lipids 1986; 40: 303–314
- Alving CR. 2006. Antibodies to lipids and liposomes: immunology and safety. J Liposome Res 16:157–166.
- Alving CR, Richards RL. Immune reactivities of antibodies against glycolipids. II. Comparative properties, using liposomes, of purified antibodies against mono-, di- and trihexosyl ceramide haptens. Immunochemistry 1977; 14: 383–389
- Alving CR, Wassef NM. Naturally-occurring antibodies to cholesterol: a new theory of LDL cholesterol metabolism. Immunology Today 1999; 20: 362–366
- Alving CR, Swartz GM, Jr. Antibodies to cholesterol, cholesterol conjugates, and liposomes: Implications for atherosclerosis and autoimmunity. CRC Crit Rev Immunol 1991; 10: 441–453
- Alving CR, Swartz GM, Jr, Wassef NM. Naturally-occurring autoantibodies to cholesterol in humans. Biochem. Soc. Trans. 1989; 17: 637–639
- Armbruster C, Stiegler GM, Vcelar BA, Jager W, Michael NL, Vetter N, Katinger HW. A phase I trial with two human monoclonal antibodies (hMAb 2F5, 2G12) against HIV-1. AIDS 2002; 16: 227–233
- Armbruster C, Stiegler GM, Vcelar BA, Jager W, Koller U, Jilch R, Ammann CG, Pruenster M, Stoiber H, Katinger HW. Passive immunization with the anti-HIV-1 human monoclonal antibody (hMAb) 4E10 and the hMAb combination 4E10/2F5/2G12. J Antimicrob Chemother 2004; 54: 915–920
- Avila JL, Rojas M, Avila A. Cholesterol sulphate-reactive autoantibodies are specifically increased in chronic chagasic human patients. Clin Exp Immunol 1996; 103: 40–46
- Baba TW, Liska V, Hofmann-Lehmann R, Vlasak J, Xu W, Ayehunie S, Cavacini LA, Posner MR, Katinger H, Stiegler G, Bernacky BJ, Rizvi TA, Schmidt R, Hill LR, Keeling ME, Lu Y, Wright JE, Chou TC, Ruprecht RM. Human neutralizing monoclonal antibodies of the IgG1 subtype protect against mucosal simian-human immunodeficiency virus infection. Nat Med 2000; 6: 200–206
- Bhattacharya J, Repik A, Clapham PR. Gag regulates association of human immunodeficiency virus type 1 envelope with detergent-resistant membranes. J Virol 2006; 80: 5292–5300
- Banerji B, Alving CR. Anti-liposome antibodies induced by lipid A. I. Influences of ceramide, glycosphingolipids, and phosphocholine on complement damage. J Immunol 1981; 126: 1080–1084
- Banerji B, Lyon JA, Alving CR. Membrane lipid composition modulates the binding specificity of a monoclonal antibody against liposomes. Biochim Biophys Acta 1982; 689: 319–326
- Banerji B, Alving CR. Antibodies to liposomal phosphatidylserine and phosphatidic acid. Biochem Cell Biol 1990; 68: 96–101
- Bate CA, Kwiatkowski D. A monoclonal antibody that recognizes phosphatidylinositol inhibits induction of tumor necrosis factor alpha by different strains of Plasmodium falciparum. Infect Immun 1994; 62: 5261–5266
- Beck AW, Luster TA, Miller AF, Holloway SE, Conner CR, Barnett CC, Thorpe PE, Fleming JB, Brekken RA. Combination of a monoclonal anti-phosphatidylserine antibody with gemcitabine strongly inhibits the growth and metastasis of orthotopic pancreatic tumors in mice. Int J Cancer 2006; 118: 2639–2643
- Berger EA, Murphy PM, Farber JM. Chemokine receptors as HIV-1 coreceptors: roles in viral entry, tropism, and disease. Annu Rev Immunol 1999; 17: 657–700
- Bhat S, Spitalnik SL, Gonzalez-Scarano F, Silberberg DH. Galactosyl ceramide or a derivative is an essential component of the neural receptor for human immunodeficiency virus type 1 envelope glycoprotein gp120. Proc Natl Acad Sci USA 1991; 88: 7131–7134
- Bhat S, Mettus RV, Reddy EP, Ugen KE, Srikanthan V, Williams WV, Weiner DB. The galactosyl ceramide/sulfatide receptor binding region of HIV-1 gp120 maps to amino acids 206–275. AIDS Res Hum Retroviruses 1993; 9: 175–181
- Bollinger CR, Teichgraber V, Gulbins E. Ceramide-enriched membrane domains. Biochim Biophys Acta 2005; 1746: 284–294
- Bomsel M, Heyman M, Hocini H, Lagaye S, Belec L, Dupont C, Desgranges C. Intracellular neutralization of HIV transcytosis across tight epithelial barriers by anti-HIV envelope protein dIgA or IgM. Immunity 1998; 9: 277–287
- Brügger B, Glass B, Haberkant P, Leibrecht I, Wieland FT, Kräusslich H-G. The HIV lipodome: a raft with an unusual composition. Proc Natl Acad Sci USA 2006; 103: 2641–2646
- Burton DR, Desrosiers RC, Doms RW, Koff WC, Kwong PD, Moore JP, Nabel GJ, Sodroski J, Wilson IA, Wyatt RT. HIV vaccine design and the neutralizing antibody problem. Nat Immunol 2004; 5: 233–236
- Cabiedes J, Cabral AR, Alarcon-Segovia D. Hidden anti-phospholipid antibodies in normal human sera circulate as immune complexes whose antigen can be removed by heat, acid, hypermolar buffers or phospholipase treatments. Eur J Immunol 1998; 28: 2108–2114
- Callahan MK, Popernack PM, Tsutsui S, Truong L, Schlegel RA, Henderson AJ. Phosphatidylserine on HIV envelope is a cofactor for infection of monocytic cells. J Immunol 2003; 170: 4840–4845
- Campbell S, Fisher RJ, Towler EM, Fox S, Issaq HJ, Wolfe T, Phillips LF, Rein A. Modulation of HIV-like particle assembly in vitro by inositol phosphates. Proc Natl Acad Sci USA 2001; 98: 10875–10879
- Canoso RT, Sise HS. Chlorpromazine-induced lupus anticoagulant and associated immunological abnormalities. Am J Hematol 1982; 13: 121–129
- Chernomordik L, Chanturiya AN, Suss-Toby E, Nora E, Zimmerberg J. An amphipathic peptide from the C-terminal region of the human immunodeficiency virus envelope glycoprotein causes pore formation in membranes. J Virol 1994; 68: 7115–7123
- Colman PM, Lawrence MC. The structural biology of type I viral membrane fusion. Nat Rev Mol Cell Biol 2003; 4: 309–319
- Dijkstra J, Swartz GM, Jr, Raney JJ, Aniagolu J, Toro L, Nacy CA, Green SJ. Interaction of anti-cholesterol antibodies with human lipoproteins. J Immunol 1996; 157: 2006–2013
- Doms RW. Beyond receptor expression: the influence of receptor conformation, density, and affinity in HIV-1 infection. Virology 2000; 276: 229–237
- Downes CP, Gray A, Lucocq JM. Probing phosphoinositide functions in signaling and membrane trafficking. Trends Cell Biol 2005; 15: 259–268
- Falco M, Sorrenti A, Priori R, Luan FL, Pittoni V, Agresti MG, Valesini G. Anti-cardiolipin antibodies in HIV infection are true antiphospholipids not associated with antiphospholipid syndrome. Ann Ital Med Int 1993; 8: 171–174
- Fantini J, Cook DG, Nathanson N, Spitalnik SL, Gonzalez-Scarano F. Infection of colonic epithelial cell lines by type 1 human immunodeficiency virus is associated with cell surface expression of galactosylceramide, a potential alternative gp120 receptor. Proc Natl Acad Sci USA 1993; 90: 2700–2704
- Fantini J, Hammache D, Delezay O, Yahi N, Andre-Barres C, Rico-Lattes I, Lattes A. Synthetic soluble analogs of galactosylceramide (GalCer) bind to the V3 domain of HIV-1 gp120 and inhibit HIV-1-induced fusion and entry. J Biol Chem 1997; 272: 7245–7252
- Fantini J, Hammache D, Delezay O, Pieroni G, Tamalet C, Yahi N. Sulfatide inhibits HIV-1 entry into CD4 − /CXCR4+ cells. Virology 1998; 246: 211–220
- Fantini J, Garmy N, Mahfoud R, Yahi N. 2002. Lipid rafts: structure, function and role in HIV, Alzheimer's and prion diseases. Expert Rev Mol Med 20 December, http://www.expertreviews.org/02005392h.htm.
- Ferguson MA MG, Low G, Cross GA. Glycosyl-sn-1,2-dimyristylphosphatidylinositol is covalently linked to Trypanosoma brucei variant surface glycoprotein. J Biol Chem 1985; 260: 14547–14555
- Fogler WE, Swartz GM, Jr, Alving CR. Antibodies to phospholipids and liposomes: binding of antibodies to cells. Biochim Biophys Acta 1987; 903: 265–272
- Freed EO, Martin MA. Virion incorporation of envelope glycoproteins with long but not short cytoplasmic tails is blocked by specific single amino acid substitutions in the human immunodeficiency virus type 1 matrix. J Virol 1995; 69: 1984–1989
- Fust G, Beck Z, Banhegyi D, Kocsis J, Biro A, Prohaszka Z. Antibodies against heat shock proteins and cholesterol in HIV infection. Mol Immunol 2005; 42: 79–85
- Guyader M, Kiyokawa E, Abrami L, Turelli P, Trono D. Role of human immunodeficiency virus type 1 membrane cholesterol in viral internalization. J Virol 2002; 76: 10356–10264
- Hammache D, Yahi N, Pieroni G, Ariasi F, Tamalet C, Fantini J. Sequential interaction of CD4 and HIV-1 gp120 with a reconstituted membrane patch of ganglioside GM3: implications for the role of glycolipids as potential HIV-1 fusion cofactors. Biochem Biophys Res Commun 1998a; 246: 117–122
- Hammache D, Pieroni G, Yahi N, Delezay O, Koch N, Lafont H, Tamalet C, Fantini J. Specific interaction of HIV-1 and HIV-2 surface envelope glycoproteins with monolayers of galactosylceramide and ganglioside GM3. J Biol Chem 1998b; 273: 7967–7971
- Hammache D, Yahi N, Maresca M, Pieroni G, Fantini J. Human erythrocyte glycosphingolipids as alternative cofactors for human immunodeficiency virus type 1 (HIV-1) entry: evidence for CD4-induced interactions between HIV-1 gp120 and reconstituted membrane microdomains of glycosphingolipids (Gb3 and GM3). J Virol 1999; 73: 5244–5248
- Harouse JM, Bhat S, Spitalnik SL, Laughlin M, Stefano K, Silberberg DH, Gonzalez-Scarano F. Inhibition of entry of HIV-1 in neural cell lines by antibodies against galactosyl ceramide. Science 1991; 253: 320–323
- Haynes BF, Fleming J, St Clair EW, Katinger H, Stiegler G, Kunert R, Robinson J, Scearce RM, Plonk K, Staats HF, Ortel TL, Liao HX, Alam SM. Cardiolipin polyspecific autoreactivity in two broadly neutralizing HIV-1 antibodies. Science 2005; 308: 1906–1908
- Hill CP, Worthylake D, Bancroft DP, Christensen AM, Sundquist WI. Crystal structures of the trimeric human immunodeficiency virus type 1 matrix protein: implications for membrane association and assembly. Proc Natl Acad Sci USA 1996; 93: 3099–3104
- Horvath A, Biro A. Anti-cholesterol antibodies in human sera. Autoimmun Rev 2003; 2: 272–277
- Horvath A, Banhegyi D, Biro A, Ujhelyi E, Veres A, Horvath L, Prohaszka Z, Bacsi A, Tarjan V, Romics L, Horvath I, Toth FD, Fust G, Karadi I. High level of anticholesterol antibodies (ACHA) in HIV patients. Normalization of serum ACHA concentration after introduction of HAART. Immunobiology 2001; 203: 756–768
- Huang CC, Tang M, Zhang M-Y, Majeed S, Montabana E, Stanfield RL, Dimitrov DS, Korber B, Sodroski J, Wilson IA, Wyatt R, Kwong PD. Structure of a V3-containing HIV-1 gp120 core. Science 2005; 310: 1025–1028
- Hug P, Lin HM, Korte T, Xiao X, Dimitrov DS, Wang JM, Puri A, Blumenthal R. Glycosphingolipids promote entry of a broad range of human immunodeficiency virus type 1 isolates into cell lines expressing CD4, CXCR4, and/or CCR5. J Virol 2000; 74: 6377–6385
- Jolly C, Sattentau QJ. Human immunodeficiency virus type 1 virological synapse formation in T cells requires lipid raft integrity. J Virol 2005; 79: 12088–12094
- Kliger Y, Aharoni A, Rapaport D, Jones P, Blumenthal R, Shai Y. Fusion peptides derived from the HIV type 1 glycoprotein 41 associate within phospholipid membranes and inhibit cell-cell Fusion. Structure-function study. J Biol Chem 1997; 272: 13496–13505
- Li H, Papadopoulos V. Peripheral-type benzodiazepine receptor function in cholesterol transport. Identification of a putative cholesterol recognition/interaction amino acid sequence and consensus pattern. Endocrinology 1998; 139: 4991–4997
- Liao Z, Cimakasky LM, Hampton R, Nguyen DH, Hildreth JE. Lipid rafts and HIV pathogenesis: host membrane cholesterol is required for infection by HIV type 1. AIDS Res Hum Retroviruses 2001; 17: 1009–1019
- Mañes S, del Real G, Martínez-A C. Pathogens: raft hijackers. Nature Rev Immunol 2003; 3: 557–568
- Mañes S, del Real G, Lacalle RA, Lucas P, Gómez-Moutón C, Sánchez-Palomino, Delgado R, Alcamí J, Mira E, Martínez-A C. Membrane raft microdomains mediate lateral assemblies required for HIV-1 infection. EMBO rep 2000; 1: 190–196
- Martin I, Defrise-Quertain F, Decroly E, Vandenbranden M, Brasseur R, Ruysschaert J-M. Orientation and structure of the NH2-terminal HIV-1 gp41 peptide in fused and aggregated liposomes. Biochim Biophys Acta 1993; 1145: 124–133
- Mascola JR, Stiegler G, VanCott TC, Katinger H, Carpenter CB, Hanson CE, Beary H, Hayes D, Frankel SS, Birx DL, Lewis MG. Protection of macaques against vaginal transmission of a pathogenic HIV-1/SIV chimeric virus by passive infusion of neutralizing antibodies. Nat Med 2000; 6: 207–210
- Matuoka K, Fukami K, Nakanishi O, Kawai S, Takenawa T. Mitogenesis in response to PDGF and bombesin abolished by microinjection of antibody to PIP2. Science 1988; 239: 640–643
- Matyas GR, Wassef NM, Rao M, Alving CR. Induction and detection of antibodies to squalene. J Immunol Methods 2000; 245: 1–14
- Matyas GR, Rao M, Pittman PR, Burge R, Robbins IE, Wassef NM, Thivierge B, Alving CR. Detection of antibodies to squalene: III. Naturally occurring antibodies to squalene in humans and mice. J Immunol Methods 2004; 286: 47–67
- McGaughey GB, Barbato G, Bianchi E, Freidinger RM, Garsky VM, Hurni WM, Joyce JG, Liang X, Miller MD, Pessi A, Shiver JW, Bogusky MJ. Progress towards the development of a HIV-1 gp 41-directed vaccine. Current HIV Res 2004; 2: 193–204
- McMichael AJ, Hanke T. HIV vaccines 1983–2003. Nat Med 2003; 9: 874–880
- McNeil HP, Simpson RJ, Chesterman CN, Krilis SA. Anti-phospholipid antibodies are directed against a complex antigen that includes a lipid-binding inhibitor of coagulation: beta 2-glycoprotein I (apolipoprotein H). Proc Natl Acad Sci USA 1990; 87: 4120–3124
- Miyakis S, Lockshin MD, Atsumi T, Branch DW, Brey RL, Cervera R, Derksen RH, De Groot PG, Koike T, Meroni PL, Reber G, Shoenfeld Y, Tincani A., Vlachoyiannopoulos PG, Krilis SA. International consensus statement on an update of the classification criteria for definite antiphospholipid syndrome (APS). J Thromb Haemost 2006; 4: 295–306
- Miyazawa A, Umeda M, Horikoshi T, Yanagisawa K, Yoshioka T, Inoue K. Production and characterization of monoclonal antibodies that bind to phosphatidylinositol 4,5-bisphosphate. Mol Immunol 1988; 25: 1025–1031
- Mooney JJ, Dalrymple JM, Alving CR, Russell PK. The interaction of Sindbis virus with liposomal model membranes. J Virol 1975; 15: 225–231
- Moore JP, Cao Y, Qing L, Sattentau QJ, Pyati J, Koduri R, Robinson J, Barbas CF 3rd, Burton DR, Ho DD. Primary isolates of human immunodeficiency virus type 1 are relatively resistant to neutralization by monoclonal antibodies to gp120, and their neutralization is not predicted by studies with monomeric gp120. J Virol 1995; 69: 101–109
- Nisole S, Krust B, Hovanessian A.G. Anchorage of HIV on permissive cells leads to coaggregation of viral particles with surface nucleolin at membrane raft microdomains. Exp Cell Res 2002; 276: 155–173
- Nguyen DH, Hildreth JEK. Evidence for budding of human immunodeficiency virus type 1 selectively from glycolipid-enriched membrane lipid rafts. J Virol 2000; 74: 3264–3272
- Ono A, Freed EO. Plasma mambrane rafts play a critical role in HIV-1 assembly and release. Proc Natl Acad Sci USA 2001; 98: 12925–13930
- Ono A, Ablan SD, Lockett SJ, Nagashima K, Freed EO. Phosphatidylinositol (4,5) bisphosphate regulates HIV-1 Gag targeting to the plasma membrane. Proc Natl Acad Sci USA 2004; 101: 14889–14894
- Parker CE, Deterding LJ, Hager-Braun C, Binley JM, Schulke N, Katinger H, Moore JP, Tomer KB. Fine definition of the epitope on the gp41 glycoprotein of human immunodeficiency virus type 1 for the neutralizing monoclonal antibody 2F5. J Virol 2001; 75: 10906–10911
- Pascual R, Moreno MR, Villalain J. A peptide pertaining to the loop segment of human immunodeficiency virus 41 binds and interacts with model biomembranes: implications for the fusion mechanism. J Virol 2005; 79: 5142–5152
- Piguet V, Sattentau Q. Dangerous liaisons at the virological synapse. J Clin Invest 2004; 114: 605–610
- Pike LJ. Lipid rafts: bringing order to chaos. J Lipid Res 2003; 44: 655–667
- Popik W, Alce T, Au W-C. Human immunodeficiency virus type 1 uses lipid raft-colocalized CD4 and chemokine receptors for productive entry into CD4+ cells. J Virol 2002; 76: 4709–4722
- Puri A, Hug P, Jernigan K, Barchi J, Kim HY, Hamilton J, Wiels J, Murray GJ, Brady RO, Blumenthal R. The neutral glycosphingolipid globotriaosylceramide promotes fusion mediated by a CD4-dependent CXCR4-utilizing HIV type 1 envelope glycoprotein. Proc Natl Acad Sci USA 1998; 95: 14435–14440
- Rajendran L, Simons K. Lipid rafts and membrane dynamics. J Cell Sci 2005; 118: 1099–1102
- Ran S, He J, Huang X, Soares M, Scothorn D, Thorpe PE. Antitumor effects of a monoclonal antibody that binds anionic phospholipids on the surface of tumor blood vessels in mice. Clin Cancer Res 2005; 11: 1551–1562
- Rauch J, Janoff AS. Phospholipid in the hexagonal II phase is immunogenic: evidence for immunorecognition of nonbilayer lipid phases in vivo. Proc Natl Acad Sci USA 1990; 87: 4112–4114
- Richards RL, Aronson J, Schoenbechler M, Diggs CL, Alving CR. Antibodies reactive with liposomal phospholipids are produced during experimental Trypanosoma rhodesiense infections in rabbits. J Immunol 1983; 130: 1390–1394
- Rietveld A, Simons K. The differential miscibility of lipids as the basis for the formation of functional membrane rafts. Biochim Biophys Acta 1998; 1376: 467–479
- Roubey RA, Eisenberg RA, Harper MF, Winfield JB. “Anticardiolipin” autoantibodies recognize beta 2-glycoprotein I in the absence of phospholipid. Importance of Ag density and bivalent binding. J Immunol 1995; 154: 954–960
- Sáez-Cirión A, Nir S, Lorizate M, Agirre A, Cruz A, Pérez-Gil J, Nieva JL. Sphingomyelin and cholesterol promote HIV-1 gp41 pretransmembrane sequence surface aggregation and membrane restructuring. J Biol Chem 2002; 277: 21776–21785
- Sanchez-Martinez S, Lorizate M, Katinger H, Kunert R, Basanez G, Nieva JL. Specific phospholipid recognition by human immunodeficiency virus type-1 neutralizing anti-gp41 2F5 antibody. FEBS Lett 2006; 580: 2395–2399
- Schuster B, Neidig M, Alving BM, Alving CR. Production of antibodies against phosphocholine, phosphatidylcholine, sphingomyelin, and lipid A by injection of liposomes containing lipid A. J Immunol 1979; 122: 900–905
- Seddon JM. Structure of the inverted hexagonal (HII) phase and non-lamellar phase transitions of lipids. Biochim Biophys Acta 1990; 1031: 1–69
- Silvestris F, Frassanito MA, Cafforio P, Potenza D, Di Loreto M, Tucci M, Grizzuti MA, Nico B, Dammacco F. Antiphosphatidylserine antibodies in human immunodeficiency virus-1 patients with evidence of T-cell apoptosis and mediate antibody-dependent cellular cytotoxicity. Blood. 1996; 87: 5185–5195
- Simons K, Ikonen E. Functional rafts in cell membranes. Nature 1997; 387: 569–572
- Simons K, Vaz WL. Model systems, lipid rafts, and cell membranes. Annu Rev Biophys Biomol Struct 2004; 33: 269–295
- Singer SJ, Nicolson GL. The fluid mosaic model of the structure of cell membranes. Science 1972; 175: 720–731
- Stollar BD, McInerney T, Gavron T, Wassef NM, Swartz GM, Jr, Alving CR. Cross-reactions of nucleic acids with monoclonal antibodies to phosphatidylinositol phosphate and cholesterol. Mol Immunol 1989; 26: 73–79
- Subczynski WK, Kusumi A. Dynamics of raft molecules in the cell and artificial membranes: approaches by pulse EPR spin labeling and single molecule optical microscopy. Biochim Biophys Acta 2003; 1610: 231–243
- Swartz GM, Jr, Gentry MK, Amende LM, Blanchette-Mackie EJ, Alving CR. Antibodies to cholesterol. Proc Natl Acad Sci USA 1988; 85: 1902–1906
- Trkola A, Kuster H, Rusert P, Joos B, Fischer M, Leemann C, Manrique A, Huber M, Rehr M, Oxenius A, Weber R, Stiegler G, Vcelar B, Katinger H, Aceto L, Gunthard HF. Delay of HIV-1 rebound after cessation of antiretroviral therapy through passive transfer of human neutralizing antibodies. Nat Med 2005; 11: 615–622
- Trommeshauser D, Krol S, Bergelson LD, Galla HJ. The effect of lipid composition and physical state of phospholipid monolayer on the binding and incorporation of a basic amphipathic peptide from the C-terminal region of the HIV envelope protein gp41. Chem Phys Lipids 2000; 107: 83–92
- Umeda M, Igarashi K, Nam KS, Inoue K. Effective production of monoclonal antibodies against phosphatidylserine: stereo-specific recognition of phosphatidylserine by monoclonal antibody. J Immunol 1989; 143: 2273–2279
- Vaarala O, Palosuo T, Kleemola M, Aho K. Anticardiolipin response in acute infections. Clin Immunol Immunopath 1986; 41: 8–15
- Vincent N, Genin C, Malvoisin E. Identification of a conserved domain of the HIV-1 transmembrane protein gp41 which interacts with cholesteryl groups. Biochim Biophys Acta 2002; 1567: 157–164
- Wassef NM, Roerdink F, Swartz GM, Jr, Lyon JA, Berson BJ, Alving CR. Phosphate binding specificities of monoclonal antibodies against phosphoinositides in liposomes. Mol Immunol 1984; 21: 863–868
- Wassef NM, Swartz GM, Jr, Alving CR, Kates M. Antibodies to liposomal phosphatidylcholine and phosphatidylsulfocholine. Biochem Cell Biol 1990; 68: 54–58
- Waugh MG, Lawson D, Tan SK, Hsuan JJ. Phosphatidylinositol 4-phosphate synthesis in immunoisolated caveolae-like vesicles and low buoyant density non-caveolar membranes. J Biol Chem 1998; 273: 17115–17121
- Wyatt R, Sodroski J. The HIV-1 envelope glycoproteins: fusogens, antigens, and immunogens. Science 1998; 280: 1884–1888
- Yu X, Yuan X, Matsuda Z, Lee T-H, Essex M. The matrix protein of human immunodeficiency virus type 1 is required for incorporation of viral envelope protein into mature virions. J Virol 1992; 66: 5966–4971
- Zwick MB, Labrijn AF, Wang M, Spenlehauer C, Saphire EO, Binley JM, Moore JP, Stiegler G, Katinger H, Burton DR, Parren PW. Broadly neutralizing antibodies targeted to the membrane-proximal external region of human immunodeficiency virus type 1 glycoprotein gp41. J Virol 2001; 75: 10892–10905