ABSTRACT
Salinity tolerance of juvenile obscure puffer Takifuge obscurus was investigated at salinities of 0, 8, 16, 24 and 32 parts per thousand (ppt). In consequence, the juveniles of obscure puffer were able to survive and grow well at salinities from freshwater (0 ppt) to 32 ppt. In addition, the effects of salinity on serum osmolality, morphology of chloride cells in gill filaments, and branchial NKT (Na+/K+-ATPase) activity and expression were also assessed. At the beginning of the trial (day 15), the serum osmolality, NKT activity and expression in fish reared at salinities all increased, followed by decreases in serum osmolality and NKT activity, and slight change in NKT expression. Besides, the chloride cells became larger and denser with increasing salinity at the end of the trial. Our results demonstrate that the juveniles can osmoregulate in a wide range of salinity and provide indirect evidence that chloride cells and NKT in gill are responsible for excretion of excess salt from body in hyperosmotic environments. The ability for juvenile obscure puffer to maintain homeostasis and grow well in a wide range of salinities, from 0 to 32 ppt, supports the hypothesis that the juveniles of obscure puffer are suitable for both freshwater and seawater aquaculture.
1. Introduction
Obscure puffer Takifugu obscurus (Tetraodontiformes, Tetraodontidae) is mainly distributed in the middle and lower reaches of the Yangtze River, and in the inshore waters of the Yellow Sea, East China Sea and Bohai Bay (Yang & Chen Citation2004). It has been the most important puffer fish cultured in China because of its quality fish meat and high market value. The obscure puffer meat is tender, nutrient rich and extremely delicious, which contains many essential vitamins, minerals and unsaturated fatty acid. The meat of this fish is well known for its delicious flavours in China. Much attention has been paid to increase the production of obscure puffer through increasing body size and improving meat quality in the past ten years. Thus, the aquaculture production of it has been developed significantly, which plays an important role in meeting the rising demand.
Obscure puffer is an anadromous species. The obscure puffer confronts with drastic salinity changes in the natural environment during spawning movement. They migrate to the Yangtze River to spawn in March every year, and the larvae live in the Yangtze River and migrate to the sea next year (Yang & Chen Citation2005). Thus, the species has the ability to live in both freshwater and saltwater. However, it is usually cultured in freshwater in China, and a considerable amount of freshwater consumption is used during culture process, which increases the cost and restricts further development of obscure puffer. Thus, if the obscure puffer could be cultured in salinity brackish or even in seawater, a lot of freshwater would be saved.
In recent years, the acclimatory response of obscure puffer to salinity regimes has been attracting much interest. Yang and Chen (Citation2006) studied the effects of salinity on the embryos of this species and indicated that the embryos can tolerate a salinity of up to 8 ppt. Additionally, Shi et al. (Citation2010) reported that the optimal and suitable salinity ranges of larviculture were 8–10 ppt and 0–20 ppt. It is well established that obscure puffer embryos and larvae possess natural ability to adapt to salinity changes (Yang et al. Citation2010; Li et al. Citation2011). As ontogenetic development, obscure puffer is expected to have better ability to regulate osmolality and adapt to high salinities (Zhang et al. Citation2010). Thus, it is hypothesized that the juvenile obscure puffer could tolerate higher salinity. However, no information is available on the salinity tolerance in this stage. Therefore, we further investigated the effects of salinity on the survival and growth of juvenile obscure puffer. The present experiment had two goals. One was to determine optimal salinity for juvenile obscure puffer, and the second was to have a preliminary understanding of its osmo-regulatory mechanism.
2. Materials and methods
2.1. Fish
Fish were obtained from Jiangsu Marine Fisheries Research Institute. The fish were spawned in April 2012 and the experiment commenced in September 2012, when the fish were about 160 days old with a mean weight of 30 g. These fish were in the early juvenile developmental stage. The study was conducted at the Fisheries Research Institute of Zhoushan. The experiment concluded in November after a period of 60 days.
2.2. Experimental design
To obtain the required salinities, different proportions of the commercially available sea salt were mixed with fresh water. Salinities used for maintenance were 0 (freshwater control), 8, 16, 24 and 32 ppt (Yang & Chen Citation2006). Four hundred and fifty puffer juveniles were used in this experiment. Thirty fish were randomly stocked in about 2-m-diameter circular tanks (volume is about 4.0 m3). Three tanks of fish were assigned to each of the five salinities.
Food was provided in excess for 2 h daily (08:30–09:30 and 14:00–15:00). Twenty per cent of the water in each tank was replaced with fresh medium daily. Dead fish were removed and counted every day, and survival was calculated as the percentage of survivors. Three puffer juveniles in each tank were sampled every 15 days. All fish were anaesthetized before sampling. The fish were dried lightly with paper towels and then weighed individually. After weighing, the fish blood samples were first collected and then gill and kidney samples were cut out from every fish.
2.3. Serum osmolality measurement
The blood samples were centrifuged for 5 min at 3000 rpm, and then the serum samples (the supernatant) were collected. The serum osmolality was determined with a vapour pressure osmometer (Wescor Model 5520; Wescor Inc., Logan, UT, USA).
2.4. Scanning electron microscope observation
Gill filaments were excised and fixed in 2.5% (vol/vol) glutaraldehyde in PBS for 2 h at 4°C. The samples were rinsed with phosphate buffer solution (PBS) followed by a secondary fixation with osmic acid. Then the samples were dehydrated in ethanol and dried using vacuum drying apparatus. Dried samples were mounted on specimen stubs and coated with platinum palladium, before examination using a scanning electron microscopy (JEOL-6380LV; Kabuskiki Kaisha, Tokyo, Japan). This process was completed with the help of Shanghai Showbio Biotech Co., Ltd (Shanghai, China).
2.5. Na+/K+-ATPase activity
Na+/K+-ATPase (NKA) activity was measured by Absorbance Microplate Readers (BioTek Epoch ELX800; BioTek Inc., USA) with a NKA activity Kit obtained from Nanjing Jiancheng Bioengineering Institute (Nanjing, China). The enzyme assay was performed according to the manufacturer’s investigational package.
2.6. Real-time quantitative PCR
The gill samples were washed with ice-cold PBS and ground using liquid nitrogen. Total RNA was extracted using an RNATMiso PLUS Kit (Takara Biotechnology, Dalian, China). The RNA was reverse transcribed into complementary DNA (cDNA) using PrimeScript 2st Strand cDNA Synthesis Kit (Takara Biotechnology, Dalian, China). Real-time cDNA amplification was performed using the SYBR Premix EX TaqTM (Takara Biotechnology, Dalian, China). cDNA was then diluted 1:10 in RNase-free, diethyl pyrocarbonate-treated water. shows the primers used for real-time quantitative RT-PCR. Amplification was carried out in 25.0 μl end volume containing 2 μl cDNA, 12.5 μl 2 × SYBR Premix EX TaqTM, 0.5 μl ROX Reference Dye II, 9 μl dH2O, and 10 μM of each primer. The amplification reactions were performed under the following PCR conditions: (i) one cycle at 95°C for 30 s, (ii) amplification including 40 cycles of 95°C for 10 s, 60°C for 20 s, and (iii) 95°C for 30 s, 55°C for 1 min, 95°C for 30 s. The data represent mean values obtained in three independent experiments performed in duplicate (Gao et al. Citation2012).
Table 1. Oligonucleotide primers used to amplify RNA transcripts.
2.7. Statistical analysis
All statistical analyses were performed using Statistical Analysis System software (SAS V8). All results are shown as the average of at least three replicates. Data are presented as means ± the standard error (SE). Duncan’s multiple range tests were used to evaluate the statistical significance of the results. Differences with p values of <.05 were considered significant.
3. Results
3.1. Fish growth experiments
Survival rates of obscure puffer in all salinities were always higher than 93% from the beginning to the end of the experiment (), and there were no significant differences among treatments (p > .05). Growth of obscure puffer over the 60-day period is shown in . Maximum growth rate was achieved from fish reared in 16 ppt. However, there were no significant differences in growth, weight gain and specific growth rate between salinities during any period (p > .05).
Table 2. Survival rates, growth, weight gain and specific growth rate of obscure puffer grown in five different salinities.
3.2. Serum osmolality
The serum osmolalities of obscure puffer ranged from 353 to 288 mOsm/l when the fish acclimated in salinity treatments (). A significant increase in serum osmolality was observed in all salinity treatments at the beginning of the trial (day 15) (p < .05). With the exception of 24 ppt, all treatment groups experienced a decrease in serum osmolality levels during the middle and latter part of the trial (days 30, 45 and 60). No obvious change trend was observed between salinity groups at each sampling date.
3.3. Scanning electron microscopic observations
Chloride cells were abundant in the surface of gill lamella (). The number of chloride cells in gill filaments tended to increase with increasing salinities. In freshwater-acclimated fish, the apical pits of most chloride cells were shallow, and the openings were large ((a)). Conversely, in salinity groups, the apical openings were small, and the apical membranes were well developed ((b)–(e)). In addition, some chloride cells in control exhibit a smooth surface whereas that of fish in salinity groups appear rougher in their appearance. Overall, the pit structures of chloride cells became abundant with increasing salinities.
Figure 2. Scanning electron micrographs of gill filaments in obscure puffer reared at salinities of 0 (a), 8 (b), 16 (c), 24 (d) and 32 (e) ppt at the end of the trial. CC: chloride cell; Arrowhead indicates an apical surface of an accessory cell next to the chloride cell, which is characteristic of the seawater-type chloride cell.
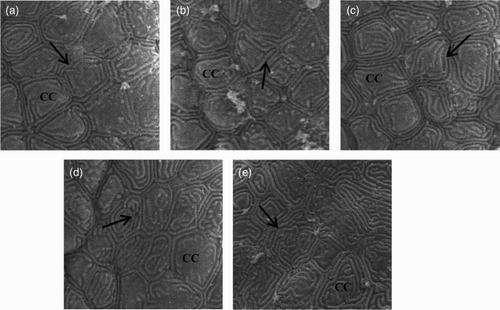
3.4. NKA activity and expression
The summarized results of the NKA activity in the gill during the course of the trial can be found in (a). Salinity had a significant effect on the mean NKA activity of obscure puffer. The NKA activity was elevated significantly in fish cultured at all salinities compared to the freshwater group (p < .05). Overall, the 32 ppt group had the highest NKA activity at days 15 and 30 between salinity groups (37.333 ± 1.7638 g; 28.333 ± 1.7638 g), whereas no significant differences were found among salinity treatments at days 45 and 60 (p > .05).
Figure 3. NKA activity (µg Pi mg protein−1 h−1) (a) and mRNA expression (fold change) (b) of gill tissue of obscure puffer reared at salinities of 0, 8, 16, 24, and 32 ppt. Different letters indicate significant differences (p < .05) between salinity groups at each sampling date.
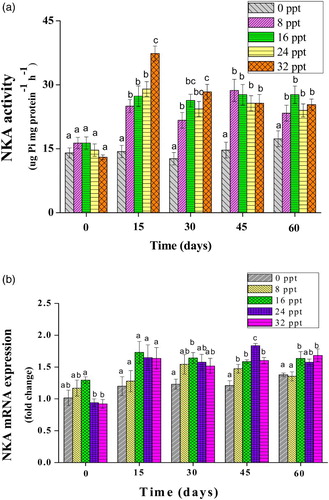
The NKA mRNA expression was also affected by salinity, whereas this effect appeared to be less significant than that of NKA activity. The mRNA levels of NKA in all salinity groups throughout the trial except 8 ppt at day 60 were higher than the control ((b)). No significant change in mRNA levels of NKA was observed during the beginning part of the trial (day 15). At day 45, a significant effect of salinity on NKA mRNA level occurred, a remarkable lower NKA level in the freshwater accounting for the difference (p < .05). A significant higher NKA level in the 24 ppt group was observed at day 45 (p < .05). At day 60, the NKA mRNA levels in 16 and 32 ppt groups were all increased significantly compared to the control (p < .05). NKA mRNA levels demonstrate a fluctuating response over time with no definite trend observed in any of the treatment groups ((b)). In addition, for all salinity groups, the NKA mRNA expression was not significantly correlated to that activity.
4. Discussion
Fish distributed in coastal waters are usually euryhaline, and their juveniles can tolerate a wide salinity range (Partridge & Jenkins Citation2002; Conides & Glamuzina Citation2004; Costa et al. Citation2008). Results of the present study suggest that juvenile obscure puffer can tolerate a wide range of salinity (0–32 ppt) and grow well at all salt treatments. Moreover, in order to deepen our knowledge, the mechanism by which the obscure puffer adjust itself and grow well in waters of different salinities needs to be understood. It has been well demonstrated that chloride cells, the ion-transporting cells in gills of teleostean fish, play a crucial role in maintenance of ionic balance. These cells are characterized by an extensive membrane system containing a high density of NKA, which plays an important role in branchial epithelial ion transport (Dang et al. Citation2000). Therefore, the morphology of chloride cells, serum osmolality, and activity and expression of NKA were studied. This investigation may help to reach a better understanding of obscure puffer and its mechanism of iron regulation.
First, alterations in serum osmolality of obscure puffer in response to salinity were tested. After direct transfer from freshwater to experimental salinities, the serum osmolality of obscure puffer increased by day 15, then decreased and returned to near-initial concentrations by day 60, indicating that obscure puffer is able to osmoregulate and maintain ionic homeostasis in a wide range of salinities. Based on serum osmolality, juvenile obscure puffer appears to successfully adjust to higher salinities. It is well established that chloride cells perform an integral role in ion homeostasis (Fielder et al. Citation2007). Not only that, the morphology of branchial chloride cells is known to alter with changes in environmental salinity; however, the degree of change in a cell can be influenced by the range of change in salinity (Dymowska et al. Citation2012). The juveniles acclimated to 24 and 32 ppt display a relative abundance of chloride cells. Moreover, the morphology of chloride cells was also significantly affected by salinity. It is likely that the morphology changes have to be made to maintain water and ion balance. Based on these results, we speculated that the changes in density and morphology of chloride cells caused by increased salinity help adapt to high-salinity conditions (Fridman et al. Citation2011). An ultra-structural hallmark of the seawater chloride cells is an invagination called apical crypt, which structure is correlated with adaptation to high salinities (Carmona et al. Citation2004). The present study is fully consistent with published findings for obscure puffer: the chloride cells of the gills of the fish living in fresh water show no apical crypt, whereas obvious invagination appears when the fish are cultured in salt water.
The successful migration of euryhaline teleosts from freshwater to seawater requires the upregulation of gill NKA (Bystriansky & Ballantyne Citation2007). Because the NKA, present in high concentrations on the basolateral side of chloride cells, is the primary driving force for flux and intra- and extracellular NaCl, and helps maintain the right concentration of solutes and amount of water in their body fluid (Imsland et al. Citation2003; Huang et al. Citation2010). It is reported that NKA activity of obscure puffer embryos and larvae exposed to salt water increases significantly compared to those in freshwater (Yang et al. Citation2010; Li et al. Citation2011). In the present study, the NKA activity and expression were all elevated by salinity, indicating that the NKA participates in the regulation of ion levels, and very likely plays a key role in maintaining the homeostasis of body’s water content. Our findings corroborate earlier studies which report a strong effect of salinity on gill NKA activity (Imsland et al. Citation2003; Ostrowski et al. Citation2011; Palacios et al. Citation2004). In addition, it has been suggested in previous investigations on branchial NKA activity and expression in fish adapted to a range of environmental salinities that the lowest activity and expression of the gill enzyme occur when the salinity of the medium is close to or slightly above that of the blood (Gaumet et al. Citation1995). Similarly, our results showed that the NKA activity and expression were lowest for fish reared in freshwater and higher in fish reared in salinities. The physiological response of NKA activity and expression in present study was consistent with the hypothesis that enzyme activity and expression are lowest in primary natural habitats.
In studies on the effects of salinity on fish ion-regulation, three compensatory mechanisms have been reported with respect to branchial ion transport activity: an increase of chloride cells numbers, changes in its morphology and increases in NKA activity and expression per chloride cell (Dang et al. Citation2000; Sun et al. Citation2012), which is further confirmed by our results. However, some other mechanisms may exist and account for the iron regulation. Because at the end of the trial, the NKA expression changed slightly, and the NKA activity decreased, while the serum osmolality decreased simultaneously. A decrease in serum osmolality should be accompanied by an increase in NKA activity. There could be some other mechanisms or processes involved in ion balance regulation, employed by obscure puffer adapt to salt water. Therefore, more work should be done to explain the discrepancy between the reduction in NKA activity and serum osmolality.
In summary, we draw two major conclusions from this study. First, the juveniles of obscure puffer can tolerate a wide range of salinity (0–32 ppt). Within this salinity range, no significant difference was observed in survival rates and growth, suggesting that the juveniles can be reared in seawater. The results of this study are useful in reducing the cost and increasing the production of the species. Second, the chloride cells became larger and denser with increasing salinity, concomitant with increases in gill NKA activity and expression, indicating that the chloride cells and NKA participate in the regulation of the osmotic pressure of body fluids to maintain the homeostasis of body’s water content. Our study has provided initial insights into the mechanisms of osmoregulation in obscure puffer.
Disclosure statement
No potential conflict of interest was reported by the authors.
Additional information
Funding
References
- Bystriansky JS, Ballantyne JS. 2007. Gill Na+-K+-ATPase activity correlates with basolateral membrane lipid composition in seawater- but not freshwater-acclimated Arctic char (Salvelinus alpinus). Am J Physiol Regul Integr Comp Physiol. 292:R1043–R1051.
- Carmona R, García-Gallego M, Sanz A, Domezaín A, Ostos-Garrido MV. 2004. Chloride cells and pavement cells in gill epithelia of Acipenser naccarii: ultrastructural modifications in seawater-acclimated specimens. J Fish Bilo. 64:553–566.
- Conides AJ, Glamuzina B. 2004. Laboratory simulation of the effects of environmental salinity on wild-caught juveniles of European sea bass Dicentrarchus labrax and gilthead seabream Sparus aurata. Aquaculture. 256:235–245.
- Costa LDF, Miranda KC, Severo MP, Sampaio LA. 2008. Tolerance of juvenile pompano Trachinotus marginatus to acute ammonia and nitrite exposure at different salinity levels. Aquaculture. 285:270–272.
- Dang ZC, Lock RAC, Flik G, Wendelaar Bonga SE. 2000. Na+/K+-ATPase immunoreactivity in branchial chloride cells of Oreochromis mossambicus exposed to copper. J Exp Biol. 203:379–387.
- Dymowska AK, Hwang PP, Goss GG. 2012. Structure and function of ionocytes in the freshwater fish gill. Respir Physiol Neurobiol. 184:282–292.
- Fielder DS, Allan GL, Pepperall D, Pankhurst PM. 2007. The effects of changes in salinity on osmoregulation and chloride cell morphology of juvenile Australian snapper, Pagrus auratus. Aquaculture. 272:656–666.
- Fridman S, Bron JE, Rana KJ. 2011. Ontogenetic changes in location and morphology of chloride cells during early life stages of the Nile tilapia Oreochromis niloticus adapted to fresh and brackish water. J Fish Biol. 79:597–614.
- Gao QX, Qi LL, Wu TX, Wang JB. 2012. An important role of IL-10 in counteracting excessive immunity in Clostridium butyricum exposed to HT-29 cells. BMC Microbiol. 12:100.
- Gaumet F, Boeuf G, Severe A, Le Roux A, Mayer-Gostan N. 1995. Effects of salinity of the ionic balance and growth of juvenile turbot. J Fish Biol. 47:865–876.
- Huang CY, Chao PL, Lin HC. 2010. Na+/K+-ATPase and vacuolar-type H+-ATPase in the gills of the aquatic air-breathing fish Trichogaster microlepis in response to salinity variation. Comp Biochem Physiol Part A. 155:309–318.
- Imsland AK, Gunnarsson S, Foss A, Stefansson SO. 2003. Gill Na+, K+-ATPase activity, plasma chloride and osmolality in juvenile turbot (Scophthalmus maximus) reared at different temperatures and salinities. Aquaculture. 218:671–683.
- Li JJ, Liu Y, Wang W, Yang Z, Chen YF. 2011. Survival and Na+/K+ ATPase specific activity in response to acute salinity change in newly hatched obscure puffer, Takifugu obscurus, larvae. J World Aquacult Soc. 42:275–280.
- Ostrowski AD, Watanabe WO, Montgomery FP, Rezek TC, Shafer TH, Morris JA. 2011. Effects of salinity and temperature on the growth, survival, whole body osmolality, and expression of Na+/K+ ATPase mRNA in red porgy (Pagrus pagrus) larvae. Aquacuture. 314:193–201.
- Palacios E, Bonilla A, Luna D, Racotta IS. 2004. Survival, Na+/K+-ATPase and lipid responses to salinity challenge in fed and starved white pacific shrimp (Litopenaeus vannamei) postlarvae. Aquacuture. 234:497–511.
- Partridge GJ, Jenkins GI. 2002. The effect of salinity on growth and survival of juvenile black bream (Acanthopagrus butcheri). Aquaculture. 210:219–230.
- Shi YH, Zhang GY, Zhu YZ, Liu JZ. 2010. Effects of photoperiod, temperature, and salinity on growth and survival of obscure puffer Takifugu obscurus larvae. Aquaculture. 309:103–108.
- Sun M, Jiang K, Zhang F, Zhang D, Shen A, Jiang M, Shen X, Ma L. 2012. Effects of various salinities on Na+-K+-ATPase, Hsp70 and Hsp90 expression profiles in juvenile mitten crabs, Eriocheir sinensis. Genet Mol Res. 11:978–986.
- Yang Z, Chen YF. 2004. Induced ovulation in obscure puffer Takifugu obscurus by injections of LHRH-a. Aquacult Int. 12:215–223.
- Yang Z, Chen YF. 2005. Effect of temperature on incubation period and hatching success of obscure puffer Takifugu obscurus (Abe) eggs. Aquaculture. 246:173–179.
- Yang Z, Chen YF. 2006. Salinity tolerance of embryos of obscure puffer Takifugu obscurus. Aquaculture. 253:393–397.
- Yang Z, Li JJ, Gu W, Liu Y, Wang W, Guo RX, Qin GX, Chen YF. 2010. Effects of salinity on survival and Na+/K+ ATPase activity of obscure puffer Takifugu obscurus embryos. J Appl Ichthyol. 26:449–452.
- Zhang G, Shi YH, Zhu YZ, Liu JZ, Zang WL. 2010. Effects of salinity on embryos and larvae of tawny puffer Takifugu flavidus. Aquaculture. 302:71–75.