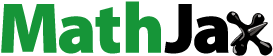
ABSTRACT
The objective of the study was to examine the effects of mannanoligosaccharide (MOS) or inulin on fourty-five HF calves’ performance and certain physiological parameters. Next to the control group (15 calves, no treatment), 15–15 calves received 18.7 g/calf/day MOS or inulin supplementation with milk replacer from 1st day of age to weaning. Feed intake was measured daily. Body weight was monitored on days 1, 14, 21 and 60. Blood samples were taken on days 30 and 60 to determine clinicochemical parameters Total faecal counts of the main faecal bacteria contents were determined from faecal samples collected on days 15 and 30. Mean feed intake, body weight and daily weight gain were similar in the groups. Inulin caused lower total protein level of the blood. Faecal concentration of total anaerobic bacteria and Lactobacillus was significantly increased by inulin compared to MOS. Inulin caused higher total aerobic bacteria count in faeces compared to control. The germ count of Clostridium perfringens from calves fed inulin was higher than that of other treatments. It should be stated that higher (18.7 g/day/calf) dietary level of inulin or MOS had minor alterations in parameters and failed to significantly modulate performance and physiological parameters of calves.
Introduction
Calf diarrhoea, causes serious problems in the early stage of life and according to Gulliksen et al. (Citation2009) it increases the risk of calf mortality. The use of antibiotics has been a typical practice in animal husbandry for disease prevention and control. To address the risk of development of antibiotic-resistant bacterial strains and the appearance of drug residues in foods, numerous restrictions were imposed on the use of antibiotics in the past 15 years [see Regulation (EC) No. 1831/2003 of the European Parliament and of the Council on additives for use in animal nutrition]. Thus, there is a need for alternative products that can ensure production, health protection and economic efficiency similar to that provided by antibiotics (Franklin et al. Citation2005). Prebiotics have been proposed as alternatives because these materials have wide-ranging physiological effects on gut function, activity of the large intestinal microflora, mineral absorption, lipid homeostasis, and immunity (Samanta et al. Citation2013).
Of the dietary fibres, fructan (a polymer of fructose molecules) supports the growth of beneficial bacteria in the intestine. The best known fructan is inulin, which is either obtained from the roots of chicory or produced synthetically from saccharose (Niness Citation1999). Inulin increased the body weight gain (BWG) and slightly improved the feed conversion ratio while decreased the length of intestinal villi and the number of goblet cells and proliferating cells and caused changes in physiological parameters of blood (Masanetz et al. Citation2010, Citation2011).
Mannanoligosaccharide (MOS) is a glucomannan–protein complex derived from the cell wall of yeast (Saccharomyces cerevisiae), which resists the action of digestive enzymes. As the lectins playing a role in the attachment of pathogenic bacteria occurring in the gut (such as Escherichia coli and Salmonella) are mostly mannose specific, MOS has excellent bacterium-binding properties. By occupying their attachment sites, MOS prevents the adherence of pathogenic bacteria to the intestinal mucosa. Supplementation of different doses of MOS (1–10 g/day) resulted in higher feed intake (Heinrichs et al. Citation2003; Morrison et al. Citation2010; Ghosh and Mehla Citation2012) and improved body weight gain (Król Citation2011). Other authors did not find significant changes in performance data of calves (Terré et al. Citation2007; Hill et al. Citation2008; Uzmay et al. Citation2011; Silva et al. Citation2012; Heinrichs et al. Citation2013; Kara et al. Citation2015).
Although there is a consensus among researchers regarding the mechanism of action of prebiotics, results from feeding MOS or inulin are variable and are not yet fully conclusive. This is due to differences between dairy farms in animal health status and in the rearing practices of milk-fed calves as well as to the use of prebiotics in different doses. The level of inclusion is one of the critical factors for measurable prebiotic effects in animals. Berge (Citation2016) reported based on earlier experimental data, that MOS supplementation ranged from 2 to 10 g per day, with an average inclusion of 3.8 g per day. Heinrichs et al. (Citation2003) fed 77 mg MOS/kg initial body weight (BW) to their calves, while Hill et al. (Citation2008) used 137 mg MOS/kg BW. These doses in calves are at the low end of the 90 to 4500 mg of MOS/kg BW range fed to other species (Swanson et al. Citation2002; Ten Bruggencate et al. Citation2005). Dietary inulin levels ranged from 3 to 10 g/kg in calf milk replacers according to the review of Verdonk et al. (Citation2005). Experimental results obtained in pigs showed that at least 3% inulin should be supplemented to achieve physiological changes (Metzler-Zebeli et al. Citation2017). Lower inclusion levels used in trials with calves may explain the small effect of MOS or inulin on animal health, faecal characteristics and blood metabolites. We hypothesized that calves supplemented with MOS or inulin in higher daily amount would have better growth rate, more favourable faecal bacterial composition compared with untreated calves. The objective of the current farm study was to examine the effect of 18.7 g /day/calf MOS or inulin supplementation on starter feed intake, body weight gain, gut microbiomes and some physiological parameters of HF calves.
Materials and methods
Experimental animals and housing
A total of 45 female calves (birth weight: 39.2 ± 3.6 kg) were included in the study. The calves received 3 litres of colostrum via an oesophageal tube within 2 h of birth only one time. The calves were individually housed in hutches (Calf-Tel Pro II, Hampel Corporation, Germantown, Wisconsin, USA) after 12–24 h of birth. The external dimensions of the hutch were 220 × 122 cm, while the interior, covered part was 212 × 114 × 122 cm in size. The floor of the hutches was crushed gravel, from where precipitation and effluent was drained off via a drain tube. Straw was spread both inside and outside the hutch, and this straw bedding was changed once or twice a week according to demand, thus continuously providing dry bedding for the calves. After weaning (between 54 and 63 days of age) the calves remained in the individual hutches for further 7 days, in order to diminish the stress caused by weaning. The calves were transferred to the heifer rearing unit at 60–70 days of age. This transfer was done once a week, and thus weaning was also performed at weekly intervals, and therefore the age of the animals was not the same at the time of this technological step.
Treatments and feeding
The calves received Sprayfow Yellow (Trouw Nutrition, Sloten, The Netherlands) calf milk replacer, at 145 g/l mixing rate, twice a day, at 12-hour intervals. A total of 45 female calves were assigned at 1 day of age to three groups. The control group (n = 15) did not receive any supplementation, 15 calves received MOS (Agrimos®, mannan min. 24%, β-glucans 25%, Lallemand Animal Nutrition, France) and 15 calves were given inulin (Frutafit® CLR, oligofructose/inulin ≥85%, Sensus, The Netherlands), mixed into their milk replacer. With the advancement of the calves’ age, the quantity of the milk replacer and supplements fed was changed according to the following principle:
Days 1–14: 2 × 2.5 l (725 g milk replacer/day) + 12 g/day MOS or inulin
Days 15–21: 2 × 3.5 l (1015 g milk replacer/day) + 12 g/day MOS or inulin
Days 22–56: 2 × 4.5 l (1305 g milk replacer/day) + 24 g/day MOS or inulin
Days 57–63: 2 × 2 l (580 g milk replacer/day) + 12 g/day MOS or inulin
Calves received 5 l/d of milk replacer from 1 to 14 d, 7 l/d from 15 to 21 d, 9 l/d from 22 to 56 d, and 4 l/d from 57 to 63 d. Earlier studies (Diaz et al. Citation2001; Jasper and Weary Citation2002) revealed that feeding high amounts of milk replacer resulted in increased faecal consistency. Thus, in order to palliate any negative effects of increasing faecal consistency, the original level of prebiotics (12 g/day/calf) was increased to 24 g/day/calf between 22 and 56 day of life. After that, calves received again 12 g/day of MOS or inulin. According to the dosage described above, the calves received an average of 18.7 g of MOS or inulin/day during the trial. The calves weaned at age between 54 and 63 days. Starter feed intake was monitored until calves moved from the calf hutches.
The calves had access to fresh water ad libitum from day one to end of the trial. The calf starters was introduced to calves at day 7 ad libitum. The nutrient content of the milk replacer used in the study is shown in . Ration formulation and chemical composition of calf starter diet are presented in .
Table 1. Composition of the calf milk replacer (as specified by the manufacturer).
Table 2. Ration formulation and chemical composition of the starter diet (as specified by the manufacturer).
Samplings and measured parameters
As we conducted our trial on an operating dairy farm of 1700 cows, when scheduling the data collections we had to adjust to the routine farm management practices and to the availability of the farm personnel for the tasks related to the study.
Individual starter feed intake was recorded for all animals every day, between 9 and 11 am unless it was contaminated with manure, urine or rainwater.
The calves were weighed at the time of their birth and then on days 14, 21 and 60. From body weight data individual body weight gains were calculated for the given periods.
For the determination of the clinicochemical parameters, blood samples were taken from all calves included in the experiment on days 30 and 60.
On days 15 and 30, fresh faecal samples were collected from 12 calves per group for microbiological examinations.
Clinicochemical parameters
We took 4-ml blood samples from each calf into vacutainer blood collection tubes at 30 and 60 days of age. Serum total protein, albumin, glucose, triglyceride, urea and total cholesterol concentrations were determined in a veterinary laboratory (Vet-Med Laboratory, Budapest, Hungary), using Roche Hitachi 912 Chemistry Analyzer (Hitachi, Tokyo, Japan) with commercial diagnostic kits (Diagnosticum LTD., Budapest, Hungary).
Microbiological examinations
Fresh faecal samples were taken from 12 calves per group at 15 and 30 days of age, 4 h after the morning feeding. From 1 g of faecal content, serial dilutions with 0.9% NaCl were made immediately after sampling and used for microbiological determination. The conditions of culturing are summarized in . Anaerobic conditions were ensured with the help of Anaeroculture dishes (Merck, Darmstadt, Germany) and ‘AnaerocultA’ (Merck, Darmstadt, Germany) gas-producing bags. The samples were incubated in an LP 104 type thermostat (LMIM, Esztergom, Hungary). After the incubation time had elapsed, the colonies were counted with an Acolyte colony counter (Aqua-Terra Lab Ltd., Veszprém, Hungary). The colony counts were expressed in log10 colony forming units (CFU) related to 1 g of sample.
Table 3. Methodology of microbial culture of faecal samples.
Statistical analysis
A total of 45 HF female calves were included in the study and they were divided into three groups (15/group). Out of 45 calves, three calves died, each from the MOS group. Two died after 30 days of age and one after 14 days of age. Furthermore, we calculated fewer data within the microbiology section, due to error in the procedure and sampling. Thus, the data from fewer number of calves presented in the results section of this paper. Except the supplementations, all of the circumstances were the same for all animals in the whole time of experiment. Statistical analysis was done using the R Commander 3.4.1 program type. Arithmetic means, standard error (SE), standard error of mean (SEM) for each evaluated variable group were calculated using descriptive statistical procedures. Most of these values presented in the tables of the results section. Exception to this was the starter feed intake, due to avoiding the unnecessarily much information. The daily starter feed intake of the animals was recorded for every day of life, unless it was contaminated with manure, urine or rainwater. Due to these missing datas, the One-Way ANOVA procedures of the programme were use for analysing and compare the variances on every single day. Differences were considered as significant if P < 0.05. The statistical model was as follows:where Xtm is the dependent variable, μ = overall mean, Ft = fixed effect of factor where t = treatment, εtm = residual error
The recorded data on body weight, daily weight gain, faecal microbiology and clinicochemical variables were analysed using Repeated Measures Analysis of Variation with use RcmdrPlugin.aRnova package of R Commander. Tukey–Kramer multiple comparisons test were used to evaluate the significances of means between the different treatment groups of calves. The effect of calf was considered as random. The following statistical model was used for repeated measures analysiswhere Xtim is the dependent variable, μ = overall mean, Ft = fixed effect of factor where t = treatment, Ti = repeated measure of time i, Calfm = random effect of calf m, εtim = residual error.
Ethics of experimentation
The location of this experiment was a common dairy farm (Bos-Frucht Agricultural Production, Processing and Marketing Co-operative) with operating permit number SOI/31/752-2/2014 (National Food Chain Safety Office, Hungary). This ensures that the procedures comply with the legal regulations.
Results
Feed intake, body weight, body weight gain
Inclusion of prebiotics in the milk replacer had no effect on calf starter intake during the study (). In the period of weaning the calves consumed 1500–2000 g of starter feed in all three groups.
Calves receiving milk replacers supplemented with MOS or inulin did not present differences in body weight and daily body weight gain ().
Table 4. Body weight (kg) and body weight gain (g/day), (mean ± SE).
Blood clinicochemical parameters
Blood clinicochemical variables of calves on days 30 and 60 are presented in . Calves fed inulin supplemented milk replacer had lower total protein concentration than control animals on day 30. Serum total protein level of calves fed inulin was significantly lower than that of the control and MOS group (P < 0.05) on day 60. Other blood metabolites in HF female calves were not affected by treatments. Although not part of the analysis of prebiotics’ effect, but the level of some blood parameters showed substantial quantitative changes in response to age (). Serum concentration of total protein and urea increased in calves with their age. Glucose levels decreased in all calves with advancing age.
Table 5. Blood clinicochemical variables of calves on days 30 and 60 (mean ± SE).
Composition of the faecal microbiota
Neither supplementation exerted an effect on the faecal occurrence of E. coli + coliforms and Bacteroides (). Count of Clostridium perfringens significantly increased on day 15 in faeces of calves receiving milk replacer supplemented with inulin compared with other treatments. Apart from the change described for Clostridium, the number of total aerobic bacteria in the faeces of control animals was significantly lower than in inulin treatment. The inulin supplementation significantly increased the number of Lactobacillus and total bacterial count of anaerobic microorganisms in faecal samples collected on day 30 compared to the calves fed MOS.
Table 6. Changes induced by the treatments in the faecal microbiota composition of calves on days 15 and 30 (mean ± SE).
Discussion
Feed intake, body weight, body weight gain
Body weight, feed intake and daily body weight gain of calves were similar between treatments throughout the study. Morrison et al. (Citation2010) and Heinrichs et al. (Citation2013) reported that 10 and 1 g MOS/day supplementation in milk replacer on HF calves resulted in increased feed intake. This statement is not supported by our data. The feed intake values measured during the present study fell short of those obtained by Hill et al. (Citation2008), Morrison et al. (Citation2010) and Uzmay et al. (Citation2011). This might be due to differences in the dry matter supply of calves. While in their study Morrison et al. (Citation2010) and Hill et al. (Citation2008) provided the calves with 600–680 g of milk replacer daily, in our study the calves received a daily allowance of 725–1305 g dry matter from milk replacer.
We did not find statistically significant differences between the control and the treated groups in body weight gain either, which is at variance with the reports of some other researchers (Masanetz et al. Citation2010; Król Citation2011; Ghosh and Mehla Citation2012; Heinrichs et al. Citation2013), according to which inulin and MOS increased the daily body weight gain. The values measured and calculated by us correspond to the data of the literature, which vary widely as a result of the already mentioned differences in the feeding technologies. The body weight gains recorded by Hill et al. (Citation2008), Uzmay et al. (Citation2011), Silva et al. (Citation2012) and Kara et al. (Citation2015) are lower than the values obtained in our study. These differences can be explained by the different milk replacer and feeding technologies applied in the cited experiments. In addition, previous studies have shown that when evaluating the effects of prebiotics, the environment affects the results obtained (Quigley et al. Citation1997). In case of stress inclusion of such supplements had significant benefits (Abe et al. Citation1995), whereas Jenny et al. (Citation1991) found no health promoting effects of dietary supplements on calves were under little stress.
Blood clinicochemical parameters
The treatments had no effect on the blood glucose, urea, albumin, triglyceride and total cholesterol levels of the animals. This is in agreement with the findings of Silva et al. (Citation2012), who reported that supplementation with 4 g MOS in the period of milk or milk replacer feeding did not affect the glucose, ammonia and butyric acid supply of the animals. It is, however, in contrast with the statement of Król (Citation2011), who reported that supplementation with 2 g or 4 g MOS per day increased the blood glucose level, while supplementation with the same amounts of inulin led to a decrease in the blood urea level. Other researchers (Jacques and Newman Citation1994) found that blood urea level tended to be lower when MOS was added at a rate of 2 g per feeding while similar to our results albumin was unaffected by supplement. We found significant treatment-related difference only in the total protein content of the blood, which was the lowest in the calves receiving inulin supplementation at both ages. Significant decrease in blood total protein level might be due to increased fermentation at the small intestine followed by increased flow of microbial nitrogen at large intestine, stable microflora at rumen, small and large intestine of calves (Verdonk et al. Citation1998).
Composition of the faecal microbiota
Prebiotics are found to reduce the population of harmful gut microflora namely Clostridium, Bacteriodes, Enterococcus, Enterobacteria etc. (Samanta et al. Citation2013). Inulin and MOS are used in calf diets to eliminate harmful bacteria from the intestine and help improve the health of animals. It is possible that these compounds affect microbial ecosystem by increasing the number of potentially beneficial bacteria and decreasing potential pathogens.
MOS are capable of binding to a variety of bacteria, including enteric pathogens and prevent the adherence of these bacteria to gut epithelial cells (Thomas Citation2010). Our experimental data did not support earlier statements. Inclusion of MOS in milk replacer to pre-weaned calves had no effect on microbiota of faeces compared to unsupplemented control animals The changes of the composition of faecal microbiota correspond to the finding of Kara et al. (Citation2015) that a MOS supplementation of 7 g/day had no effect on the faecal Clostridium perfringens and E. coli. Terré et al. (Citation2007) saw no effect of MOS on faecal coliform counts. Dildey et al. (Citation1997) found only a tendency in lowering faecal coliform concentration in response to MOS at the first sampling time, however the number of coliform was higher at the second. Inclusion of MOS into milk replacer did not effect faecal clostridial and coliform concentrations in an earlier study (Newman et al. Citation1993). In turn, Ghosh and Mehla (Citation2012) reported lower faecal coliform content in calves fed MOS supplemented diet (4 g MOS/day) compared with control group. Kara et al. (Citation2015) found that MOS supplementation decreased the counts of lactobacilli. Indirect factor like environment can cause varying results across research studies with MOS. Halas and Nochta (Citation2012) concluded that MOS in monogastric species can efficiently reduce the number of pathogens during an infection but results in a clean environment are inconsistent.
Inulin had a significant effect on the faecal microbiota in two cases compared to the control. Count of Clostridium perfringens and total aerobic bacteria significantly increased on day 15 in calves fed inulin supplemented milk replacer. The change observed for Clostridium perfringens also showed a significant increase relative to MOS. Higher faecal count of Clostridium perfringens in inulin treated calves is unexpected and remains to be studied later. Inulin has been proposed to have effect on immune regulation and inflammation both systemically and locally in the gut of calves (Masanetz et al. Citation2011). Decreased inflammatory status of calves fed inulin may be due to a possible decrease in intestinal pathogen load.
At day 30, significantly higher faecal number of total anaerobic bacteria and Lactobacillus was observed with inulin supplementation compared with MOS. However, Bunce et al. (Citation1995) observed decreased population of faecal E. coli and total anaerobic microflora in calves fed milk replacer supplemented with inulin owing to beneficial effect of inulin followed by its fermentation at hindgut of calves. In their in vitro experiment, Huebner et al. (Citation2007) observed that an inulin-based medium significantly (P < 0.05) supported the growth of Lactobacillus paracasei and decreased that of E. coli. Diverse stimulatory effects on beneficial bacterial subpopulations and fermentation product profiles were reported for inulin in a comparative in vitro evaluation (Rycroft et al. Citation2001).
Our results indicate that the effect of inulin is more pronounced, but the effect is only observed in the faeces of younger animals when compared to the control. Later, the bacterial number of faeces collected on day 30 showed only a difference between MOS and inulin.
In conclusion, supplementation with 18.7 g MOS or inulin during the milk-feeding period seemed to have no or little effect on animal performance, faecal flora of calves compared to untreated animals. Higher dietary amount of MOS and inulin did not indicate health benefit by promoting the growth of lactobacilli and decreasing potential pathogens in this experiment. Further research needs to explore the role of higher level of prebiotics as alternatives to antibiotics in calves suffering from dyspeptic scouring. On the other hand, further trials are necessary to evaluate the effects of MOS and inulin administration with starter diet. On referring to previous reports regarding prebiotic feeding to calves liquid feed was mostly used. Prebiotic effect on gastrointestinal tract might be modified by the type of feed (milk replacer or/and starter feed) in calves during the preweaning period. Starter feed supplemented with MOS or inulin might be another type of prebiotic utilization due to the improved colonization of specific groups of rumen microbes (e.g. lactic acid-utilizing bacteria, fibrolytic bacteria).
Disclosure statement
No potential conflict of interest was reported by the author(s).
ORCID
Melinda Kovács http://orcid.org/0000-0001-5988-3934
Additional information
Funding
References
- Abe F, Ishibashi N, Shimamura S. 1995. Effect of administration of Bifidobacteria and lactic acid bacteria to newborn calves and piglets. J Dairy Sci. 78:2838–2846. doi: 10.3168/jds.S0022-0302(95)76914-4
- Berge AC. 2016. A meta-analysis of the inclusion of Bio-Mos® in milk or milk replacer fed to dairy calves on daily weight gain in the pre-weaning period. J Anim Res Nutr. 1:19. doi: 10.21767/2572-5459.100020
- Bunce TJ, Howard MD, Kerley MS, Allece GI. 1995. Feeding fructooligosaccharides to calves increased Bifidobacterium and decreased Escherichia coli. J Anim Sci. 73(suppl.):281.
- Diaz MC, Van Amburgh ME, Smith JM, Kelsey JM, Hutten EL. 2001. Composition of growth of Holstein calves fed milk replacer from birth to 105-kilogram body weight. J Dairy Sci. 84:830–842. doi: 10.3168/jds.S0022-0302(01)74541-9
- Dildey D, Sellars K, Burrill M, Trei J, Newman K, Jacques KJ. 1997. Effect of mannanoligosaccharide supplementation on performance and health of Holstein calves. J Dairy Sci. 80(suppl.):188.
- Franklin ST, Newman MC, Newman KE, Meek KI. 2005. Immune parameters of dry cows fed mannanoligosaccharide and subsequent transfer of immunity to calves. J Dairy Sci. 88:766–775. doi: 10.3168/jds.S0022-0302(05)72740-5
- Ghosh S, Mehla RK. 2012. Influence of dietary supplementation of prebiotics (mannanoligosaccharide) on the performance of crossbred calves. Trop Anim Health Prod. 44:617–622. doi: 10.1007/s11250-011-9944-8
- Gulliksen SM, Lie KI, Løken T, Østerås O. 2009. Calf mortality in Norwegian dairy herds. J Dairy Sci. 92:2782–2795. doi: 10.3168/jds.2008-1807
- Halas V, Nochta I. 2012. Mannan oligosaccharides in nursery pig nutrition and their potential mode of action. Animals. 2:261–274. doi: 10.3390/ani2020261
- Heinrichs AJ, Jones CM, Heinrichs BS. 2003. Effects of mannanoligosaccharide or antibiotics in neonatal diets on health and growth of dairy calves. J Dairy Sci. 86:4064–4069. doi: 10.3168/jds.S0022-0302(03)74018-1
- Heinrichs AJ, Jones CM, Heinrichs BS. 2013. Fecal and saliva IgA secretion when feeding a concentrated mannanoligosaccharide to neonatal dairy calves. Prof Anim Sci. 29:457–462. doi: 10.15232/S1080-7446(15)30266-7
- Hill TM, Bateman HG, Aldrich JM, Schlotterbeck RL. 2008. Oligosaccharides for dairy calves. Prof Anim Sci. 24:460–464. doi: 10.15232/S1080-7446(15)30881-0
- Huebner J, Wehling RL, Hutkins RW. 2007. Functional activity of commercial prebiotics. Int Dairy J. 17:770–775. doi: 10.1016/j.idairyj.2006.10.006
- Jacques KA, Newman KE. 1994. Effect of oligosaccharide supplements to milk replacer on calf performance and health pre-weaning. J Dairy Sci. 77(suppl.):295.
- Jasper J, Weary DM. 2002. Effects of ad libitum milk intake on dairy calves. J Dairy Sci. 85:3054–3058. doi: 10.3168/jds.S0022-0302(02)74391-9
- Jenny BF, Vandijk HJ, Collins JA. 1991. Performance and fecal flora of calves fed a Bacillus subtilis concentrate. J Dairy Sci. 74:1968–1973. doi: 10.3168/jds.S0022-0302(91)78364-1
- Kara C, Cihan H, Temizel M, Catik S, Meral Y, Orman A, Yibar A, Gencoglu H. 2015. Effects of supplemental mannanoligosaccharides on growth performance, faecal characteristics and health in dairy calves. Asian Austral J Anim Sci. 28:1599–1605. doi: 10.5713/ajas.15.0124
- Król B. 2011. Effect of mannanoligosaccharides, inulin and yeast nucleotides added to calf milk replacers on rumen microflora, level of serum immunglobulin and health condition of calves. Electron J Pol Agric Univ. 14:18.
- Masanetz S, Preiβinger W, Meyer HHD, Pfaffl MW. 2011. Effects of the prebiotics inulin and lactulose on intestinal immunology and hematology of preruminant calves. Animal. 5:1099–1106. doi: 10.1017/S1751731110002521
- Masanetz S, Wimmer N, Plitzner C, Limbeck E, Preiβinger W, Pfaffl MW. 2010. Effects of inulin and lactulose on the intestinal morphology of calves. Animal. 4:739–744. doi: 10.1017/S1751731109991728
- Metzler-Zebeli BU, Trevisi P, Prates JAM, Tanghe S, Bosi P, Canibe N, Montagne L, Freire J, Zebeli Q. 2017. Assessing the effect of dietary inulin supplementation on gastrointestinal fermentation, digestibility and growth in pigs: A meta-analysis. Anim Feed Sci Technol. 233:120–132. doi: 10.1016/j.anifeedsci.2017.05.010
- Morrison SJ, Dawson S, Carson AF. 2010. The effects of mannanoligosaccharide and Streptococcus faecium addition to milkreplacer on calf health and performance. Livest Sci. 131:292–296. doi: 10.1016/j.livsci.2010.04.002
- Newman KE, Jacques KA, Buede RP. 1993. Effect of mannanoligosaccharide supplementation of milk replacer on gain, performance and fecal bacteria of Holstein calves. J Dairy Sci. 76(suppl.):271.
- Niness KR. 1999. Inulin and oligofructose: What are they? J Nutr. 129:1402–1406. doi: 10.1093/jn/129.7.1402S
- Quigley JD, Drewry JJ, Murray IM, Ivey SJ. 1997. Body weight gain, feed efficiency, and fecal scores of dairy calves in response to galactosyl-lactose or antibiotics in milk replacers. J Dairy Sci. 80:1751–1754. doi: 10.3168/jds.S0022-0302(97)76108-3
- Rycroft CE, Jones MR, Gibson GR, Rastall RA. 2001. A comparative in vitro evaluation of the fermentation properties of prebiotic oligosaccharides. J Appl Microbiol. 91:878–887. doi: 10.1046/j.1365-2672.2001.01446.x
- Samanta AK, Jayapal N, Senani S, Kolte AP, Sridhar M. 2013. Prebiotic inulin: Useful dietary adjuncts to manipulate the livestock gut microflora. Braz J Microbiol. 44:1–14. doi: 10.1590/S1517-83822013005000023
- Silva JT, Bittar CMM, Ferreira LS. 2012. Evaluation of mannan-oligosaccharides offered in milk replacers or calf starters and their effect on performance and rumen development of dairy calves. Rev Bras Zootecn. 41:746–752. doi: 10.1590/S1516-35982012000300038
- Swanson KS, Grieshop CM, Flickinger EA, Bauer LL, Healy H, Dawson KA, Merchen NR, Fahey Jr GC. 2002. Supplemental fructooligosaccharides and mannanoligosaccharides influence immune function, ileal and total tract nutrient digestibilities, microbial populations and concentrations of protein catabolites in the large bowel of dogs. J Nutr. 132:980–989. doi: 10.1093/jn/132.5.980
- Ten Bruggencate SJM, Bovee-Oudenhoven IMJ, Lettink-Wissink MLG, Van der Meer R. 2005. Dietary fructooligosaccharides increase intestinal permeability in rats. J Nutr. 135:837–842. doi: 10.1093/jn/135.4.837
- Terré M, Calvo MA, Adelantado C, Kocher A, Bach A. 2007. Effects of mannanoligosaccharides on performance and microorganism fecal counts of calves following an enhanced-growth feeding program. Anim Feed Sci Technol. 137:115–125. doi: 10.1016/j.anifeedsci.2006.11.009
- Thomas RJ. 2010. Receptor mimicry as novel therapeutic treatment for biothreat agents. Bioeng Bugs. 1:17–30. doi: 10.4161/bbug.1.1.10049
- Uzmay C, Kilic A, Kaya I, Özkul H, Önenc SS, Polat M. 2011. Effect of mannanoligosaccharide addition to whole milk on growth and health of Holstein calves. Arch Tierzucht. 54:127–136.
- Verdonk JMAJ, Beelan GM, Jansman AJM, Huisman J. 1998. Effect of soya carbohydrate fractions on the ileal digestibility and endogenous flow of nitrogen in veal calves. In: Jansman A.J.M., Hill G.D., Huisman J., Vander Poel A.F.B., editors. Recent advances of research in antinutritional factors in legume seeds. Wageningen, The Netherlands; p. 341–343.
- Verdonk JMAJ, Shim SB, van Leeuwen P, Verstegen MWA. 2005. Application of inulin-type fructans in animal feed and pet food. Br J Nutr. 93(suppl):S125–S138. doi: 10.1079/BJN20041355