ABSTRACT
Buffaloes are compulsory vaccinated against foot-and-mouth disease virus (FMDV) in many countries as part of the official control programmes. Serological testing aimed to indirectly assess herd immunity is currently performed using the same Enzyme-linked immunosorbent assays (ELISAs) applied for bovine sera, assuming an agreement between the ELISA’s diagnostic results and those obtained using the virus neutralization test (VNT). Here we evaluated the accuracy of different ELISA tests to assess vaccine-induced antibodies against FMDV in buffalo’s sera classified according to their VNT titres. Currently used liquid-phase blocking ELISA yielded very low specificity, producing high titres for many samples with low VNT titres. To increase specificity, we developed an indirect ELISA using purified 140S viral particles and an avidity single-dilution ELISA, which includes a urea washing step after the incubation of the diluted serum sample, to detach weak binders. Combining these two high-throughput single-dilution tests, an excellent concordance with VNT was achieved. This is the first study analysing the diagnostic agreement of traditional and novel serological tests with VNT for the indirect assessment of antibodies against FMDV capsid proteins in buffalo serum samples.
1. Introduction
Foot-and-mouth disease (FMD) is an acute, highly contagious viral disease that affects a wide range of hosts, including buffaloes (Grubman & Baxt Citation2004; OIE Citation2012), and is distributed worldwide. Argentina is free of the disease, with a northern region under vaccination programmes and a southern region, Patagonia, free of vaccination. Bovines and buffaloes older than 2 years of age are vaccinated once a year, while immunizations are performed every six months to calves aged up to 2 years old (SENASA Citation2013). The current vaccine in Argentina is oil-adjuvanted and contains four foot-and-mouth disease virus (FMDV) strains.
Water buffalo (Bubalus bubalis) population in Argentina is exceeding 100,000 heads, which is the third largest population in America, after Brazil and Venezuela. Buffaloes are located in the north-east of the country within the FMD vaccination territories (Crudeli Citation2014) and in neighbouring areas close to the border with Paraguay, where the latest regional FMDV-type O outbreak occurred in 2011 (Maradei et al. Citation2013; Brito et al. Citation2015). The role of African buffalo (Syncerus caffer) in the epidemiology of the disease is well documented (Dawe et al. Citation1994; Weaver et al. Citation2013; Knight-Jones et al. Citation2016), but the behaviour of FMDV in water buffaloes, which are domesticated in several areas of the world, has been less studied. FMDV transmission between cattle and water buffaloes has been observed in natural outbreaks (Dutta et al. Citation1983; Samara & Pinto Citation1983) and in experimental studies (Gomes et al. Citation1997; Maroudam et al. Citation2008). A recent study demonstrated that Indian buffaloes (Bubalus bubalis) have the potential to spread FMD by direct contact to naïve susceptible animals (Madhanmohan et al. Citation2014). In this context, the accurate assessment of immunity induced by compulsory vaccination of buffaloes is paramount.
According to the World Organization for Animal Health (OIE), the virus neutralization test (VNT) is considered as the ‘gold standard’ for the detection of antibodies reactive to FMDV structural proteins (OIE Citation2012). Liquid-phase blocking ELISA (LPBE) and VNT results have been used to develop tables that relate the serological titres of vaccinated cattle to the probability of protection, called the ‘expected percentage of protection’ (EPP). EPP estimates the likelihood that cattle would be protected against a challenge after vaccination (OIE Citation2012). In South America, a cut-off of a 75% (EPP-75%) is used to estimate protective vaccine-induced responses, statistically predicted from challenge experiments in cattle, using either VNT or LPBE titres. These cuts-off values are considered when assessing herd immunity (Maradei et al. Citation2008; Robiolo et al. Citation2010b).
LPBE entails the use of standardized capture (rabbit) and detection antibodies, either a mix of monoclonal antibodies (LPBE-Mab) or hyperimmune guinea-pig sera (LPBE-HIS). LPBE has been validated for bovine samples, as being as reliable and precise as the VNT (Robiolo et al. Citation1995; Maradei et al. Citation2008; Robiolo et al. Citation2010b) and is routinely used to assess herd immunity (Leon et al. Citation2014). A single-dilution LPBE-Mab has also been validated for the four vaccine strains included in the Argentinean commercial vaccine (Robiolo et al. Citation2010a). It has been demonstrated that the kinetics of neutralizing responses against FMDV in cattle and buffaloes is similar (Gomes et al. Citation1997; Maddur et al. Citation2009), but concordance between VNT and LPBE has not been assessed for buffalo serum samples. Moreover, information on the protective VNT or LPBE titres following a challenge in buffaloes is not available.
VNT is a time-consuming assay that requires the manipulation of live virus and cell culture, and it is difficult to standardize and set-up. In vitro neutralizing activity usually correlates with avidity (Bachmann et al. Citation1997) which can be easily measured by ELISA. Correlation between avidity and VNT has been reported for vesicular stomatitis virus (Bachmann et al. Citation1997), measles virus (Saika et al. Citation2008), tick-borne encephalitis (Leonova & Pavlenko Citation2009) and bovine viral diarrhoea virus (Franco Mahecha et al. Citation2011). We have also shown that the avidity indexes of anti-FMDV bovine antibodies are concordant with the VNT results (Lavoria et al. Citation2012; Brito et al. Citation2014).
Here we set-up a high-throughput single-dilution avidity ELISA (AE) together with an indirect ELISA (IE) for buffalo samples that use purified virus as capture antigen and do not require detection antibodies. The aim of this study was to evaluate the diagnostic agreement of traditional and novel ELISAs with VNT, to be applied for the indirect assessment of herd immunity against FMDV using buffalo serum samples.
2. Materials and methods
2.1. Serum samples
Serum samples (n = 91) were collected from two water buffalo herds (Mediterranean breed) placed in different areas of the province of Corrientes, Argentina. Samples were selected to comprise all the spectrum of neutralizing antibody levels. One herd was sampled on 5th November 2013, 5 days post-vaccination. It consisted of 43 animals, of which 25 were adult females over 3 years old, which had received at least 3 vaccinations within the official vaccine FMD programme (last one in November 2012). The other 18 animals were calves between 5 and 6 months of age with VNT titres below detection levels (Log10 titre ≤ 1). The other herd consisted of 48 animals: 33 adult vaccinated males and females (over 3 years old) that had received at least 3 vaccinations (last one in November 2012) and 15 naïve non-vaccinated calves or steers, between 7 and 12 months old, with VNT titres below detection levels (Log10 titre ≤ 1). Samples were taken on November 7th, 2013, 22 days before the corresponding vaccination campaign.
Blood samples were obtained from the jugular vein using sterile needles and syringes, placed in glass tubes (10 ml) and centrifuged at 1000 rpm for 15 min to recover serum. The samples were aliquoted in six 0.5 mL fractions and preserved at −20°C until processing. All samples tested negative for non-structural proteins by the PrioCHECK® FMDV NS Antibody ELISA Kit (ThermoFisher Scientific), performed following the manufacturer's instructions.
A positive control was generated by pooling 10 buffalo serum samples from animals with more than 5 vaccinations, with VNT and LPBE titres over Log10 = 4. A negative pool of n = 10 samples with individual titres below Log10 = 1 was used as negative plate control. These samples individually and pooled were used to estimate the intermediate precision (see below) of the IEs.
2.2. Vaccine
Animals had been immunized as part of the national control programme with an officially approved commercial tetravalent single-oil emulsion vaccine, free of non-structural proteins, containing four inactivated FMDV strains: O1/Campos/Brazil/58 (O1/Campos), A24 Cruzeiro/Brazil/55 (A24/Cruzeiro), A/Argentina/2001 (A/Arg/01) and C3/Indaial/Brazil/71 (C3/Indaial) (SENASA Citation2006b, Citation2013). This vaccine induced LPBE antibody titres compatible with an EPP-75% measured at 60 dpv for each strain, according to the national sanitary authority (SENASA) regulations (SENASA Citation2006a; SENASA Citation2010). The vaccine was applied intramuscularly in the side of the neck (2 mL/dose) according to the manufacturer’s recommendations and official regulations (SENASA Citation2010).
2.3. Serology
Serology was performed using the O1/Campos vaccine strain provided by SENASA. This strain was selected because the latest regional outbreaks were caused by O strains (Brito et al. Citation2015).
2.3.1. Neutralization assay
Serum neutralizing antibodies were titrated by a conventional VNT using infective culture-adapted FMDV O1/Campos strain. Virus titer was 107 TCID50/ml (Tissue Culture Infectious Dose 50%) on Baby Hamster Kidney cell line, as described in the OIE Manual of Diagnostic Tests and Vaccines for Terrestrial Animals and adapted by Bucafusco et al. (OIE Citation2012; Bucafusco et al. Citation2014). The endpoint titres of the serum samples were expressed as the logarithm (Log10) of the reciprocal of the last dilution of serum that neutralized 100 × TCID50 of the virus in 50% of the wells.
The EPP was used to estimate and quantify protective vaccine-induced responses. The EPP represents the correlation between bovine antibody titres at 60 days post-vaccination (dpv), measured by LPBE or VNT, and the percentages of protection achieved for the same groups of animals after in vivo challenge experiments, performed at 90 dpv following the ‘protection against generalized foot infection test’ (OIE Citation2012). The titre corresponding to EPP-75% for the FMDV O1/Campos strain is 1.65 for VNT.
2.3.2. Liquid-phase blocking ELISAs
Total anti-FMDV O1/Campos antibody responses were assessed by LPBE, performed as stated in the OIE Manual (OIE Citation2012), using two different detector antibodies: a guinea-pig anti-FMDV O1/Campos hyperimmune sera (LPBE-HIS) (Bucafusco et al. Citation2014), or a cocktail of strain-specific monoclonal antibodies (LPBE-Mab) (Robiolo et al. Citation1995; Robiolo et al. Citation2010b). Both LPBEs use a rabbit antiserum to capture the virus and an inactivated O1/Campos preparation from clarified-infected cell cultures (kindly provided by a local vaccine producer). The serum samples were incubated with a standardized dilution of inactivated virus, and free (unbound) virus was revealed using the corresponding detector antibody preparation. Antibody titres were expressed as the reciprocal log10 of serum dilutions, giving 50% of the absorbance recorded in the virus control wells without serum. The titre corresponding to EPP-75% for the FMDV O1/Campos strain is Log10 = 2.11 for LPBE using bovine serum samples (Maradei et al. Citation2008). Both LPBEs are used for assesing vaccine efficacy (SENASA Citation2010).
2.3.3. Single-dilution total antibody and AE
Avidity assessment of specific antibodies was performed as described by Lavoria et al. (Citation2012) and adapted for buffalo serum samples. Briefly, purified-inactivated O1/Campos 140S particles were used as capture antigen. Whole viral particles were obtained by 15–45% sucrose density gradient centrifugation (Barteling & Meloen Citation1974) optimized in our laboratory (Pega et al. Citation2013). The test entails a single dilution of the sample (1:50) and the presence of antibodies is expressed as OD values either after a regular PBS wash (for indirect ELISA, “IE”) or by adding a urea washing step (AE). The IE assesses total antibodies, while the AE is an assay including a urea washing step to detach low avidity binders.
2.4. Data acquisition
Buffalo serum samples were tested for neutralizing antibodies against O1/Campos strain and classified according to their titres: 27 had VNT titres below 1.65 (Log10 titre) for O1/Campos strain and 64 were above this value. The samples were then tested by two standard LPBEs and by a single-dilution ELISA to assess total antibodies (IE). The avidity of these specific antibodies was measured by the IE by adding a washing step with a chaotropic agent after incubating the serum sample, aimed to detach low-binding antibodies (AE). The results obtained by all these ELISAs were compared to VNT titres to evaluate their concordance with this test in predicting a protective status.
2.5. Statistical analysis
Serological assays were performed blinded to the results of the other tests and were then compared all together. VNT titres were used as a gold standard to classify antibody levels related to the VNT EPP-75% for the FMDV O1/Campos strain (Log10 = 1.65).
Receiver operator characteristic (ROC) curves (Greiner et al. Citation2000) using O1/Campos LPBE titres, IE and AE OD values computed with VNT titres were constructed. The area under the curve (AUC) was calculated and tested for statistical significance. The sensitivity (Se), specificity (Sp) and accuracy of the dichotomized test results were determined by the optimal AUC value in the ROC curves for results obtained with LPBE-Mab, LPBE-HIS, IE and AE.
Correlation between the different tests was assessed using Pearson’s correlation analysis (Pearson’s r value) with a 95% confidence interval (CI). Linear regression was applied to compare both LPBEs, and also ELISA results with VNT titres. Goodness of fit was estimated by the r2 value and a runs test was used to calculate deviation from linearity. Intermediate precision was estimated by computing the coefficient of variations of values obtained with the 20 positive control samples and 15 negative samples run by two different operators using the same measurement procedure, and replicate measurements obtained over an extended period of time, repeated with three different reagent lots. The results were compared by ANOVA 2-factor repeated measures followed by a Bonferroni multiple comparisons test. The CI was 95%. These analyses were carried out using GraphPad Prism v5.0 (GraphPad Software).
To determine the pair-wise level of agreement between results obtained by the different ELISAs and VNT, we computed Cohen’s Kappa (κ) value and its 95% CIs. The level of agreement was interpreted according to κ value as none (0.0–0.2), minimal (0.21–0.39), weak (0.40–0.59), moderate (0.60–0.79), strong (0.80–0.90) or excellent (above 0.91) (McHugh Citation2012).
To optimize diagnostic accuracy, the Se and Sp of all possible 2-test combinations were also estimated (using MedCalc software Version 16.8). We exercised a sequential evaluation meant to augment the overall specificity: the samples were first run using one of the high-sensitive tests (called ‘T1’), negative results were accepted and those samples with values above the T1 cut-off were retested using a high specific assay (T2).
We computed ROC curves, AUC values, CI, correlation and cut-off test values aimed to maximize accuracy in terms of Se and Sp using ROCR package in R. (Sing et al. Citation2005). An online calculator was used to obtain the κ value (http://analyse-it.com/docs/220/method_evaluation/qualitative.htm).
3. Results
3.1. Performance of the ELISAs with buffalo serum samples
In order to verify the correct performance of the IEs with buffalo serum samples, we estimated the intra-plate and intra-day repeatability of both IEs. The intra-laboratory repeatability (intermediate precision) was estimated with results obtained by two different technicians, using three replicates of 10 samples with high VNT titres against the O1/Campos strain. The samples were run on three different days, using three different reagent batches. Coefficients of variation for positive control samples were below 10% for all the operators for both assays. No significant differences were found between mean IE and AE intra- or inter-plate OD values (p > 0.05). ANOVA analysis showed there were no differences in the OD values due to the operator, the urea treatment or the reagent lot used (p = 0.45, 0.54 and 0.84, respectively). For IE, no significant differences in OD were found due to the operator or reagent lot (p > 0.05).
We then analysed if both LPBEs (Mab and HIS) performed equally, as they do for bovine serum samples. The titres obtained in both assays were plotted. LPBE-Mab and LPBE-HIS gave concordant results () with significant correlation (Pearson’s r = 0.8041, CI 0.7154–0.8675; p < .0001). Only five non-concordant results were found between both LPBE tests. Virus neutralization titres of these samples indicated that three of them were classified correctly by LPBE-HIS and the other two samples were correctly categorized by LPBE-Mab. VNT titres of outliers are shown in .
3.2. Comparative agreement between ELISAs and VNT diagnostic results
In order to assess the sensitivity and specificity of the novel tests using buffalo’s serum samples, ROC curves were built () using LPBE titres or OD values (for IE and AE). The values obtained for the AUC for each ELISA indicated that the four tests were accurate and useful to classify buffalo serum samples. The values were very good for LPBE (LPBE-HIS: AUC = 0.82; LPBE-Mab AUC = 0.76) and excellent for IE and AE (AUC: 0.92 and 0.97, respectively). For all the tests, the overall sensitivity and specificity were determined by using the cut-off value, giving the best diagnostic performance. Sensitivity was about 84% for both LPBEs, but specificity was low (∼ 48%; ). The cut-off values were OD = 0.99 for IE and OD = 0.7 for AE. These assays yielded an overall result of good sensitivity (71.2% for IE and 74.6% for AE) and very good specificity. The highest specificity value was achieved by the AE (93.8%; ).
Figure 1. Individual LPBE titres (log scale) against O1/Campos measured by LPBE-HIS or LPBE-Mab were plotted to study the concordance between both tests. Dotted lines indicate the cut-off value for EPP-75%. Open squares correspond to three samples classified correctly by LPBE-HIS and open circles correspond to those classified correctly by LPBE-Mab. VNT titres of outliers are indicated above each tick.
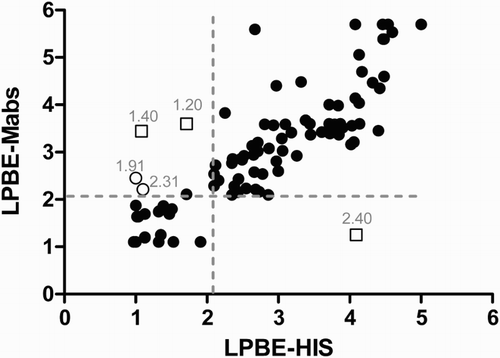
Figure 2. ROC curves were built for LPBE-His (A), LPBE-Mab (B), indirect ELISA ‘IE’ (C) and avidity ELISA ‘AE’ (D). Values for the AUC, standard error, CI and p-values are shown for each individual curve.
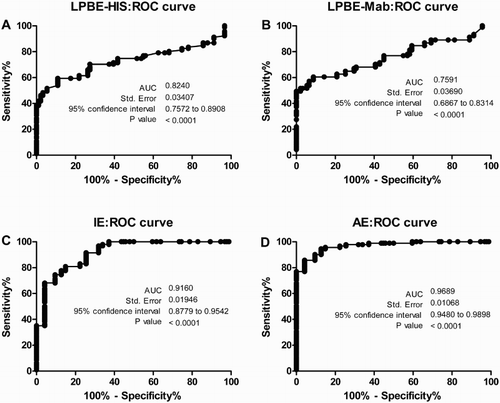
Table 1. Concordance between VNT and the ELISAs.
A weak agreement was found between the four ELISAs and VNT; although correlation was significant (p < 0.05), Pearson’s r values were low for the four assays (). Concordance between VNT and the ELISAs was assessed by computing the Kappa value. Only AE showed a weak agreement with VNT results (κ = 0.62, ), while the other assays yielded only a minimal agreement (κ = 0.35).
Table 2. Correlation between the different ELISAs and VNT.
3.3. Optimizing the diagnostic accuracy of the serological tests
The pattern of relative frequency distribution of different values organized by range was then analysed. The results are shown in . The frequency graph shows that AE classified correctly 85% of the samples with VNT titres below Log10 = 1.65, most of them with low OD values (<0.3). However, about 50% of the positive values were found in an OD range coincident with 36% of the negative samples. These samples had VNT titres close to the cut-off value. Most of the positive samples were classified correctly by IE as 78% of the samples with VNT titres above Log10 = 1.65 gave OD values >1. The graphs also showed that LPBE-HIS and LPBE-Mab were good in identifying high positive samples, with VNT titres over Log10 = 3. However, negative samples were distributed with a similar frequency among all the titre ranges.
Figure 3. The graphs depict the percentage of samples with VNT titres above or below the EPP-75% cut-off value within each range of values obtained by AE and IE (OD values) or LPBE (titres). Arrows indicate the approximate position of the cut-off values used for each assay.
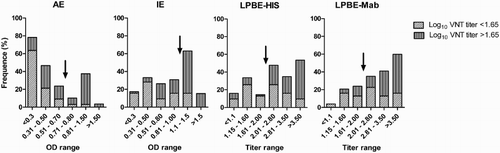
Since LPBEs’ assessment yielded low specificity when using buffalo samples, we exercised a sequential evaluation meant to augment the overall specificity using LPBE-HIS (). The samples were first classified as negative by LPBE when their titres were equal or below 1.6. Using this cut-off, only 10% of the samples were found to be false positives. The samples with values above LPBE Log10 titre = 1.6 were retested in the AE (Test 2) and classified as positive when the OD values were above 0.7 (cut-off for best accuracy by ROC, ). This serial testing yielded an excellent specificity (Sp = 90.6) and a very good sensitivity (Se = 87.9), and concordance with VNT was moderate (κ = 0.76).
Table 3. The combined application of different ELISAs enables a high concordance with VNT results.
In a second exercise, we combined AE and IE, which can be run simultaneously and in a single dilution. These tests had high specificity but lacked sensitivity. Parallel testing aimed to increase sensitivity implies to consider a sample as ‘positive’ when any of the tests or both give a positive result, and negative only when both give negative results. Serial testing using one test to accept positives reduced the specificity to unacceptable levels (data not shown).
Based on frequency graphs, we considered that a first selection could be done with OD values from AE testing, considering as negative samples those with OD values equal to or below 0.35, and as positive those over 1.1. Samples with ODs between those values were reassessed by IE, with a cut-off of 1.1. Results () show that in fact this combination increased sensitivity to 94.8%, the highest value estimated so far, while specificity was still acceptable (84.4%). This combined testing yielded a strong concordance with the VNT titres (κ = 0.803). Thus, running a single plate containing serum samples by these IEs, a strong concordance with VNT results can be achieved, allowing an accurate surveillance of vaccine performance in the field using buffalo serum samples.
4. Discussion
An accurate assessment of herd immunity to estimate the expected protection against FMDV is paramount for countries undergoing compulsory vaccination programmes. Serology studies measuring antibodies to structural proteins induced by vaccines are not available for buffaloes; there is no information about the protective VNT titres in this species, and there is only one paper published in the 1990s (Araujo et al. Citation1996) analysing if LPBE titres match with those measured by the VNT.
Here we tested 91 buffalo serum samples with different VNT titres and compared the results with those obtained with traditional LPBE and two novel IEs. The currently used LPBEs are performed either using monoclonal antibodies or hyperimmune sera as the detector antibodies. Our results show that both assays perform equally with buffalo serum samples, meaning that they will provide equivalent information as they do with bovine samples. Only five samples were classified differently by these tests, considering their VNT titres as reference values.
Here we found that, unlike the results obtained with bovine samples, anti-FMDV serum neutralizing titres did not match those measured by the LPBEs. In fact, although sensitivity was acceptable for this assay, specificity was low. Based on the literature, we cannot attribute this difference to an intrinsic performance of the neutralization tests with buffalo antibodies. Maddur et al. (Citation2009) demonstrated that the kinetics of the serum neutralizing antibody response to FMDV Asia 1 infection in buffaloes was similar to that of cattle. Gomes et al. (Citation1997) also reported similarity in the kinetics of VNT titres in the sera of FMDV type O-infected buffaloes and cattle. Our data show that the lack of agreement between LPBEs and VNT is mainly due to high LPBE titres achieved from serum samples with low VNT titres. We speculate that the presence of low binders capturing FMDV particles may account for this difference. A biologic activity like virus neutralization (assessed by VNT) may be more dependent on high-avidity antibody binding to the viral particle. This same hypothesis can explain why the AE rendered the highest specificity. From our data, we infer there might be larger amount of low-avidity binders, such as low affinity natural antibodies (Nab) (Casali & Notkins Citation1989) in buffalo than in bovine sera. Nab have been found in cattle (Ploegaert et al. Citation2011) and buffaloes (Matheswaran et al. Citation2003), and although we could not find in the literature evidence on the presence of higher levels of low affinity antibodies or B1 cells in buffaloes compared to cattle, it has been previously described that although buffaloes are susceptible to most of the pathogens that affect cattle, diseases in these animals are often less harmful (Yang et al. Citation2012; Reichel et al. Citation2015).
Using LPBE for testing a large number of samples is labour intensive. With the purpose of providing a high-throughput, low labour test, we developed two IEs using purified-inactivated virus as capture antigen, which minimizes the presence of cross-reactive binders as a strategy to improve specificity. These assays avoid the need of developing coating and detector antibodies for each particular strain and allow high-throughput assessment as they are performed with a single dilution of the sera, for detecting both total (IE) and high-avidity antibodies (AE). AEs are usually used to discriminate between chronic and acute infections, or as an alternative to neutralization assays, among other applications (Thomas & Morgan-Capner Citation1988; Enders & Knotek Citation1989; Schubert et al. Citation1998; Fox et al. Citation2006; Hamkar et al. Citation2006; Franco Mahecha et al. Citation2011). This strategy has been applied before to detach low binders and increase the specificity of the ELISA applied for FMDV diagnosis in cross-protection trials (Brito et al. Citation2014). In this study the AE yielded high specificity (∼90%), although the sensitivity was not good enough as to be used as a sole alternative assay to the VNT.
Considering the different sensitivity and specificity of the serological assays, we investigated if combining two tests in a sequence-based analysis could render higher diagnostic agreement with VNT. Using a simple serial testing approach by running the highest sensitive ELISA (LPBE), accepting negatives (low cut-off) and retesting positives with a highly specific assay (AE) increased concordance with VNT to 76% and sensitivity to 88%. The best agreement values (κ = 80%) were obtained when combining the results of both single-dilution ELISAs, meaning that a single-dilution ELISA assay can produce similar diagnostic results as VNT when using buffalo serum samples, with the additional benefit of having a high-throughput reproducible assessment that only takes a few hours of work to provide the result.
The aim of this study was to evaluate the concordance between VNT and ELISA results when using buffalo serum samples; thus, the samples were classified according to their VNT titre, without any further consideration. We have a selection bias due to the compulsory vaccination campaign applied in Argentina, as all non-vaccinated animals are between 6 and 18 months old. Data from our laboratory and others show that calves are immunologically identical to adult animals at this age (Sadir et al. Citation1988; Spath et al. Citation1995; Bucafusco et al. Citation2014); thus, we do not expect an impact on antibody titres due to this bias. We cannot rule out, however, the role of IgMs, Nabs or high-affinity IgGs as a result of multiple vaccinations in the results obtained by either test.
Our results need to be confirmed and extended to more samples and also using different vaccine preparations. Here we tested only 91 samples against the O1/Campos vaccine strain, selected due to the importance of serotype O in the most recent outbreaks in the region and because O1/Campos 140S particles are the most unstable antigen in the current vaccine formulation (Bucafusco et al. Citation2014). Preliminary data from our laboratory show that the results are similar when testing these samples with the different ELISAs against the A24 Cruzeiro strain. This is expected as all the commercial vaccines produced in Argentina are controlled for antibody responses against each vaccine strain, and differences in the immune response induced by these strains in terms of total and neutralizing antibodies have never been reported.
VNT tests are expensive; they require dedicated facilities and manipulating live virus, which needs to be produced, titrated and stored. Cell culture adds variability and contamination is always a threat. About 40 serum samples can be run each time by one trained operator, who will provide results supported by visual reading not before than 48 h upon sample arrival. LPBE is a great improvement to VNT, but relies on capture and detector antibodies that need to be produced and standardized. The novel IEs only use purified 140S particles. In our study, 30 ml of a PEG-concentrated inactivated virus batch yielded about 2 mg of purified 140S particles, which is enough for preparing 1000 plates; this means 40,0000 serum samples can be evaluated with a single antigen batch. A trained operator can run up to 10 plates (400 samples) in half-day of work, producing results that are objective and traceable.
This is the first study that analyses the diagnostic accuracy of traditional and novel tests routinely utilized for the assessment of antibodies against FMDV in buffaloes. The biological features of the humoral responses in bubaline species may interfere with the traditional ELISA tests, causing an inaccurate assessment of the vaccine-induced antibody responses, especially due to false-positive results. In this regard, the combination of two single-dilution high-throughput ELISAs may afford a cheaper, simpler and faster alternative to the VNT. Provided a thorough validation is performed, these assays may be useful for monitoring the effectiveness of vaccination campaigns in buffalo populations.
Disclosure statement
No potential conflict of interest was reported by the authors.
ORCID
Alejandra Victoria Capozzo http://orcid.org/0000-0002-7117-9955
Additional information
Funding
References
- Araujo JP, Montassier HJ, Pinto AA. 1996. Liquid-phase blocking sandwich enzyme-linked immunosorbent assay for detection of antibodies against foot-and-mouth disease virus in water buffalo sera. Am J Vet Res. 57:840–843.
- Bachmann MF, Kalinke U, Althage A, Freer G, Burkhart C, Roost H-P, Aguet M, Hengartner H, Zinkernagel RM. 1997. The role of antibody concentration and avidity in antiviral protection. Science. 276:2024–2027. doi: 10.1126/science.276.5321.2024
- Barteling SJ, Meloen RH. 1974. A simple method for the quantification of 140S particles of foot-and-mouth disease virus (FMDV). Arch Gesamte Virusforsch. 45:362–364. doi: 10.1007/BF01242879
- Brito BP, Rodríguez LL, Hammond JM, Pinto J, Pérez AM. 2015. Review of the global distribution of foot-and-mouth disease virus from 2007 to 2014. Transbound Emerg Dis. doi:10.1111/tbed.12373.
- Brito BP, Perez AM, Capozzo AV. 2014. Accuracy of traditional and novel serology tests for predicting cross-protection in foot-and-mouth disease vaccinated cattle. Vaccine. 32:433–436. doi: 10.1016/j.vaccine.2013.12.007
- Bucafusco D, Di Giacomo S, Pega J, Juncos MS, Schammas JM, Pérez-Filgueira M, Capozzo AV. 2014. Influence of antibodies transferred by colostrum in the immune responses of calves to current foot-and-mouth disease vaccines. Vaccine. 32:6576–6582. doi: 10.1016/j.vaccine.2014.06.056
- Casali P, Notkins AL. 1989. CD5+ B lymphocytes, polyreactive antibodies and the human B-cell repertoire. Immunol Today. 10:364–368. doi: 10.1016/0167-5699(89)90268-5
- Crudeli GA, Exequiel P, Maldonado Vargas P, Konrad JL. 2014. Past, present and future of buffalo in Argentina. Revista Veterinaria. 25:140–145.
- Dawe PS, Sorensen K, Ferris N, Barnett I, Armstrong R, Knowles N. 1994. Experimental transmission of foot-and-mouth disease virus from carrier African buffalo (Syncerus caffer) to cattle in Zimbabwe. Vet Rec. 134:211–215. doi: 10.1136/vr.134.9.211
- Dutta PK, Sarma G, Das SK. 1983. Foot-and-mouth disease in Indian buffaloes. Vet Rec. 113:134. doi: 10.1136/vr.113.6.134
- Enders G, Knotek F. 1989. Rubella IgG total antibody avidity and IgG subclass-specific antibody avidity assay and their role in the differentiation between primary rubella and rubella reinfection. Infection. 17:218–226. doi: 10.1007/BF01639523
- Fox JL, Hazell SL, Tobler LH, Busch MP. 2006. Immunoglobulin G avidity in differentiation between early and late antibody responses to West Nile virus. Clin Vaccine Immunol. 13:33–36. doi: 10.1128/CVI.13.1.33-36.2006
- Franco Mahecha OL, Ogas Castells ML, Combessies G, Lavoria MA, Wilda M, Mansilla FC, Seki C, Grigera PR, Capozzo AV. 2011. Single dilution Avidity-Blocking ELISA as an alternative to the Bovine Viral Diarrhea Virus neutralization test. J Virol Methods. 175:228–235. doi: 10.1016/j.jviromet.2011.05.022
- Gomes I, Ramalho AK, Auge de Mello P. 1997. Infectivity assays of foot-and-mouth disease virus: contact transmission between cattle and buffalo (Bubalus bubalis) in the early stages of infection. Vet Rec. 140:43–47. doi: 10.1136/vr.140.2.43
- Greiner M, Pfeiffer D, Smith RD. 2000. Principles and practical application of the receiver-operating characteristic analysis for diagnostic tests. Prev Vet Med. 45:23–41. doi: 10.1016/S0167-5877(00)00115-X
- Grubman MJ, Baxt B. 2004. Foot-and-mouth disease. Clin Microbiol Rev. 17:465–493. doi: 10.1128/CMR.17.2.465-493.2004
- Hamkar R, Mahmoodi M, Nategh R, Jelyani KN, Eslami MB, Mohktari-Azad T. 2006. Distinguishing between primary measles infection and vaccine failure reinfection by IgG avidity assay. East Mediterr Health J. 12:775–782.
- Knight-Jones TJD, Robinson L, Charleston B, Rodriguez LL, Gay CG, Sumption KJ, Vosloo W. 2016. Global foot-and-mouth disease research update and gap analysis: 2 – epidemiology, wildlife and economics. Transbound Emerg Dis. 63(Suppl 1):14–29. doi: 10.1111/tbed.12522
- Lavoria MA, Di-Giacomo S, Bucafusco D, Franco-Mahecha OL, Pérez-Filgueira DM, Capozzo AV. 2012. Avidity and subtyping of specific antibodies applied to the indirect assessment of heterologous protection against foot-and-mouth disease virus in cattle. Vaccine. 30:6845–6850. doi: 10.1016/j.vaccine.2012.09.011
- Leon EA, Perez AM, Stevenson MA, Robolio B, Mattion NM, Seki C, La Torre JL, Torres A, Cosentino B, Duffy SJ. 2014. Effectiveness of systematic foot and mouth disease mass vaccination campaigns in Argentina. Rev Sci Tech. 33:917–926. doi: 10.20506/rst.33.3.2329
- Leonova GN, Pavlenko EV. 2009. Characterization of neutralizing antibodies to Far Eastern of tick-borne encephalitis virus subtype and the antibody avidity for four tick-borne encephalitis vaccines in human. Vaccine. 27:2899–2904. doi: 10.1016/j.vaccine.2009.02.069
- Maddur MS, Kishore S, Gopalakrishna S, Singh N, Suryanarayana VV, Gajendragad MR. 2009. Immune response and viral persistence in Indian buffaloes (Bubalus bubalis) infected with foot-and-mouth disease virus serotype Asia 1. Clin Vaccine Immunol. 16:1832–1836. doi: 10.1128/CVI.00302-09
- Madhanmohan M, Yuvaraj S, Nagendrakumar SB, Srinivasan VA, Gubbins S, Paton DJ, Parida S. 2014. Transmission of foot-and-mouth disease virus from experimentally infected Indian buffalo (Bubalus bubalis) to in-contact naive and vaccinated Indian buffalo and cattle. Vaccine. 32:5125–5130. doi: 10.1016/j.vaccine.2014.03.094
- Maradei E, La Torre J, Robiolo B, Esteves J, Seki C, Pedemonte A, Iglesias M, D’Aloia R, Mattion N. 2008. Updating of the correlation between lpELISA titers and protection from virus challenge for the assessment of the potency of polyvalent aphtovirus vaccines in Argentina. Vaccine. 26:6577–6586. doi: 10.1016/j.vaccine.2008.09.033
- Maradei E, Malirat V, Beascoechea CP, Benitez EO, Pedemonte A, Seki C, Novo SG, Balette CI, D’Aloia R, La Torre JL, et al. 2013. Characterization of a type O foot-and-mouth disease virus re-emerging in the year 2011 in free areas of the southern cone of South America and cross-protection studies with the vaccine strain in use in the region. Vet Microbiol. 162:479–490. doi: 10.1016/j.vetmic.2012.10.035
- Maroudam V, Nagendrakumar SB, Madhanmohan M, Santhakumar P, Thiagarajan D, Srinivasan VA. 2008. Experimental transmission of foot-and-mouth disease among Indian buffalo (Bubalus bubalis) and from buffalo to cattle. J Comp Pathol. 139:81–85. doi: 10.1016/j.jcpa.2008.05.003
- Matheswaran K, Dhinakar Raj G, Nachimuthu K. 2003. Demonstration of alternative and classical complement pathway activity in colostrum from buffalo (Bubalus bubalis). Vet Res Commun. 27:445–452. doi: 10.1023/A:1025785506954
- McHugh ML. 2012. Interrater reliability: the kappa statistic. Biochem Med (Zagreb). 22:276–282. doi: 10.11613/BM.2012.031
- OIE. 2012. World Organization for Animal Health Capter 8.8. Paris: OIE.
- Pega J, Bucafusco D, Di Giacomo S, Schammas JM, Malacari D, Capozzo AV, Arzt J, Perez-Beascoechea C, Maradei E, Rodriguez LL, et al. 2013. Early adaptive immune responses in the respiratory tract of foot-and-mouth disease virus-infected cattle. J Virol. 87:2489–2495. doi: 10.1128/JVI.02879-12
- Ploegaert TC, Tijhaar E, Lam TJGM, Taverne-Thiele A, van der Poel JJ, van Arendonk JAM, Savelkoul HFJ, Parmentier HK. 2011. Natural antibodies in bovine milk and blood plasma: variability among cows, repeatability within cows, and relation between milk and plasma titers. Vet Immunol Immunopathol. 144:88–94. doi: 10.1016/j.vetimm.2011.07.008
- Reichel MP, McAllister MM, Nasir A, Moore DP. 2015. A review of Neospora caninum in water buffalo (Bubalus bubalis). Vet Parasitol. 212:75–79. doi: 10.1016/j.vetpar.2015.08.008
- Robiolo B, Grigera PR, Periolo OH, Seki C, Bianchi T, Maradei E, La Torre JL. 1995. Assessment of foot and mouth disease vaccine potency by liquid-phase blocking ELISA: a proposal for an alternative to the challenge procedure in Argentina. Vaccine. 13:1346–1352. doi: 10.1016/0264-410X(94)00084-Z
- Robiolo B, La Torre J, Duffy S, Leon E, Seki C, Torres A, Mattion N. 2010a. Quantitative single serum-dilution liquid phase competitive blocking ELISA for the assessment of herd immunity and expected protection against foot-and-mouth disease virus in vaccinated cattle. J Virol Methods. 166:21–27. doi: 10.1016/j.jviromet.2010.02.011
- Robiolo B, La Torre J, Maradei E, Beascoechea CP, Perez A, Seki C, Smitsaart E, Fondevila N, Palma E, Goris N, et al. 2010b. Confidence in indirect assessment of foot-and-mouth disease vaccine potency and vaccine matching carried out by liquid phase ELISA and virus neutralization tests. Vaccine. 28:6235–6241. doi: 10.1016/j.vaccine.2010.07.012
- Sadir AM, Schudel AA, Laporte O, Braun M, Margni RA. 1988. Response to foot-and-mouth disease vaccines in newborn calves. Influence of age, colostral antibodies and adjuvants. Epidemiol Infect. 100:135–144. doi: 10.1017/S0950268800065626
- Saika S, Matsunaga T, Ogawa T, Ichinohe S. 2008. [Gelatin particle agglutination (PA) antibody: comparison to neutralizing antibody (NT) and hemagglutination inhibition (HI) antibody and relationship to IgG avidity]. Kansenshogaku Zasshi. 82:310–316. doi: 10.11150/kansenshogakuzasshi1970.82.310
- Samara SI, Pinto AA. 1983. Detection of foot-and-mouth disease carriers among water buffalo (Bubalus bubalis) after an outbreak of the disease in cattle. Vet Rec. 113:472–473. doi: 10.1136/vr.113.20.472
- Schubert J, Zens W, Weissbrich B. 1998. Comparative evaluation of the use of immunoblots and of IgG avidity assays as confirmatory tests for the diagnosis of acute EBV infections. J Clin Virol. 11:161–172. doi: 10.1016/S0928-0197(98)00061-0
- SENASA. 2006a. Animal Health Service. Act no. 351/2006. In: Boletín Oficial No. 30.940, Argentina, July 5th; 2006. Available from: http://infoleg.mecon.gov.ar/infolegInternet/anexos/115000-119999/117636/norma.htm
- SENASA. 2006b. Resolución 351/2006. Servicio Nacional de Sanidad y Calidad Agroalimentaria.
- SENASA. 2010. ‘Asunto Productos destinados a combatir la fiebre aftosa’.
- SENASA. 2013. Resolución 82/2013. Servicio Nacional de Sanidad y Calidad Agroalimentaria.
- Sing T, Sander O, Beerenwinkel N, Lengauer T. 2005. ROCR: visualizing classifier performance in R. Bioinformatics. 21:3940–3941. doi: 10.1093/bioinformatics/bti623
- Spath EJ, Smitsaart E, Casaro AP, Fondevila N, Fernández F, Leunda MR, Compaired D, Buffarini M, Pessi H. 1995. Immune response of calves to foot-and-mouth disease virus vaccine emulsified with oil adjuvant. Strategies of vaccination. Vaccine. 13:909–914. doi: 10.1016/0264-410X(95)00009-P
- Thomas HI, Morgan-Capner P. 1988. Rubella-specific IgG subclass avidity ELISA and its role in the differentiation between primary rubella and rubella reinfection. Epidemiol Infect. 101:591–598. doi: 10.1017/S0950268800029459
- Weaver GV, Domenech J, Thiermann AR, Karesh WB. 2013. Foot and mouth disease: a look from the wild side. J Wildl Dis. 49:759–785. doi: 10.7589/2012-11-276
- Yang J, Fu Z, Feng X, Shi Y, Yuan C, Liu J, Hong Y, Li H, Lu K, Lin J. 2012. Comparison of worm development and host immune responses in natural hosts of Schistosoma japonicum, yellow cattle and water buffalo. BMC Vet Res. 8:25. doi: 10.1186/1746-6148-8-25