ABSTRACT
An experiment was conducted to evaluate the biochemical changes and digestive enzyme activities of juvenile Panulirus homarus lobsters kept under three different feeding regimes, namely starvation, feeding with live clam and feeding with formulated feeds. A marked reduction in the hepatosomatic index (HSI) and a decrease in levels of both protein and lipid in the hepatopancreas of starved animals were observed at the end of the trial. Results indicate that hepatopancreas forms the primary organ for mobilization of energy reserves and that both proteins and lipids are mobilized as energy sources during starvation. Starvation induced a significant increase in proteolytic digestive enzymes of the hepatopancreas. In the group fed on formulated diet, amylase activity was found to be high, probably in response to the high carbohydrate content of the feed used in the present study. These animals also had a significantly lower free amino acid content in the hepatopancreas Histological studies showed that feeding with formulated diet-induced vacuolation in the hepatopancreas caused by hypertrophy of B cells, and a distinct thickening of the lumen walls. These results suggest difficulty in metabolism and mobilization of nutrients absorbed from formulated feeds in tropical spiny lobsters.
KEYWORDS:
Introduction
The development of environment friendly and cost effective formulated feed has been recognized as one of the major constraints for large-scale culture of the spiny lobsters (Williams Citation2007). At present, it is not possible to achieve the best growth and survival in spiny lobsters without the use of raw molluscan flesh (Johnston et al. Citation2007; Simon and James Citation2007). However, the use of this natural food during culture is far from ideal for large-scale operations (Smith et al. Citation2003). A number of reasons have been propounded for the reduced growth and survival of spiny lobsters fed exclusively on formulated diets (Williams Citation2007). Feeding formulated diet represents a deviation from the natural feeding habits of spiny lobsters (Juinio and Cobb Citation1992; Cox and Johnston Citation2003) and to understand the consequences, the underlying physiological mechanisms of digestion and nutrient utilization must be understood. Food limitation induces nutrient deficiency and is an indicator of the utilization of nutrient reserves from body tissues (Sanchez-Paz et al. Citation2006). Therefore, a comparison of starved animals with the animals fed with formulated feed may reveal information about the inability of the lobsters to utilize nutrients from formulated feeds and consequently remaining starved despite ingesting the pellets. This may shed light on the biochemical and physiological processes associated with the inability of the lobsters to adapt and grow on formulated feeds.
Growth performance is also controlled by the efficiency of the animal to digest and absorb specific nutrients from food (Simon and Jeffs Citation2008). This capacity to obtain nutrients from a particular food source is largely determined by the digestive enzymes. Most of the investigations of the digestive enzymes in lobsters undertaken so far have been mainly to study biochemical characteristics, especially changes in the enzyme activity with changing life stages (Johnston et al. Citation2004; Perera et al. Citation2008). Although the effect of feeding on the digestive enzyme secretion of temperate lobster (Simon Citation2009) and other crustaceans have been studied in detail (Barker and Gibson Citation1977; Al-mohanna et al. Citation1985; Ong and Johnston Citation2006), such studies are lacking in tropical lobsters.
Panulirus homarus is an important tropical lobster of food value and efforts to standardize the culture technology of this species are underway (Rao et al. Citation2010). The main aim of this study was to compare the biochemical changes and digestive enzyme activities of P. homarus under different feeding regimes of starvation, natural food and formulated feed. The knowledge gained from this study may be used as a basis to optimize the nutrition of P. homarus during large-scale farming.
Material and methods
Experimental animals
Juvenile rock lobster P. homarus lobsters, having a carapace length of 44.93 ± 1.88 mm and weighing 75.66 ± 8.43 g, collected in trap gill nets were procured from fishers with least stress and injuries. They were held in quarantine for a few days to reduce the stress and acclimatized in the wet lab facility. The animals were reared for 15 days prior to the start of the experiment and fed freshly cut clam (Meretrix casta) to satiation, twice daily.
Experimental design
After acclimatization to the laboratory environment, 27 animals in the intermolt stage were randomly distributed into three groups of 9 animals each. Each group was further divided into three replicates of three experimental units each. The first group (T1) was provided a diet of freshly cut (as fed) clam, M. casta, at the rate of 5% of body weight. The second group (T2) was maintained at complete starvation. The third group was provided formulated diet (on dry matter basis) at 5% of body weight. The experiment was carried out for 20 days. Daily monitoring of the water quality parameters was carried out.
Experimental feeds
For the present study, the live clams (M. casta) were collected from Muttukadu Lake, Chennai. The clams were brought to the Kovalam Field laboratory, CMFRI, rinsed and maintained in filtered seawater (500L) tanks, and fed with mixed algal culture. The formulated feed used for the experiment was a soybean and shrimp head extract based diet ().
Table 1. Formulation of pellet diet and proximate composition of the pellet diet and clam meat used as food (g%).
Hepatosomatic index and tail weight index
The hepatopancreas weight and tail weight of animals of different treatment groups were recorded and the hepatosomatic index and tail weight index were calculated as:
Biochemical analysis
After the 20 days feeding experiment, the animals were sacrificed and muscle and hepatopancreas were removed carefully and weighed. The lipid content of hepatopancreas and muscle tissue was estimated by the method of Folch et al. (Citation1957) using chloroform–methanol (2:1) as the extractant. The determination was made using the sulfophospho-vanillin method described by Frings and Dunn (Citation1970). Total protein of each tissue was estimated by the method of Lowry et al. (Citation1951).
Enzyme assays and free amino acid content
Hepatopancreas was homogenized using tissue homogenizer in chilled sucrose solution (0.25M) added to obtain 5% tissue homogenate concentration. The tubes were continuously kept on ice to avoid thermal influence. The homogenates were centrifuged at 5000 rpm for 10 min at 4°C. The supernatant collected was stored at −20°C until further use. This was used for enzyme analysis and free amino acid analysis.
The hepatopancreas protease activity was determined by the method of casein hydrolysis (Walter Citation1984). Each assay consisted of 100 mMTris, 50 mMNaCl buffer pH 8.0 and 1% casein (w/v) dissolved in 100 mMTris, 50mMNaCl buffer pH 8.0. The reaction commenced with the addition of enzyme extract, incubated for 60 min at 37°C, and stopped by adding 8% (w/v) trichloroacetic acid. Reaction tubes were placed immediately on ice for 30 min, centrifuged at 1200 rpm (968×g) for 10 min and the absorbance of the supernatant read at 280 nm. One unit of total protease activity was calculated from a tyrosine standard curve that was generated by diluting aliquots of the tyrosine stock solution with 100 mMHCl.
Trypsin activity was assayed using N-α-benzoylarginine-p-nitroanilide hydrochloride (Sigma) dissolved in DMSO as a substrate (Johnston Citation2003). The assay mixture contained a final concentration of 2 mM BAPNA in 100 mM ammonium bicarbonate, 100 mMNaCl and 0.2% (w/v) polyethylene glycol 6000 (PEG) (pH 8.0), incubated at 37°C. The release of p-nitroaniline was measured at 405 nm over 20 min using a p-nitroaniline standard curve. A positive control of bovine pancreas trypsin dissolved in HCl was used.
Chymotrypsin activity was determined by using benzoyl-L-tyrosine ethyl ester (Sigma) dissolved in dimethylsulphoxide as substrate (Johnston Citation2003). The assay mixture contained a final concentration of 0.4 mM benzoyl-L-tyrosine ethyl ester in 200 mM Tris, 200 mM NaCl, 10 mM CaCl2 and 0.2% (w/v) polyethylene glycol 6000 (PEG) (pH 7), incubated at 37°C. Assays were initiated by the addition of enzyme extract, and the release of benzoyl-tyrosine was measured at 256 nm over 8 min, and compared with a positive control of bovine pancreas trypsin dissolved in HCl.
Lipase activity was determined according to a modified method of Gjellesvik et al. (Citation1992) using 4-nitrophenyl caproate (Sigma) dissolved in ethanol as substrate. The assay mixture contained a final concentration of 2.5 mM 4-NPC in 6 mM sodium taurocholate, 500 mM Tris and 100 mM NaCl buffer (pH 8.5), incubated at 37°C. Assays were initiated by the addition of enzyme extract, and the release of nitrophenol was measured at 405 nm. One unit of the enzyme was defined as 1 µmol of the product released per minute at 37°C.
Hepatopancreatic amylase activity was determined using the method of Biesiot and Capuzzo (Citation1990). The assay mixture of 15 mg/ml starch in 100 mM phosphate buffer (pH 5.5) containing 50 mM NaCl was pre-incubated for 10 min, and the assay was initiated with the addition of enzyme extract. Following incubation for 30 min at 37°C, di-nitrosalicylic acid reagent (DNS) was added, and the assay solution was boiled for 10 min. Assay tubes were quickly cooled on ice, and the absorbance was read at 540 nm. A standard curve for maltose was used to calculate the amount of reducing sugar liberated per minute. One unit of the enzyme was defined as 1µ mole of the product released per minute at 37°C.
α- and ß-Glucosidase activities were determined using the substrates p-nitrophenylα-D-glucopyranoside and p-nitrophenyl ß-D glucopyranoside, respectively (Johnston Citation2003). Each assay mixture contained a final concentration of 4 mM substrate in 200 mM Tris, 200 mM NaCl, 10 mM CaCl2 and 0.2% (w/v) polyethylene glycol 6000 (pH 4.5), incubated at 37°C. Assays were initiated with the addition of enzyme extract. Aliquots of assay mixture were then removed at time intervals and added to 1 M Na2CO3 (pH 11), to terminate the reaction. Liberation of p-nitrophenol was measured at 400 nm.
The free amino acid content (mg/g tissue) in the hepatopancreas extract was determined using ninhydrin reagent (Yemm et al. Citation1955) in citrate buffer. Tyrosine was used as the standard amino acid.
Histological analysis
The hepatopancreas of the different experimental groups was fixed in 10% Neutral Buffer Formalin (NBF) for histological analysis. The sections were maintained at 5 μm thickness and stained by routine histological procedures using Meyer’s haematoxylin and eosin. The sections were observed under light microscope (Carl Zeiss, Model No. 49573) at 40× magnification using Axiovision 4 software.
Statistical analysis
Each assay was done in triplicate. The results were treated by one-way analysis of variance (ANOVA) using SPSS v. 16. Comparison among different treatments was made using Duncan’s Multiple Range Test (DMRT). All analyses were performed considering a level of 95% of confidence (p < .05).
Results
Hepatosomatic index and tail weight index
The hepatosomatic index of the group maintained at complete starvation (T2) was significantly reduced, compared to that fed on clam (T1) and formulated feed (T3) (). Although the tail weight index of T3 group was reduced compared to T1 and T2, the reduction was not statistically significant (p < .05).
Table 2. Tissue biochemical status, hepatosomatic index (HSI) and tail weight index (TWI) of different experimental groups.
Biochemical analysis
The three experimental groups exhibited a significant difference in the lipid content of hepatopancreas (). The group fed formulated feed (T3) exhibited the highest value followed by the clam fed group (T1). The lowest hepatopancreas lipid content was found in the starved group (T2). The protein content of the hepatopancreas was significantly higher in T1 compared to T2 and T3. There was no significant difference in the hepatopancreas protein content of T2 and T3. The protein and lipid contents in the muscle did not reveal any significant difference between the different experimental groups.
Enzyme assays and free amino acid content
No significant difference was observed in the hepatopancreas lipase activity in the different experimental groups. Hepatopancreas protease activity in the starved group (T2) was significantly higher (p < .05) compared to T1 and T3 (). A similar trend was observed in trypsin and chymotrypsin activities, with T2 exhibiting significantly higher values, as compared to T1 and T3 (). Amylase activity was higher in the group fed with formulated diet (T3) compared to T1 and T2 (). A similar trend was exhibited by α-Glucosidase, whereas no significant difference was observed in hepatopancreas ß-Glucosidase activity ().
Figure 1. Hepatopancreas protease and lipase activity in the different experimental groups. Values in the same column having different superscript are significantly different. Data expressed as Mean ± SE. One unit of protease expressed as units micromoles of tyrosine released/min. One unit of lipase expressed as micromoles of p-nitrophenol released/min.
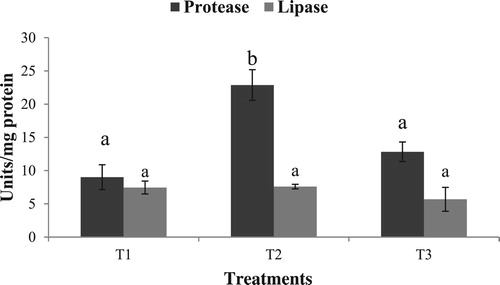
Figure 2. Hepatopancreas trypsin and chymotrypsin activity in the different experimental groups. Values in the same series having different superscript are significantly different. Data expressed as Mean ± SE. One unit of trypsin expressed as micromoles of p-nitroaniline released/min. One unit of chymotrypsin expressed as micromoles of benzoyl-tyrosine released/min.
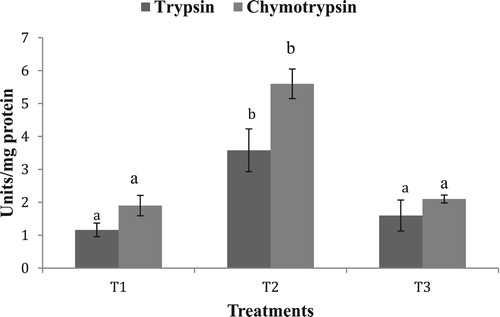
Figure 3. Hepatopancreas amylase activity in the different experimental groups. Value having different superscript is significantly different. Data expressed as Mean ± SE. One unit of amylase expressed as micromoles of maltose released/min.
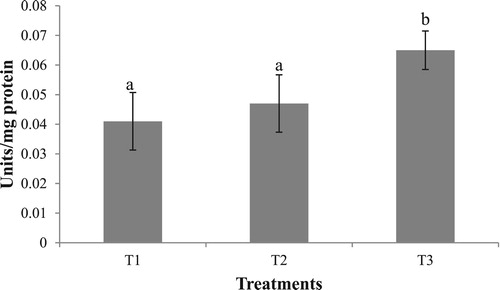
Figure 4. Hepatopancreas α Glucosidase and ß Glucosidase activity in the different experimental groups. Values having different superscript are significantly different. Data expressed as Mean ± SE. One unit of α Glucosidase expressed as micromoles of p-nitrophenol released/min. One unit of ß Glucosidase expressed as micromoles of p-nitrophenol released/min.
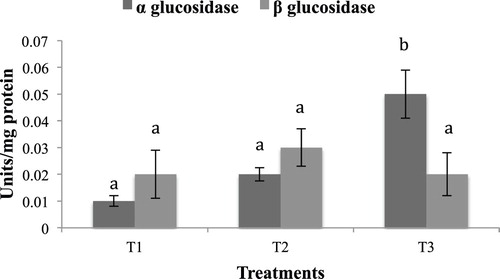
Free amino acid determination of the hepatopancreas revealed a significantly higher content of free amino acid in the group fed with clam (T1), compared to T2 and T3 (). There was no significant difference in the free amino acid content of the hepatopancreas in the groups T2 and T3.
Histological analysis
Histological examination of the hepatopancreas of T3 group revealed increased vacuolation caused by hypertrophy of B cells, with one or more large vacuoles tending to coalesce into single larger ones (). The hypertrophy was visible along the longitudinal as well as the transverse sections of hepatopancreas in the group fed the formulated feeds. The lumen walls of hepatopancreas were also considerably thicker in T3, compared to T1 and T2.
Figure 6. Histological micrographs of digestive gland tubules of P. homarus juveniles reared on different feeding regimes of fresh clam (transverse section-A, lateral section-B), starvation (transverse section-C, lateral section-D) and pelletized feed (transverse section E, lateral section F). Hypertrophy of B-cells (HB) in the group fed pellet feed (T3) can be observed both in pictures E and F. T3 also exhibits reduced lumen area (RL) due to thickening of the lumen wall and loses the typical ‘star shape’ (S), which is clearly observed in group fed with clam meat (A).
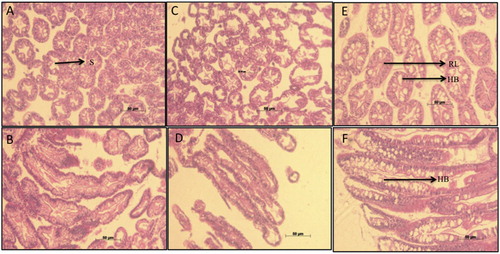
Discussion
A common factor in crustaceans is their constant feeding activity during intermolt and fasting, which begins a few days before ecdysis. Artificially induced starvation reveals the metabolic preference of the animal to use a particular body reserve as fuel to sustain itself (Guderley et al. Citation2003). In the present experiment, we did not focus our attention on the growth performance of the animal and economic indicators like feed conversion ratio and protein efficiency ratio. Instead, the study was aimed at revealing the changes that take place in the animal’s digestive capacity and the histological architecture of the digestive organ when it is shifted from wet feeds to formulated dry feeds. This may lead us to reveal the metabolic bottleneck in spiny lobsters, which limits the utilization of the nutrients from formulated feeds. Therefore, we compared the lobsters fed with formulated feed with a group fed with freshly cut clams (wet feed).
The body indices of aquatic animals are indicators of wellbeing and fitness (Jensen Citation1979; Delahunty and de Vlaming Citation1980). These indices are signs of the metabolic stores of the body and exhibit fluctuation in response to nutritional status (Larsen et al. Citation2001). The present study revealed significant variations in the HSI but no significant difference in TWI of the different experimental groups. Starvation severely affected the hepatopancreas and caused a reduction in the hepatosomatic index, probably due to mobilization of the nutrient reserves, as suggested by Sacristan et al. (Citation2016). We observed predominant energy utilization from the hepatopancreas and no significant changes in the lipid and protein content in the tail muscle. This may explain why no significant difference was observed in TWI among the treatments.
Starvation gives indications about energy resources utilized by crustaceans and provides clues to biochemical pathways involved in these processes. We found that during starvation, both lipid and protein content in the hepatopancreas were reduced significantly. Several decades ago, it was proposed that in crustaceans, the primary source of energy is protein (New Citation1976). However, numerous studies that followed, established that both lipids and protein might be used as fuel reserves from the body. Hervant and Renault (Citation2002) found mobilization of more than one fuel reserve from crustaceans. Similar results were also observed by Schafer (Citation1968) supporting that both lipids and proteins might be mobilized during starvation. The preferential use of both proteins and lipids has also been confirmed in Peneaus esculentus (Barclay et al. Citation1983).
Lobsters have generally shown to tolerate high dietary levels of lipid (Tolomei et al. Citation2003; Barclay et al. Citation2006). Also, feeding of raw molluscan meat has been associated with higher lipid content in the hepatopancreas (Ward and Carter Citation2009), an observation contrary to the present findings. However, on an as-fed basis, in the present experiment, the lipid content of the formulated feed was much higher than in clam meat. This, exacerbated by compromised digestion of lipids, which is established in other lobster species (Glencross et al. Citation2001), may have also caused the increase in the lipid content of the hepatopancreas of the T3 group.
Proteolytic activity (total protease, trypsin, chymotrypsin) in the starved group (T2) was significantly higher (p < .05) compared to T1 and T3 groups. In contrast to our finding, a 5-days starvation study in L. vannamei revealed that proteases reduce their activity as well as gene expression during starvation (Muhlia-Almazán and García-Carreńo Citation2002), which may be a measure to conserve the body enzymes (and thus prevent expenditure on synthesis) as a response to short term starvation stress in face of higher metabolic rate of the shrimps, as compared to lobsters. It may however be argued that during more prolonged starvation, as in the present study, when cell internal reserves must be mobilized, it is possible that the enzymes are produced or activated and finely regulated inside the cells (Sanchez-Paz et al. Citation2006). However, the processes and mechanisms whereby this occurs are still unknown. Indeed it will be interesting to decipher the temporal response of digestive enzymes to artificially induced starvation. There was no significant difference in the activities of trypsin and chymotrypsin in the treatments T1 and T3, fed fresh clam and formulated diet, respectively. Although lobsters have the capacity to differentially regulate the major proteases (Hoyle Citation1973) and compensate for low digestibility of formulated feed (Perera et al. Citation2005), Simon (Citation2009) also observed no significant difference in the specific activity (units/mg protein) of hepatopancreas trypsin enzyme in J. edwardsii fed with fresh mussel flesh and formulated feed. This implies that major limitation is not in protein digestion but in the absorptive or post-absorptive processes that are more severely affected by formulated feeds.
Although a reduction in the lipid content of the hepatopancreas of the starved group was observed, lipase activity showed no significant difference among the treatment groups. Rivera-Pérez and García-Carreño (Citation2011) showed that there are two types of lipases in L. vannamei, a digestive lipase and an intracellular lipase (lysosomal), both present in the hepatopancreas. The digestive lipase is negatively regulated during fasting, whereas the intracellular lipase is positively regulated during starvation. Since the hepatopancreas acts as a single organ that is involved in digestion, absorption, storage and mobilization of nutrients, it may be suggested that the two types of lipases, which are oppositely regulated by starvation, may have cancelled each other’s effects on the overall lipase activity of the hepatopancreas in T2.
Amylase activity was higher in the group fed with formulated diet (T3) compared to T1 and T2. According to Johnston and Freeman (Citation2005), digestive enzyme activity is closely related to dietary components. Thus, high proteinase, carbohydrase and lipase activities reflect a diet rich in protein, starch or cellulose and lipids, respectively (Johnston and Yellowlees Citation1998; Johnston Citation2003). The feed used in the present study had soyabean and wheat flour as the major ingredients rich in carbohydrate. The nitrogen-free extract of the feed was much higher than clam meat on an as fed basis, which might have caused an increase in the amylase activity. A similar increase in the amylase activity due to a carbohydrate-rich diet was observed in mud crab (Pavasovic et al. Citation2004). The difference observed in the hepatopancreas amylase activity may also be attributed to the difference in biochemical nature of the predominant carbohydrate in the diets T1 and T3. In the present experiment, the clam, which has carbohydrate in predominantly glycogen form, is biochemically different to the carbohydrate present in the formulated feeds, which is predominantly starch based. Rodríguez-Viera et al. (Citation2014) have shown that in vitro hydrolysis rates of different carbohydrate sources vary significantly in P. argus and are generally higher for starch-based substrates. Rodríguez-Viera et al. (Citation2017) further demonstrated that after 30 hours of feeding, the amylase activity in the gastric secretions are increased when P. argus is fed with dietary starch as compared to feeding with fresh fish. These observations support our finding of increased amylase activity in the T3 group.
Protein turnover can be divided into its constituent processes, protein synthesis, protein growth and protein degradation. At any particular time, protein growth is the net balance between protein synthesis and protein degradation. A higher content of free amino acids in the group fed with fresh clam meat (T1) indicates that more amino acids are available for protein synthesis. Since the hepatopancreas is an organ exhibiting high protein synthesis and degradation (Mente Citation2002), the higher free amino acid content seen along with higher protein content of hepatopancreas in the clam fed group is an indication of a physiologically active hepatopancreas and a healthy animal condition. Formulated feed fed animals had significantly lower free amino acid content in the hepatopancreas. This, seen along with a lower protein content of the hepatopancreas indicates a difficulty in metabolizing the amino acids absorbed from the feed.
Histological examination of the hepatopancreas revealed increased vacuolation in the group fed the formulated feeds. Simon (Citation2009) observed similar results and attributed it to the increased number of B-cells as an attempt to eliminate the waste products (Brunet et al. Citation1994) in response to poor digestibility of feeds (Al-mohanna and Nott Citation1987). The vacuolation may also be due to difficulty in mobilizing the nutrients absorbed from formulated feeds to the different body tissues for energy and growth. The thickened lumen walls of the hepatopancreas in T3 indicate intensified digestive effort for formulated diets compared to that for raw clam meat.
In the present study, biochemical responses and the histological changes in hepatopancreas due to formulated diets arose after only 20 days of feeding. Although similar changes have been observed in other lobster species before (Pavasovic et al. Citation2004; Simon Citation2009), this occurs usually under prolonged periods of feeding of formulated feeds. In the present study, the observed changes being promptly induced may be because the formulated feed was a soybean-based diet, which is considered inferior ingredient for spiny lobsters as compared to animal protein meals (Jones et al. Citation1996; Ward et al. Citation2003).
Conclusion
Our study gives an insight into the pattern of utilization of nutrient reserves in the tropical spiny lobster P. homarus under conditions of food limitation. Both proteins and lipids are mobilized equally as the main energy sources during starvation. Results of enzyme and histological studies from animals fed with formulated diet demonstrated a general difficulty in the absorption and mobilization of nutrients from the feed. This information will form a basis while formulating feed for farming of this species.
Acknowledgements
The authors are grateful to Director, ICAR-Central Marine Fisheries Research Institute, India for providing support and facilities to carry out this research.
Disclosure statement
No potential conflict of interest was reported by the authors.
ORCID
Adnan Gora http://orcid.org/0000-0001-6207-9410
References
- Al-Mohanna SY., Nott JA. 1987. R-cells and the digestive cycle in Penaeus semisulcatus (Crustacea:Decapoda). Mar. Biol. 95:129–137. doi: 10.1007/BF00447494
- Al-Mohanna SY, Nott JA, Lane DJW. 1985. Mitotic E-and secretory F-cells in the hepatopancreas of the shrimp Penaeus semisulcatus (Crustacea: Decapoda). J Mar Biol Assoc United Kingdom. 65(4):901–910. doi: 10.1017/S0025315400019408
- Barclay MC, Dall W, Smith DM. 1983. Changes in lipid and protein during starvation and the moulting cycle in the tiger prawn, Penaeus esculentus Haswell. J Exp Mar Biol Ecol. 68(3):229–244. doi: 10.1016/0022-0981(83)90055-2
- Barclay MC, Irvin SJ, Williams KC, Smith DM. 2006. Comparison of diets for the tropical spiny lobster Panulirus ornatus: astaxanthin-supplemented feeds and mussel flesh. Aquacult Nutr. 12(2):117–125. doi: 10.1111/j.1365-2095.2006.00390.x
- Barker PL, Gibson R. 1977. Observations on the feeding mechanism, structure of the gut, and digestive physiology of the European lobster Homarus gammarus (L.)(Decapoda: Nephropidae). J Exp Mar Biol Ecol. 26(3):297–324. doi: 10.1016/0022-0981(77)90089-2
- Biesiot PM, Capuzzo JM. 1990. Changes in digestive enzyme activities during early development of the American lobster Homarus americanus Milne Edwards. J Exp Mar Biol Ecol. 136(2):107–122. doi: 10.1016/0022-0981(90)90190-N
- Brunet M, Arnaud J, Mazza J. 1994. Gut structure and digestive cellular processes in marine Crustacea. Oceanogr Mar Biol. 32:335–367.
- Cox SL, Johnston DJ. 2003. Feeding biology of spiny lobster larvae and implications for culture. Rev Fish Sci. 11(2):89–106. doi: 10.1080/713610924
- Delahunty G, de Vlaming VL. 1980. Seasonal relationships of ovary weight, liver weight and fat stores with body weight in the goldfish, Carassius auratus (L.). J Fish Biol. 16(1):5–13. doi: 10.1111/j.1095-8649.1980.tb03683.x
- Folch J, Lees M, Sloane Stanley GH. 1957. A simple method for the isolation and purification of total lipids from animal tissues. J Biol Chem. 226(1):497–509.
- Frings CS, Dunn RT. 1970. A colorimetric method for determination of total serum lipids based on the sulfo-phospho-vanillin reaction. Am J Clin Pathol. 53(1):89–91. doi: 10.1093/ajcp/53.1.89
- Gjellesvik DR, Lombardo D, Walther BT. 1992. Pancreatic bile salt dependent lipase from cod (Gadus morhua): purification and properties. Biochim Biophys Acta Lipids Lipid Metab. 1124(2):123–134. doi: 10.1016/0005-2760(92)90088-D
- Glencross B, Smith M, Curnow J, Smith D, Williams K. 2001. The dietary protein and lipid requirements of post-puerulus western rock lobster, Panulirus cygnus. Aquaculture. 199:119–129. doi: 10.1016/S0044-8486(01)00520-8
- Guderley H, Lapointe D, Bédard M, Dutil JD. 2003. Metabolic priorities during starvation: enzyme sparing in liver and white muscle of Atlantic cod, Gadus morhua L. Comp Biochem Physiol A: Mol Integ Physiol. 135(2):347–356. doi: 10.1016/S1095-6433(03)00089-8
- Hervant F, Renault D. 2002. Long-term fasting and realimentation in hypogean and epigean isopods: a proposed adaptive strategy for groundwater organisms. J Exp Biol. 205(14):2079–2087.
- Hoyle RJ. 1973. Digestive enzyme secretion after dietary variation in the American lobster (Homarus americanus). J Fish Board Canada. 30(11):1647–1653. doi: 10.1139/f73-264
- Jensen AJ. 1979. Energy content analysis from weight and liver index measurements of immature pollock (Pollachius virens). J Fish Board Canada. 36(10):1207–1213. doi: 10.1139/f79-174
- Johnston DJ. 2003. Ontogenetic changes in digestive enzymology of the spiny lobster, Jasus edwardsii Hutton (Decapoda, Palinuridae). Mar Biol. 143(6):1071–1082. doi: 10.1007/s00227-003-1154-0
- Johnston D, Freeman J. 2005. Dietary preference and digestive enzyme activities as indicators of trophic resource utilization by six species of crab. Biol Bull. 208(1):36–46. doi: 10.2307/3593099
- Johnston D, Melville-Smith R, Hendriks B. 2007. Survival and growth of western rock lobster Panulirus cygnus (George) fed formulated diets with and without fresh mussel supplement. Aquaculture. 273(1):108–117. doi: 10.1016/j.aquaculture.2007.09.020
- Johnston DJ, Ritar AJ, Thomas CW. 2004. Digestive enzyme profiles reveal digestive capacity and potential energy sources in fed and starved spiny lobster (Jasus edwardsii) phyllosoma larvae. Comp Biochem Physiol B Biochem Mol Biol. 138(2):137–144. doi: 10.1016/j.cbpc.2004.02.013
- Johnston DJ, Yellowlees D. 1998. Relationship between dietary preferences and digestive enzyme complement of the slipper lobster Thenus orientalis (Decapoda: Scyllaridae). J Crustac Biol. 18(4):656–665. doi: 10.2307/1549140
- Jones PL, De Silva SS, Mitchell BD. 1996. Effects of replacement of animal protein by soybean meal on growth and carcass composition in juvenile Australian freshwater crayfish. Aquacult Int. 4(4):339–359. doi:10.1007/BF00120950.
- Juinio MAR, Cobb JS. 1992. Natural diet and feeding habits of the postlarval lobster Homarus americanus. Mar Ecol Prog Ser. 85:83–91. doi: 10.3354/meps085083
- Larsen DA, Beckman BR, Dickhoff WW. 2001. The effect of low temperature and fasting during the winter on metabolic stores and endocrine physiology (insulin, insulin-like growth factor-I, and thyroxine) of coho salmon, Oncorhynchus kisutch. Gen Comp Endocrinol. 123(3):308–323. doi: 10.1006/gcen.2001.7677
- Lowry OH, Rosebrough NJ, Farr AL, Randall RJ. 1951. Protein measurement with the Folin phenol reagent. J Biol Chem. 193(1):265–275.
- Mente E, Coutteau P, Houlihan D, Davidson I, Sorgeloos P. 2002. Protein turnover, amino acid profile and amino acid flux in juvenile shrimp Litopenaeus vannamei: effects of dietary protein source. J Exp Biol. 205(20):3107–3122.
- Muhlia-Almazán A, García-Carreńo FL. 2002. Influence of molting and starvation on the synthesis of proteolytic enzymes in the midgut gland of the white shrimp Penaeus vannamei. Comp Biochem Physiol B Biochem Mol Biol. 133(3):383–394. doi: 10.1016/S1096-4959(02)00163-X
- New MB. 1976. A review of dietary studies with shrimp and prawns. Aquaculture. 9:101–144. doi: 10.1016/0044-8486(76)90055-7
- Ong BL, Johnston D. 2006. Influence of feeding on hepatopancreas structure and digestive enzyme activities in Penaeus monodon. J Shellfish Res. 25(1):113–121. doi: 10.2983/0730-8000(2006)25[113:IOFOHS]2.0.CO;2
- Pavasovic M, Richardson NA, Anderson AJ, Mann D, Mather PB. 2004. Effect of pH, temperature and diet on digestive enzyme profiles in the mud crab, Scylla serrata. Aquaculture. 242(1–4):641–654. doi: 10.1016/j.aquaculture.2004.08.036
- Perera E, Fraga I, Carrillo O, Díaz-Iglesias E, Cruz R, Báez M, Galich GS. 2005. Evaluation of practical diets for the Caribbean spiny lobster Panulirus argus (Latreille, 1804): effects of protein sources on substrate metabolism and digestive proteases. Aquaculture. 244(1–4):251–262. doi: 10.1016/j.aquaculture.2004.11.022
- Perera E, Moyano FJ, Díaz M, Perdomo-Morales R, Montero-Alejo V, Rodríguez-Viera L, Alonso E, Carrillo O, Galich GS. 2008. Changes in digestive enzymes through developmental and molt stages in the spiny lobster, Panulirus argus. Comp Biochem Physiol B Biochem Mol Biol. 151(3):250–256. doi: 10.1016/j.cbpb.2008.07.005
- Rao GS, George RM, Anil MK, Saleela KN, Jasmine S, Kingsly HJ, Rao G. 2010. Cage culture of the spiny lobster Panulirus homarus (Linnaeus) at Vizhinjam, Trivandrum along the south-west coast of India. Indian J Fish. 57(1):23–29.
- Rivera-Pérez C, García-Carreño F. 2011. Effect of fasting on digestive gland lipase transcripts expression in Penaeus vannamei. Mar Genomics. 4(4):273–278. doi: 10.1016/j.margen.2011.07.002
- Rodríguez-Viera L, Perera E, Casuso A, Perdomo-Morales R, Gutierrez O, Scull I, Carrillo O, Martos-Sitcha JA, Garcia-Galano T, Mancera JM. 2014. A holistic view of dietary carbohydrate utilization in lobster: digestion, postprandial nutrient flux, and metabolism. PloS one. 9(9):e108875. doi: 10.1371/journal.pone.0108875
- Rodríguez-Viera L, Perera E, Montero-Alejo V, Perdomo-Morales R, García-Galano T, Martínez-Rodríguez G, Mancera JM. 2017. Carbohydrates digestion and metabolism in the spiny lobster (Panulirus argus): biochemical indication for limited carbohydrate utilization. PeerJ. 5:e3975. doi: 10.7717/peerj.3975
- Sacristán HJ, Ansaldo M, Franco-Tadic LM, Gimenez AVF, Greco LSL. 2016. Long-Term starvation and posterior feeding effects on biochemical and physiological responses of midgut gland of Cherax quadricarinatus juveniles (Parastacidae). PloS one. 11(3):e0150854. doi: 10.1371/journal.pone.0150854
- Sánchez-Paz A, García-Carreño F, Muhlia-Almazán A, Peregrino-Uriarte AB, Hernández-López J, Yepiz-Plascencia G. 2006. Usage of energy reserves in crustaceans during starvation: status and future directions. Insect Biochem Mol Biol. 36(4):241–249. doi: 10.1016/j.ibmb.2006.01.002
- Schafer HJ. 1968. Storage materials utilized by starved pink shrimp, Penaeus duorarum Burkenroad. FAO Fish. Rep. 57:393–403.
- Simon CJ. 2009. Digestive enzyme response to natural and formulated diets in cultured juvenile spiny lobster, Jasus edwardsii. Aquaculture. 294(3–4):271–281. doi: 10.1016/j.aquaculture.2009.06.023
- Simon CJ, James PJ. 2007. The effect of different holding systems and diets on the performance of spiny lobster juveniles, Jasus edwardsii (Hutton, 1875). Aquaculture. 266(1–4):166–178. doi: 10.1016/j.aquaculture.2007.02.050
- Simon CJ, Jeffs A. 2008. Feeding and gut evacuation of cultured juvenile spiny lobsters, Jasus edwardsii. Aquaculture. 280(1–4):211–219. doi: 10.1016/j.aquaculture.2008.05.019
- Smith DM, Williams KC, Irvin S, Barclay M, Tabrett S. 2003. Development of a pelleted feed for juvenile tropical spiny lobster (Panulirus ornatus): response to dietary protein and lipid. Aquacult Nutr. 9(4):231–237. doi: 10.1046/j.1365-2095.2003.00248.x
- Tolomei A, Crear B, Johnston D. 2003. Diet immersion time: effects on growth, survival and feeding behaviour of juvenile southern rock lobster, Jasus edwardsii. Aquaculture. 219(1–4):303–316. doi: 10.1016/S0044-8486(02)00507-0
- Walter HE. 1984. Proteases and their inhibitors. 2. 15. 2 method with haemoglobin, casein and azocoll as substrate. Method Enzym Anal. 5:270–277.
- Ward LR., Carter CG. 2009. An evaluation of the nutritional value of alternative lipid sources to juvenile southern rock lobster, Jasusedwardsii. Aquaculture. 296:292–298. doi: 10.1016/j.aquaculture.2009.08.025
- Ward LR, Carter CG, Crear BJ. 2003. Apparent digestibility of potential ingredients as protein sources in formulated feeds for the southern rock lobster Jasus edwardsii. In: Williams K.C., editor. The nutrition of juvenile and adult lobsters to optimize survival, growth and condition: final report of FRDC 2000/212. Canberra: Fisheries Research and Development Corporation; p. 40–49.
- Williams KC. 2007. Nutritional requirements and feeds development for post-larval spiny lobster: a review. Aquaculture. 263(1–4):1–14. doi: 10.1016/j.aquaculture.2006.10.019
- Yemm EW, Cocking EC, Ricketts RE. 1955. The determination of amino-acids with ninhydrin. Analyst. 80(948):209–214. doi: 10.1039/an9558000209