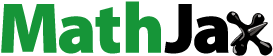
ABSTRACT
This study was done to evaluate the effects of two dietary probiotic preparations (Bacillus subtilis and Bacillus licheniformis) on growth performance, intestinal microbiota, nutrient digestibility and cytokine gene expression in broiler chickens. A total of 180 male broiler chicks (one-day-old Ross 308 strain, average initial body weight = 40.05 ± 0.12) were used in a completely randomized design (CRD) composed of 3 treatments and 6 replicates. Experimental diets included: (1) basal diet (without additive), (2) basal diet plus 0.5 g/kg diet B. subtilis preparation (1 × 109 CFU/g), (3) basal diet plus 0.5 g/kg diet B. licheniformis preparation (1 × 109 CFU/g). The results showed that supplementation of B. licheniformis improved (P < 0.05) broilers’ body weight gain (BWG), feed conversion ratio (FCR), and production efficiency factor (PEF). Adding B. licheniformis caused the lowest (P < 0.01) feed cost per kilogram weight gain and the highest (P < 0.05) return of investment (ROI). Probiotic treatments significantly decreased (P < 0.01) ileal pH of the broilers. Probiotic treatments improved (P < 0.01) apparent metabolizable energy (AME) and total tract digestibility of protein. It was concluded that although both probiotic bacteria improved AME and total tract protein digestibility, B. licheniformis was superior to B. subtilis in improving broiler chickens’ BWG (2580.70 vs. 2427.45 g) and their PEF (418.95 vs. 374.49).
Introduction
Regarding critical problems of using antibiotics in chicken diets, such as antibiotic resistance and residue in meat and eggs, nowadays, poultry nutritionists are searching to find functional dietary additives as antibiotic alternatives to improve birds’ growth performance and immunity and prevent necrotic enteritis and other diseases (Khan and Naz Citation2013; Yadav et al. Citation2016). It is well known that probiotics are nonpathogenic living microorganisms which can promote the health status of the consumer if they are consumed in an appropriate amount (FAO/WHO Citation2002). Probiotic microorganisms must have the ability to adhere to epithelial cells and tolerate the gastrointestinal secretions, such as digestive enzymes, bile, and gastric acid (Khan and Naz Citation2013). It was reported that probiotics can promote the immune responses and decrease the population of pathogenic microorganisms in the body of poultry (Ubeda and Pamer Citation2012; Vieira et al. Citation2013). Attia et al. (Citation2011) reported that probiotic improved the growth of broiler chickens and their immune responses and livability. Also, it was shown that probiotics have beneficial effects on energy and protein utilization in poultry (Attia et al. Citation2011; Houshmand et al. Citation2012; Ayasan, Citation2013). These days, many species of Bacillus bacteria are used as probiotic supplements in animal diets. Bacillus species are facultative anaerobe gram-positive bacteria that have endospores (Cutting, Citation2011). The characteristic of spore production by these bacteria is an advantage for them, which allows the bacteria to maintain their livability for a long time under high temperature or pressure than non-producing spore bacteria. Also, they can tolerate the hard and acidic environment of the stomach and reach safely to the end of the digestive system (Cutting Citation2011). Bacillus spp. have the ability to produce different antioxidants (Latorre et al. Citation2017), and probiotic supplementation can help to reduce oxidative stress in broilers (Shah et al. Citation2019a). In a study conducted by Khan et al. (Citation2014b), adding probiotic (Protexin®, Hilton Pharma, Holland) to Hubbard broiler breeders’ diet increased the level of paraoxonase (a member of anti-oxidant defence system) in serum of the birds. In addition, Bacillus spp. can produce antimicrobials (Urdaci et al. Citation2004), such as bacteriocins and bacteriocin like inhibitory materials (e.g. Subtilin, Coagulin), and antimicrobial substances, which contain a high amount of peptides and polyketides e.g. Bacilysin, Difficidin, Macrolactin, and Macrolactin (Belih et al. Citation2015). Also, Bacillus spp. have the potential to improve immune response and they can be considered as probiotic microorganisms (Lee et al. Citation2010a, Citation2010b). Some of Bacillus species, which are used as probiotics in humans and animals, include Bacillus coagulans, Bacillus clausii, Bacillus cereus, B. Subtilis, and B. licheniformis (Cutting Citation2011; Inci and Ayasan, Citation2019). Upadhaya et al. (Citation2019) reported that B. subtilis supplementation improved BW and FCR of broiler chickens during grower and finisher periods. The term licheniformis means the ability of producing Lichenicidine. The Lichenicidine is a two-peptide lantibiotic, which has antimicrobial effects against all Listeria monocytogenes, methicillin-resistant Staphylococcus aureus, and vancomycin-resistant enterococcus strains, and has been noticed by some lantibiotic producers. A few investigations have been done on the effects of B. licheniformis in poultry, and little information is available about the effect of B. licheniformis on nutrient metabolism in chickens (Liu et al. Citation2012). Adding B. licheniformis spores to diet as probiotic improved the performance of broiler chickens challenged with necrotic enteritis under commercial-like condition (Knap et al. Citation2010). Also, another probiotic bacterium, B. subtilis, was used in broilers’ diet by some researchers (Lee et al. Citation2010b; Lee et al. Citation2015) to evaluate the effect of B. subtilis on immunity and Eimeria infection in broilers. This bacterium inhibited pathogenic bacteria proliferation, helped gut integrity, and improved the performance of broilers challenged with Eimeria spp. and Clostridium perfringens (Lee et al. Citation2010b; Lee et al. Citation2015). Bai et al. (Citation2016) reported that supplementation of B. subtilis (2–4 × 1010 CFU/kg) improved the average of daily gain, average of daily feed intake (ADFI), and FCR of broiler chickens during the whole period of the experiment (1–42 d). Preparation of proper probiotic microorganism as a growth promoter additive is not an easy task and it needs extensive inquiries. So, before choosing special strains of bacteria as probiotics, in vivo trails are necessary to determine their advantages or disadvantages for the host animal. Hence, the aim of the present study was to compare the effects of B. subtilis (1×109 CFU/g) and B. licheniformis (1×109 CFU/g) on growth performance, intestinal microbiota, nutrient digestibility, IL6, IL10, or interferon gamma gene expression in broiler chickens.
Materials and methods
Broiler chickens and house management
A total of 180-day-old male broiler chicks (Ross 308) were purchased from a local hatchery (Morghdasht Hatchery, Karaj, Iran). The birds were weighed (average initial weight = 40.05 ± 0.12) and divided into 18 litter pens (1m× 0.67 m) in a completely randomized design with three treatments, 6 replicates, and 10 birds per replicate for 4 wk. The pens had wood shavings as bedding on a concrete floor. At the end of the fourth week, 60 male broiler chicks transferred to individual metabolic cages to measure the digestibility of nutrients. The birds were raised in accordance with the guidelines of the Institutional Animal Care and Ethics Committee of the Iranian Council of Animal Care (Care ICoA, Citation1995). The initial brooding temperature was set at 32 ± 1°C during the first week of age, then gradually reduced 3°C weekly to reach 22°C, the final temperature was kept until the end of the experiment. The relative humidity was kept around 55–60% during the whole period of the experiment. Cross-ventilation was run at this study, and the lighting programme was as 23 h light: 1 h dark.
Experimental diets
The experimental diets were formulated according to Ross 308 nutrient specifications (2014, with some modification according to Zaghari et al. Citation2017) during starter (1–10 d), grower (11–24 d), and finisher (25–44 d) periods (). The main ingredients of the diets were corn and soybean meal. Dietary treatments included: 1 – basal diet (without additive), 2 – basal diet plus 0.5 g/kg diet B. subtilis (1×109 CFU/g), 3 – basal diet plus 0.5 g/kg diet B. licheniformis (1×109 CFU/g). All diets were given in mash form and prepared weekly. Each probiotic preparation was first mixed with 1 kg of the experimental diet, then mixed with the whole amount of feed. The feed of each experimental unit was kept in a separate bucket. Feed and water was provided ad libitum throughout the experimental period (1–44 d). The probiotic strains used in the present study were provided from a commercial company (Biorun Company, Tehran, Iran). The probiotic preparations were single-strain probiotic composed of spore-forming bacteria including B. subtilis CH201 at 1 × 109 CFU/g, and B. licheniformis CH200 at 1 × 109 CFU/g. At the end of the experiment, 3 samples of each diet used in different period of rearing were examined to see the livability of B. subtilis and B. licheniformis in those diets. Diet samples were weighed and 1:4 wt/vol dilutions were used with sterile 0.9% saline. Then, 10-fold dilutions of each were prepared in a sterile 96-well Bacti flat-bottom plate. The bacteria livability was studied by using two culture medium, nutrient agar, and brain heart infusion agar. The plates were incubated at 37°C for 18 h and bacterial counts were expressed as Log10 CFU/g of tissue (Habib et al. Citation2015). The results of evaluating the livability of probiotic strains in diets showed that B. licheniformis had higher livability than B. subtilis ().
Table 1. Ingredients and nutrient composition of basal dietsa.
Table 2. Presence of the bacteria in the feed at the end of the experiment (44 d).
Growth performance
Growth performance parameters, such as BW, BWG, feed intake (FI), were measured for the birds in each pen, weekly. After adjusting for mortality, adjusted FCR of each pen was also recorded weekly. In addition, the PEF was calculated for the entire duration of the experiment based on the following formula: PEF = [live ability (%) ×live weight (kg)]/ [age (d) × FCR] × 100 according to Marcu et al. (Citation2013).
Economic indicators
The feed cost per kilogram weight gain was calculated for the whole period of the experiment, as multiplying FCR by the average cost of the diets. Return on investment (ROI) was used to compare speculation’s benefit in the experimental groups. For the calculation of ROI the benefit of an investment was divided by the cost of the investment (Zaghari et al. Citation2017).
Relative weight of digestive organs
At 44 d, 20 birds from each experimental group were selected according to the average BW within the group, weighted individually, and killed by cervical dislocation. Then, liver, proventriculus, and gizzard were separated from the carcass. The gallbladder was separated from the liver and the content of proventriculus and gizzard removed, then the liver, proventriculus, and gizzard were weighed. The organ weights were expressed as a percentage of live BW.
Measurement of gastrointestinal tract pH
At 44 d, just after GIT removal from 20 birds per treatment, the pH of the proventriculus, gizzard, ileum, and cecum contents was measured by a pH-meter (Testo 206 pH-meter, Germany). The pH of proventriculus and gizzard was measured by placing the electrode of the pH-meter in an incision that was made in the centre of the organ. For measuring the pH of ileum digest content, the electrode of the pH-meter was placed in an incision that was made 4 cm proximal to the ileocecal junction. For measuring the pH of ceca, both ceca were opened and their pH was measured (Teuchert Citation2014).
Ileal and cecal microflora
After slaughter at 44 d, the contents of ileum and ceca were collected immediately in sterile glass containers. Conventional microbiological techniques using selective agar media were used for the microbial analysis. One gram of the sample was taken into sterile test tubes and diluted 1:10 in 9 ml Ringer’s diluent (pH 6.8∼7.2) and homogenized. Then, 1 mL from dilutions was spread on appropriate selective agar media for enumeration of total aerobic bacteria, Lactobacillus, Yeast, and Mold, Enterobacteriaceae, Coliform, Clostridium perfringens. Bacterial colonies were counted by the pour plate method. Selective agar media were used for enumeration of Lactobacillus bacteria (MRS agar, Merck, Darmstadt, Germany), total aerobic bacteria (TSA agar, Merck, Darmstadt, Germany), Yeast and Mold (YGC agar, Merck, Darmstadt, Germany), Enterobacteriaceae (VRBD agar, Merck, Darmstadt, Germany), Coliform bacteria (VRBD agar, Merck, Darmstadt, Germany), and Clostridium perfringens (SIA agar, Merck, Darmstadt, Germany). The average number of colonies was multiplied by the reciprocal of the dilution factor and expressed as CFU/g of the content (Torlak et al. Citation2013; Menconi et al. Citation2014).
Total tract and ileal nutrient digestibility
At d 29, in order to investigate the nutrient digestibility, 60 male broilers with similar average BW were divided into individual metabolic cages with 0.15 m2 area. Measurement of apparent digestibility of nutrients was done by collecting both total excreta and ileal digesta. Apparent digestibility coefficients of nutrients were determined by using acid insoluble ash (AIA, Celit 281, Merck, Darmstadt, Germany) as an analytical marker. All experimental diets were mixed with 1% AIA for 2 d before collecting excreta. During the 2 d collection period, excreta from each cage were collected 2 times daily. Feed residue, feathers, and scales in the excreta trays were carefully removed and stored in sealed bags at −20°C. At the end of the 2 d collection period, all birds were killed by cervical dislocation. The carcasses were subsequently opened and the entire gastrointestinal (GI) tract was exposed carefully. The ileum was ligated and then separated from the rest of the GI tract and subsequently the ileal content was immediately stored in sealed bags at −20°C. After drying for 72 h at 50°C in an oven, the feed, excreta, and ileal samples were finely ground and analysed for crude protein (CP), gross energy (GE), calcium (Ca), and phosphorus (P). All analyses were performed by analysing each sample in triplicate. Determination of CP was performed by the AOAC method (AOAC, International. Citation2000. Official Methods of Analysis of AOAC International. 16th ed. AOAC International, Gaithersburg, MD) using an auto analyzer (KJETEK auto 1030 analyzer unit). The GE was also measured by adiabatic bomb calorimeter (IKA-Calorimeter C400). Total P was determined by a spectrophotometer (Envisense UV-2100 spectrophotometer) at the wavelength of 400 nm, and Ca was measured by an atomic absorption spectrophotometer (Shimadzu AA-670) at the wavelength of 422 nm. After determining the amount of nutrients and marker in feed samples, excreta, and ileal sample, apparent nutrient digestibility coefficients and AME (Kcal/ kg) were calculated using the following formulas (Stefanello et al., Citation2016).
Mi: | = | Concentration of acid insoluble ash in the diet |
Mo: | = | Concentration of acid insoluble ash in the excreta and ileal digesta |
Ei: | = | Concentration of CP, GE, Ca, P in the diet |
Eo: | = | Concentration of CP, Ca, P in the excreta and ileal digesta and GE in the ileal digesta |
GEi: | = | GE (Kcal/kg) in the diet |
GEo: | = | GE (Kcal/kg) in the excreta |
Isolation of RNA, reverse transcription, and real-time PCR
At 42 d, blood samples were collected from the brachial vein from 12 birds in each treatment. The samples were collected into Eppendorf tubes with ethylene-diamine-tetra-acetic acid (EDTA) and were centrifuged, and their plasmas were stored at −80°C until analysis. Total RNA was extracted from blood plasma samples using Trizol (GeneAll, South Korea), and alcoholic sediment RNA samples were treated with DNase I (Fermentase, USA) to remove contaminating genomic DNA. The concentration of RNA was determined using a Nano-drop 2000 spectrophotometer (Thermo Scientific, USA). The purity of RNA was verified at an optical density ratio of 260–280 nm. Two microgram of RNA was reverse-transcribed to cDNA in the presence of reverse transcription enzyme (Thermo, USA) using the Oligo-dT and random primers. The primers included interleukin 10 (IL10), interleukin 6 (IL6), Interferon gamma (INF-y), and glyceraldehyde 3 phosphate dehydrogenase (GAPDH) as internal control. Their sequence was found in national centre of biotechnological database (NCBI). Real-time quantitative polymerase chain reaction (qRT-PCR) was performed in triplicate reaction using both forward and reverse primers, cDNA, SYBR Green (Takara, Japan). The qRT-PCR was performed using step one thermal cycler (96Grad, PeQlab). During the PCR reaction, samples were subjected to an initial denaturation phase at 94°C for 30 secs, followed by 40 cycles of denaturation at 95°C for 3 secs and annealing and extension at 60°C for 30 sec. The sequences of the primers used in the present study are shown in . The results from qRT-PCR were analysed using the step one real-time PCR (ABI, USA). Average gene expression relative to the GAPDH, as endogenous control for each sample, was calculated using the 2− ΔΔCt method, where ΔΔCT was calculated by the following formula: ΔΔCT = Ct target gene − Ct GAPDH (Livak and Schmittgen, Citation2001).
Table 3. Chicken GAPDH and cytokine primer sequences.
Bacterial penetration into the blood
Blood samples collected from 12 birds per treatment at 42 d were used to investigate the probiotic bacteria penetration into the blood. About 0.1 CC of blood was cultured on blood agar culture medium, then the plates were incubated at 35 ± 2°C under aerobic conditions for 24–48 h, then the presence of bacteria on the medium was evaluated (Brouillette et al. Citation2004).
Statistical model and analysis
The statistical design was completely randomized design using the following model: Xij = μ + Ti + eij; where Xij is the observation, μ is the overall mean, Ti is the effect of the treatment, (i = 5), and eij is the residual error. The data of the present study were analysed by the GLM procedure of SAS software (SAS Citation2004). Variation of the data was shown as standard error of means (SEM). Differences among treatments were analysed by Duncan’s multiple range tests. Probability values as P < 0.05 were considered significant. Also, microbial loads were analysed after log10 transforming.
Results and disscussion
Growth performance
Supplementation of B. licheniformis increased (P < 0.05) FCR of broiler chicks during the first week of rearing. Also, adding dietary B. licheniformis improved (P < 0.05) BWG, FCR of the broilers during the fifth week. The PEF of the broilers fed with B. licheniformis was higher (P < 0.05) than other groups in the whole period of rearing (). However, the FI of the broilers in different experimental group was not different (P>0.05).
Table 4. Effect of B. subtilis and B. licheniformis on body weight gain, feed intake, and feed conversion ratio of broilers.
It is well known that gut health is a key point for animal performance due to its critical importance on nutrient digestion, absorption and metabolism, incidence of enteric diseases, and immune responses (Yegani and Korver Citation2008; Hamasalim Citation2016). Previous studies have shown that probiotics improved broilers’ growth performance and promoted greater populations of symbiotic microbiota in the intestine of broilers (Mountzouris et al. Citation2007; Latorre et al. Citation2017; Rhayat et al. Citation2017). In a recent study, Shah et al. (Citation2019b) found that supplementation of Lactobacillus bacteria increased intestinal villus’ height and absorptive capacity in broiler chickens that led to higher final BW of the birds. In our study, adding dietary B. licheniformis CH200, improved BWG, FCR, and PEF with no effect on FI during the whole period of the experiment (1–44 d). However, B. subtilis CH201 had no effect on birds’ growth performance traits. This is in agreement with the results of Abudabos et al. (Citation2017) who reported that dietary B. subtilis did not change BW and FCR of broiler chickens compared with the control group. In contrast to our results, Jayaraman et al. (Citation2017) reported that using B. subtilis PB6 improved BW and FCR of broiler chickens. The reasons for the inconsistencies may be due to the broilers’ strain, house condition, probiotic livability, or administration dosage. In agreement with our result, Liu et al. (Citation2012) reported that B. licheniformis improved BWG and FCR in broiler chickens. Hong et al. (Citation2005) found that Bacillus bacteria improved immune function and increased the level of endogenous antimicrobial peptides in the gut, which led to higher growth performance. Gerard et al. (Citation2008) reported that adding different species of Lactobacillus could stimulate gut-associated immunity and enhanced the growth performance of broiler chickens. Zhang et al. (Citation2012, Citation2013) found that the BWG was increased by the administration of a Bacillus-based probiotic (105 or 108 CFU/kg) in broilers’ diet. It is clear that FCR is an important parameter which can influence the economic profits of poultry farms, so improving birds’ FCR by adding dietary probiotic is a valuable finding which may lead to the reduction of the feed cost per kg meat production, parallel to get the expected weight gain or even higher (Jahromi et al. Citation2016). Additional studies have demonstrated the potential of B. licheniformis in improving the broilers’ FCR (Mountzouris et al. Citation2007; Awad et al. Citation2009; Karimi Torshizi et al. Citation2010). Yeo and Kim (Yeo and kim Citation1997) suggested that the mechanisms by which probiotics improve FCR can include changing the intestinal microbiota, promoting the growth of nonpathogenic bacteria with the ability of producing lactic acid and hydrogen peroxide, decreasing the growth of gut harmful microbiota, or increasing nutrient digestion and utilization in the body of the probiotic consumer. In contrast, several other studies reported no changes in the performance of broilers that consumed probiotic (Knap et al. Citation2011; Shams Shargh et al. Citation2012; Afsharmanesh et al. Citation2013). There are many variables that can affect broilers’ response to dietary additives. Some of these variables are the house environment, management practice, diet composition, strains of probiotics, technique of producing and administration, level of consumption, bird’s strain and age, level of barn cleanliness, and persistence of strain in the bird (Mountzouris et al. Citation2007; Lee et al. Citation2010b; Molnar et al. Citation2011).
Economic indicators
The results showed that adding dietary B. licheniformis decreased (P < 0.01) average feed cost ($) per kg gain compared to other treatments (). Also, supplementation of B. licheniformis increased (P < 0.05) ROI.
Table 5. Effect of B. subtilis and B. licheniformis on the feed cost per kilogram weight gain and return on investment (1–44 d).
There is some evidence that certain probiotic bacteria can improve the economic efficiency of broiler production. In a previous study, Panda (Citation2006) reported that Lactobacillus sporo genes improved the net income and economic efficiency of broiler chickens compared to the control group. Bonsu et al. (Citation2012) reported that a multi-strain probiotic (Lactobacilli 1 × 108 cfu/g, Bacillus 1 × 1012 cfu/g, and Saccharomyces cerevisiae 1 × 105 cfu/g) improved the economic benefit of Cobb hybrid broilers. They related this economic improvement to the positive influence of probiotic on the broilers’ performance. Also, Jadhav et al. (Citation2015) reported that using probiotic in poultry diet has several positive effects on the birds’ gut health state and performance. Jadhav et al. (Citation2015) concluded that probiotic supplementation is so useful for increasing economic efficiency of broiler production. In the present study, the economic profits improved by applying B. licheniformis. It decreased FCR of the broiler chickens that lead to lower feed cost per kg gain of the broiler chickens and it also increased ROI. However, B. subtilis increased feed cost per kg gain compared to other treatments and it did not change ROI compared to the control group. Zaghari et al. (Citation2017) indicated that birds fed with B. subtilis had the lowest feed cost per kg gain which is not seen in the present study. This contrast in findings could be related to the difference in the rearing condition and level of probiotic supplementation.
Relative digestive organ weight and pH gastrointestinal tract
The relative weights of proventriculus, liver, and gizzard were not affected (P > 0.05) by adding B. subtilis or B. licheniformis (). Supplementation of B. subtilis or B. licheniformis decreased (P < 0.01) ileal pH content of broiler chickens compared to the control group ().
Table 6. Effect of B. subtilis and B. licheniformis on the relative weight of digestive organs and gastrointestinal tract pH in broilers.
The range of digestive tract pH must be proper for the probiotic activity and the probiotic should be stable in the digestive system (Svihus Citation2014). In the present study, adding probiotics decreased the pH of ileum in broiler chickens. It has been demonstrated that B. subtilis spores create an anaerobic environment in the gut which is helpful for the lactic acid producing bacteria (Hoa et al. Citation2000). The lactic acid bacteria produce lactic acid, reduce the intestinal pH, and prevent the growth of harmful bacteria (Spring et al. Citation2000). Thus, changing the pH may result in shifting intestinal microbiota profile (Ptak et al. Citation2015). Probiotics have the ability to produce digestible proteins, vitamin, digestive enzymes, such as amylase and protease. Also, they can reduce the intestinal pH by producing lactic acid which helps for nutrient digestion and absorption.
Ileal and cecal microflora
Adding dietary B. subtilis or B. licheniformis had not significant (P > 0.05) effect on the population of Total aerobic bacteria, Lactobacillus, Coliforms, Enterobacteriaceae, Clostridium perfringens, Yeast and Mold in the ileum or ceca of the broilers ( and ). In the present study, probiotic bacteria did not affect the ileal or cecal microbial population. Cengiz et al. (Citation2015) reported that adding dietary probiotic (Lactobacillus acidophilus, Lactobacillus casei, Enterococcus faecium, and Bifidobacterium thermophilus) did not change the population of total aerobes, Salmonella, and Lactobacilli in gut. There are some reasons for this event such as lack of success of probiotics to: (1) survive from transiting through stomach to intestine, or (2) adhere physically and colonization on the gut surfaces (Jin et al. Citation1998). Regarding the results of the present study, it seems that under normal condition (healthy birds fed on corn-soybean meal diet), probiotics have little effect on the microbial population of the intestine.
Table 7. Effect of B. subtilis and B. licheniformis on microflora in the ileum of broilers (log CFU/g).
Table 8. Effect of B. subtilis and B. licheniformis on ceca microflora in broilers (log CFU/g).
Apparent ileal and total tract nutrient digestibility
The apparent ileal digestibility of CP, GE, Ca, and P was not affected by dietary B. subtilis or B. licheniformis (P > 0.05, ). However, AME and total tract digestibility of CP were significantly higher (P < 0.01) in probiotic fed groups than those of the control birds.
Table 9. Apparent Ileal and total tract nutrient digestibility in broilers.
It is interesting to note that probiotics may be considered as ‘ live enzyme factory’ because of their potential in producing amylase, protease, and lipase (Dhama and Singh Citation2010). So, probiotics can enhance the digestion and absorption of carbohydrate, proteins, and lipids and thus contribute in improving the efficiency of feed consumed (Dhama and Singh Citation2010). Also, it was recognized that Bacillus spp. produced cellulase and xylanase (Kogut and Arsenault Citation2016).
In this study, adding B. subtilis or B. licheniformis to broilers’ diet improved apparent digestibility of protein and ME compared to the control group. In agreement with our findings, a recent study has shown that supplementing B. subtilis improved total tract digestibility of DM, CP, and ME in broiler chickens (Reis et al. Citation2017). As mentioned before, the ileal pH of broilers fed with probiotics was lower than control birds which can help the absorption of protein and minerals like Copper, Ca, Iron, Manganese, and Magnesium (Raghuwanshi et al. Citation2015). Also, other researchers have confirmed that B. subtilis improved protein digestibility (Knap et al. Citation2011; Mahmoud et al. Citation2017). The positive effect on protein digestibility can be related to probiotics’ mechanisms of action, which regulates the intestinal microbial population, reduces digestive disorders, inhibits intestinal harmful microorganisms, and improves the feed efficiency in body (Abaza et al. Citation2008). In addition, some researchers have reported that probiotics increased enzyme secretion from pancreas and intestinal mucosa (Chen et al. Citation2009). On the other hand, it was reported that using B. subtilis spore (GalliPro®) at 0.2 g/kg as a feed additive reduced broilers’ requirements of amino acids, CP, and consequently their feed cost (Zaghari et al. Citation2015).
Cytokine gene expression
The gene expressions of IL6, INF-gamma, and IL10 are shown in (A, B, C). The IL6 gene expression tended (P = 0.056) to be higher in B. licheniformis group than the control group. Dietary supplementation of probiotics did not affect the relative gene expression of INF-gamma (P = 0.3753), or IL10 (P = 0.1204).
Figure 1. Effect of B. subtilis and B. licheniformis on the gene expression of IL6 (A), INF- gamma (B), and IL10 (C) in the blood plasma (n = 36) of broilers at 42 d of age. Each bar on the graph shows a treatment mean ± SEM.
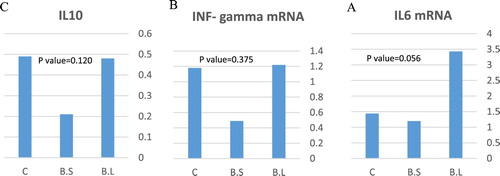
Immunomodulation property of probiotic organisms is exerted through their effect on T helper cells in a strain-specific manner (Fong et al. Citation2016). Also, they can activate various immune cells (Fong et al. Citation2016). Probiotics can also modulate intestinal barriers and stimulate the production of mucins, defensins, chemokines, and anti- and pro-inflammatory cytokines (Kogut and Swaggerty Citation2012). The mode of action of probiotics is not completely known yet; however it is obvious that these microorganisms affect birds’ microflora by their immunomodulatory characteristics (Hardy et al. Citation2013). On the other hand, cytokines are proteins or peptides secreted by specific cells which play an important role in immunity and regulation of inflammatory responses. In fact, probiotics have stabilizing effects in the intestine by stimulating the secretion of the anti- and pro-inflammatory cytokines, increasing the number of lymphocytes in the lamina propria and intraepithelial cells, and prevents the pathogenic bacteriás growth and illness of the bird (Dhama and Singh Citation2010). Cytokines produced by Th2 cells, like interleukin 10 and interleukin 4, are very important in the progress of humoral immune responses (production of antibodies), and the cytokines secreted by Th3 or regulatory T cells are critical in controlling the immune responses (Palamidi et al. Citation2016). Interleukin 6, produced by T cells, monocytes, and macrophages, acts as both pro- and anti-inflammatory cytokines, and also helps differentiate Th17 cells (Waititu et al. Citation2014). Increasing the IL-6 gene expression helps define the population of heterophiles that can eliminate pathogens (Hong et al., Citation2006). In this study, B. licheniformis stimulated the immune system by enhanced IL6 expression in broilers’ plasma. The effect of probiotics on the immune system of broiler chickens varies in different environmental conditions. In the study of Cao et al. (Citation2013), using dietary Enterococcus faecium in broiler chickens stimulated immune response as the birds fed E. faecium had a greater level of IL-4 in their jejunal mucosa. Several studies on human and animal have also provided the evidence that certain species of probiotics can stimulate innate immunity (Amit-Romach et al. Citation2010; Weiss et al. Citation2010) and humoral immune responses (Nermes et al. Citation2011; Khan et al. Citation2014a). Exploration of the effects of Bacillus strains supplementation on performance, microbiota establishment, nutrient digestibility, and immunity status may provide a better understanding of how different probiotics can be commercially used in order to deliver an alternative to the use of antibiotics. However, there is no much information about the enzymes or substances secreted from B. Licheniformis or B. subtilis in broilers’ intestine, so further investigations are needed to clarify the exact functions of the probiotics in broilers.
Bacterial penetration into the blood
The results of the bacterial detection in the blood showed that no bacteria have entered the blood stream of the broiler chickens.
Translocation of probiotic bacteria from the intestine to the blood and the following bacteremia is one of the critical issues that should be considered when probiotics are supplemented in diet (Lopetuso et al. Citation2017). It was reported that certain strains of bacteria have high potential to adhere on intestinal mucosa which increase the possibility of bacterial penetration to the blood. This happening is even more possible in hosts who have epithelial barrier dysfunction (De Marco et al. Citation2018). It is well known that the health state of the birds depends on some certain factors such as integrated intestinal barrier (Wu et al. Citation2019). Integrated intestinal barrier can protect the bird against the entrance of antigens and pathogenic bacteria. As described by Ohland and MacNaughton (Citation2010), the intestinal barrier includes epithelial cells, epithelial junctions, and mucous layer which contain immunoglobulin A and antimicrobial peptides. Previous research studies have shown that using probiotic can help to improve intestinal barrier function (Zareie et al. Citation2006; Wu et al. Citation2019). In addition, Wu et al. (Citation2019) have found that adding Paenibacillus polymyxa to broilers’ diet could up-regulate gene expression of claudin-1, occluding, and mucin-2 as the genes related to intestinal barrier. In the present study, lack of Bacillus subtilis and Bacillus licheniformis in broilers’ blood confirmed that probiotic bacteria only function in the birds’ digestive tract and do not penetrate into the animals’ circulation system. So, there is no likelihood of complications from high microbial count and septicemia arising from the presence of these bacteria and bacteriocin produced by them in the blood of broiler chickens.
Conclusions
Adding 0.5 g B. Licheniformis preparation to broilers’ diet improved BWG and PEF of the birds. The experimental diets did not have any significant effect on the relative weight of the proventriculus, gizzard, and liver of the broilers. Supplementation of B. subtilis or B. licheniformis had no significant effect on the population of microflora in the ileum or ceca of the broilers. Adding B. Licheniformis to broilers’ diet improved AME and total tract protein digestibility of the broiler chickens; however, dietary treatments did not have any significant effect on ileal digestibility of CP, GE, Ca, and P. The expressions of IL6, IL10, or interferon gamma genes were not changed among the different groups. Regarding our results, we recommend using 0.5 g B. licheniformis preparation (1×109 CFU/g) per kg of diet of broiler chickens during 1–42 d.
Acknowledgements
The authors thank the vice president at the University of Tehran for supporting this research study.
Disclosure statement
No potential conflict of interest was reported by the authors.
Additional information
Funding
References
- Abaza IM, Shehata MA, Shoieb MS, Hassan II. 2008. Evaluation of some natural feed additive in growing chick’ diets. Int J Poult Sci. 7:872–879. doi: 10.3923/ijps.2008.872.879
- Abudabos AM, Alyemni AH, Dafalla YM, Khan RU. 2017. Effect of organic acid blend and Bacillus subtilis alone or in combination on growth traits, blood biochemical and antioxidant status in broilers exposed to Salmonella typhimurium challenge during the starter phase. J Appl Anim Res. 45:538–542. doi: 10.1080/09712119.2016.1219665
- Afsharmanesh M, Sadaghi B, Silversides FG. 2013. Influence of supplementation of prebiotic, probiotic, and antibiotic to wet-fed wheat-based diets on growth, ileal nutrient digestibility, blood parameters, and gastrointestinal characteristics of broiler chickens. Comp Clin Path. 22:245–251. doi: 10.1007/s00580-011-1393-2
- Amit-Romach E, Uni Z, Reifen R. 2010. Multistep mechanism of probiotic bacterium, the effect on innate immune system. Mol Nutr Food Res. 54:277–284. doi: 10.1002/mnfr.200800591
- AOAC, International. 2000. Official methods of analysis of AOAC International, 16th ed. Gaithersburg, MD: AOAC International.
- Attia YA, Zeweil HS, Alsaffar AA, El-Shafy AS. 2011. Effect of non-antibiotic feed additives as an alternative to flavomycin on productivity, meat quality and blood parameters in broilers. Arch Geflügelk. 75:40–48.
- Awad WA, Ghareeb K, Abdel-Raheem S, Böhm J. 2009. Effects of dietary inclusion of probiotic and symbiotic on growth performance, organ weights, and intestinal histomorphology of broiler chickens. Poult Sci. 88:49–56. doi: 10.3382/ps.2008-00244
- Ayasan T. 2013. Effects of dietary inclusion of protexin (probiotic) on hatchability of Japanese quails. Indian J Anim Sci. 83:78–81.
- Bai K, Huang Q, Zhang J, He J, Zhang L, Wang T. 2016. Supplemental effects of probiotic Bacillus subtilis fmbJ on growth performance, antioxidant capacity, and meat quality of broiler chickens. Poult Sci. 96:74–82. doi: 10.3382/ps/pew246
- Belih SS, Labib ZM, Ragab AM. 2015. Role of Saltose probiotic for the control of the experimental infection of the clostridium perfringens and the coccidia in chickens. AJVS. 46:20–41. doi: 10.5455/ajvs.188631
- Bonsu FRK, Donkoh A, Osei SA, Okai DB, Baah J. 2012. Effect of direct-fed microbial and antibiotics supplementation on the health status and growth performance of broiler chickens under hot humid environmental conditions. Int J Livest Prod. 3:66–71.
- Brouillette E, Martinez A, Boyll BJ, Allen NE, Malouin F. 2004. Persistence of a Staphylococcus aureus small-colony variant under antibiotic pressure in vivo. FEMS Immunol Med Microbiol. 41:35–41. doi: 10.1016/j.femsim.2003.12.007
- Cao GT, Zeng XF, Chen AG, Zhou L, Zhang L, Xiao YP, Yang CM. 2013. Effects of a probiotic, Enterococcus faecium, on growth performance, intestinal morphology, immune response, and cecal microflora in broiler chickens challenged with Escherichia coli K88. Poult Sci. 92:2949–2955. doi: 10.3382/ps.2013-03366
- Care ICoA. 1995. Guide to the care and use of experimental animals. Isfahan: University of Technology Isfahan.
- Cengiz Ö, Köksal BH, Tatlı O, Sevim Ö, Ahsan U, Üner AG, Ulutas PA, Beyaz D, Buyukyoruk S, Yakan A, et al. 2015. Effect of dietary probiotic and high stocking density on the performance, carcass yield, gut microflora, and stress indicators of broilers. Poult Sci. 94:2395–2403. doi: 10.3382/ps/pev194
- Chen KL, Kho WL, You SH, Yeh RH, Tang SW, Hsieh CW. 2009. Effects of Bacillus subtilis var. natto and Saccharomyces cerevisiae mixed fermented feed on the enhanced growth performance of broilers. Poult Sci. 88:309–315. doi: 10.3382/ps.2008-00224
- Cutting SM. 2011. Bacillus probiotics. Food Microbiol. 28:214–220. doi: 10.1016/j.fm.2010.03.007
- De Marco S, Sichetti M, Muradyan D, Piccioni M, Traina G, Pagiotti R, Pietrella D. 2018. Probiotic cell-free supernatants exhibited anti-inflammatory and antioxidant activity on human gut epithelial cells and macrophages stimulated with LPS. Evid. Based Complement. Altern. Med. 1756308.
- Dhama K, Singh SD. 2010. Probiotics improving poultry health and production: an overview. Poult Punch. 26:41–41.
- FAO/WHO. 2002. Guidelines for the evaluation of probiotics in food [Cited Oct 2012]. Available from: http://www.who.int/foodsafety/fs_management/en/probiotic_guidelines.pdf.
- Fong FLY, Shah NP, Kirjavainen P, El-Nezami H. 2016. Mechanism of action of probiotic bacteria on intestinal and systemic immunities and antigen-presenting cells. Int Rev Immunol. 35:179–188. doi: 10.3109/08830185.2015.1096937
- Gérard P, Brézillon C, Quéré F, Salmon A, Rabot S. 2008. Characterization of cecal microbiota and response to an orally administered lactobacillus probiotic strain in the broiler chicken. J Mol Microbiol Biotechnol. 14:115–122. doi: 10.1159/000106090
- Habib F, Rind R, Durani N, Bhutto AL, Buriro RS, Tunio A, Aijaz N, Lakho SA, Bugti AG, Shoaib M. 2015. Morphological and cultural characterization of Staphylococcus aureus isolated from different animal species. JAEBS. 5:15–26.
- Hamasalim HJ. 2016. Synbiotic as feed additives relating to animal health and performance. Adv Microbiol. 6:288–302. doi: 10.4236/aim.2016.64028
- Hardy H, Harris J, Lyon E, Beal J, Foey AD. 2013. Probiotics, prebiotics and immunomodulation of gut mucosal defences: Homeostasis and immunopathology. Nutrients. 5:1869–1912. doi: 10.3390/nu5061869
- Hoa NT, Baccigalupi L, Huxham A, Smertenko A, Van PH, Ammendola S, Ricca E, Cutting AS. 2000. Characterization of Bacillus species used for oral bacteriotherapy and bacterioprophylaxis of gastrointestinal disorders. Appl Environ Microbiol. 66:5241–5247. doi: 10.1128/AEM.66.12.5241-5247.2000
- Hong HA, Duc LH, Cutting SM. 2005. The use of bacterial spore formers as probiotics. FEMS Microbiol Rev. 29:813–835. doi: 10.1016/j.femsre.2004.12.001
- Hong YH, Lillehoj HS, Lillehoj EP, Lee SH. 2006. Changes in immune-related gene expression and intestinal lymphocyte subpopulations following Eimeria maxima infection of chickens. Vet Immunol Immunopathol. 114:259–272. doi: 10.1016/j.vetimm.2006.08.006
- Houshmand M, Azhar K, Zulkifli I, Bejo MH, Kamyab A. 2012. Effects of non-antibiotic feed additives on performance, immunity and intestinal morphology of broilers fed different levels of protein. S Afr J Anim Sci. 42:23–32. doi: 10.4314/sajas.v42i1.3
- Inci H, Ayasan T. 2019. The effect of probiotic use on growth performance and some blood parameters ın Japanese quail exposed to temperature stress. ISPEC 2. Uluslararasi Tarim Ve Kirsal Kalkinma Kongresi, 27–29 Eylül 2019, Kiev –UKRAYNA.
- Jadhav K, Sharma KS, Katoch S, Sharma VK, Mane BG. 2015. Probiotics in broiler poultry feeds: A review. J Anim Nutr Physiol. 1:04–16.
- Jahromi MF, Altaher YW, Shokryazdan P, Ebrahimi R, Ebrahimi M, Idrus Z, Tufarelli V, Liang JB. 2016. Dietary supplementation of a mixture of Lactobacillus strains enhances performance of broiler chickens raised under heat stress conditions. Int J Biometeorol. 60:1099–1110. doi: 10.1007/s00484-015-1103-x
- Jayaraman S, Das PP, Saini PC, Roy B, Chatterjee PN. 2017. Use of Bacillus Subtilis PB6 as a potential antibiotic growth promoter replacement in improving performance of broiler birds. Poult Sci. 96:2614–2622. doi: 10.3382/ps/pex079
- Jin LZ, Ho YW, Abdullah N, Jalaludin S. 1998. Growth performance, intestinal microbial populations, and serum cholesterol of broilers fed diets containing Lactobacillus cultures. Poult Sci. 77:1259–1265. doi: 10.1093/ps/77.9.1259
- Karimi Torshizi MA, Moghaddam AR, Rahimi SH, Mojgani N. 2010. Assessing the effect of administering probiotics in water or as a feed supplement on broiler performance and immune response. Br Poult Sci. 51:178–184. doi: 10.1080/00071661003753756
- Khan RU, Naz S. 2013. Applications of probiotics in poultry production. World’s Poult. Sci J. 69:621–632.
- Khan RU, Rahman ZU, Javed I, Muhammad F. 2014a. Effect of vitamins, protein level and probiotics on immune response of moulted male broiler breeders. J Anim Physiol Anim Nutr. 98:620–627. doi: 10.1111/jpn.12087
- Khan RU, Zia-ur R, Javed I, Muhammad F. 2014b. Serum antioxidants and trace minerals as influenced by vitamins, probiotics and proteins in broiler breeders. J Appl Anim Res. 42:249–255. doi: 10.1080/09712119.2013.822815
- Knap I, Kehlet AB, Bente TL. 2011. B. subtilis-improved protein digestibility and equal performance in energy-reduced diets for broilers [Conference poster]. Actes des 9èmes Journées de la Recherche Avicole, Tours, France, 29 et 30 mars 2011, p. 349–352.
- Knap I, Lund B, Kehlet AB, Hofacre C, Mathis G. 2010. Bacillus licheniformis prevents necrotic enteritis in broiler chickens. Avian Dis. 54:931–935. doi: 10.1637/9106-101509-ResNote.1
- Kogut MH, Arsenault RJ. 2016. Gut health: The new paradigm in food animal production. Front Vet Sci. 3:1–4. doi: 10.3389/fvets.2016.00071
- Kogut MH, Swaggerty CL. 2012. Effects of prebiotics and probiotics on the host immune response. In: Direct-Fed Microbials and Prebiotics for Animals. NY: Springer, New York; p. 61–72.
- Latorre JD, Hernandez-Velasco X, Vicente JL, Wolfenden R, Hargis BM, Tellez G. 2017. Effects of the inclusion of a Bacillus direct-fed microbial on performance parameters, bone quality, recovered gut microflora, and intestinal morphology in broilers consuming a grower diet containing corn distillers dried grains with solubles. Poult Sci. 96:2728–2735. doi: 10.3382/ps/pex082
- Lee KW, Kim DK, Lillehoj HS, Jang SI, Lee SH. 2015. Immune modulation by Bacillus subtilis-based direct-fed microbials in commercial broiler chickens. Anim Feed Sci Tech. 200:76–85. doi: 10.1016/j.anifeedsci.2014.12.006
- Lee KW, Lee SH, Lillehoj HS, Li GX, Jang SI, Babu US, Park MS, Kim DK, Lillehoj EP, Neumann AP, et al. 2010b. Effects of direct-fed microbials on growth performance, gut morphometry, and immune characteristics in broiler chickens. Poult Sci. 89:203–216. doi: 10.3382/ps.2009-00418
- Lee KW, Lillehoj HS, Jang SI, Li G, Lee SH, Lillehoj EP, Siragusa GR. 2010a. Effect of Bacillus-based direct-fed microbials on Eimeria maxima infection in broiler chickens. Comp Immunol Microbiol Infect Dis. 33:e105–e110. doi: 10.1016/j.cimid.2010.06.001
- Liu X, Yan H, Lv L, Xu Q, Yin C, Zhang K, Wang P, Hu J. 2012. Growth performance and meat quality of broiler chickens supplemented with Bacillus licheniformis in drinking water. Asian Austral J Anim Sci. 25:682–689. doi: 10.5713/ajas.2011.11334
- Livak KJ, Schmittgen TD. 2001. Analysis of relative gene expression data using real-time quantitative PCR and the 2− ΔΔCT method. Methods. 25:402–408. doi: 10.1006/meth.2001.1262
- Lopetuso L, Graziani C, Guarino A, Lamborghini A, Masi S, Stanghellini V. 2017. Gelatin tannate and tyndallized probiotics: a novel approach for treatment of diarrhea. Eur Rev Med Pharmacol Sci. 21:873–883.
- Mahmoud KZ, Obeidat BS, Al-Sadi MZ, Hatahet SR. 2017. Effect of Bacillus subtilis supplementation and dietary crude protein level on growth performance and intestinal morphological changes of meat type chicken. Livest Sci. 195:99–104. doi: 10.1016/j.livsci.2016.11.015
- Marcu A, Vacaru-Opriş I, Dumitrescu G, Ciochina LP, Marcu A, Nicula M, Peţ I, Dronca D, Kelciov B, Mariş C. 2013. The influence of the genotype on economic efficiency of broiler chickens growth. Sci P Anim Sci Biotechnol. 46:339–346.
- Menconi A, Kallapura G, Latorre JD, Morgan MJ, Pumford NR, Hargis BM, Tellez G. 2014. Identification and characterization of lactic acid bacteria in a commercial probiotic culture. Biosci Microb Food H. 33:25–30.
- Molnar AK, Podmaniczky B, Kurti P, Tenk I, Glavits R, Virag G, Szabo Z. 2011. Effect of different concentrations of Bacillus subtilis on growth performance, carcass quality, gut microflora and immune response of broiler chickens. Br Poult Sci. 52:658–665. doi: 10.1080/00071668.2011.636029
- Mountzouris KC, Tsirtsikos P, Kalamara E, Nitsch S, Schatzmayr G, Fegeros K. 2007. Evaluation of the efficacy of a probiotic containing Lactobacillus, Bifidobacterium, Enterococcus, and Pediococcus strains in promoting broiler performance and modulating cecal microflora composition and metabolic activities. Poult Sci. 86:309–317. doi: 10.1093/ps/86.2.309
- Nermes M, Kantele JM, Atosuo TJ, Salminen S, Isolauri E. 2011. Interaction of orally administered Lactobacillus rhamnosus GG with skin and gut microbiota and humoral immunity in infants with atopic dermatitis. Clin Exp Allergy. 41:370–377. doi: 10.1111/j.1365-2222.2010.03657.x
- Ohland CL, MacNaughton WK. 2010. Probiotic bacteria and intestinal epithelial barrier function. Am J Physiol Gastroint Liver Physiol. 298:G807–G819. doi: 10.1152/ajpgi.00243.2009
- Palamidi I, Fegeros K, Mohnl M, Abdelrahman WHA, Schatzmayr G, Theodoropoulos G, Mountzouris KC. 2016. Probiotic form effects on growth performance, digestive function, and immune related biomarkers in broilers. Poult Sci. 95:1598–1608. doi: 10.3382/ps/pew052
- Panda AK, Rao SVR, Raju MV, Sharma SR. 2006. Dietary supplementation of Lactobacillus sporogenes on performance and serum biochemico-lipid profile of broiler chickens. J Poult Sci. 43:235–240. doi: 10.2141/jpsa.43.235
- Ptak A, Bedford MR, Świątkiewicz S, Żyła K, Józefiak D. 2015. Phytase modulates ileal microbiota and enhances growth performance of the broiler chickens. PLoS One. 10:e0119770. doi: 10.1371/journal.pone.0119770
- Raghuwanshi S, Misra S, Bisen PS. 2015. Indian perspective for probiotics: A review. Indian J Dairy Sci. 68:195–205.
- Reis MP, Fassani EJ, Garcia Júnior AAP, Rodrigues PB, Bertechini AG, Barrett N, Persia ME, Schmidt CJ. 2017. Effect of Bacillus subtilis (DSM 17299) on performance, digestibility, intestine morphology, and pH in broiler chickens. JAPR. 4:573–583.
- Rhayat L, Jacquier V, Brinch KS, Nielsen P, Nelson A, Geraert PA, Devillard E. 2017. Bacillus subtilis s train specificity affects performance improvement in broilers. Poult Sci. 96:2274–2280. doi: 10.3382/ps/pex018
- SAS Institute. 2004. SAS User’s Guide. Statistics. Version 9.1 Edition. Cary, NC: SAS Inst. Inc.
- Shah M, Zaneb H, Masood S, Khan RU, Ashraf S, Sikandar A, Rehman HFU, Rehman HU. 2019b. Effect of dietary supplementation of zinc and multi-microbe probiotic on growth traits and alteration of intestinal architecture in broiler. Probiotics Antimicrob Proteins. 11:931–937. doi: 10.1007/s12602-018-9424-9
- Shah M, Zaneb H, Masood S, Khan RU, Din S, Khan I, Tariq A, ur Rehman H. 2019a. Ameliorative effect of zinc and multistrain probiotic on muscle and bone characteristics in broiler reared under cyclic heat stress. Pak J Zool. 51:1041–1046.
- Shargh MS, Dastar B, Zerehdaran S, Khomeiri M, Moradi A. 2012. Effects of using plant extracts and a probiotic on performance, intestinal morphology, and microflora population in broilers. J Appl Poult Res. 21:201–208. doi: 10.3382/japr.2010-00145
- Spring P, Wenk C, Dawson KA, Newman KE. 2000. The effects of dietary mannaoligosaccharides on cecal parameters and the concentrations of enteric bacteria in the ceca of salmonella challenged broiler chicks. Poult Sci. 79:205–211. doi: 10.1093/ps/79.2.205
- Stefanello C, Vieira SL, Rios HV, Simões CT, Sorbara JOB. 2016. Energy and nutrient utilisation of broilers fed soybean meal from two different Brazilian production areas with an exogenous protease. Anim Feed Sci Tech. 221:267–273. doi: 10.1016/j.anifeedsci.2016.06.005
- Svihus B. 2014. Function of the digestive system. J Appl Poult Res. 23:306–314. doi: 10.3382/japr.2014-00937
- Teuchert N. 2014. Comparison of production parameters, gut histology, organ weights, and portion yields of broilers supplemented with Ateli plus (Doctoral dissertation, Stellenbosch: Stellenbosch University).
- Torlak E, Sert D, Serin P. 2013. Fate of Salmonella during sesame seeds roasting and storage of tahini. Int J Food Microbiol. 163:214–217. doi: 10.1016/j.ijfoodmicro.2013.03.010
- Ubeda C, Pamer EG. 2012. Antibiotics, microbiota, and immune defense. Trends Immunol. 33:459–466. doi: 10.1016/j.it.2012.05.003
- Upadhaya SD, Rudeaux F, Kim IH. 2019. Effects of inclusion of Bacillus subtilis (Gallipro) to energy-and protein-reduced diet on growth performance, nutrient digestibility, and meat quality and gas emission in broilers. Poult Sci. 98:2169–2178. doi: 10.3382/ps/pey573
- Urdaci MC, Bressollier P, Pinchuk I. 2004. Bacillus clausii probiotic strains: antimicrobial and immunomodulatory activities. J Clin Gastroenterol. 38:S86–S90. doi: 10.1097/01.mcg.0000128925.06662.69
- Vieira AT, Teixeira MM, Martins FDS. 2013. The role of probiotics and prebiotics in inducing gut immunity. Front Immunol. 4:1–12. doi: 10.3389/fimmu.2013.00445
- Waititu SM, Yitbarek A, Matini E, Echeverry H, Kiarie E, Rodriguez-Lecompte JC, Nyachoti CM. 2014. Effect of supplementing direct-fed microbials on broiler performance, nutrient digestibilities, and immune responses. Poult Sci. 93:625–635. doi: 10.3382/ps.2013-03575
- Weiss G, Rasmussen S, Zeuthen LH, Nielsen BN, Jarmer H, Jespersen L, Frøkiær H. 2010. Lactobacillus acidophilus induces virus immune defence genes in murine dendritic cells by a Toll-like receptor-2-dependent mechanism. Immunol. 131:268–281. doi: 10.1111/j.1365-2567.2010.03301.x
- Wu Y, Wang B, Zeng Z, Liu R, Tang L, Gong L, Li W. 2019. Effects of probiotics Lactobacillus plantarum 16 and Paenibacillus polymyxa 10 on intestinal barrier function, antioxidative capacity, apoptosis, immune response, and biochemical parameters in broilers. Poult Sci. 98:5028–5039. doi: 10.3382/ps/pez226
- Yadav AS, Kolluri G, Gopi M, Karthik K, Singh Y. 2016. Exploring alternatives to antibiotics as health promoting agents in poultry-A review. J Exp Biol. 4(3S-10.18006):368–383.
- Yegani M, Korver DR. 2008. Factors affecting intestinal health in poultry. Poult Sci. 87:2052–2063. doi: 10.3382/ps.2008-00091
- Yeo J, Kim KI. 1997. Effect of feeding diets containing an antibiotic, a probiotic, or yucca extract on growth and intestinal urease activity in broiler chicks. Poult Sci. 76:381–385. doi: 10.1093/ps/76.2.381
- Zaghari M, Derakhshani Diba M, Moravej H, Zahroojian N. 2017. Estimation of metabolizable energy equivalency of Bacillus subtilis spore for male broiler chickens. J Livest Sci Technol. 5:9–18.
- Zaghari M, Zahroojian N, Riahi M, Parhizkar S. 2015. Effect of Bacillus subtilis spore (GalliPro®) nutrients equivalency value on broiler chicken performance. Ital J Anim Sci. 14:94–98. doi: 10.4081/ijas.2015.3555
- Zareie M, Johnson-Henry K, Jury J, Yang PC, Ngan BY, McKay DM, Soderholm JD, Perdue MH, Sherman PM. 2006. Probiotics prevent bacterial translocation and improve intestinal barrier function in rats following chronic psychological stress. Gut. 55:1553–1560. doi: 10.1136/gut.2005.080739
- Zhang ZF, Cho JH, Kim IH. 2013. Effects of Bacillus subtilis UBT-MO2 on growth performance, relative immune organ weight, gas concentration in excreta, and intestinal microbial shedding in broiler chickens. Livest Sci. 155:343–347. doi: 10.1016/j.livsci.2013.05.021
- Zhang ZF, Zhou TX, Ao X, Kim IH. 2012. Effects of β-glucan and Bacillus subtilis on growth performance, blood profiles, relative organ weight and meat quality in broilers fed maizesoybean meal based diets. Livest Sci. 150:419–424. doi: 10.1016/j.livsci.2012.10.003