Abstract
We have shown previously that truncating all of the variable cytoplasmic C-terminus of Kv1.1 potassium channels to G421stop had only a small inhibitory effect on their cell surface conductance density levels and cell surface protein levels. Here we investigated the role of a highly conserved cytoplasmic C-terminal charged region of five amino acids (HRETE) of the S6 transmembrane domain in the protein and conductance expression of Kv1.1, Kv1.2, and Kv1.4 channels. For Kv1.1 we found that E420stop, T419stop, and E418stop showed cell surface conductance densities and cell surface protein levels similar to full length control, whereas R417stop and H416stop exhibited essentially no conductance but their surface protein levels were similar to full length control. A bulky non-negatively charged hydrophilic amino acid at position 417 appeared to be critical for wild type gating of Kv1.1 because R417K and R417Q rescued conductance levels whereas R417A or R417E did not. The R417A mutation in the full length Kv1.1 also exhibited surface protein levels similar to control but it did not exhibit significant conductance. In contrast, mutation of the equivalent arginine to alanine in full length Kv1.2 and Kv1.4 appeared to have little or no effect on channel conductance but rather decreased cell surface protein levels by inducing partial high ER retention. These findings are consistent with the notion that the arginine amino acid in the HRETE region plays a different role in affecting conductance levels or cell surface protein levels of very closely related Kv1 potassium channels.
Introduction
Voltage-gated potassium (K) channels play critical roles in action potential repolarization and comprise a large gene family Citation[1]. Kv type K channels within a subfamily can form functional homo- or heterotetramers in expression systems and many amino acid regions have been identified that affect function Citation[1–3].
Kv1 channels are found throughout many regions of the brain as heteromers, and in some cases as homomers, that may be associated with different auxiliary subunits Citation[1–3]. Kv type channels appear to fold into tetramers in the endoplasmic reticulum (ER) and regions involved in tetramerization include an N-terminus domain and S1 and S2 transmembrane segments Citation[1–6]. Regions involved in high surface expression in the Kv1 subfamily include motifs mapped to the cytoplasmic C-terminus Citation[7] and the pore region Citation[8], Citation[9]. For example, and it has been proposed that outer pore region amino acids Citation[8], Citation[9] as well as the deep pore region amino acids Citation[10] in the Kv1 subfamily are major determinants for cell surface expression trafficking. Trafficking defects in membrane proteins are the cause of a number of human disorders Citation[11]. Kv1 channel's cytoplasmic C-terminus is composed of a nearly constant region of five amino acids (HRETE), which directly follows the functionally important S6 transmembrane domain, and a variable C-terminal region of 70–90 amino acids. Truncating the cytoplasmic C-terminus variable region of Kv1.1 had only a small inhibitory effect on trafficking to the cell surface Citation[8], Citation[12], whereas it had a large inhibitory effect on Kv1.4 due to the cytoplasmic trafficking determinant VXXSL Citation[7]. The HRETE region directly follows the S6 transmembrane domain, which is important in pore formation and activation gating, but yet its possible role in Kv1 biology is not well understood. It is known that a truncation in this region in Kv1.1, Kv1.1R417stop codon, is one mutation in human Episodic Ataxia type-I (EA-1) that appears to prevent functional expression of the mutant [Citation[13], and see 11 for review].
Given the above observations, the aim of this study was to investigate the role of this constant C-terminus region (HRETE) in the protein and conductance expression of Kv1.1, Kv1.2, and Kv1.4 potassium channels, which are heavily expressed in the brain.
Materials and methods
Molecular biology and transfections
Rat brain Kv1.1, Kv1.2, and Kv1.4 Citation[14] cDNAs were used for mutagenesis by polymerase chain reaction or replication to construct various cDNAs in the pcDNA3 vector Citation[8], Citation[12] and the cDNA was sequenced to confirm its integrity. CHO (chinese hamster ovary) pro5 cells were maintained and transiently transfected with 0.5 microgram plasmid that contained a Kv1 cDNA construct per 35mm dish and cells were analyzed 24 hours posttransfection as described previously Citation[8], Citation[12].
Cell surface Kv1 conductance density by patch-clamping
CHO cells were cotransfected with 0.5 microgram Kv1 containing plasmid and 0.1 microgram green fluorescence protein (GFP) containing plasmid for visualization of transfected cells by fluorescence microscopy. Patch-clamping of transiently transfected CHO cells has been described in detail previously Citation[8], Citation[12]. The holding potential was −80mV, and then the cells were depolarized to 50mV for 80msec. Current amplitudes were used to calculate conductance maximum and then conductance maximum/capacitance (Gm/Cm) values were calculated Citation[8], Citation[12]. Data are shown as normalized values to a control condition, which was taken as 100.0±SEM. Nontransfected CHO cells exhibited either no voltage-gated outward currents (hold −80mV, depolarize to 50mV) or occasionally very small whole cell outward currents of ∼50–80pA Citation[8], whereas cells transfected with wild type Kv1 channels exhibited currents that were >30-fold this level. Some Kv1 mutant constructs exhibited outward whole cell currents of ∼50–80pA (hold −80mV, depolarize to 50mV), and we considered this level to represent essentially no conductance expression above background as defined as Gm/Cm. From patch clamp recordings, the order of surface conductance densities of Kv1 channels used in this study is, Kv1.4≫Kv1.2 > Kv1.1.
Cell surface Kv1 protein and Kv1 total protein levels
Intact cells were biotinylated by the hydrazide-LC-biotin (Pierce) reagent method as we have described Citation[8], Citation[12]. An aliquot of the total 1ml solubilized lysate was saved for total Kv1 protein estimations and the biotinylated cell surface proteins isolated with streptavidin-beads were used for cell surface Kv1 protein determination by immunoblotting. Samples were run on 9% SDS gels and processed for immunoblotting with Kv1.1 (1:1000 polyclonal) Citation[8], Kv1.2 (1:2000 monoclonal, Upstate Biotechnology, NY) Citation[15], Kv1.4 (1:2000 monoclonal, Upstate Biotechnology, NY) Citation[15] antibodies. Horseradish peroxidase-linked anti-specific secondary antibodies were used to visual Kv1 proteins by enhanced chemiluminscence (ECL detection kit, Amersham) and preflashed X-ray film (AR5, Kodak). Nontransfected CHO cells do not express endogenous Kv1.1, Kv1.2, or Kv1.4 proteins as assayed by immunoblotting (data not shown). The filter was then stripped and incubated with actin antibodies (clone AC-40, Sigma) to estimate actin amounts to control for any cell density differences between wells. Signals on an X-ray film were quantified as follows. A microtex 8700 scanmaker (dynamic range of 0–3.5) was used to scan a film image and densitometry analysis was performed with the NIH Image 1.6 software with an internal and external standard as we have described Citation[8], Citation[12]. Kv1 cell surface proteins and Kv1 cell total proteins are normalized to a control value, which was taken as 100.0±SEM. Relative surface expression levels are calculated by dividing normalized surface values by normalized total values to compare with a control value of 1.0. The ECL detection system was linear to ∼1–20 fold range by an immunoblot assay on serially diluted total cell protein from transfected cells (data not shown).
Another method was used to estimate cell surface protein levels of some Kv1.1 constructs. Kv1.1, Kv1.1R417stop, and Kv1.1R417A were engineered with the flag epitope on their extracellular S1-S2 linkers (the flag epitope is DYKDDDDK with the N terminus D amino acid of the epitope engineered after amino acid D197 of the S1-S2 loop). The integrity of the DNAs was confirmed by DNA sequenceing. Untransfected cells or cells transfected with Kv1.1 (without the flag epitope) showed no immunoreactivity to the flag monoclonal antibody (Kodak) on immunoblots (data not shown). Intact transfected cells (with Kv1.1-flag, Kv1.1R417stop-flag, or Kv1.1R417A-flag) were placed at 5°C and the flag monoclonal antibody (Kodak) was added at 1/100 dilution for 40 minutes. Under these conditions the flag antibody should only bind to Kv1.1 constructs on the cell surface. Cells were then washed, solubilized in Triton X-100 cell lysis bufffer in 1ml, and Kv1.1 construct surface proteins that bound the flag antibody were precipitated with protein A-beads (50 µl of a 1:1 slurry of protein-A beads (Sigma)). The beads were washed and eluted with SDS sample buffer and then immunoblotted with the Kv1.1 antibody. Cells transfected with Kv1.1 (without the flag epitope) and processed as above showed no immunoreactivity on immunoblots (data not shown).
Kv1 protein levels in an enriched ER fraction
CHO cells were transfected as above and scraped and homogenized in hypotonic media as we have described previously Citation[8]. The homogenate was microcentrifuged at 7,000 rpm for 5 min to remove nuclei. The 1 ml supernant containing vesicles was transferred to another tube and calnexin antibody was added (1:300 calnexin polyclonal antibody to the cytoplasmic tail of calnexin (Sressgen)). Calnexin is a transmembrane chaperone protein enriched in the ER but some cells may also have a small fraction (<5%) of this protein that goes to the cell surface Citation[16]. 50 µl of a 1:1 slurry of protein-A beads (Sigma) was added to affinity purify or enrich for an ER fraction. After bead washing, the eluted material was immunoblotted with Kv1 monoclonal antibodies and the signal detected and estimated as outlined above. Protein A-beads added without calnexin antibodies did not purify any Kv1 immunopositive material (data not shown).
Results
In the Kv1.1 HRETE region, the arginine affected channel conductance levels but had little effect on cell surface channel protein levels
To investigate the effect of the highly conserved HRETE region, which is nearly identical in the Kv1 known subfamily members, on the conductance level and cell surface protein level of Kv1 channels, we first truncated the complete C-terminus (constant and variable regions) by mutating H416 to a stop codon in Kv1.1 and expressing it in CHO cells. In our study here conductance levels are defined as detectable whole cell channel conductance levels (conductance/capacitance) (see methods). Kv1.1H416stop essentially did not express any detectable whole cell conductance versus full length Kv1.1 (referred to as Kv1.1) as determined by whole cell patch-clamping and conductance/capacitance (A). Kv1.1H416stop and Kv1.1 surface protein levels and total protein levels were determined by biotinylation/immunoblotting and immunoblotting, respectively, and levels were expressed as normalized values to Kv1.1. The Kv1.1H416stop mutant exhibited ∼85% the cell surface protein level (B) and ∼80% the total protein level (C) as Kv1.1. Dividing the surface protein (B) by the total protein (C) gave a relative surface expression level for Kv1.1H416stop that was similar to the control value for Kv1.1 (D) and this suggested that Kv1.1H416stop was not retained intracellularly to a higher degree versus Kv1.1. Relative surface expression levels can be viewed as a reflection of the trafficking ability of a test construct (e.g. Kv1.1H416stop) compared with a control (e.g., Kv1.1). These results suggested that the Kv1.1H416stop mutant inhibited conductance levels dramatically, whereas there was only a small inhibitory effect on cell surface protein and cell total protein levels, which was presumably due to a decreased protein stability of the mutant. As we have shown previously, the truncation of the variable C-terminus region did not affect the conductance levels or trafficking of Kv1.1 Citation[8] and thus the effects above were due to truncation of the HRETE region. These results suggested that the HRETE region in Kv1.1 was critical in promoting wild type conductance levels but it had little effect on protein trafficking to the cell surface.
Figure 1. Kv1.1H416stop affected conductance levels more than cell surface protein levels. Transfected CHO cells were analyzed for Kv1 functional expression (Gm/Cm) by patch-clamping and for Kv1 cell surface protein levels by biotinylation/immunoblotting and Kv1 total protein levels by immunoblotting as described in methods. (A) Conductance densities (Gm/Cm, maximum conductance divided by capacitance) of Kv1.1 and Kv1.1H416stop by patch-clamping. Kv1.1 values were taken as 100.0±SEM and Kv1.1H416stop values were normalized to it (n=8–12). Representative current traces for the Kv1.1 constructs are shown at the bottom with ms = milliseconds and nA = nanoamp. (B) Surface protein levels of Kv1.1 and Kv1.1H416stop from biotinylation/immunoblots. Kv1.1 values were taken as 100.0±SEM and Kv1.1H416stop values were normalized to it (n=3). (C) Total protein levels of Kv1.1 and Kv1.1H416stop (immunoblots not shown). Kv1.1 values were taken as 100.0±SEM and Kv1.1H416stop values were normalized to it (n=3). (D) Relative surface expression by dividing the surface protein values in B by the total protein values in C.
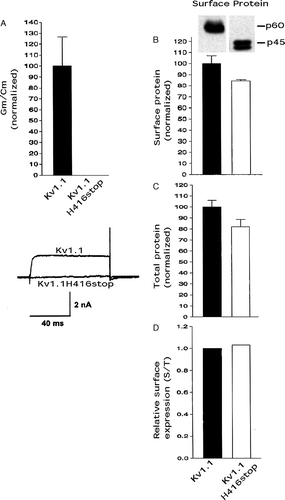
We then tested the effects of truncating the C-terminus at each amino acid in the HRETE region (A) and normalized these mutants’ surface conductance densities, surface protein levels, and total protein levels to full length Kv1.1. G421stop, E420stop, T419stop, and E418stop all exhibited similar conductance densities as Kv1.1 (B), whereas cell surface protein levels were at least ∼85% (C) and cell total protein levels were ∼60–80% (D) of Kv1.1. The relative surface expression level of the mutants and control Kv1.1 were similar (E) and this suggested they were not retained intracellularly to a higher degree versus Kv1.1. In contrast, R417stop, one of the mutations in human EA-1, essentially did not functionally express (B), as has been previously reported by other investigators [17, and see 11 for review]. However, R417stop cell surface protein and cell total protein levels were only modestly decreased to ∼80–85% of Kv1.1 (C & D). The relative surface expression level of R417stop was similar to control Kv1.1 (E) and this suggested it was not retained intracellularly to a higher degree versus Kv1.1. These findings for R417stop were similar to those for H416stop (A–C) and suggested that the dramatically decreased function recorded for this mutant were mostly due to the removal of the R417 amino acid. These results suggested that an amino acid at the arginine position in the HRETE region of Kv1.1 was required for wild type channel conductance level, whereas both cell surface protein and cell total protein levels for the mutant were only slightly decreased by the arginine removal. The slightly decreased surface protein level appeared due to a similarly decreased total protein level, presumably due to decreased protein stability.
Figure 2. Kv1.1R417stop affected conductance levels more than cell surface protein levels and a bulky hydrophilic amino acid substitution at R417 restored conductance expression. Transfected CHO cells were analyzed for conductance densities, surface protein levels, and total protein levels as described in the legend. (A) The HRETE sequence in full length Kv1.1 and Kv1.1 truncation mutants used here. (B) Kv1.1 conductance density (Gm/Cm) was taken as 100.0±SEM and the Kv1.1 truncation mutants were normalized to it (n=8–12). (C) Kv1.1 surface protein was taken as 100.0±SEM and the Kv1.1 truncation mutants were normalized to it (n=3) (immunoblots not shown). (D) Kv1.1 total protein was taken as 100.0±SEM and the Kv1.1 truncation mutants were normalized to it (n=3) (immunoblots not shown). (E) Relative surface expression by dividing the surface protein values in C by the total protein values in D.
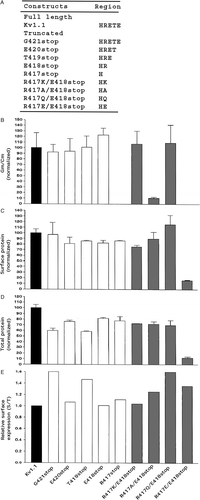
Figure 3. Kv1.1R417A affected conductance levels more than cell surface protein levels. Transfected CHO cells were analyzed for conductance densities, surface protein levels, and total protein levels as described in the legend. (A) The HRETE sequence in full length Kv1.1 and the full length Kv1.1 site-directed mutants used here. (B) Kv1.1 conductance density (GmCm) was taken as 100.0±SEM and the Kv1.1 site-directed mutants were normalized to it (n=8–12). (C) Kv1.1 surface protein was taken as 100.0±SEM and the Kv1.1 site-directed mutants were normalized to it (n=3). (D) Kv1.1 total protein was taken as 100.0±SEM and the Kv1.1 site-directed mutants were normalized to it (n=3) (immunoblots not shown). (E) Relative surface expression by dividing the surface protein values in C by the total protein values in D. (F) Enriched ER (endoplasmic reticulum) Kv1.1 protein as outlined in methods. Kv1.1 enriched ER protein was taken as 100.0±SEM and the Kv1.1R417A was normalized to it (n=3) (immunoblots not shown). (G) Cell surface protein of Kv1.1-flag, Kv1.1R417A-flag, and Kv1.1R417stop-flag (flag #1 and flag #2 are two cDNAs recovered from different bacterial colonies) by flag antibody binding to cell surface Kv1.1 construct proteins in intact cells followed by immunopurification of these complexes with flag antibody and then immunoblotting with Kv1.1 antibody.
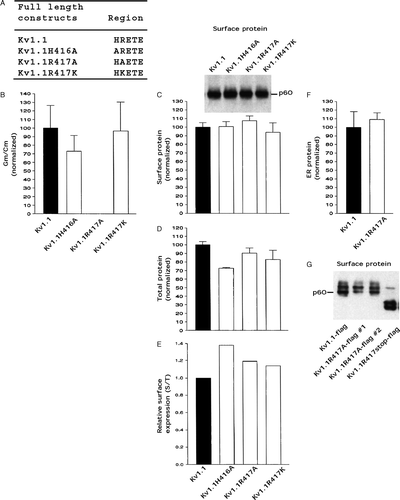
In the Kv1.1 HRETE region, a bulky non-negatively charged hydrophilic amino acid at the arginine position appeared to be required for wild type conductance levels
Which amino acid substitutions at the R417 position in the HRETE region of Kv1.1 will restore wild type conductance levels? A number of amino acid substitutions at position 417 were engineered on to the E418stop truncated construct (A). R417K/E418stop and R417Q/E418stop both restored conductance density levels (B) and their cell surface protein levels were at least ∼80% (C) and their cell total protein levels were ∼70% (D) of Kv1.1. In contrast, both R417A/E418stop and R417E/E418stop did not restore wild type function and they exhibited decreased conductance densities similar to R417stop (B). R417A/E418stop cell surface protein and cell total protein levels were ∼70–90% of Kv1.1 (C & D). In contrast, R417E/E418stop cell surface protein and cell total protein levels were ∼15% of Kv1.1 (C & D) and this suggested that their low surface protein level was due to decreased protein stability. The relative surface expression levels of these four mutants were not less than the 1.0 of Kv1.1 (E) and this was consistent with the notion that they were not retained intracellularly to a higher degree than Kv1.1.
Next, single amino acid substitutions were made at pertinent positions in the HRETE region of full length Kv1.1 ( A). Kv1.1H416A and Kv1.1R417K both exhibited somewhat similar conductance densities versus Kv1.1, whereas Kv1.1R417A exhibited little or no conductance (B). All three Kv1.1 mutants had at least ∼95% the cell surface protein levels (C) and ∼70–90% the cell total protein levels (D) as Kv1.1. Thus these mutants also had a relative surface expression level that was not less than the 1.0 of Kv1.1 (E) and this indicated that they were not retained intracellularly to a higher degree versus Kv1.1. Consistent with this notion, the protein level of Kv1.1 and Kv1.1R417A in an enriched ER membrane fraction (see Materials and methods) were similar (F).
In addition, we used another technique described in Materials and methods to confirm that both Kv1.1R417A full length and Kv1.1R417stop were on the cell surface by using Kv1.1, Kv1.1R417A full length, and Kv1.1R417stop with a flag epitope tag engineered on their extracellular S1-S2 loops. Consistent with our data using the cell surface biotinylation method on transfected cells, we found that Kv1.1R417A-flag and Kv1.1R417stop-flag were detected at similar protein levels on the cell surface as Kv1.1-flag (G). The explanation for the apparent increased heterogeneity of bands detected using Kv1.1 flag constructs in G is currently unknown.
In the Kv1.2 and Kv1.4 HRETE regions, the arginine affected channel surface protein levels but had little effect on channel conductance levels
We also tested the effect of the arginine in the HRETE region in full length Kv1.2 and full length Kv1.4 which both have similar amino acid sequences to Kv1.1. Kv1.2R419A and Kv1.4R571A (A) exhibited conductance densities that were only ∼15% and ∼40%, respectively, of Kv1.2 and Kv1.4 (B). Somewhat surprisingly Kv1.2R419A and Kv1.4R571A exhibited surface protein levels of only ∼5% and ∼10%, respectively, of Kv1.2 and Kv1.4 (C), whereas their total protein levels were ∼65% and ∼70%, respectively, of the level of Kv1.2 and Kv1.4 (D), and thus both Kv1.2R419A and Kv1.4R571A had low relative surface expression levels of ∼0.05 and ∼0.10, respectively, versus wild type (E). This finding suggested that both Kv1.2R419A and Kv1.4R571A were retained intracellularly to a higher degree versus wild type. Consistent with this notion it was found that the protein level of Kv1.2R419A in an enriched ER membrane fraction (see methods) was higher than Kv1.2 (F).
Figure 4. An R to A mutation in HRETE in Kv1.2 and Kv1.4 affected cell surface protein levels more than conductance levels. Transfected CHO cells were analyzed for conductance density, surface protein level, and total protein level as described in the legend. (A) The HRETE sequence in full length Kv1.2, Kv1.2R419A, Kv1.4, and Kv1.4R571A. (B) Kv1.2 conductance density (Gm/Cm) was taken as 100.0±SEM and Kv1.2R419A was normalized to it (n=8–12). Kv1.4 conductance density (Gm/Cm) was taken as 100.0±SEM and Kv1.4R571A was normalized to it (n=8–12). Representative current traces for the Kv1.2 and Kv1.4 constructs are shown at the bottom. (C) Kv1.2 surface protein was taken as 100.0±SEM and Kv1.2R419A was normalized to it (n=3). Kv1.4 surface protein was taken as 100.0±SEM and Kv1.4R571A was normalized to it (n=3). (D) Kv1.2 total protein was taken as 100.0±SEM and Kv1.2R419A was normalized to it (n=3) (immunoblots not shown). Kv1.4 total protein was taken as 100.0±SEM and Kv1.4R571A was normalized to it (n=3) (immunoblots not shown). (E) Relative surface expression by dividing the surface protein values in C by the total protein values in D. (F) Enriched ER (endoplasmic reticulum) Kv1.2 protein as outlined in methods. Kv1.2 enriched ER protein was taken as 100.0±SEM and the Kv1.2R419A was normalized to it (n=3) (immunoblots not shown).
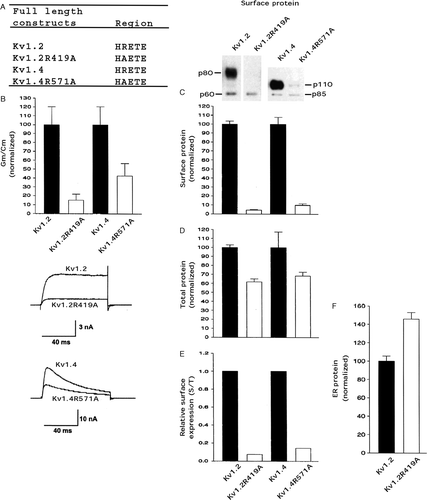
The differential effect of arginine substitution in the HRETE region of Kv1.1, Kv1.2, and Kv1.4 appears to be dependent on a Kv1 trafficking code
It has been proposed that Kv1 subfamily members have different trafficking codes Citation[7–10]. For example, we showed that two amino acids in their deep pore regions appear to be a trafficking code and dictated whether these proteins were expressed at high or low cell surface levels and the amino acid pair order that promoted the highest relative surface expression was T,K (Kv1.4)≫S,V (Kv1.2) > S,Y (Kv1.1) Citation[10].
In our current study, it appears that Kv1 members with a weak trafficking code, such as Kv1.1, exhibit channel dysfunction with an arginine to alanine mutation in the HRETE region, whereas members with a stronger trafficking code, such as Kv1.4 or Kv1.2, did not, but rather exhibited a decreased relative surface protein level. To explore this observation further, we used Kv1.1S369T, which has less ER retention and greater relative surface protein levels versus Kv1.1 Citation[10] and constructed the Kv1.1S369T/R417A double mutant. Kv1.1S369T/R417A (A), compared with control Kv1.1S369T, exhibited a conductance density and a surface protein level of ∼35% (B & C), a total protein level of at least 100% (D), and a relative surface protein level of only ∼0.25 (E). Kv1.1S369T/R417A functionally expressed and exhibited a decreased conductance density because it had a decreased cell surface protein level, due to high partial intracellular retention. The differential effects of arginine to alanine substitution in the HRETE region of Kv1 channels appears dependent on a Kv1 trafficking code.
Figure 5. The double mutant Kv1.1S369T/R417A affected cell surface protein levels more than conductance levels. Transfected CHO cells were analyzed for conductance densities, surface protein levels, and total protein levels as described in the legend. (A) The HRETE sequence in full length Kv1.1S369T and Kv1.1S369T/R417A. (B) Kv1.1S369T conductance density (Gm/Cm) was taken as 100.0±SEM and Kv1.1S369T/R417A was normalized to it (n=8–12). (C) Kv1.1S369T surface protein was taken as 100.0±SEM and Kv1.1S369T/R417A was normalized to it (n=3). (D) Kv1.1S369T total protein was taken as 100.0±SEM and Kv1.1S369T/R417A was normalized to it (n=3) (immunoblots not shown). (E) Relative surface expression by dividing the surface protein values in C by the total protein values in D.
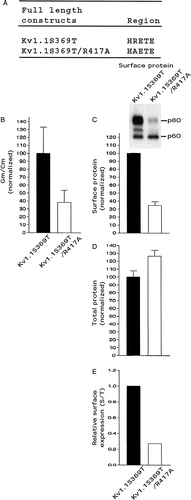
Discussion
The HRETE region, and the S6, is identical in Kv1.1, Kv1.2, and Kv1.4, whereas Kv2, Kv3, and Kv4 subfamily members have very different amino acids here. This suggested that the HRETE region may play a similar role in Kv1 subfamily members. Surprisingly, we found that mutation of an arginine to alanine in the HRETE region affected Kv1.1, Kv1.2, and Kv1.4 differentially. The R417A mutation in Kv1.1 caused channel dysfunction and had little effect on cell surface protein levels, whereas the R419A mutation in Kv1.2 and the R571A mutation in Kv1.4 had little or no effect on channel conductance but rather decreased cell surface protein levels. These differential effects on the conductance and protein surface levels of Kv1.1 versus Kv1.2 and Kv1.4 appeared to be dictated by a Kv1 trafficking code. Subtle differences between Kv1.1 and Kv1.2, Kv1.4 in their 3D folded structures around the HRETE region are presumably responsible for these differential effects. Complete insight into the underlying mechanism of these differential effects would require the 3D crystal structure of the open and closed states of each channel. With limited information about these structures, in the following discussion we attempt to give possible explanations concerning underlying mechanisms.
A crystal structure of a mammalian Kv1.2/Kvβ complex has been reported and proposed to be in an open conformation Citation[17], Citation[18]. A subregion of the S6 distal region, amino acids NFNYFY, has been termed a receptor or a platform for the S4-S5 linker Citation[17], Citation[18], because they are in contact with each other. The S4-S5 linker region is identical in Kv1.1 and Kv1.2 but Kv1.4 has two amino acids that are different in this region. It has been proposed that activating depolarizations move the S4 region outward and this moves the S4-S5 linker:NFNYFY region (activation gate) which opens the channel Citation[18]. The HRET region backbone helix in the crystal structure was resolved, but not the amino acid side chains and the helix is immediately distal to this contact region, whereas the rest of the C-terminus was not resolved and its structure/position is unknown. Although the exact position of the R419 side chain of Kv1.2 is not known it is localized in a region that could play a role in affecting channel gating.
The arginine substitution in the HRETE region of Kv1.1
Kv1.1R417stop is one mutation in human EA- I and homomeric mutant channels essentially do not express functionally Citation[19]. In our current study, we also found that homomeric Kv1.1R417stop, as well as homomeric full length Kv1.1R417A, exhibited essentially no conductance and that this was due to dysfunctional gating because the protein was expressed on the surface at similar levels as wild type Kv1.1. Thus it appears that in wild type Kv1.1 the R417 is located in a region that affects channel gating. Although we did not make all amino acid substitutions at R417 of Kv1.1, we found the arginine in the HRETE region of Kv1.1 could be replaced with a bulky non-negatively charged hydrophilic amino acid, such as positively charged lysine or neutral glutamine, and exhibit wild type gating, whereas replacement with an alanine or a glutamic acid caused channel dysfunction. We suggest that lysine or glutamine substitution at 417 promotes channel gating because they are both relatively large hydrophilic amino acids, whereas an alanine or glutamic acid substitution does not due to their small size- and for glutamic acid because of its negative charge. We can not eliminate the possibility that the lack of detectable whole cell conductance in these later Kv1.1 mutants could also be due to a very large decrease in single channel conductance and/or in mean channel open time. In any event the result is a nonfunctional channel.
Kv1.1R417A on the cell surface exhibited no conductance, whereas as Kv1.1S369T/R417A did and its phenotype was more similar to Kv1.2R419A and Kv1.4R571A. Thus the effect of the arginine to alanine mutation for Kv1.1 was dependent on an amino acid in the pore region at 369 which we have identified as playing a role in trafficking Citation[8], Citation[10], Citation[11]. We speculate that this differential effect on the phenotypic readout of Kv1.1R417A versus Kv1.1R417A/S369T is due to subtle differences in their deep pore structures. However, Kv1.2 also has a serine at an equivalent position whereas Kv1.4 has a threonine. Thus the presence of a serine alone does not dictate the phenotypic effect of an arginine to alanine mutation in the HRETE region in the Kv1 subfamily.
The arginine substitution in the HRETE region of Kv1.2 and Kv1.4
The arginine to alanine substitution in the HRETE region of full length Kv1.2 or Kv1.4 did not appear to affect channel conductance to the extent we showed for Kv1.1. We speculate that this may be due to differences in the local amino acid environments of these two channels versus Kv1.1 surrounding the HRETE region. That is, the C-terminus amino acids, which show numerous differences in these channels, could have their amino acid side chains positioned near the HRETE region and this could affect the arginine side chain position differentially. For Kv1.2 and Kv1.4 the arginine to alanine mutation decreased channel cell surface protein levels by inducing partial high intracellular ER retention. We speculate that for Kv1.2 and Kv1.4 the arginine promotes ER exit by promoting proper or timely protein folding and/or it may interact directly or indirectly with an ER chaperone like-protein(s) which promotes efficient trafficking of these proteins from the ER to the Golgi, and thus to the cell surface. Our additional investigations suggested that the ER chaperone calnexin, which helps in the folding of N-glycosylated proteins, appeared to be associated with Kv1.2 to a higher degree than Kv1.2R419A (data not shown). This observation is consistent with the notion that less association of calnexin with Kv1.2R419A may inhibit its exit from the ER, and thus affect its surface expression levels, or that endogenous calnexin amount may be a limiting factor. For example, another group suggested that endogenous calnexin may be a limiting factor in COS cells and that cotransfection of exogenous calnexin may help in the ER export of some Kv1 channels Citation[20].
Other studies on Kv1.1R417stop
The Kv1.1R417stop protein level in cells has been investigated by other groups. One group found that Kv1.1 and Kv1.1R417stop in transfected COS cells had similar total protein levels by immunoblotting (as we found in our current study with CHO cells) but that Kv1.1R417stop, which was assembled into tetramers, exhibited several characteristics associated with a misfolded protein- but this study did not determine whether the Kv1.1R417stop protein was on the cell surface Citation[21]. Truncation of the variable C-terminus of Kv1.1 did not result in misfolded protein characteristics, which suggested that possible folding determinants on Kv1s were localized to the highly conserved region just distal to the S6 Citation[21]. In contrast, the full length Kv1.1R417A mutant, or other individual amino acid mutations in this conserved region, did not give the misfolded phenotype of Kv1.1R417stop, which suggested that the truncation of the short conserved region was required for the EA-I phenotype Citation[21]. Thus from data in our current report, Kv1.1R417stop and full length Kv1.1R417A channel dysfunction does not appear to be due solely to protein misfolding. In addition, expression of a full length Kv1.1R417A mutant may also result in an EA-I phenotype if the defective function is not rescued by another wild type Kv1 subunit.
Another group estimated cell surface protein and cytoplasmic protein levels for GFP-Kv1.1 and GFP-Kv1.1R417stop by using confocal laser scanning fluorescence microscopy Citation[19]. GFP-Kv1.1R417stop protein was barely detected on the cell surface or in the cytoplasm which suggested that the truncation decreased channel protein stability. These data are different from our current work where we found by using two different methods (cell surface biotinylation and epitope tagging of extracellular loops) that Kv1.1R417stop and Kv1.1R417A had ∼85% the surface and total protein levels as Kv1.1. The explanations for these differences is currently unknown but possibilities include expression system differences (we used mammalian cells versus frog oocytes) and/or construct differences (we used Kv1.1 and Kv1.1R417stop and estimated protein levels with antibodies by two different methods versus the other study which used GFP-Kv1.1 and GFP-Kv1.1R417stop and estimated protein levels by fluorescence microscopy).
Conclusion
Our results suggest that the arginine in the HRETE region affected Kv1 subfamily members differentially. When the arginine in the Kv1.1 HRETE region was truncated or mutated to alanine, the mutant protein was expressed on the cell surface at similar levels as control but it exhibited little or no conductance. In contrast, when the arginine in the HRETE regions of Kv1.2 or Kv1.4 was mutated to alanine, the mutant protein surface protein levels were less than control and thus the conductance levels were also equivalently less. Kv1 potassium channels play critical roles in animal physiology and thus defining the different subregions on these channels that are involved in their folding, trafficking, and/or function are of considerable interest.
This paper was first published online on iFirst on 10 January 2007.
Acknowledgements
This research was supported by NIH Grant, USA, NS048906 (W.B.T.).
References
- Hille B. Ionic channels of excitable membranes3rd ed. Sinauer, Sunderland, MA 2001
- Jan LY, Jan YN. Cloned potassium channels from eukaryotes and prokaryotes. Annu Rev Neurosci 1997; 20: 91–123
- Coetzee WA, Amarillo Y, Chiu J, Chow A, Lau D, McCormack T, Moreno H, Nadal MS, Ozaita A, Pountney D, Saganich M, Vega-Saenz de Miera E, Rudy BB. Molecular diversity of K channels. Ann NY Acad Sci 1999; 868: 233–285
- Trimmer JS, Rhodes KJ. Localization of voltage-gated ion channels in mammalian brain. Annu Rev Physiol 2004; 66: 477–519
- Papazian DM. Potassium channels: some assembly required. Neuron 1999; 23: 7–10
- Deutsch C. Potassium channel ontogeny. Annu Rev Physiol 2002; 64: 19–46
- Li D, Takimoto K, Levitan ES. Surface expression of Kv1 channels is governed by a C-terminal motif. J Biol Chem 2000; 275: 11597–11602
- Zhu J, Watanabe I, Gomez B, Thornhill WB. Determinants involved in Kv1 potassium channel folding in the endoplasmic reticulum, glycosylation in the Golgi, and cell surface expression. J Biol Chem 2001; 276: 39419–39427
- Manganas LN, Wang Q, Scannevin RH, Antonucci DE, Rhodes KJ, Trimmer JS. Identification of a trafficking determinant localized to the Kv1 potassium channel pore. PNAS USA 2001; 98: 14055–14059
- Zhu J, Gomez B, Watanabe I, Thornhill WB. Amino acids in the pore region of Kv1 potassium channels dictate cell-surface levels: a possible trafficking code in the Kv1 subfamily. Biochem J 2005; 388: 355–362
- Ashcroft FM. Ion channels and disease. Academic Press, New York 2000
- Zhu J, Watanabe I, Gomez B, Thornhill WB. Trafficking of Kv1.4 potassium channels: interdependence of a pore region determinant and a cytoplasmic C-terminal VXXSL determinant in regulating cell surface trafficking. Biochem J 2003; 375: 761–768
- Eunson LH, Rea R, Zuaberi SM, Youroukos S, Panayiotopoulos CP, Liguori R, Avoni P, McWilliam RC, Spephenson JBP, Hanna MG, Kullmann DM, Spauschus A. Clinical, genetic, and expression studies of mutations in the potassium channel gene KCNA1 reveal new phenotypic variability. Ann Neurol 2001; 48: 647–654
- Stuhmer W, Ruppersberg JP, Schruer KH, Sakmann B, Stocker M, Giese KP, Perschke A, Baumann A, Pongs O. Molecular basis of functional diversity of voltage-gated potassium channels in mammalian brain. EMBO J 1989; 8: 3235–3244
- Bekele-Acruri Z, Matos MF, Manganas L, Strassle BW, Monaghan MM, Rhodes KJ, Trimmer JS. Generation and characterization of subtype-specific monoclonal antibodies to K+ channel alpha- and beta-subunit polypeptides. Neuropharmacology 1996; 35: 851–865
- Okazaki Y, Ohno H, Takase K, Ochiai T, Saito T. Cell surface expression of calnexin, a molecular chaperone in the endoplasmic reticulum. J Biol Chem 2000; 275: 35751–35758
- Long SB, Campbell EB, MacKinnon R. Crystal structure of a mammalian voltage-dependent Shaker family K+ channel. Science 2005; 309: 897–903
- Long SB, Campbell EB, MacKinnon R. Voltage sensor of Kv1.2: structural basis of electromechanical coupling. Science 2005; 309: 903–908
- Rea R, Spauschus A, Eunson LH, Hanna MG, Kullmann DM. Variable K+ channel subunit dysfunction in inherited mutations of KCNA1. J Physiol 2002; 538: 5–23
- Manganas LN, Trimmer JS. Calnexin regulates mammalian Kv1 channel trafficking. Biochem Biophy Res Comm 2004; 322: 577–584
- Manganas LN, Akhtar S, Antonucci DE, Campomanes CR, Dolly JO, Trimmer JS. Episodic ataxia type-1 mutations in the Kv1.1 potassium channel display distinct folding and intracellular trafficking properties. J Biol Chem 2001; 276: 49427–49434