ABSTRACT
The shortage of organs for transplantation is a well-known issue of modern medicine. The domestic pig has proved to be most suitable for xenotransplantation purposes. It is necessary to modify the pig genome to eliminate the immunological differences resulting from its phylogenetic remoteness from humans. We present a generation of pigs with human CD55 and ULBP1 gene knockout. Both modifications were introduced using the CRISPR/Cas9 system. A mixture of Cas9-D10A mRNA, a pair of sgRNAs and the pCD55 donor vector were introduced into the pronucleus of the fertilized porcine oocyte. After microinjection the three surrogates delivered a total of 13 piglets. The analysis showed four piglets with indels in the targeting site of exon 2 of the pULBP1 gene. All four pigs were altered in a biallelic manner, showing different sequences in each mutant allele. One piglet also showed one allele interrupted with a CD55-expressing cassette. The analyses confirmed the integration and the expression of the CD55 transgene in the targeted site. The human serum cytotoxicity test results showed that the highest viability of modified cells was 84.42% compared to the control. The cytometric analysis suggests that the CD55-expressing cassette was integrated with the genome in the transcription active site.
1. Introduction
The problem of modern transplantation lies primarily in the lack of enough organs, tissues and cells for use in transplantation. Despite the increasing knowledge in the field of transplantology, the organ shortage means that the number of patients waiting for a transplant is constantly increasing. In connection with the search for new sources of organs, more and more scientists focus on the use of transgenic animals for xenotransplantation. The selection of a potential donor is primarily associated with the presence of antigens on the surface of the cells. Antigens influence the rejection of a transplant, and their compatibility in the donor-recipient system is of key importance in transplantology. In xenotransplantation, where the recipient is the human body, non-human primates (NHPs) as well as the domestic pig are considered as donors. Due to the distant phylogenetic relationship between domestic pigs and humans, the problem of the occurrence of diseases caused by donor pathogens is avoided. Therefore, the domestic pig (Sus scrofa domestica) is used as a potential donor of organs, tissues and cells for humans. The pig has anatomical and physiological similarities to the human body, and breeding is easy and relatively cheap. However, direct grafts in the pig-human system are not possible due to high immunological incompatibility, which includes the occurrence of different major histocompatibility complex (MHC) antigens specific for each species – human leukocyte antigens (HLA) and swine leukocyte antigens (SLA). These differences cause the recipient's immune system to recognize the antigens found on xenograft cells, resulting in activation of the transplant rejection process (Cooper Citation2012; Cooper et al. Citation2016; Niu et al. Citation2017).
In the case of transplantation of organs, tissues or cells from a donor of another species, the recipient's immune system is activated. The mechanisms of rejection may be different, which is influenced by various triggering factors. Depending on the type and mechanism of the rejection process and, most importantly, its occurrence, we can distinguish four types of xenotransplant rejection. HAR (hyperacute rejection) is observed within a few minutes of the transplant and leads to organ failure. The main cause of hyperacute rejection is the activation of the complement system through xenoreactive antibodies against MHC molecules and specific endothelial surface antigens. The α1,3Gal antigen is located on glycoproteins and glycolipids of pig endothelial cells. Xenoreactive antibodies recognize the immunogenic molecule galactosyl-1,3-galactose, which is located at the end of the porcine Gal antigen (Brostoff Citation2012). Gal (Galα1,3Gal) is a result of the reaction of adding a galactose molecule to N-acetyllactosamine by means of α1,3 glycosidic linkage. This reaction is catalyzed by the enzyme α1,3-galactosyltransferase (GGTA1). The HAR process can be reduced by using as donors genetically modified pigs that do not express GGTA1. In another strategy to reduce α1,3-Gal epitopes on the surface of swine cells, modifications consisting of the expression of α1,2-fucosyltransferase (α1,2-FT) were used. Both enzymes compete for the same substrate, which consequently leads to a reduction in the number of Gal epitopes on the surface of the donor's cells. Another approach used to overcome HAR is the expression of complement regulatory proteins, such as CD46 (membrane cofactor protein, MCP), CD55 (decay acceleration factor, DAF) and CD59 (membrane inhibitor of reactive lysis, MIRL) (Niemann et al. Citation2001). In the case of hyperacute rejection suppression, acute humoral xenograft rejection (AHXR) occurs, which is also called delayed xenograft rejection (DXR). The course of DXR rejection involves attachment of xenoactive antibodies to antigens located on the surface of endothelial cells lining the vascular lumen and activation of the antibody-dependent cytotoxic response. It is assumed that this rejection is not dependent on the complement system, but on antibodies, macrophages and NK cells. The result of their cytotoxic action is the formation of a clot and narrowing of the vascular lumen that causes rejection of the transplanted organ (Yang and Sykes Citation2007). Due to the priority role of hyperacute rejection and acute vascular rejection, acute cellular rejection (ACR) is a reaction that is relatively rare in vivo. In the course of acute cellular rejection, two types of immune reactions play a dominant role, i.e. a delayed hypersensitivity reaction, in which the fundamental function is played by CD4+ T lymphocytes, and a cytotoxicity reaction of T lymphocytes. They secrete lymphokines that increase the permeability of blood vessels, as well as the accumulation of inflammatory mononuclear cells, i.e. lymphocytes and macrophages. In the case of T lymphocytes’ cytotoxicity reaction, a specific cytostatic T cell lymphocyte (CD8+ T cell lymphocyte) is recognized on the transplant cell, resulting in death due to apoptosis or lysis (Ganji and Broumand Citation2007; Brostoff Citation2012).
There are many methods for obtaining transgenic animals. By using nucleases for genome editing, the creation of transgenic animals has become more efficient and more precise. The nucleases used for genome editing include zinc finger nucleases (ZFNs), transcription activator-like effector nucleases (TALENs), and the Cas9 protein in a system called CRISPR/Cas (clustered regularly interspaced short palindromic repeats/CRISPR-associated). The CRISPR/Cas technique is characterized by high specificity and low toxicity. This system is one of the types of resistance of archaea and bacteria to the introduction of exogenous nucleic acids. The CRISPR/Cas method for binding to the target DNA sequence involves a short, single-stranded and complementary RNA molecule, sgRNA (single-guide RNA), and for hydrolysis of the target sequence, the Cas9 protein. Imperfect repair of double-strand breaks (DSBs) causes modification of the targeted site. The CRISPR/Cas9 technique, thanks to its relative simplicity in experimental design and high specificity, is one of the most promising methods for obtaining transgenic animals. In addition, the ability to introduce several or even dozens of genes allows multitransgenic animals to be obtained (Ma et al. Citation2014; Xiao-Jie et al. Citation2014; Zhang et al. Citation2014). The generation of ULBP-1 knock-out pigs expressing CD55 is a necessary step for their use as organ donors for humans. CD55 is a membrane protein expressed on the surface of all blood cells, vascular endothelial cells and various other tissue cells. It plays a role in T cell and macrophage activation and protects host cells from the destructive action of autologous complement (Delves Citation1998). UL16-binding protein 1 (ULBP1) – a cell surface glycoprotein – is a ligand of natural killer group 2, member D (NKG2D), an immune system-activating receptor on NK cells and T cells. The elimination of porcine ligands for receptors activating NK cells is a step to prevent xenograft rejection, in which NK cells are involved (Lilienfeld et al. Citation2006; Hryhorowicz et al. Citation2017; Zeyland et al. Citation2018). In this study, we present a generation of pigs with human CD55 gene and ULBP1 gene knockout (-/- ULBP1, +/− CD55) modified by CRISPR-Cas9.
2. Materials and methods
2.1. Cas9-D10A/gRNA and donor template design
The design of two sgRNAs targeting the region of exon 2 of the pULBP1 gene has been described before (Zeyland et al. Citation2018). The donor vector (pCD55) consisted of a left arm comprising the 800 bp sequence of exon 2 of the porcine ULBP1 gene, the CMV promoter sequence fused to the human CD55 coding sequence, human polyA from the GH gene and the right arm comprising 800 bp of exon 2 of the porcine ULBP1 gene inserted into the pUC57 plasmid. The donor vector was provided by Sigma-Aldrich ().
Figure 1. Scheme of the pCD55 donor vector based on pUC57 vector. The donor construct was designed to incorporate into the ULBP1 gene. The hydrolysis site within the ULBP1 gene has been previously described (Zeyland et al. Citation2018, figure 1A).
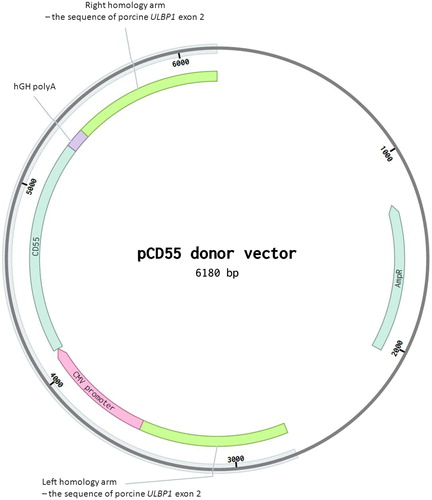
2.2. Production of porcine zygotes
To produce a sufficient number of zygotes for transfection, the standard superovulation protocol was utilized (Jura et al. Citation2007). Zygotes were flushed out from the oviducts of donor gilts under full anesthetic control. Subsequently, after collection of zygotes was completed, they were morphologically evaluated. Only zygotes with intact cytoplasm and two visible polar bodies were subjected to the transfection procedure performed by pronucleus co-microinjection of the mixture of donor vector, gRNAs and mRNA of Cas9-D10/A. Prior to the transfection procedure, zygotes were centrifuged (15,000 g/3 min) to visualize the pronuclei. Centrifuged zygotes were transferred into the manipulation chamber filled with PBS containing 20% fetal bovine serum (FBS) (Sigma, USA). The mixture was injected into one of the visualized pronuclei (up to the moment of its enlargement of about 50%) under an inverted microscope equipped with Nomarski’s optics and two mechanical micromanipulation units. The concentration of Cas9-D10A mRNA was 3.5 ng/μl. The concentration of each gRNA was 3 ng/μl and the concentration of the donor vector was 2 ng/μl. After completion of the transfection procedure zygotes were transferred into another chamber containing manipulation medium and morphological evaluation was performed. For the procedure of transfer into oviducts of synchronized surrogates, positively checked for signs of estrus, only zygotes with no morphological changes in the cytoplasm were selected. Transfected zygotes were transferred into the fallopian tubes of recipient gilts under full surgical anesthesia. The oviducts were pulled out through a 2–3 cm-long incision made along the white line. Transfected zygotes suspended in PBS supplemented with 20% FCS were expelled into one oviduct by the insertion of a fine plastic cannula connected to a Hamilton syringe. Recipient gilts were checked for pregnancy after 30 days.
2.3. CD55 transgene integration and Cas9/gRNA mutation screening
Genomic DNA was extracted from ear biopsy specimens of potentially genetically modified pigs using a Kapa Express Extract DNA Extraction Kit with PCR ReadyMix (KAPA BIOSYSTEMS). Analysis of CD55 transgene integration included PCR with two pairs of primers. Both F primers were complementary to the CMV promoter sequence, and both R primers were complementary to the CD55 coding sequence. The first PCR product of 332 bp was formed using the dCD55-F1 (5′-GTCAGATCCGCTAGCGCTAC-3′) and dCD55-R1 (5′-CGCAGCTACGATTGCAGAAC-3′) primers, whereas the second product of 547 bp was formed using the dCD55-F2 (5′-TCATATGCCAAGTACGCCCC-3′) and dCD55-R2 (5′-TTGTACGGCCTTCCAAAGCT-3′) primers. PCR was performed under the following conditions: 94 °C for 180 s, followed by 35 cycles of 94 °C for 30 s, 58 °C for 45 s, 72 °C for 30 s, and a final extension at 72 °C for 360 s.
2.4. Homology directed repair analysis
To confirm HDR-mediated transgene integration, PCR with two pairs of primers was used. Both primers were complementary to the homologous sequence of the ULBP1 gene and to the CD55 donor sequence. Amplification with primers UCD55-F1 (5- GCACCCAACTCTTTTTGGTCC-3′)/UCD55-R1 (5′-ACCGTAAGTTATGTAACGCGGA-3′) and UCD55-F4 (5′-GGGACGCTAGTAACCATGGG-3′)/UCD55-R4 (5′-CCTCGTGTTTAGGGTCAGCA-3′) results in products of 985 and 1076 bp, respectively, only in the case of successful HDR-mediated CD55 transgene integration into the allele of the ULBP1 porcine gene. Cas9/gRNA mutation screening and sequencing were performed as described previously (Zeyland et al. Citation2018).
2.5. Skin fibroblast isolation and cell cultivation
In order to isolate skin fibroblast cells, skin fragments of pig were obtained to establish and conduct primary cultures. The procedure of fibroblast tissue isolation included rinsing of sterile fragments in Hanks’ balanced salt solution with the addition of antibiotics (2%). The tissue was then placed in subsequent vessels for thorough cleaning. After purification, the tissues were cut and digested in 5 ml of trypsin-EDTA solution (0.25% trypsin, 0.02%EDTA). The cell sediment was suspended in Dulbecco’s modified eagle medium with 10% fetal bovine serum (FBS) and 1% antibiotic/antimycotic. The culture vessels were placed in an incubator under standard conditions: 37°C and 5% CO2. The cells were grown until 80–90% confluence was achieved. After reaching the appropriate confluence, the passage was made. According to this procedure, commercial prostate cancer cells (PC-3, ATCC CRL-1435) were cultivated.
2.6. CD55 transgene expression by reverse transcription
The template in the reverse transcription reaction was RNA isolated from the fibroblasts. The quality and concentration of the preparations were evaluated both by electrophoresis and spectrophotometry. The cDNA synthesis was performed using the Synthesis Kit SuperScript VILO cDNA (Thermo Fisher) according to the manufacturer's instructions (25°C for 10 min; 42°C for 60 min; 85°C for 5 min). In addition, a positive control was performed by PCR on the template of the obtained cDNA with bACT-FCD (5′-CCAACTGGGACGACATGG AG-3′) and bACT-RCD (5′-AGGTCCAGACGCAGGATGGC-3′) primers specific for the β-actin cDNA (300 bp) as well as a negative control on the template of total RNA with bACT-RGE (5′-CGCACCACTGGCATTGTCA-3′) and bACT-FGE (5′-CATGCCTGAGAGGCCGACA-3′) primers specific for the genomic sequence of β-actin (445 bp) in order to confirm purity of RNA preparations. The reaction conditions were as follow: 94°C–5 min, 94°C–30 s, 56°C–30 s, 72°C–1 min, 30 cycles, 72°C–5 min. Finally analysis of CD55 transgene expression at the mRNA level was performed on the cDNA template by PCR with the CD55ExF1 (5′-GTGGAATATGAGTGCCGTCCA-3′) and CD55ExR1 (5′-GTACTCCGTGTTGCTTGAGCA-3′) primers. The total reaction volume was 20 μl containing 2×PCR Master Mix, 10 pmol of each primer, and 100 ng of cDNA template. Amplifications were performed on a PTC-100 Thermal Cycler (MJ Research) programmed as follows: 95°C-5 min, followed by 30 cycles of 94°C-30 s, 55°C-30 s, and 72°C-1 min, with a final extension at 72°C for 7 min. The amplified products of the individual reactions were separated in 1.5% agarose gels in 1 x TBE buffer in the presence of size markers.
2.7. Immunofluorescence staining of cells. Flow cytometry
Porcine skin fibroblasts, showing full cellular confluence, were washed with Hanks’ solution and detached from the bottom of the vessel using a cell scraper. Fibroblasts were collected by centrifugation. For the analysis cells with a concentration of 1 × 106/ml were used. The cells were washed 2 times with PBS 0.1% Tween 20 and treated with 3% BSA for 5 min. After washing with PBS 0.2% Tween 20 the cells were incubated with antibodies for 45 min 4°C in the dark (1 μg/ml) to detect the CD55 epitopes present on the surface of porcine fibroblasts. Monoclonal antibody (FITC Mouse Anti-Human CD55, BD Biosciences) specifically binds to CD55. Green fluorescence (range: 515–545 nm) emitted by the excited FITC was quantitatively recorded, allowing determination of the levels of surface antigen on cells derived from different types of pigs and from human prostate cancer (PC-3). After a washing step (PBS 0.2% Tween 20) samples were analyzed using the BD FACS Aria III (Becton Dickinson) flow cytometer, equipped with 4 lasers (375, 405, 488 and 633 nm), 11 fluorescence detectors, forward scatter (FSC) and side scatter (SSC) detectors. FACSFlow solution (Becton Dickinson) was used as sheath fluid. The configuration of the flow cytometer was as follows: 100 μm nozzle and 20 psi (0.138 MPa) sheath fluid pressure. The cells were characterized by two non-fluorescent parameters, i.e. forward scatter (FSC) and side scatter (SSC), and one fluorescent parameter, i.e. green fluorescence (FITC detector), from FITC-labelled CD55 monoclonal antibodies collected using the 530/30 band pass filter. For excitation of FITC-conjugated antibodies a 488 nm blue laser was employed. The flow cytometry analyses were performed using the logarithmic gains and specific detector settings. The FSC detector was fitted with an ND (neutral density) optic filter to optimize the FSC signal in order to display cells on a bivariate FSC vs. SSC dot plot. The threshold was set for the FSC signals in order to discriminate cells from the cellular debris. Data were acquired in a four-decade logarithmic scale as area signals (FSC-A, SSC-A, FITC-A) and analyzed with FACS DIVA software (Becton Dickinson). The analysis of fluorescence signal preceded the doublet discrimination procedure using height versus width scatter signals measurement, in order to discriminate single events (cells) from conglomerates (agglomerated cells). The green fluorescence signals from cell populations were displayed in FITC-A histograms. Each sample was analyzed in triplicate. The determination of CD55 epitope levels on the surface of analyzed cells was based on the median values of fluorescence intensity signals from the FITC detector.
2.8. Human serum cytotoxicity test
Cell viability was determined prior to the serum effect test. Cells with a viability above 80% were analyzed. The medium was discarded, then the cells were washed with PBS, trypsinized and centrifuged. The supernatant was removed and the pellet was washed with 400 ml of Hanks’ liquid. 200 μl of A or B media were added to the previously prepared cell pellet and incubated for 30 min at 37°C with intense mixing. Solutions containing serum consisted of the following components: A – 50% DMEM, 15% FBS, 35% PBS; B – 50% HS AB (human serum from human AB plasma). Then 20 μl of fibroblast suspension and medium A or B were collected and 20 μl of 0.4% trypan blue solution was added. Live and dead cells were counted in the Bürker chamber according to the rule of two sides in twelve repetitions. Cells were also counted using the Scepter 2.0 Handheld Automated Cell Counter (Merck). To quantify the number of living cells, a proliferation assay was used (Cell Counting Kit-8). The test consisted in measuring cell viability using a tetrazolium salt reduction to insoluble formazan. The amount of coloured reduced MTT is proportional to the number of metabolically active cells in the population. For this purpose, the cells were pipetted on a 96-well plate in a suitable configuration. Serum (A, B) was added to each well and incubated for 2 h. After the individual incubation times, the absorbance was measured at 450 nm. For each of three repetitions the percentage of live cells was calculated.
2.9. Metaphase preparations
To stop the cell division in mitotic metaphase, colcemid (0.05 µg/ml) was added to the culture dishes of 80% confluence. The cultures were incubated at 37 °C for 3 h and were subjected to osmotic shock by adding 75 mM KCl solution for 30 min. Next, the material was fixed with a cold mixture of methanol and acetic acid (3:1) three times. The number of metaphase plates was estimated by observation with a Nikon Eclipse E400 optical microscope in phase contrast mode.
2.10. Karyotype evaluation
Karyotype analysis of the modified pig was based on the GTC band pattern. This assay included the trypsinization of chromosome preparations and staining with Giemsa solution. The obtained GTC band patterns were analyzed using a microscope with a camera (Axio Imager M.2, Carl Zeiss Microscopy GmbH, Germany) and appropriate software (Ikaros, MetaSystems) and were compared with the standard karyotype of domestic pig (Gustavsson Citation1988).
3. Results
3.1. Generation of ULBP1-KO pigs with human CD55 gene
Two sgRNAs were designed previously to target the region of exon 2 of the porcine UL16-Binding Protein 1 (pULBP1) gene (Zeyland et al. Citation2018). The pCD55 donor vector was designed for one-step modification of the targeted site (). The mixture of Cas9-D10A mRNA, a pair of sgRNAs and the pCD55 donor vector was introduced into the pronucleus of the fertilized porcine oocyte based on the micro-injection procedure applied previously (Jura et al. Citation2007). As the result of superovulation treatment and insemination, 122 zygotes were flushed out. All obtained zygotes positively passed morphological evaluation and were subjected to the transfection procedure. For the transfer procedure into oviducts of 4 surrogates, 112 transfected zygotes were qualified (28 each). Three surrogates became pregnant and delivered a total of 13 piglets – #301–#313 (F0 generation). Twelve piglets (#301–#305, #307–#313) were subjected to CD55 transgene analysis and Cas9/gRNA mutation screening to detect integration and CRISPR-induced mutations. The 246 bp PCR products obtained from different individuals that showed in direct PCR sequencing mixed base calls (overlapping peaks in the sequencing chromatographs) were cloned. Plasmid DNA was sequenced. The analysis showed eight F0 generation piglets with wild type (+/+ULBP1) genotype (8/12, 66%). Four piglets showed indels in the targeting site of exon 2 of the pULBP1 gene, indicating that mutation efficiency reached about 33% (4/12) (). All four pigs were altered in a biallelic manner, showing different sequences in each mutant allele (4/4). We did not observe more than two different alleles in each individual. Piglet #308 (boar) also showed one allele interrupted with the CD55 expressing cassette. PCR analysis confirmed the presence of the CD55 transgene only in the case of one piglet (#308-boar). Further analysis of the homologous recombination of the transgene into the allele of the ULBP1 porcine gene was provided only for individual #308 showing one allele of the pULBP1 gene interrupted with the CD55-expressing cassette ().
Figure 2. Sequencing of UL16-binding protein 1 gene locus of targeted pigs using CRISPR-Cas9 technology. a Direct sequencing of PCR products from +/+ ULBP1, and −/− ULBP1 pig alleles separated by cloning. b List of mutations. The inverted or inserted bases are marked in lower case Bars indicate deletion of bases.
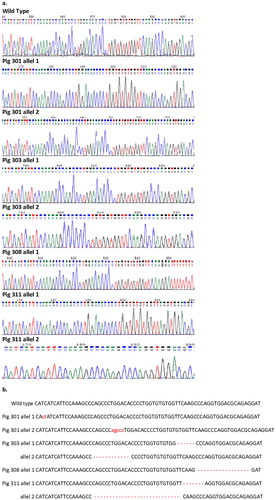
Figure 3. Analysis of the homologous recombination CD55 transgene into allele of the ULBP1 porcine gene for #308 individual. Polymerase chain reaction (PCR) was used to amplify DNA fragments of 985 bp (lane 1) and 1076 bp (lane 2). PCR products are only amplified in case of HDR-mediated transgene integration. The KAPA Universal Ladder Kits was used as size marker (lane 3). Separation of DNA fragments was conducted in 1.5% agarose gel.
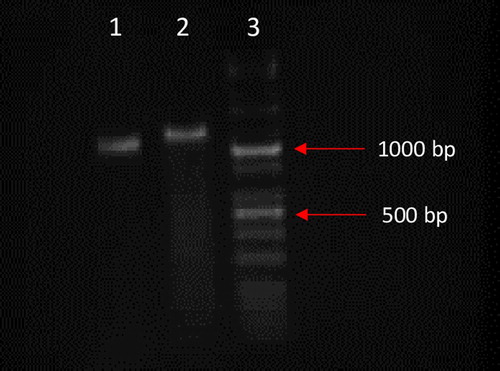
3.2. Analysis of mRNA of CD55 expression
Previously described analyses confirmed the integration of the CD55 transgene in the targeted site. Reverse transcription based analysis showed expression of the transgene at the mRNA level in the case of line #308. As a result of the amplification reaction, the presence of the expected product length of 620 bp was observed. No expression was found in the wild type control line (NT-14) analyzed in parallel (no PCR product). The extracted RNA was free of DNA, as evidenced by the total lack of amplification product in both analyzed lines (control PCR). The control reaction of cDNA quality showed PCR products of expected length (300 bp) in both analyzed lines.
3.3. Human serum cytotoxicity test
The human serum cytotoxicity test was conducted to evaluate the impact of human serum on modified (-/- ULBP1, +/− CD55) and wild type porcine fibroblast cell lines. To verify the functionality of the modification (-/-ULBP1, +/-CD55) in the cell lines, survival rate analysis of modified and wild type cell lines in the presence of human serum (source of complement system) was performed. Complement activation involves a series of enzymatic and non-enzymatic cascade reactions, which in turn leads to formation of a protein structure, MAC (membrane attacking complex). MAC participates in one of the important mechanisms of a non-specific immune response. As a result of its actions, cell lysis occurs. The results of absorbance measurement after cells’ incubation with human serum allow us to conclude that the highest viability of modified cells was 84.42% compared to the control. The viability of wild type cells is significantly lower at 43.8%. In connection with the above, the protective effect of the introduced genetic modification is noticeable. The average values of the three repetitions are shown in .
Figure 4. Survival rate analysis with standard deviations of wild type (NT-14) and modified (#308, (-/- ULBP1, +/-CD55)) pig cells treated with human serum. Cells suspended in human serum free medium were used as a control. The survival rate was measured after 2 h of incubation and compared to the total number of counted cells (3 repetitions). The absorbance value is directly proportional to the number of living cells and is marked on the Y axis.
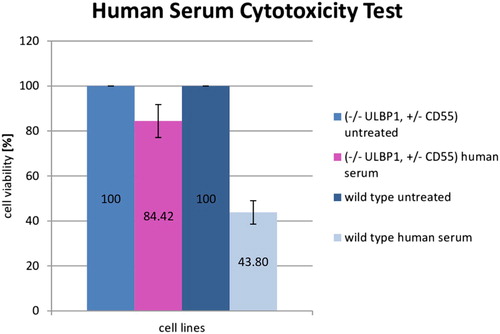
3.4. Flow cytometry
Immunofluorescence staining included skin fibroblast cells derived from pig #308 (-/- ULBP1, +/− CD55), fibroblasts derived from a wild type (WT control) pig, NT14, and a positive control sample – cells of a prostate cancer line, PC-3. The aim of the analysis was to prove the presence of CD55 protein on the surface of modified pig cells. Flow cytometric analysis was used to evaluate the CD55 epitope levels on the surface of pig cells. Monoclonal antibody FITC Mouse Anti-Human CD55 was used to reveal the differences in fluorescence intensity signals between wild type cells, modified (-/- ULBP1, +/− CD55) cells and PC-3 (positive control). The mean values of the medians of green fluorescence intensity from FITC-stained antibody were 92, 228 and 1330 relative fluorescence units (RFU) for the wild type, modified cells (-/- ULBP1, +/− CD55) and PC-3, respectively. The immunofluorescence study demonstrated a significant increase of the levels of CD55 epitope in modified individual (-/- ULBP1, +/− CD55) compared to the wild type porcine fibroblasts (4-fold higher FITC emission signals from the antibody bound to the CD55 antigen on the surface of the pig #308 cells versus wild type cells). Simultaneously, a 5.8-fold signal reduction was observed for cells derived from modified individuals compared to the positive control, PC-3 line.
Cytometric analysis of the cell lines suggests that the CD55-expressing cassette was integrated with the genome in the transcription active site and the product was transported to the outer cell membranes ().
Figure 5. Flow cytometric analysis of CD55 epitope levels on the surface of pig cells. Cell lines of fibroblasts from wild type, modified individual (-/- ULBP1, +/− CD55) and PC-3 cell line labeled with monoclonal anti-human CD55 FITC-conjugated mouse antibody were analyzed. X axis on overlay histogram shows the green fluorescence (FITC) intensity, Y axis shows the number of cells (cell count) in each fluorescence intensity channel. Individual histograms are labeled as follows: crosshatch, thick solid line and dotted line correspond to wild type pig, modified individual (-/- ULBP1, +/− CD55) and PC-3 cells, respectively. The analysis demonstrated a 4-fold increase in the CD55 epitope level in modified animals compared to WT control individual and 4-fold reduction in comparison with PC-3 positive control.
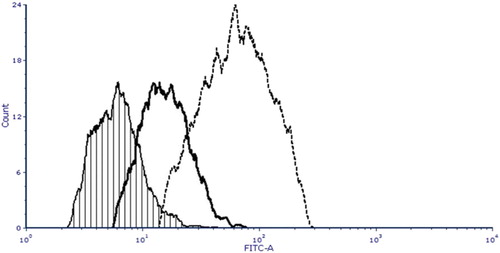
3.5. Karyotype evaluation
Karyotype evaluation of boar #308 was performed by GTC banding technique. Normal chromosome counts and structure were observed in analyzed cells ().
4. Discussion
Lymphocytes that are involved in the immune recognition are NK cells produced by bone marrow from the common lymphoid progenitor. Unlike other cells, NK cells do not engage somatic recombination of genes of receptors expressed on their surface to achieve diversity in recognition specificity. Instead of recombination, NK cells use the ‘missing-self’ mode (Ljunggren and Kärre Citation1990). NK cells’ activity is a result of the mechanisms of their activation and inhibition as a consequence of specific receptor signalling after target cell recognition.
NK cells expose a wide range of inhibitory receptors binding to MHC I molecules that lead to inhibition of NK cells. NKG2D is one of the best described homodimeric C-type lectin-like receptors involved in the NK cell activating pathway and costimulation of other reactive cells. NKG2D captures ligands that are expressed through their upregulation. These ‘induced-self’ molecules are poorly or not at all expressed on normal cells (Shifrin et al. Citation2014). NKG2D ligands include the UL-16 binding protein family. The inhibition of successive reactions of the primate immune system as a response to the pig xenograft is essential for its long survival. In this paper we report preliminary results of research concerning one-step modification of a targeted pig UL-16 binding protein gene to prevent interactions between pig ligand and primate activating NKG2D receptor. NKG2D leads to cell-mediated cytotoxicity of NK cells and causes production of cytokines (e.g. tumour necrosis factor and interferon) (Young et al. Citation2017). NK cell-mediated rejection is a huge obstacle in clinical application of pig-to-human xenotransplantation. Lilienfeld et al. reported that inhibition of NK cells (fresh and IL-2 activated) mediated cytotoxicity through the NKG2D receptor using the anti-porcine ULBP1 polyclonal antibody. Ortholog of ULBP-1 (pULBP-1) was shown to bind to human NKG2D, whereas pMIC2 (an NKG2D ligand) was not involved in pEC destruction (Lilienfeld et al. Citation2006). The elimination of potential porcine ligands for activating receptors might be helpful in xenograft rejection involving NK cells. In this study we have used sgRNA designed to target exon 2 of the pULBP1 gene as mentioned in our previous work. Four of twelve piglets showed indels in the targeting site of exon 2 of the pULBP1 gene, indicating that mutation efficiency reached about 33%. All were altered in a biallelic manner, showing different sequences in each mutant allele but not more than two per individual. As we described before, the level of pULBP1 on the surface of modified cells demonstrated by ELISA was decreased. The capacity of human NK cells to lyse -/-ULBP cells was reduced in comparison to wild type cells (Zeyland et al. Citation2018).
Human complement regulatory proteins such as human CD55, CD46 and CD59 have been used to overcome HAR in xenotransplantations. CD55 plays a role at the critical step of C3 activation in both the classical and alternative pathways of complement activation. CD55 inhibits C3 and C5 activation by preventing the development of C3 and C5 convertases and accelerating their decay (Zhou et al. Citation2005). It is commonly known that expression or combined expression of human CD55, CD59, or CD46 in transgenic pigs can suppress the activation of complements and inhibit the complement-mediated DXR (Shimizu et al. Citation2006; Fischer et al. Citation2016). In this paper we also report results of one-step modification of targeted sited of the pig genome with the hCD55 expression cassette. The donor vector was designed to disrupt exon 2 of the pULBP1 gene with the human CD55 coding sequence under the CMV promoter with polyA from the hGH gene. Only one tested animal had exon 2 of the pULBP1 gene interrupted by the CD55 sequence in one allele (0.08% efficiency of homology directed repair). The second allele was repaired by non-homologous end joining. Expression of CD55 was detected at the mRNA level; CD55 epitopes were also detected on the cell surface of animal #308 and their functionality was confirmed by human serum cytotoxicity assay. The complement regulator CD55 has long been valuable for xenotransplantation and experiments are ongoing (Rieblinger et al. Citation2018).
In this study we present a pULBP1-deficient and human CD55 coagulation regulator expressing potential founder of a donor animal line of organs for xenotransplantation. The combination of these two genetic modifications is presented for the first time and is very promising and gives hope for xenograft survival prolongation but probably will need other modifications to be combined. As we have confirmed in this study and previously, hCD55 expression reduced complement-mediated lysis of cells. We have also demonstrated that coexpression of more than one coagulation regulator did not guarantee their additional effect (Zeyland et al. Citation2012). Introduction of an additional copy of the gene encoding human CD55 under the control of the constitutive promoter into the pig genome did not have a negative impact on the health, development and reproduction of modified animals, as was also observed by others (Langford et al. Citation1994). We are convinced that the pig modifications described and characterized in this paper are an important step on the way to overcoming interspecies rejection of organs.
5. Conclusions
In our research we present a pULBP1-deficient, and expressing human CD55 (DAF), potential founder of a donor animal line of organs for xenotransplantation. The combination of these two genetic modifications is very hopeful for xenograft survival prolongation. Survival rate analysis of modified and wild type cell lines in the presence of human serum indicated that the protective effect of the introduced genetic modification is noticeable. Cytometric analysis of the cell lines suggests that the CD55-expressing cassette was integrated with the genome in the transcription active site and the product was transported to the outer cell membranes. We are convinced that the pig modifications discussed in this paper are an important step in the prevention of xenograft rejection.
Author contributions
ANT wrote the manuscript with support from JZ, performed skin fibroblast isolation and cell cultivation, immunofluorescence staining of cells for flow cytometry and conducted the human serum cytotoxicity test. DL designed the research framework and analyzed data concerning Cas9/gRNA mutation screening. MH carried out metaphase preparations, karyotype evaluation and homology directed repair analysis. JW performed the flow cytometry experiment. JJ performed microinjection and production of porcine zygotes. RS contributed to the design and implementation of the research and obtained funding for research. NM checked the manuscript in terms of language correctness and performed mutation screening. BG performed analysis of mRNA of CD55 expression. JZ developed Cas9-D10A/gRNA and donor template design, performed CD55 integration analysis and supported ANT in writing the manuscript.
Ethics approval and consent to participate
All applicable international, national, and/or institutional guidelines for the care and use of animals were followed. Permission 1181/2015 from 21 May 2015 II Local Ethic Commission in Krakow.
Disclosure statement
No potential conflict of interest was reported by the author(s).
Data availability
The datasets used and/or analyzed during the current study are available from the corresponding author on reasonable request.
ORCID
Agnieszka Nowak-Terpiłowska http://orcid.org/0000-0002-4844-3121
Additional information
Funding
References
- Brostoff J. 2012. Immunology. 8th ed. London: Elsevier Science Publishers.
- Cooper DK. 2012. A brief history of cross-species organ transplantation. Proc (Bayl Univ Med Cent). 25:49–57. doi:10.1080/08998280.2012.11928783.
- Cooper DK, Ekser B, Ramsoondar J, Phelps C, Ayares D. 2016. The role of genetically engineered pigs in xenotransplantation research. J Pathol. 238:288–299. doi:10.1002/path.4635.
- Delves PJ. 1998. Encyclopedia of immunology. 2nd ed. London: Elsevier Science Publishers.
- Fischer K, Kraner-Scheiber S, Petersen B, Rieblinger B, Buermann A, Flisikowska T, Flisikowski K, Christan S, Edlinger M, Baars W, et al. 2016. Efficient production of multi-modified pigs for xenotransplantation by ‘combineering’, gene stacking and gene editing. Sci Rep. 29(6):29081. doi:10.1038/srep29081.
- Ganji MR, Broumand B. 2007. Acute cellular rejection. Iran J Kidney Dis. 1(2):54–56.
- Gustavsson I. 1988. Standard karyotype of the domestic pig. Committee for the standardized karyotype of the domestic pig. Hereditas. 109(2):151–157. doi:10.1111/j.1601-5223.1988.tb00351.x.
- Hryhorowicz M, Zeyland J, Słomski R, Lipiński D. 2017. Genetically modified pigs as organ donors for xenotransplantation. Mol Biotechnol. 59(9–10):435–444. doi:10.1007/s12033-017-0024-9.
- Jura J, Smorąg Z, Słomski R, Lipiński D, Gajda B. 2007. Factors affecting the production of potential transgenic pigs by DNA microinjection; a six-year retrospective study. J. Anim. Feed Sci. 16(4):636–645. doi:10.22358/jafs/66820/2007.
- Langford GA, Yannoutsos N, Cozzi E, Lancaster R, Elsome K, Chen P, Richards A, White DJ. 1994. Production of pigs transgenic for human decay accelerating factor. Transplant Proc. 26(3):1400–1401.
- Lilienfeld BG, Garcia-Borges C, Crew MD, Seebach J. 2006. Porcine UL16-binding protein 1 expressed on the surface of endothelial cells triggers human NK cytotoxicity through NKG2D. J Immunol. 177:2146–2152. doi:10.4049/jimmunol.177.4.2146.
- Ljunggren HG, Kärre K. 1990. In search of the ‘missing self': MHC molecules and NK cell recognition. Immunol Today. 11(7):237–244. doi:10.1016/0167-5699(90)90097-S.
- Ma Y, Zhang L, Huang X. 2014. Genome modification by CRISPR/Cas9. FEBS J. 281(23):5186–5193. doi:10.1111/febs.13110.
- Niemann H, Verhoeyen E, Wonigeit K, Lorenz R, Hecker J, Schwinzer R, Hauser H, Kues WA, Halter R, Lemme E, et al. 2001. Cytomegalovirus early promoter induced expression of hCD59 in porcine organs provides protection against hyperacute rejection. Transplantation. 72:1898–1906. doi:10.1097/00007890-200112270-00006.
- Niu D, Wei H-J, Lin L, George H, Wang T, Lee I-H, Zhao H-Y, Wang Y, Kan Y, Shrock E, et al. 2017. Inactivation of porcine endogenous retrovirus in pigs using CRISPR-Cas9. Science. 357(6357):1303–1307. doi:10.1126/science.aan4187.
- Rieblinger B, Fischer K, Kind A, Saller BS, Baars W, Schuster M, Wolf-van Buerck L, Schäffler A, Flisikowska T, Kurome M, et al. 2018. Strong xenoprotective function by single-copy transgenes placed sequentially at a permissive locus. Xenotransplantation. 25(2):e12382. doi:10.1111/xen.12382.
- Shifrin N, Raulet DH, Ardolino M. 2014. NK cell self tolerance, responsiveness and missing self recognition. Semin Immunol. 26(2):138–144. doi:10.1016/j.smim.2014.02.007.
- Shimizu I, Smith NR, Zhao G, Nedof E, Sykes M. 2006. Decay-accelerating factor prevents acute humoral rejection induced by low levels of anti-alphaGal natural antibodies. Transplantation. 81:95–100. doi: 10.1097/01.tp.0000188176.18666.68
- Xiao-Jie L, Hui-Ying X, Zun-Ping K, Jin-Lian C, Li-Juan J. 2014. CRISPR-Cas9: a new and promising player in gene therapy. J Med Genet. 52(5):289–296. doi:10.1136/jmedgenet-2014-102968.
- Yang YG, Sykes M. 2007. Xenotransplantation: current status and a perspective on the future. Nat Rev Immunol. 7(7):519–531. doi:10.1038/nri2099.
- Young A, Ngiow SF, Gao Y, Patch AM, Barkauskas DS, Messaoudene M, Lin G, Coudert JD, Stannard KA, Zitvogel L, et al. 2017. A2AR Adenosine signaling suppresses natural killer cell maturation in the tumor microenvironment. Cancer Res. 78(4):1003–1016. doi:10.1158/0008-5472.CAN-17-2826.
- Zeyland J, Hryhorowicz M, Nowak-Terpiłowska A, Jura J, Słomski R, Smorąg Z, Lipiński D. 2018. The production of UL16-binding protein 1 targeted pigs using CRISPR technology. 3 Biotech. 8(1):70. doi:10.1007/s13205-018-1107-4.
- Zeyland J, Lipiński D, Słomski R. 2012. Human CD46, CD55 and CD59 expression influence on swine transgenic fetal fibroblasts’ survivability in the presence of human complement components. Ann Anim Sci. 12(4):515–526. doi:10.2478/v10220-012-0043-1.
- Zhang F, Wen Y, Guo X. 2014. CRISPR/cas9 for genome editing: progress, implications and challenges. Hum Mol Genet. 23:40–46. doi:10.1093/hmg/ddu125 doi: 10.1093/hmg/ddt394
- Zhou CY, McInnes E, Copeman L, Langford G, Parsons N, Lancaster R, Richards A, Carrington C, Thompson S. 2005. Transgenic mice expressing human CD59, in combination with human membrane cofactor protein and human decay-accelerating factor. Xenotransplantation. 12:142–148. doi:10.1111/j.1399-3089.2005.00209.x.