Abstract
Although all of the tendinous structures can be injured, the digital flexor tendons are commonly affected. The goals of tendon surgical reconstruction are to provide a strong anastomosis site, re-establish normal vascularization, restore the tendon microanatomy, functional length and minimize formation of restrictive adhesions that adversely affect the gliding function of the injured tendons. Various techniques of digital flexors tenorrhaphy have been described; either by different suture pattern, prosthetic materials, natural bio-absorbable materials, tendon transplantation and tendon shielding. This review highlights the recent assessment of different techniques used for digital flexors tenorrhaphy in equine and evaluation of the extent and quality of the digital flexor tendons healing and case prognosis after these surgical manoeuvres by ultrasonography, magnetic resonance imaging (MRI), biomechanical properties and histopathological examinations. It could be concluded that the use of preserved bio-absorbable augmentation devices either glycerolized bovine pericardium (GBP) xenograft, allograft and allograft shielding with GBP were a good alternative technique accelerating digital flexor tendons repair with restoring their biomechanical properties. Ultrasonography and MRI have provided accurate methods in monitoring the status of healing progression after tenorrhaphy in equine.
1. Introduction
1.1. Anatomy of the digital flexor tendons
Horses have no muscles below their carpus and tarsus, except the tendons and ligaments, which are attached to the muscles above these joints, acting as levers and pulleys to lift, move, support and flex the hoof, pastern and fetlock joints (Goodship & Birch Citation1996). The superficial digital flexor tendon (SDFT) is located under the skin surface, running along the back of the cannon bone. Proximal to the proximal sesamoid bones, a fibrous ring called manica flexoria is attached to its borders and encircles the deep digital flexor tendon (DDFT), which passes through it. At the distal end of the first phalanx, the distal branches of the SDFT separate and end between the axial and abaxial palmar ligaments of the proximal interphalangeal joint (; Spaulding Citation1984; Watkins Citation1999).
MCIII, third metacarpal bone; SDFT, superficial digital flexor tendon; DDFT, deep digital flexor tendon; SL, suspensory ligament; CDF, common digital flexor.
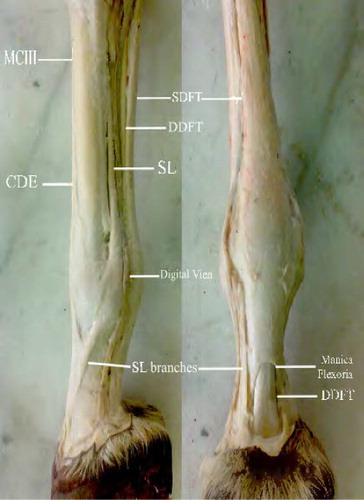
The SDF apparatus contribute to initiate the flexion of the carpal, metacarpo-phalangeal and proximal interphalangeal joints. However, the DDFT is the most effective agent of the distal interphalangeal joint extension. The tension of the DDF apparatus contributes passively to initiate flexion of the carpal, metacarpo-phalangeal and interphalangeal joints (Dyce et al. Citation1996; Dyson & Genovese Citation2003).
The digital flexor tendon sheath is running from the distal third of the metacarpus/metatarsus to the palmar/plantar aspect of the limb. The sheath contained the SDFT and DDFT. The manica flexoria and the palmar/plantar digital annular ligament formed the wall of the sheath (Schultz Citation2004).
2. Treatment of the digital flexor tendons lacerations
Treatment of tendon injuries has stimulated much attention in both medical and veterinary literatures, focusing on various materials used to reconstruct damaged or ruptured tendons. Various techniques have been described that provide forceful tendon anastomosis with minimal gap formation, limited adhesion and preservation of the tendon’s intrinsic blood supply (Crowson et al. Citation2004; El-Shafaey et al. Citation2013).
Kumar et al. (Citation2002) mentioned that, following mechanical injuries of tendons, the major clinical problems encountered during their surgical management are the formation of a gap between the cut ends which prevents bringing together the cut ends by simple tenorrhaphy. Crowson et al. (Citation2004) added that disruption of the anastomotic vascular network within the tendon could presumably cause a disease process similar to that of naturally developed tendonitis. Tenorrhaphy should be accomplished in a manner that causes minimal disruption to the intrinsic vasculature and provides maximal strength.
Taylor et al. (Citation1995) stated that, definitive treatment of digital flexor lacerations might be either conservative or surgical according to the nature of the injury, intended use of the horse and economic constraints. Conservative management is often advocated to salvage horses with flexor tendons lacerations for reproductive purposes. The digits should be supported by the use of special splints, casts or shoes during the initial phases of repair to prevent hyperextension.
Current treatments of tendon lacerations have focused on promoting extrinsic repair, by tenorrhaphy in many repair techniques including different suture pattern, carbon fibres implants and tendon transplantation to minimize the tension placed on the injuried tissue (Foland et al. Citation1991; Kang et al. Citation2012). While, promoting intrinsic repair by stimulating the fibroblast like cells to migrate and/or proliferate into the injuried site, resulting in production and regeneration of new collagen matrix which gradually replaced by a new matrix with more mature collagen matrix. This gradual remodelling improving the collagen fibril alignment parallel to the long axes of the tendon (Reiners et al. Citation2002; Williams & Smith Citation2005).
3. Different techniques of digital flexors tenorrhaphy
Tendon implantation has been recommended in cases of ruptured or severed tendons. The ideal implant should have mechanical and fibrogenic properties similar to those of normal tendon and serves as a scaffold for the migration of fibroblasts derived from normal tenocytes (Valdez-Vazquez et al. Citation1996).
Autogenous and allogenic transplantation of tendon have been performed with varying degrees of success (Gillis Citation1997). Allogenic tendon transplantation has been undertaken experimentally in chicken (Zhang et al. Citation2001). Heterogenic or xenograft tendon transplantation has been applied by using flexor tendons of bovine embryos collected from a slaughterhouse and transplant them in rabbit flexor tendons experimentally (Kumta et al. Citation2000; Dehghani et al. Citation2005).
Eliashar et al. (Citation2001) and Kummer and Iesaka (Citation2005) mentioned that various biomaterials have been used as an augmentation device for the repair and attachment of chicken Achilles tendon. The graft materials tested were Gore-tex soft tissue patch, Graftjacket, bovine pericardium and an experimental graft material from Xylos cooperation. Testing was performed in shear to stimulate forces on a torn tendon repair and pull off to stimulate those on a tendon reattachment to bone. Compared to unaugmented suture; grafts increased suture fixation strength from 10% to 60% in shear and from 0% to 36% in pull off with bovine pericardium graft providing significant improvement in both tests.
The uses of bio-absorbable augmentation devices either xenogenic or allogenic not only accelerate tendon healing but mainly restoring the gliding and biomechanical properties of digital flexors with adhesion restriction (Eliashar et al. Citation2001; Zhang et al. Citation2001). Also, it may have advantageous over non-absorbable materials as they are biocompatible, not potentiate infection, resistant to premature enzyme degeneration, provide exceptional support for the repaired tendon, forming a fibrous bridge for the tendon to regenerate, cheap, available and easy to be applied without any special instruments or techniques (Dehghani et al. Citation2005; longo et al. Citation2010).
The use of banked or preserved bioscaffold materials by the processes of freezing, cryo-preservation or chemical preservation significantly reduces the immunogenicity of the tissue by killing fibroblasts within the graft, make it available and suitable at any time without exhibiting any antigenicity against the host tissue and prevent development of post-operative adhesion (Saini et al. Citation1998). Also, decreasing the surgical time with smaller surgical incision (Kumar et al. Citation2002; Bigham et al. Citation2010).
The implant tenograft should be trimmed probably double to the proper length and size to the gap defect at the recipient tendon. A large graft segment resulted in exuberant connective tissue formation and adhesion at the implantation site with subsequent wound dehiscence (Saini et al. Citation1996; El-Shafaey et al. Citation2012).
El-Shafaey et al. (Citation2013) studied the healing characteristics of SDFT tenorrhaphy in 18 clinically healthy donkeys by using three bioscaffold materials (bovine pericardium xenograft, tendon allograft and allograft shielding by bovine pericardium). All of these bioscaffold materials give good healing properties for SDFT with significant preference for the allograft shielding technique.
3.1. Digital flexors tenorrhaphy with xenograft
Milthrope (Citation1994) mentioned that, collagenous materials, usually of bovine or equine origin, have been popular starting point for the development of xenograft prosthesis for tendon and ligament repair. Xenografts are highly attractive as they carry small risk of infectious disease, do not compromise the patient’s remaining tissues and may have the ‘correct’ structures as the component being replaced. Animal studies, on dog, rabbit and chicken have shown tremendous potential for this use of xenograft material as tendon replacement.
Longo et al. (Citation2010) and El-Shafaey et al. (Citation2013) mentioned that the emerging field of tissue engineering holds the promise to use biomaterials (scaffolds) to provide an alternative for tendon augmentation in cases of tendon deficiencies. Many biomaterials are available for tendon augmentation as bovine pericardium, fascia lata and extracellular matrix.
Rossouw and Villiers (Citation2005) stated that the manufactured form of bovine pericardium which chemically treated by glutaraldehyde is a strong collagenous material that provides a non-stretch bio-integrate for tendon and ligament replacement/augmentation. The membrane is fashioned to meet the particular tendon/ligament requirement.
Nezih et al. (Citation2006) and Longo et al. (Citation2010) mentioned that bovine pericardium can be used for reconstruction of the sheaths successively for prevention of the adhesive bands in flexor tendon surgery. In experimental study, the sheaths of flexor tendons of chicken toe were excised in three groups: group I, primary sheath not repaired; group II, sheath treated with autogenous fascia; and group III, sheath reconstruction with bovine pericardium. The histological evaluations of the three groups on the 3rd and 12th weeks showed less formation of adhesions in group III compared to other groups.
3.2. Digital flexors tenorrhaphy with allograft
The use of allografts appeared as successful as that of autograft, but if fresh allografts are used a donor animal is required each time (Valdez-Vazquez et al. Citation1996; Soliman et al. Citation1999). The efficacy of carbon fibres and plasma preserved tendon allograft for gap repair in the SDFT in 12 crossbred calves. In the carbon fibres group, peritendinous adhesion and acute inflammatory reaction were noticed on the seventh day and gradually reduced. By day 90, the formed neotendon stimulate the picture of normal tendon. While, in the grafted tendon group, there was a normal healing tissue at the junction sites between the host and grafted tendon (Kumar et al. Citation2002).
The use of preserved, ready-to-use (banked) tendon grafts would solve this problem. Tendon grafts stored in plasma at 4°C or by deep freezing have been reported to survive for up to two months. Plasma preserved allogenic tendon grafts were found to be satisfactory for the repair of tendon defects in buffalo calves and donkeys (Saini et al. Citation1996; Saini et al. Citation1998; Bigham et al. Citation2010).
El-Shafaey et al. (Citation2012, Citation2013) examined that the efficacy of preserved tendon allograft for gap repair in the SDFT in 18 donkeys. By the day 90 post-allograft tenorrhaphy there was a normal healing tissue at the junction sites between the host and grafted tendon.
3.3. Digital flexors tenorrhaphy with allograft shielding
Strength and function of autogenic and xenogenic reconstruction of the digital extensor tendons were examined in an ovine model by Rogers et al. (Citation1995) who declared that tendon-graft junctions were formed by either suture augmented with a woven polyester tube (A) or augmented and shielded from surrounding tissues by chemically treated bovine pericardium (S). By the 12th week, both A and S sheep had returned to full range of motion. Mechanical strength of both the autograft-host and xenograft-host repair sites was similar, with a pooled strength of 131 ± 25 N. Similarly, the mid-portion xenograft strengths were constant at approximately 366 ± 97 N. In contrast, mid-portion autograft strengths decreased from 380 ± 110 N to 120 ± 66 N if shielding was omitted.
Saini et al. (Citation1996) and (Citation1998) concluded that tendon healing was more rapid in animals where deep frozen grafts had been used. Experimental tenotomies were performed in the mid-metacarpus of the SDFT and DDFT in 10 donkeys. The frozen tendons that collected from freshly sacrificed donkeys were used to grafting the tendon deficit. Amniotic membrane casing was performed around grafted SDFT and DDFT. The gross and histological observations showed increase in fibroblastic activity with the bundle formation at 2–3 months that appeared near normal.
The use of allograft shielding as a bio-absorbable augmentation devices is the current technique of choice for both SDFT and DDFT tenorrhaphy, as it restores the biomechanical properties of the digital flexor tendons with adhesion restriction. Also, it may have advantageous over non-absorbable materials because it is cheap, available, easy to be applied, biocompatible and capable to provide exceptional support for the repaired tendon (Dehghani et al. Citation2005; Longo et al. Citation2010; El-Shafaey et al. Citation2012).
The allograft shielding of both SDFT and DDFT provides a grafting structure that enhancing the surgical repair of the tendon defect. There was neither clinical evidence of gross infection nor tissue reaction in the donkeys treated with the natural grafts. Although there were some scaring at the tendon junction, the grafts remained relatively free of thick adhesions to their sheaths throughout the entire healing process (Valdez-Vazquez et al. Citation1996; Reiners et al. Citation2002).
4. Pathophysiology of tendon healing
Tendons injury follows a sequence of repair similar to that of other vascularized connective tissues. This sequence consists of four overlapping phases: injury, inflammation (haemostasis), repair (proliferation/fibroplasia) and remodelling phase (consolidation stage and maturation stage). Numerous factors influence tendon healing including: the nature of tendon injury, the tendon injured, the type of tissue surrounding the tendon at the site of injury and the treatment (Goodship et al. Citation1994).
Most tendon injuries healed by extrinsic and/or intrinsic healing. Extrinsic healing predominates with the majority of wound constituents derived from the vascular elements of the extratendinous tissues and relies on adhesions formation; disrupt tendon gliding, infiltration of inflammatory cells and fibroblasts and characterized initially by type III collagen. However, intrinsic tendon healing may provide better quality of repair in injured tendons and preserve the normal gliding mechanism as type I collagen is likely the product of more differentiated tenocytes and proliferation of epitenon and endotenon with intratendinous vascular supply (Kakar et al. Citation1998; Masuda et al. Citation2002).
5. Evaluation of the digital flexor tendons repair
5.1. The clinical index score
The clinical index score can provide useful indications about the tendon healing and the efficiency of scaffold material (). Restoring of normal weight bearing, without apparent lameness and tissue reaction are the best indicators of clinical recovery (Adams & Santschi Citation2000).
Table 1. The clinical index score for subjective assessment of clinical parameters in animal subjected to digital flexors tenorrhaphy at 45 and 90 days post-operatively.
5.2. Ultrasonography
Ultrasonography is widely used to evaluate the tendon lesions severity and progression. While this technique is considered one of the most accurate diagnostic tools in the early stage, advanced healing lesions tend to present normal echogenicity although the tendon has not yet completely recovered (Denoix et al. Citation2005; Whitcomb, Citation2009). In particular, the mechanical properties of tendon play a determinant role in its ability to resume work (Vergari et al. Citation2012).
Wood et al. (Citation1994) and El-Shafaey et al. (Citation2013) mentioned that, the application of ultrasonographic scan for the diagnosis of flexor tendon injuries in horses has provided a safe, non-invasive objective means of for assessments of tendon shape, echogenicity, oedema and fibres alignment as indicators for the stage of tendon healing () and comparisons of ultrasonographic and pathological findings that accurately reflect the extent of lesions in horse. Also, allow the patient rehabilitation programme to be tailored to tendon healing as evidenced by direct visualization of tendon architecture.
Table 2. The ultrasonographic, gross and histomorphological parameters for subjective assessment of digital flexors tenorrhaphy in donkeys at each point time during healing.
Normally the conventional way of ultrasonographic imaging of the horse’s limb ligaments and tendons is underweight bearing situation which making easier to determine the tendon size. While scanning the non-weight bearing limb longitudinally with flexing and extending the fetlock to watch the dynamics of the tendons, detecting small amount of fluid in tendon sheath and freely movement of tendon or if there are fibrous adhesions (Rantanen Citation1993; Reef Citation1998). For accurate ultrasonographic examinations of the tendon, two sonographic views are performed: one longitudinal, to observe the normal alignment of the tendon fibres and the other one is transverse, perpendicular to the mid-point of each area, to obtain structural dimensions. Ultrasonographic appearance of normal tendons characterized by intense linear echoes bands separated with intermittent lines exhibiting the acoustic impedance interface between the tendons (Cuesta et al. Citation1995; Susan, Citation2005; El-Shafaey et al. Citation2013).
Ultrasonographic scan at the upper and middle palmar metacarpus identified the SDFT, DDFT, inferior check ligament (ICL) and suspensory ligament (SL). The SDFT and DDFT have similar echogenicity, although the DDFT is sometimes more echogenic for reasons yet to be determined (Majid Citation2008). While the ultrasonographic evaluation of injured digital flexor tendons beings below the level of the carpal or tarsal joint and extend to the proximal sesamoid bones. Normal values for the cross-sectional area and the ratio of the superficial to the deep flexor tendon size, fibre pattern, shape, margination, position and as well as their echogenicity have been established ultrasonographically (Smith et al. Citation1994; Davis et al. Citation2006).
The alteration in tendon echogenicity reflects changes in the cellular and extracellular composition of injured tendons along with the repair tissue, it ranged from a barely perceptible decrease in echogenicity with mild loss of fibre pattern as in mild tendonitis, to complete disruption of fibre pattern seen with fibre rupture accompanied with haemorrhage or with serious fluid accumulation and oedema (Denoix et al. Citation2005; Duenwald et al. Citation2011).
The presence of an echoic shadow between the skin and SDFT was noted in cases with marked fibrosis of the paratenon which was less echogenic than the tendon. Adhesion between SDFT and DDFT produced poor definition of the border between these two structures (Bosch et al. Citation2011).
The ultrasonographic examination of ruptured digital flexor tendons was characterized by presence of the two echogenic ruptured tendinous segments and the cut area appeared anechoic in between with peritendinous an echoic fluid. This core lesion was occupying about 65% of SDFT in cross section, while the longitudinal view showed lack of fibre arrangement. This fibres tearing could be multiple, showed hypoechoic to an echoic area with slight loss of fibres alignment at the dorsal aspect and centre of SDFT (Seliem et al. Citation2002; El-Husseiny Citation2005; Gillis Citation2007). While the interval between tendon fragments in partial and complete tendon rupture might be filled with haemorrhage, serous effusion or oedema yielding an echo pattern of increased and decreased echogenicity. Serous fluid and oedema are hypo-to-anechoic.
The resolution of the initial peritendinous and intratendinous oedema, a more accurate assessment of the lesion may be often made. Marked ultrasonographic improvement will be recognized in the initial 4–8 weeks, with some degree of echogenicity corresponding to granulation tissue. As healing progress, echogenicity gradually increases possibly corresponding to increasing collagen and fluid content (Ali et al. Citation1992; Nicoll et al. Citation1992).
After repair of an acute tendon laceration or rupture, the tendon is often markedly enlarged and may have ill-defined contours with inhomogeneous echoes at the repair site within the tendon. The scar in the overlying skin can result in shadowing (Marr et al. Citation1993).
At comparable ultrasonographic evaluation of the SDFT repair in donkeys by three bio-absorbable implants at each point time of the experiment, there was a significant difference in echogenicity (p < 0.0039), cross-sectional area (p < 0.0490) and fibres alignment (p < 0.0077) in the allograft shielding group; which was confirmed also by the median and range analysis for each (El-Shafaey et al. Citation2013). The negative correlations observed between echogenicity and cross-sectional area in all grafted tendons can be related to the fact that both echogenicity decreased and cross-sectional area increased by initial fibres disruption and random orientation (loss in collagen density and disorganization of acoustic interfaces) which improved as healing progress with collagen deposition and tendon fibres alignment (Denoix et al. Citation2005).
5.3. Magnetic resonance imaging
MRI provides the opportunity to evaluate structures within the limbs that were previously unable to be visualized in live horses. MRI has also enabled accurate and useful information of osseous and soft tissue structures visible with radiography and ultrasonography to be evaluated in greater anatomic and physiological details (Kraft & Gavin Citation2001). It enables the generation of high resolution and thin cross-sectional images of the extremities. These images can be acquired in any desired orientation but commonly acquired in transverse, sagittal and dorsal planes. The number and thickness of the slices can be varied to cover a region of interest or focus on a specific structure (Tucker & Sande Citation2001). It is necessary to use several sequences in multiple image planes to accurately identify normal and pathological conditions (Ferrell et al. Citation2002).
The pathological changes in tendons and ligaments are seen on MRI as changes in size, shape and/or signal intensity of the affected structures. All tendons and ligaments have individual characteristics and variations in normal appearance. The tendinous and ligamentous structures in the metacarpal/metatarsal regions are best to be evaluated in the transverse plan; as the sagittal and dorsal images are of little value other than to use as a reference and source for comparison and lesion confirmation. The SDFT forms a crescent shape when viewed transversely with a concave dorsal surface and convex palmar/plantar surface while the DDFT cross-sectional shape is more rounded (Dyson & Murray Citation2007; Zubrod & Barrett Citation2007).
The acutely injured tendons and ligaments will have increased fluid content which results in increased signal intensity. As the injured tendon or ligament begins to heal, the amount of fluid decreases and early scar formation begins. At the intermediate stage post-injury, there is an increase from normal low signal intensity on all sequences than at the acute stage (Murray Citation2011). However, chronic scaring usually has low to intermediate signal intensity. In some cases of chronic scaring, the low signal intensity is the same as that of a normal tendon or ligament but the irregulatory of the shape is indicative of chronic disease (Werpy Citation2006; Zubrod & Barrett Citation2007).
MRI for SDFT tenorrhaphy by three bioscaffold augmentation devices in donkeys provides the opportunity to accurately evaluate different stages of tendon repair. MRI in tendon pathology demonstrated a very high signal intensity of acute tendon lesions that then decreased as the tendon healed (El-Shafaey et al. Citation2013).
A collaboration between MRI and ultrasonography provide excellent tools for objective assessment of the effectiveness of the digital flexor tenorrhaphy with different tendon grafts in horses, monitoring of the repair trajectory as it provide intravital method of investigation, allow more adequate treatment selection and timely adjustment of therapies or rehabilitation procedures and it may even provide a basis for better early prognostication (Zhang et al. Citation2001; Werpy Citation2006; Zubrod & Barrett Citation2007). El-Shafaey et al. (Citation2012) were the first use a combination of MRI and ultrasonography for evaluating the healing characteristics of digital flexors tenorrhaphy in donkeys with three bioscaffold augmentation devices at different times post-operatively.
5.4. Histopathology
Each tendon specimen was histomorphologically analysed qualitatively using the following parameters: vascularization, cellularity, collagen fibres alignment, inflammatory cells and granulation tissues as shown in . The graft function as an organizer of tendon healing is known to increase the rate of maturation of tendon repair in comparison to spontaneous healing or synthetic materials repair (Kumar et al. Citation2002). Natural bioscaffold augmentation devices yielded histologically superior healing by improving fibroblast and collagen fibre orientation and enhancing vascularity, which serve as a barrier to the formation of extrinsic adhesion, and act as a guide for remodelling tendon and improving tendon gliding and movement biomechanics (Kummer & Iesaka Citation2005).
Histopathological investigation in the SDFT reconstruction in donkeys, gradual improvement with time was obtained which initially appeared in the form of randomly distributed active fibroblasts as well as immature collagen fibres at 45 days post-operatively and then become well organized with parallel wavy bundles of densely packed, collagen fibres at 90 days post-operatively. These healing properties were confirmed with the Masson’s trichrome stain, where the mature wavy collagen bundles of the newly formed tendon stained blue (Bruna et al. Citation2013; El-Shafaey et al. Citation2013) and support the suggestion that the graft materials used in the digital flexors tenorrhaphy may act as a connecting device providing flexor support until complete tendon healing. Abundant fibrous tissue and vascular growth present in and around the graft bed form a fibrous bridge for tendon regeneration and a scaffold for laying down fibroblasts with newly formed collagen relatively as normal ones (Chvapil et al. Citation2004; Demirkan et al. Citation2004; Rossouw & Villiers Citation2005; El-Shafaey et al. Citation2012).
5.5. Biomechanical properties of the digital flexor tendons
The biomechanical parameters of the equine digital flexor tendons involved the ultimate tensile stress, ultimate tensile strain and the modulus elasticity. The mechanical factors are important in the aetiology of tendon and ligament lesion. The distribution of loads among several tendons in the equine limb has been studied extensively in vitro and in vivo (James et al. Citation2008; Vergari et al. Citation2011; Barrett et al. Citation2014).
The tensile strain was defined as the change in length of a substance normalized by the original length (Riemersa et al. Citation1996). The failure stress is fundamentally simple to measure, where a constant load is applied to a tissue and the progressive time dependant elongation is measured (Smith & Webbon Citation1996). However, the load to failure represents the continuous loading of a tendon sample till complete rupture (Woo et al. Citation1997; Smith et al. Citation2011).
Tensile properties of tendons and ligaments form the core of most biomechanical investigations of tendons and ligaments. Tendon’s repair can be strong or weak, rough or smooth and those aspects can be separately controlled (Momose et al. Citation2000). The repaired tendons during the early stages of healing must be protected from full weight bearing through some form of external cooptation, regardless of the method and material used to reconstruct the damaged tendon until the collagenous scar has developed adequate tensile strength to support weight bearing function (Lin et al. Citation2004; Dehghani & Varzandian Citation2007).
Valdez-Vazquez et al. (Citation1996) found that in vitro data for healing of SDFT revealed load failure ranged from less than 150 kg at 6th week to 600 kg at 9–12th weeks and up to 700 kg at 24th week. However, load failure of the DDFT at 6th week is slightly more than 100 kg, by 12th week it is about 260 kg and by 24th week, it is 575 kg.
At comparable limb loading, the results of biomechanical testing in the proved no significant differences between control and treatment groups except the property failure stress (p < 0.05). Moreover, in all treated groups, the failure stress was found to increase significantly with time with significance difference in the stress values in the allograft shielding group (87.6 ± 3.7 N/mm2). The difference between tensile strength of control and test groups was because of full loading of the tendon and incomplete collagen fibrils alignment in 45–90 days post-operatively, as remodelling of tendon needs at least 3–6 months to complete (El-Shafaey et al. Citation2012).
6. Conclusions
The use of preserved bio-absorbable augmentation devices either GBP xenograft, allograft and allograft shielding with GBP were a good alternative technique accelerating digital flexor tendons repair with restoring their biomechanical properties. The collaboration between MRI and ultrasonography has initiated a new paradigm in monitoring the healing progression of digital flexors tenorrhaphy as it provide intravital method of investigation, allow more adequate treatment selection and timely adjustment of therapies or rehabilitation procedures and it may even provide a basis for better early prognostication.
Disclosure statement
No potential conflict of interest was reported by the authors.
References
- Adams AB, Santschi EM. 2000. Management of congenital and acquired flexural limb deformities. AAEP Proc. 46:117–125.
- Ali EL, Makady FM, Ahmed IH, Seliem MA. 1992. Ultrasonographic diagnosis of tendonitis and tenosynovitis in donkeys. Assiut Vet Med J. 26:230–240.
- Barrett E, Amelia S. Munsterman, R. Hanson R, Jackson RL. 2014. Biomechanical testing of a novel tendon implant device for the repair of equine flexor tendon lacerations. Vet Surg. 43:685–690.
- Bigham AS, Shadkhast HN, Shafiei Z. 2010. Fresh autogenous and allogenous graft in rabbit model. Comp Clin Pathol. 20:109–113.
- Bosch G, Weeren P, Barneveld A, Schie H. 2011. Computerised analysis of standardized ultrasonographic images to monitor the repair of surgically created core lesions in equine superficial digital flexor tendons following treatment with intratendinous platelet rich plasma or placebo. Vet J. 187:92–98. 10.1016/j.tvjl.2009.10.014
- Bruna M, Maria V, Luiza N, Marlene I. 2013. Immunohistochemistry of factor viii, histology and morphometry in equine tendon treated with platelet-rich plasma. Rev Bras Med Vet. 35:169–184.
- Chvapil M, Gibeault D, Wang T. 2004. Use of chemically purified and cross-linked bovine pericardium as a ligament substitute. J Biomed Mater Res. 21:1383–1393.
- Crowson CL, Jann, HW, Stein LE, Claypool LP, Moll HD, Blaik MA. 2004. Quantitative effect of tenorrhaphy on intrinsic vasculature of the equine superficial digital flexor tendon. AJVR. 65:279–282.10.2460/ajvr.2004.65.279
- Cuesta I, Riber C, Pinedo M, Gota, JA, Castejon F. 1995. Ultrasononographic measurement of palmer metacarpal tendon and ligament structures in the horse. Vet Radiol Ultrasound. 36:131–136.10.1111/j.1740-8261.1995.tb00232.x
- Davis CS, Ely ER, Verheyen KL, Price JC.2006. The value of ultrasonography in monitoring tendon health in racehorses in training. 13th ESVOT Congress; Sep 7–10; Munich (Germany).
- Dehghani SN, Tadjalli M, Akbari A. 2005. Transplantation of bovine fetal tendon in rabbit flexor tendon. Vet Arch. 75:153–158.
- Dehghani SN, Varzandian S. 2007. Biomechanical study of repair of tendon gap by bovine fetal tendon transplant in horse. J Anim Vet Adv. 6:1097–1100.
- Demirkan I, Atalan G, Cihan M, Sozmen, M. 2004. Replacement of ruptured Achilles tendon by fascia lata grafting. Veter Cerra Dergisi. 10:21–26.
- Denoix CN, Ruel Y, Dardillat C, Jerbi H, Sanaa M, Collobert-Laugier C, Ribot X, Denoix J-M, Pourcelot P. 2005. Correlations between mean echogenicity and material properties of normal and diseased equine superficial digital flexor tendons: an in vitro segmental approach. J Biomechanics. 38:2212–2220. 10.1016/j.jbiomech.2004.09.026
- Duenwald S, Kobayashi H, Frisch K, Lakes R, Vanderby, R. 2011. Ultrasound echo is related to stress and strain in tendon. J Biomech. 44:424–429. 10.1016/j.jbiomech.2010.09.033
- Dyce KM, Sack WO, Wensing GI. 1996. Textbook of veterinary anatomy. 2nd ed. Philadelphia (PA): W.B. Saunders Co; p. 675–688.
- Dyson S, Genovese R 2003 The suspensory apparatus. In: Ross M, Dyson S, editors. Diagnosis and management of lameness in the horse. St Louis (MO): WB Saunders Co; p. 654–666
- Dyson S, Murray R. 2007. Magnetic resonance imaging of equine foot. Clin Tech Equine Pract. 6:46–61. 10.1053/j.ctep.2006.11.005
- El-Husseiny IN. 2005. The distal limb affections in equine with special reference to the diagnostic methods. J Egypt Vet Med Assoc. 65:57–77.
- Eliashar E, Schramme MC, Schumacher J, Smith RK, Ikada, Y.2001. Use of a bioabsorbable implant for the repair of severed digital flexor tendons in four horses. Vet Rec. 506–509. 10.1136/vr.148.16.506
- El-Shafaey EA, Karrouf GI, Zaghloul AE. 2012. A comparative study evaluating three bioscaffold augmentation devices used for superficial digital flexor tenorrhaphy in donkeys (Equus asinus) by magnetic resonance imaging and ultrasonography. J Equine Vet Sci. 32:728–739. 10.1016/j.jevs.2012.02.019
- El-Shafaey EA, Karrouf GI, Zaghloul AE. 2013. Clinical and biomechanical evaluation of three bioscaffold augmentation devices used for superficial digital flexor tenorrhaphy in donkeys (Equus asinus): an experimental study. J Adv Res. 4:103–113. 10.1016/j.jare.2012.02.001
- Ferrell E, Gavin P, Tucker R, Sellon DC, Hikes M. 2002. Magnetic resonance for evaluation of neurologic disease in 12 horses. Vet Rad Ultrasound. 43:510–516. 10.1111/j.1740-8261.2002.tb01041.x
- Foland JW, Trotter GW, Stashak TS, Mcllwraith CW, Turner AS, Aanes WA. 1991. Traumatic injuries involving tendons of the distal limb in horses: a retrospective study of 55 cases. Equine Vet J. 23:422–425. 10.1111/j.2042-3306.1991.tb03754.x
- Gillis CL. 1997. Rehabilitation of tendon and ligaments injuries. Proc Am Ass Equine Pract. 43:306–309.
- Gillis C.2007. Ultrasonography for monitoring healing and rehabilitation. Clin Tech Equine Pract. 6:174–178. 10.1053/j.ctep.2007.08.003
- Goodship AE, Birch HL. 1996. The pathophysiology of the flexor tendons in the equine athlete. In: Proceedings Dubai international equine symposium. Rantanen NW, Hauser ML, editors. Bonsall (CA): Neyenesch Printers Inc.; p. 83–107.
- Goodship AE, Birch HL, Wilson AM. 1994. The pathobiology and repair of tendon and ligament injury. Vet Clin North Am Equine Pract. 10:323.
- James R, Kesturu G, Balian G, Chhabra B. 2008. Tendon: biology, biomechanics, repair, growth factors, and evolving treatment options. J Hand Surg. 33:102–112. 10.1016/j.jhsa.2007.09.007
- Kakar S, Khan U, McGrouther DA. 1998. Differential cellular responses within the rabbit tendon unit following tendon injury. J Hand Surg. 23:627–632. 10.1016/S0266-7681(98)80017-X
- Kang H, Lee D, Kim J, Ki S, Roh S, Yang J. 2012. Flexor tenorrhaphy using absorbable suture materials. Arch Plast Surg. 39:397–403. 10.5999/aps.2012.39.4.397
- Kraft S, Gavin P. 2001. Physical principles and techniques considerations for equine computed tomography and magnetic resonance imaging. Vet Clin North Am. 17:115–130.
- Kumar N, Sharma AK, Kumar S. 2002. Carbon fibers and plasma-preserved tendon allografts for gap repair of flexor tendon in bovines: gross, microscopic and scanning electron microscopic observations. JAVMA. 49:269–276.
- Kummer J, Iesaka K. 2005. Role of graft materials in suture augmentation for tendon repairs and reattachment. J Biomed Mater Res Part B: Appl Biomater. 74:789–791. 10.1002/jbm.b.30296
- Kumta SM, Panozzo A, Leung PC, Fong SL. 2000. Repair of muscle and musclotendinous junction injuries with an autogenous fascial patch. Arch Orthop Trauma Surg. 120:198–200. 10.1007/s004020050043
- Lin TW, Cardenas L, Soslowsky LK. 2004. Biomechanics of tendon injuries and repair. J Biomech. 37:865–877. 10.1016/j.jbiomech.2003.11.005
- Longo U, Lamberti A, Maffulli N, Denaro V. 2010. Tendon augmentation graft: a systematic. Brit Med Bull. 10:1093.
- Majid M. 2008. Principles of ultrasonography of tendons and ligaments in the horse. IJVS. 72–81.
- Marr CM, Millan M, Boyd JS, Wright NC, Murray M. 1993. Ultrasonography and histopathological findings in equine in SDFT injury. Equine Vet. J. 25:23–29. 10.1007/s007760200118
- Masuda K, Ishii S, Ito K, Kuboki Y. 2002. Biochemical analysis of collagen in adhesive tissues formed after digital flexor tendon injuries. J Orthop Sci. 7:665–671. 10.1007/s007760200118
- Milthrope K. 1994. Xenografts for tendon and ligament repair. Biomaterials. 15: 745–752.
- Momose T, Amadio PC, Zhao C, Zobitz ME, An K-N. 2000. The effect of knot location, suture material, and suture size on the gliding resistance of flexor tendons. J Biomed Mater Res. 53:806–811. 10.1002/1097-4636(2000)53:6<806::AID-JBM23>3.0.CO;2-P
- Murray R. 2011. Equine MRI. Oxford: Wiley-Blackwell Publishing Ltd.
- Nezih S, Afsin U, Ugur K, Onder K. 2006. Prevention of tendon adhesions by the reconstruction of the tendon sheath with solvent dehydrated bovine pericardium: an experimental study. J Trauma: Inj Infect Crit Care. 61:1467–1472. 10.1097/01.ta.0000196344.61787.62
- Nicoll RG, Wood AK, Rothwell TL. 1992. Ultrasonographical and pathological studies of the equine SDFT; initial observations including tissue characterization by analysis of image gray scale in a Thoroughbreds gelding. Equine Vet J. 24:318–320. 10.1111/j.2042-3306.1992.tb02844.x
- Rantanen N. 1993. Tendon imaging using flexion and extension. Diagnosting ultrasound principles and techniques. Ultrasound Update. 13:683–684.
- Reef DV. 1998. Textbook of equine diagnostic ultrasound. Philadelphia (PA): W. B. Saunders Company.
- Reiners SR, Jann HW, Stein LE, Good JK, Claypool PL. 2002. An evaluation of two autologous tendon grafting techniques in ponies. Vet Surg. 31:155–166. 10.1053/jvet.2002.31048
- Riemersa DJ, Bogert AJ, Janssen MO, Schamhardt HC. 1996. Tendon strain in the forelimbs as a function gait and ground characteristic and in vitro limb loading in ponies. Equin Vet J. 28:133–138. 10.1111/j.2042-3306.1996.tb01605.x
- Rogers J, Milthrope K, Schindhelm K, Howlett R. 1995. Shielding of augmented tendon-tendon repair. Biomaterials. 16:803–807.
- Rossouw P, Villiers M. 2005. Bovine pericardial ligament and tendon augmentation: a new and revolutionary ligament. J Bone Joint Surg. 87:277.
- Saini NS, Mirakhur KK, Roy KS.1996. Gross and histomorphological observations following homologous deep frozen tendon grafting in equines. J Equine Vet Sci. 16:524–533. 10.1016/S0737-0806(96)80009-8
- Saini NS, Mirakhur KK, Singh M, and Roy KS. 1998. Homologous plasma stored tendon grafting in equids. Gross and histomorphological observations. Res Vet Sci. 64:85–90. 10.1016/S0034-5288(98)90000-5
- Schultz L. 2004. Howell equine handbook of tendon and ligament injuries. Hoboken (NJ): Wiley Publishing, Inc.
- Seliem MA, El-Sayad, GA, Kamel AM .2002. Ultrasonographic diagnosis of some tendons and ligament affections in equine limbs. J Egypt Vet Assoc. 62:323–338.
- Smith R, David JM, Robert ED, Guy D. 2011. An ex vivo biomechanical study comparing strength characteristics of a new technique with the three-loop pulley for equine tenorrhaphy. Lester Vet Surg. 40:768–773.
- Smith KW, Jones R, Webbon PM. 1994. The cross sectional areas of normal equine flexor tendons determined ultrasonographically. Equin Vet J. 26:460. 10.1111/j.2042-3306.1994.tb04050.x
- Smith KW, Webbon PM. 1996. The physiology of normal tendon ligament. Proceeding of the Dubai international equine symposium, Bonsall (CA): MR Rantanen Design.
- Soliman AS, El-Husseiny IN, Easa MS 1999. Tenorrhaphy of experimentally transected superficial digital flexor tendon in donkeys. World Vet. Congress WVA; Lyon (France).
- Spaulding K. 1984. Ultrasonic anatomy of the tendons and ligaments in the distal metacarpal-metatarsal region of the equine limb. Vet Rad. 25:155–166. 10.1111/j.1740-8261.1984.tb02136.x
- Susan LM. 2005. Is there a role for ultrasound and electrical stimulation following injury to tendon and nerve? J Hand Ther. 18:292–296. 10.1197/j.jht.2005.02.013
- Taylor DS, Pascoe JR, Meagher DM, Honnas CM. 1995. Digital flexor tendon lacerations in horses: 50 cases (1985–1990). JAVMA. 206:342.
- Tucker R, Sande R. 2001. Computed tomography and magnetic resonance imaging in equine musculoskeletal conditions. Vet Clin North Am. 17:145–157.
- Valdez-Vazquez MA, McClure JR, Oliver JL. 1996. Evaluation of an autologous tendon graft repair method for gap healing of the deep digital flexortendon in horses. Vet Surg. 25:342. 10.1111/j.1532-950X.1996.tb01423.x
- Vergari C, Pourcelot P, Holden L, Ravary-Plumioen B. 2011. True stress and Poisson's ratio of tendons during loading. J Biomech. 44:719–724. 10.1016/j.jbiomech.2011.10.016
- Vergari C, Pourcelot P, Ravary-Plumioen B, Dupays A, Jacquet S, Audigié F, Denoix J-M, Laugier P, Mitton D, Crevier-Denoix N. 2012. Axial speed of sound for the monitoring of injured equine tendons: a preliminary study. J Biomech. 45:53–58. 10.1016/j.jbiomech.2011.10.016
- Watkins PJ 1999. Tendon and ligament biology in: equines surgery. Auer, AJ and Stick, AJ. 2nd ed. Philadelphia (PA): W.B. Saunders Co; p. 704–710.
- Werpy N. 2006. Magnetic resonance imaging for diagnosis of tendon and ligament injury. Introduction, in proceedings of the American colleague of veterinary surgeon. Washington (DC): Elsevier Health Sciences; p. 143–146.
- Whitcomb M. 2009. Ultrasonographic evaluation of the distal extremity. J Equine Vet Sci. 29:47–59. 10.1016/j.jevs.2008.11.010
- Williams C, Smith TH. 2005. A-Cell therapy offers renewed hope for horses incurring tendon and ligament injuries. Horse Care; p. 134–137.
- Woo SL, Livsay G, Runco T. 1997. Structure and function of tendon and ligaments. In: Mow V, Hayes W, editors. Philadelphia (PA): Basic Orthopedic Biomechanics; p. 209–252.
- Wood KW, Sehgal CM, Reef VB. 1994. Three-dimensional sonographic imaging of theequine superficial digital flexor tendon. Am J Vet Res. 55:1505–1508.
- Zhang YL, Wang SI, Gao XS. 2001. Experimental study of allogenic tendon with sheet grafting in chicken. Wai Keza Zhi. 15:92–95.
- Zubrod C, Barrett M. 2007. Magnetic resonance imaging of tendon and ligament injuries. Clin Tech Equin Pract. 6:217–229. 10.1053/j.ctep.2007.08.008