ABSTRACT
The present study aimed to analyse the effects of exogenous surfactant administration in premature lambs on oxidative stress profile, oxygenation and lung histology. Preterm lambs (n = 22) were randomly assigned to: Control (n = 5), Single Surfactant (n = 5; single intratracheal administration of 100 mg/kg surfactant), Double Surfactant (n = 6; two equal doses of surfactant at 0 and 30 min) and Triple Surfactant (n = 6; three doses at 0, 20 and 40 min). Lambs were subjected to vitality score, oximetry, arterial blood pH, lactatemia, oxidative stress and antioxidant profile during 24 h. Lambs that died were subjected to pulmonary histology. Double group survived longer than Triple group. However, Control presented higher survival time, vitality, oxymetry and blood pH in comparison to surfactant groups. Fractionalized groups had higher vitality, oxymetry and blood pH in comparison to single group. Lactatemia of Control was lower in comparison to surfactant groups, while single group was higher than fractionalized groups. Catalase concentration was higher in fractionalized groups compared to single group. Treated groups had higher superoxide dismutase concentration compared to Control. In conclusion, single dose of intratracheal surfactant in preterm lambs compromises pulmonary adsorption and impairs neonatal antioxidant performance, leading to poor clinical and respiratory outcome, besides being preventive for excessive invasive manipulation.
Introduction
Respiratory distress syndrome (RDS) is a lung disorder of preterm neonates characterized by incomplete pulmonary maturation and deficiency of surfactant synthesis, leading to progressive alveolar atelectasis, intrapulmonary shunt, perfusion alterations and acid–base imbalance and, ultimately, severe respiratory dysfunction (Adas et al. Citation2005). Consequently, the main goal of treatment is to preserve blood oxygenation and adequate lung ventilation through prophylactic and therapeutic measures, as for example exogenous surfactant administration, ventilatory assistance and oxygen therapy.
Intratracheal instillation of surfactant is the current manner of RDS treatment or prophylaxis (Shah et al. Citation2004), reducing neonatal mortality and morbidity, such as pneumothorax and intraventricular hemorrhage of the newborn (Halliday Citation2006). The association with Continuous Positive Airway Pressure (CPAP) therapy is desired, in order to achieve a homogenous distribution of surfactant throughout pulmonary alveoli (Hilgendorff et al. Citation2006). The prophylactic strategy is performed within the first 15 min of premature birth, while pulmonary clearance is still taking place (Adas et al. Citation2005). Thus, we assume that the total volume of surfactant instilled can delay pulmonary clearance and gas-exchange, impairing the effectiveness of the procedure. Hence, our hypothesis is that the employment of fractionalized doses of intratracheal surfactant may lead to a more effective mechanism of action, ultimately enhancing pulmonary gas-exchange and overall newborn recovery.
Neonates are particularly susceptible to oxidative stress, due to an imbalance between proxidants and the antioxidant system, caused by a partially developed antioxidant defense. Consequently, generated reactive oxygen species (ROS) can lead to cellular damage, mainly brain and lung injuries (Gitto et al. Citation2002). During feto-neonatal transition, the newborn is exposed to a hyperoxic environment, which is considered an important challenge, especially for premature neonates (Buonocore et al. Citation2002; Buonocore and Groenendaal Citation2007). Hypoxia can increase oxidative stress during the perinatal period, and high plasmatic levels of ROS are frequently found in premature hypoxemic newborns at birth (Buonocore et al. Citation2002; Vento et al. Citation2003). Therefore, the more prominent hypoxia caused by the total volume of surfactant replacement via tracheal can lead to an increase in ROS formation, delaying neonatal recovery during the transition period. Hence, we expect that surfactant administration in a fractionalized manner can minimize such adverse effects.
The aim of the present research was to analyse the effects of distinct protocols of exogenous surfactant administration in premature neonatal lambs on oxidative stress profile and oxygenation.
Materials and methods
The Bioethics Committee of the Faculty of Veterinary Medicine and Animal Science, University of São Paulo (protocol number 2039/2010) approved the current study.
Animals and experimental design
We used 15 healthy ewes and their respective lambs (n = 22) belonging to the Faculty of Veterinary Medicine and Animal Science (USP) flock. Premature vaginal labour was induced to occur at 135 days of gestation, considering a term pregnancy of 150 days (Silva et al. Citation2018). Lambing induction was performed with the use of an antiprogestinFootnote1 administered twice subcutaneously every 24 h at a dose of 10 mg/kg. First application occurred 41 h prior to the estimated date of parturition.
Ewes were constantly monitored during lambing by a complete obstetric examination. Foetuses in dystocia were excluded from the experiment. Immediately at birth, neonatal lambs had their foetal membrane removed and the amniotic fluid drained from nasal surface, while being dried and vigorously stimulated by thoracic massage. Newborns were maintained in an infant warmer with a radiant warmer (articulate reflector)Footnote2 and adjustable temperature at 30–33°C during all experimental period. The oral and nasal cavity were gently aspirated, using an urethral catheter n°6 attached to a secretion aspirator. Subsequently (not more than 5 min after birth), lambs were submitted to orotracheal intubation with a cuffed endotracheal 3.0 or 3.5 tube attached to a handheld resuscitation device, self-inflating bag, of 250 mL capacity volume, with a safe valve limited to 40 ± 5 cm of H2O and air pressure gauge and equipped with a manometer. Lambs were ventilated for 2 consecutive hours with a controlled respiratory rate of 60–70 rpm (Vannucchi et al. Citation2012) and pressure between 30 and 40 cm H2O. After 2 h of manual ventilation, lambs were extubated and allowed to breathe spontaneously, while closely followed throughout a 24 h experimental period.Footnote2
Immediately after orotracheal intubation, lambs were randomly allocated to the following experimental groups:
Control Group (n = 5): newborns were only submitted to manual ventilation.
Single Surfactant Group (n = 5): newborns were submitted to manual ventilation coupled by intratracheal administration of a single volume of surfactant, immediately after orotracheal intubation.
Double Surfactant Group (n = 6): newborns were submitted to manual ventilation coupled by intratracheal administration of surfactant. The whole dose (volume of surfactant) was divided into two doses of equal volume and administrated at 0 and 30 min after orotracheal intubation, respectively.
Triple Surfactant Group (n = 6): newborns were submitted to manual ventilation coupled by intratracheal administration of surfactant. The whole dose (volume of surfactant) was divided into three doses of equal volume and administrated at 0, 20 and 40 min after orotracheal intubation, respectively.
The study was performed in a single-blind experiment for the personnel responsible for all experimental analyses. Lambs were weighed in a digital scale and the dose of 100 mg/kg of surfactant was calculated. We used a natural pulmonary surfactant of porcine origin presented in a non-pyrogenic suspension with approximately 100 mg of phospholipids, at a mean concentration of 25 mg/mLFootnote3 (Rebello et al. Citation2014). Previously heated at 37°C, intratracheal instillation of surfactant was performed with an urethral plastic 04 probe, inserted into the endotracheal tube. During instillation, lambs were positioned in a ventral decubitus, while constantly ventilating.
Newborn assessment
The complete physical examination through neonatal vitality score adapted to lambs (Vannucchi et al. Citation2012) was performed immediately after birth (0 min), 5, 10, 20, 30, 40, 50, 60, 70, 80 and 90 min, and 2, 3, 4, 5, 6 and 24 h thereafter. Additionally, we measured pulse oximetry with a sensor situated at the femoral artery topography (medial hindlimb).Footnote4
Immediately after birth (0 min), and 2, 4, 6 and 24 h thereafter, arterial blood samples were collected using heparinized sterile needles by femoral artery puncture. We used a portable analyser,Footnote5 which provided the blood pH data. Lactatemia (mmol/L)Footnote6 evaluation was performed from samples collected from jugular vein at the same time points.
Antioxidant status analysis
Analysis occurred after birth (0 min), and at 2, 4, 6 and 24 h postnatal.
The activity of thiobarbituric acid reactive substances (TBARS) was analysed in serum samples, based on the reaction of two molecules of thiobarbituric acid (TBA) with a molecule of malondialdehyde (MDA) (Nichi et al. Citation2007).
The antioxidant enzymes superoxide dismutase (SOD), glutathione peroxidase (GPx) and catalase assays were performed with the use of role blood sample, which made possible to calculate the concentration of antioxidant enzymes in the concentrated of erythrocytes (Drabkin and Austin Citation1935), expressed as U/g Hb. The activity of SOD was measured by the indirect reduction in cytochrome by the superoxide anion (Flohe and Otting Citation1984). Glutathione Peroxidase activity was measured by the consumption of NADPH, which the reaction between H2O2 and reduced glutathione (GSH) is catalyzed by the GPx (Nichi et al. Citation2006). The catalase activity was determined by evaluating the consumption of H2O2 in the spectrophotometer (Beutler Citation1975).
Lung histopathologic analysis
Newborn lambs that spontaneously died within the experimental period were submitted to a necroscopic examination and pulmonary lobectomy for further histological processing. Initially, lungs were evaluated macroscopically and the presence of gross lesions was annotated. Subsequently, lung parenchymal samples were obtained (1 cm3), preferably collected from both cranial and caudal right lung lobe and from cranial, medium and caudal left lung lobe. Fragments were washed with 0.9% saline solution and fixed in metacarn solution at ambient temperature for 12 h. Then, fragments were transferred to a 70% alcohol solution until processing. Lung fragments were embedded in paraffin, cut in 0.5-μm tissue sections, stained with hematoxylin and eosin, and evaluated under light microscopy (magnification: × 10–100). Microscopic analyses were based on a score from 0 to 3 (0: absent; 1: mild; 2: moderate; 3: intense and 4: severe) of edema, congestion, haemorrhage, hemociderosis, alveolar macrophages, monocytes, degree of parenchyma expansion, presence of neutrophils and wall thickening.
For the purpose of analysing the effect of both ventilation and surfactant therapy, cranial and caudal lung lobes of 3 premature lambs that were not subjected to experimental treatment (namely Non Ventilated-Control Group) were also histologically evaluated.
Statistical analysis
Data were statistically analysed using SAS.Footnote7 Effect of the experimental groups, time of evaluation, and interaction between these factors were estimated by repeated measures ANOVA (SAS MIXED procedure). In the absence of significant interactions, the effect of groups was analysed by merging all the evaluation moments, and conversely, evaluation times were compared by combining all groups, taking into account the Bonferroni correction (PROC GLIMMIX).
Differences between groups were analysed by an orthogonal contrast. Orthogonal comparisons were performed to determine the three main effects: effect of surfactant treatment (Control Vs. Single Surfactant Group + Double Surfactant Group + Triple Surfactant Group), effect of fractionalized surfactant doses (Single Surfactant Group Vs. Double Surfactant Group + Triple Surfactant Group) and the effect of the number of fractionalized doses (Double Surfactant Group Vs. Triple Surfactant Group). The Least Significant Difference (LSD) test was used to compare moments of evaluation within the experimental period.
Additionally for the histophatologic analysis, variables were analysed comparing three groups using the LSD test, taken into account the effects of mechanical ventilation and surfactant treatment, i.e. Non-ventilated Control Group, Ventilated Control Group and Surfactant treated Group (Single Surfactant Group + Double Surfactant Group + Triple Surfactant Group). Results are reported as untransformed means ± S.E.M and the level of significance was P ≤ 0.05.
Results
No significant interaction between moment of evaluation and surfactant treatment was observed, except for blood lactate concentration (P = 0.01).
We had a mortality rate of 60% in the Control Group, 100% in the Single Surfactant Group, 67% in the Double Surfactant Group and 100% in the Triple Surfactant Group. The Control Group had a survival time superior (P = 0.006) than treated groups. In addition, lambs subjected to double surfactant instillation survived longer (P = 0.0006) than the Triple Surfactant Group ().
Table 1. Mean ± SE of the vitality score (0–10), oximetry (%), blood pH and survival time (hours) of control and treated groups and probability values (P) of statistical contrast analysis.
The Control Group presented higher (P < 0.0001) vitality score in comparison to surfactant treated groups (). However, when lambs were subjected to fractionalized instillation of surfactant, vitality score was higher (P < 0.0001) in comparison to the single instillation group ().
Oximetry of the Control Group was higher (P < 0.0001) than surfactant treated groups (). On the other hand, fractionalized surfactant treated groups had higher (P < 0.0001) oximetry compared to lambs submitted to a single instillation of surfactant ().
Non-treated group presented higher (P = 0.004) blood pH in comparison to surfactant treated lambs (). Conversely, lambs treated with fractionalized dose of surfactant had higher (P = 0.03) blood pH than the Single Surfactant Group ().
At birth, 120 and 240 min thereafter, blood lactate of the Control Group was lower (P = 0.002, 0.01 and 0.002, respectively) in comparison to surfactant treated groups (). Blood lactate concentration of lambs subjected to a single instillation of surfactant was higher than fractionalized surfactant treated groups at 120 min (P = 0.01) and 240 min (P = 0.005). In regards to the time-point analysis, the Control Group presented higher blood lactate at 6 h after birth in comparison to 24 h thereafter. For the Single Surfactant Group, blood lactate was higher at 240 min compared to birth. In addition, blood lactate of the Double Surfactant Group had a significant decrease after 24 h after birth ().
Figure 1. Lactatemia (mmol/L) of lambs in control, single surfactant, double surfactant, triple surfactant groups. *Contrast C1 – Comparison between Control and Treated Groups (Single Surfactant Group + Double Surfactant Group + Triple Surfactant Group); †Contrast C2 – Comparison between Single Instillation (Single Surfactant Group) and Fractionalized Instillation (Double Surfactant Group + Triple Surfactant Group); a–b indicate significant differences between time (P < 0.05). •Indicates lamb mortality.
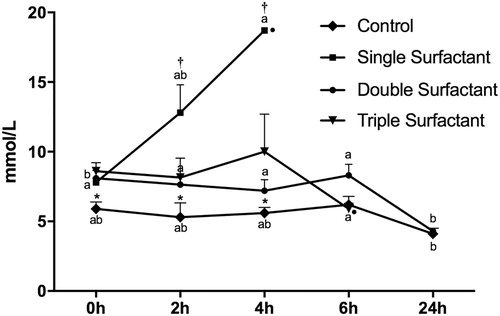
Lambs treated with fractionalized volume of surfactant showed higher blood catalase concentration (P = 0.01) comparing to the single instillation group, regardless of the moment of evaluation (). Additionally, we observed that treated groups had higher SOD concentration in comparison to Control Group (P = 0.03) (). In regards to the oxidative stress (TBARS) and GPx analysis, it was not possible to observe significant differences among groups and evaluation times ().
Table 2. Mean ± SE of serum thiobarbituric acid reactive substances (TBARS), superoxide dismutase (SOD) and glutathione peroxidase (GPx) of control and treated groups and probability values (P) of statistical contrast analysis.
No significant difference was observed among groups for edema, congestion, haemorrhage, hemociderosis and degree of parenchyma expansion for both lung lobes (cranial and caudal). However, cranial lung lobe of the Double Surfactant Group had higher score of neutrophils and lower score of monocytes and wall thickening compared to the Triple Surfactant Group (). Conversely, for the caudal lung lobe, we observed a higher score of neutrophils, alveolar macrophages and wall thickening; and lower score of monocytes in the Triple Surfactant Group compared to the Double Surfactant Group (). Additionally, the Double Surfactant Group had higher score of monocytes compared to the Single Surfactant Group. The comparison between Control and Treated Groups (Single Surfactant Group + Double Surfactant Group + Triple Surfactant Group) revealed a higher monocyte score in lambs subjected to surfactant treatment, regardless of the fractionalized protocol ().
Table 3. Mean ± SE of histologic findings (score 0–3) of the cranial lung lobe in control and treated groups and probability values (P) of statistical contrast analysis.
Table 4. Mean ± SE of histologic findings (score 0–3) of the caudal lung lobe in control and treated groups and probability values (P) of statistical contrast analysis.
Surfactant treated groups had higher monocyte score at both cranial and caudal lung lobe compared, respectively, to the Non-ventilated Control Group and to both Control Groups (). Moreover, lambs submitted to surfactant treatment had higher lung wall thickening compared to the Non-ventilated Control Group ().
Table 5. Mean ± SE of histologic findings (score 0–3) of lung lobes in control and treated groups.
Pulmonary expansion degree was markedly irregular for all experimental groups, mostly between cranial and caudal lobes. Hence, we observed areas of atelectasis side by side to expanded alveolar areas (), as well as hyperextension of pulmonary ducts and alveoli; and pulmonary emphysema. All groups had mild to moderate inflammatory edema, marked by granular and proteinaceous pattern of alveolar infiltrate ( and ). All ventilated groups had moderate to severe tissue congestion (). More importantly at cranial lobes, we observed a mild to severe neutrophilic infiltrate ( and ).
Figure 2. Irregular pulmonary expansion, lung lobe, ovine. Areas of atelectasis (left side) and expansion (right side) separated by a septum (arrow). H&E stain; bar = 100 μm.
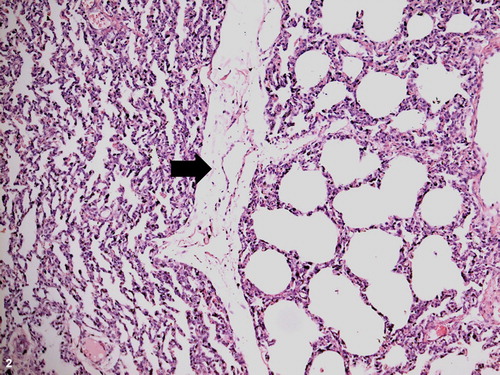
Figure 3. Pulmonary inflammation, cranial lung lobe, ovine. Diffuse and moderate edema (e), irregular alveolar expansion and congestion (arrow). H&E stain; bar = 100 μm.
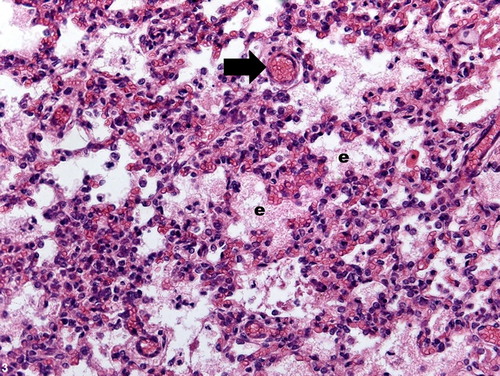
Figure 4. Pulmonary inflammation, lung lobe, ovine. Alveolar fibrin content and moderate amount of inflammatory cells dispersed within the parenchyma. H&E stain; bar = 100 μm.
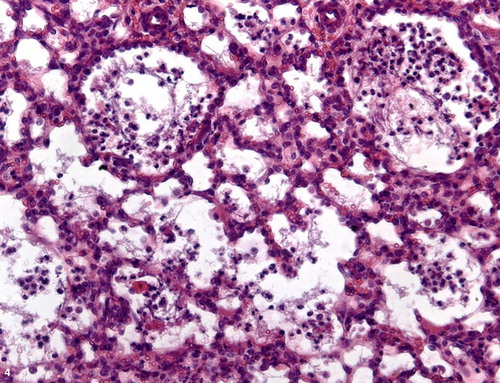
Figure 5. Pulmonary congestion, lung lobe, ovine. Septal distension with fibrin content (f) and congested vessels (arrows). H&E stain; bar = 100 μm.
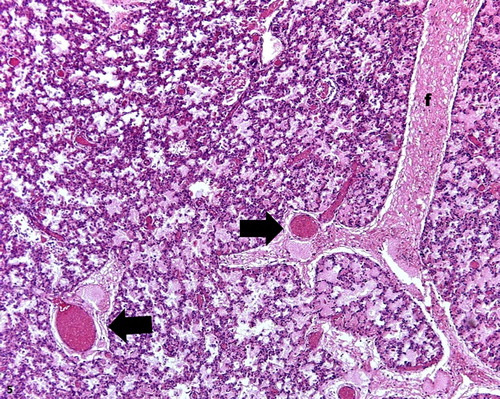
Figure 6. Intense inflammatory reaction, lung lobe, ovine. Neutrophilic infiltrate within bronchioles and alveoli. H&E stain; bar = 100 μm.
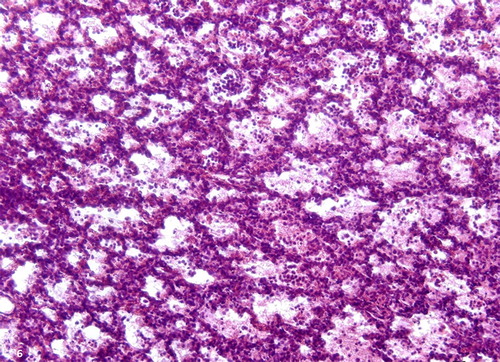
The Control Group had a positive correlation between oximetry and blood SOD (r = 0.57; P = 0.008) and catalase (r = 0.47; r = 0.03) concentration, as well as between blood pH and catalase (r = 0.72; P = 0.0001). For the Single Surfactant Group, we noticed a negative correlation between vitality score and GPx concentration (r = −0.65; P = 0.06), as well as between oximetry and TBARS concentration (r = −0.83; P = 0.01). Additionally, blood pH correlated negatively with catalase concentration (r = −0.63; P = 0.06). In the Double Surfactant Group, we observed a negative correlation between the vitality score and GPx concentration (r = −0.46; P = 0.03), while a positive correlation occurred between blood pH and SOD concentration (r = 0.41; P = 0.06). Regarding the Triple Surfactant Group, there was a negative correlation between blood lactate and SOD concentration (r = −0.6; P = 0.01).
Discussion
Although widely employed as a prophylactic measurement for RDS premature newborns (Dani et al. Citation2011; Van Kaam Citation2011), we observed that surfactant replacement immediately at birth (treated groups) promoted a survival time significantly inferior compared to the Control Group. In addition, pulse oximetry of treated groups remained below 81%, which is considered inferior to the expected oxygen saturation after 10 min of life (85–95% [Dawson et al. Citation2010]). These results indicate poor postnatal respiratory adaptation in preterm lambs submitted to prophylactic surfactant instillation. Furthermore, the Single surfactant Group showed lower peripheral oxygen saturation in relation to the fractionalized instillation groups. Therefore, we assume that intratracheal administration of surfactant, mainly at full-dose, impairs alveolar gas-exchange during the immediate postnatal period, compromising oxygenation level in the blood. Exogenous surfactant is known to compose a functional alveolar line with the endogenous surfactant film only after 2 h of instillation (Adas et al. Citation2005). However, concomitant lung disorders such as persistent pulmonary hypertension, alveolar edema, hyaline membranes or atelectasis may compromise alveolar distribution of exogenous surfactant due to excessive local dilution (edema) or sequestration. Hence, low concentration or poor distribution of exogenous surfactant interfere with the maintenance of alveolar surface activity, thus, impairing adequate oxygenation.
In this study, surfactant treated lambs had lower blood pH and higher lactatemia compared to the Control group. Additionally, neonatal lambs treated with fractionalized volume of surfactant had higher blood pH and lower lactate concentration at 120 and 240 min than single surfactant instillation. Thus, our proposed surfactant treatment protocol did not benefit preterm neonatal respiratory performance (Hughes Citation2006; Souza and Elias Citation2006), notably when a single volume instillation is employed. In fact, we observed a negative correlation between lactatemia and vitality score, indicating that lambs in lactic acidosis had severe hypoxia, clinical depression and, consequently, higher risk of mortality (Shah et al. Citation2004). Moreover, neonatal survival time and vitality score were significantly higher in the Control Group compared to treated lambs. However, among treated groups; vitality score was higher for lambs subjected to fractionalized instillation of surfactant. In addition, survival time was superior in the Double Surfactant Group, compared to lambs that received three fractionalized doses of surfactant. Therefore, we can infer that a high volume of surfactant instilled intratracheally resulted in decreased lung gas-exchange areas. However, both the Control Group and Double surfactant Group had decreased levels of serum lactate at 24 h after birth, suggesting that a compensatory mechanism is taking place, despite being partial and tardy.
Regarding the redox system, the Single Surfactant Group had significantly lower blood catalase levels during the first day of life compared to fractionalized instillation groups, indicating that the number of surfactant doses interfere with the antioxidant system related to catalase. Thus, we believe that the total volume of surfactant instilled at once had an irregular adsorption by pulmonary alveoli and therefore, an extensive area of pulmonary parenchyma remained partially collapsed, contributing to the respiratory instability. In fact, we observed irregular pulmonary expansion, with continuous areas of atelectasis and expansion in our lung histological examination. Additionally, in the Single Surfactant Group, blood pH correlated negatively with catalase concentration and oximetry correlated negatively with TBARS concentration, while in the Control group, blood pH and oximetry correlated positively with catalase, indicating that the hypoxic environment induced oxidative stress, lipid peroxidation and catalase consumption. Catalase is an extremely efficient enzyme, being saturated only when high concentration of hydrogen peroxide is formed (Lledías et al. Citation1998). As an acute antioxidant enzyme, catalase was rapidly consumed in order to counteract the local pulmonary oxidative stress in lambs subjected to a single volume of surfactant instillation. Conversely, for the fractionalized surfactant groups, with a lower volume of surfactant instilled at a time, the dispersion of surfactant by the lung was more homogeneous and efficient, leading to an improvement in respiratory performance and maintenance of blood catalase concentration.
In relation to SOD analysis, we observed that treated groups had higher blood concentration compared to the Control Group. Accordingly, higher serum total antioxidant capacity has been reported in premature infants diagnosed with RDS and treated with porcine surfactant, which was attributed to increased activity of SOD and catalase (Dizdar et al. Citation2011). Moreover, SOD concentration correlated positively with oximetry and blood pH, respectively, in the Control and Double Surfactant Group; whereas a negative correlation with lactatemia occurred for the Triple Surfactant Group, indicating that hypoxia and lactic acidosis may compromise the antioxidant activity of the superoxide dismutase system, regardless of surfactant instillation. SOD enzyme acts by reducing the rate of production of new radicals; therefore, is known as a preventive antioxidant (Lindeman et al. Citation1989). However, the extreme hyperoxic environment faced by the newborn immediately after birth requires instantaneous antioxidants, such as catalase (Alfonso-Prieto et al. Citation2009; Awad et al. Citation2011).
For the Single and Double Surfactant Group, we noticed a negative correlation between vitality score and GPx concentration. Given this information, we can suggest the participation of GPx in maintaining neonatal homeostasis, mainly regarding vital functions. In fact, GPx plays a role in foetal lung maturation, ensuring proper ventilation in an oxygen-rich extrauterine environment after birth (Davis and Auten Citation2010). Although in the present study serum GPx concentration remained unchanged, it is not possible to rule out the participation of GPx in counteracting cell oxidative damage at local pulmonary parenchyma in preterm neonatal lambs.
In the present experiment we employed the manual resuscitator or self-inflating bag in order to provide positive pressure ventilation with a hand-held device. Despite been easily handled, it was not possible to provide a constant peak of inspiratory pressure, intermediary oxygen concentration or even to administer a reliable PEEP (positive end expiratory pressure) (Almeida and Guinsburg Citation2011). Therefore, we can assume that the overall parenchyma inflammatory reaction observed at histological analysis was caused by our manual ventilation modality, irrespective of surfactant administration. In fact, Björklund et al. (Citation1997) performed distinct ventilation maneuvers with self-inflating bag concurrently with surfactant therapy in premature lambs verifying pneumothorax, local hyperextension areas, atelectasis and edema. Although the manual ventilation employed herein (with regulatory valve, controlled volume of instilled air and peak of inspiratory pressure) has prevented the occurrence of pneumothorax, we could not avoid hyperextension, atelectasis and edema. Hence, the inexistence of a controlled PEEP was the main cause for successive expansions and collapses of the airways and alveoli, leading to distinct degrees of atelectrauma and inflammatory reaction (Muscedere et al. Citation1994).
Premature newborns have low total pulmonary capacity due to generalized proteinaceous edema (Jackson et al. Citation1990), similarly to the lung content found in our Control group. Nonetheless, assisted ventilation severely worsened this pattern, leading to lesion of alveolar epithelium (Schmölzer et al. Citation2008). Interestingly, we observed that Non-treated lambs (Control group) had lower pulmonary monocyte score than Treated groups (Single Surfactant Group + Double Surfactant Group + Triple Surfactant Group), suggesting that surfactant treatment could not prevent pulmonary inflammatory reaction that may occur as a consequence of positive pressure ventilation (Sato et al. Citation2010; Dani et al. Citation2011). Therefore, we can assume that our modality of manual ventilation did not allow adequate adsorption of surfactant by pulmonary parenchyma. Therefore, it remained accumulated in the conducting airways, worsening respiratory performance, pulmonary mechanics and, ultimately, increasing inflammation.
It is interesting to point out that despite the evidence of an ongoing pulmonary inflammation, no correlation with increased oxidative stress was observed in the present experiment. Both cytokine-activated neutrophils and inflammatory mediators are responsible for the release of free radicals, which in turn are responsible for the lesion of pulmonary extracellular matrix (Schock et al. Citation2001). In fact, Dani et al. (Citation2011) attested correlation between local pulmonary inflammation and oxidative stress in ventilated premature lambs. Hence, we can suggest that our modality of manual ventilation has indeed triggered a local inflammatory process, however not enough to induce oxidative stress or even disruption of the structural support of pulmonary parenchyma. However, futures studies on local redox system and specific analysis of the extracellular matrix are needed to prove such assertive.
In addition to have positively diminished pulmonary wall thickness score, Double Surfactant Group rendered lower neutrophil, monocyte and macrophage infiltration score at caudal lung lobe in comparison to the Triple Surfactant Group. However, Double Surfactant Group had higher neutrophil score than Single Surfactant Group. These findings suggest a higher postnatal exposure of pulmonary parenchyma during repeated procedures of surfactant instillation, leading to the conclusion that the single protocol is preferred over fractionalized instillation in order to avoid facilitated contamination.
In conclusion, a single dose of intratracheal instillation of surfactant in manually ventilated preterm lambs compromises pulmonary adsorption and impairs neonatal antioxidant performance, leading to poor clinical and respiratory outcome, besides being a preventive protocol for excessive invasive manipulation. Additionally, the surfactant therapy was beneficial for superoxide dismutase activity during the first day of life. However, new strategies for surfactant administration have been developed in recent years. Therefore, further studies are necessary to better understand the oxidative profile in different strategies of surfactant administration, as well as to achieve a better intervening protocol to combat RDS in neonatal lambs.
Acknowledgements
Conceptualization was done by Liege C G Silva, Claudia B Fernandes and Camila I Vannucchi; methodology by Liege C G Silva, Daniel S R Angrimani, Fernanda M Regazzi, Cristina F Lúcio, Gisele A L Veiga, Claudia B Fernandes and Camila I Vannucchi; formal analysis by Liege C G Silva and Camila I Vannucchi; writing – original draft preparation – by Liege C G Silva, Daniel S R Angrimani and Camila I Vannucchi; writing – review and editing – by Liege C G Silva and Camila I Vannucchi.
Disclosure statement
No potential conflict of interest was reported by the author(s).
ORCID
Camila I. Vannucchi http://orcid.org/0000-0002-8198-2223
Notes
1 Aglepristone, Alizin®, Virbac.
2 Fanem®, São Paulo, Brazil.
3 Instituto Butantan®.
4 Oximax N85®, Nellcor, EUA.
5 i-STAT® portable analyzer, Abbott.
6 Accutrend® Lactate, Roche Diagnostica.
7 SAS Institute Inc., Cary, NC, USA.
References
- Adas J, Albuquerque E, Zucchi L. 2005. Síndrome do desconforto respiratório do recém-nascido. In: Adas J, Albuquerque E, Zucchi L, editors. Ventilação pulmonar mecânica em pediatria e neonatologia. 3rd ed. São Paulo: Atheneu; p. 267–280.
- Alfonso-Prieto M, Biarnés X, Vidossich P, Rovira C. 2009. The molecular mechanism of the catalase reaction. J Am Chem Soc. 131:11751–11761. doi: 10.1021/ja9018572
- Almeida MFB, Guinsburg R. 2011. Programa de Reanimação Neonatal da Sociedade Brasileira de Pediatria: Condutas. [accessed 2019 Dec 4]. www.sbp.com.br/pdfs/PRN-SBP-2011.
- Awad N, Khatib N, Ginsberg Y, Weiner Z, Maravi N, Thaler I, Ross MG, Itsokovitz-Eldor J, Beloosesky R. 2011. N-acetyl-cysteine (NAC) attenuates LPS-induced maternal and amniotic fluid oxidative stress and inflammatory responses in the preterm gestation. Am J Obstet Gynecol. 204:450.e15–450.e20. doi: 10.1016/j.ajog.2011.01.030
- Beutler E. 1975. Glutathione in red blood cell metabolism. In: Beutler E, editor. A manual of biochemical methods. 2nd ed. New York (NY): Grune and Stratton; p. 112–114.
- Björklund LJ, Ingimarsson J, Curstedt T, John J, Robertson B, Werner O, Vilstrup CT. 1997. Manual ventilation with few large breaths at birth compromises the therapeutic effect of subsequent surfactant replacement in immature lambs. Pediatr Res. 42:348–355. doi: 10.1203/00006450-199709000-00016
- Buonocore G, Groenendaal F. 2007. Anti-oxidant strategies. Semin Fetal Neonatal Med. 12:287–295. doi: 10.1016/j.siny.2007.01.020
- Buonocore G, Perrone S, Longini M, Vezzosi P, Marzocchi B, Paffetti P, Bracci R. 2002. Oxidative stress in preterm neonates at birth and on the seventh day of life. Pediatr Res. 52:46–49. doi: 10.1203/00006450-200207000-00010
- Dani C, Corsini I, Burchielli S, Cangiamila V, Romagnoli R, Jayonta B, Longini M, Paternostro F, Buonocore G. 2011. Natural surfactant combined with beclomethasone decreases lung inflammation in the preterm lamb. Respiration. 82:369–376. doi: 10.1159/000328928
- Davis JM, Auten RL. 2010. Maturation of the antioxidant system and the effects on preterm birth. Semin Fetal Neonatal Med. 15:191–195. doi: 10.1016/j.siny.2010.04.001
- Dawson JA, Kamlin COF, Vento M, Wong C, Cole TJ, Donath SM, Davis PG, Morley CJ. 2010. Defining the reference range for oxygen saturation for infants after birth. Pediatrics. 125:e1340–7. doi: 10.1542/peds.2009-1510
- Dizdar EA, Uras N, Oguz S, Erdeve O, Sari FN, Aydemir C, Dilmen U. 2011. Total antioxidant capacity and total oxidant status after surfactant treatment in preterm infants with respiratory distress syndrome. Ann Clin Biochem. 48:462–467. doi: 10.1258/acb.2011.010285
- Drabkin DL, Austin JH. 1935. Spectrophotometric studies. IV hemochromogens. J Biol Chem. 112:89–104.
- Flohe L, Otting F. 1984. Superoxide-dismutase assays. Methods Enzym. 105:93–104. doi: 10.1016/S0076-6879(84)05013-8
- Gitto E, Reiter RJ, Karbownik M, Tan D-X, Gitto P, Barberi S, Barberi I. 2002. Causes of oxidative stress in the pre- and perinatal period. Biol Neonate. 81:146–157. doi: 10.1159/000051527
- Halliday HL. 2006. Recent clinical trials of surfactant treatment for neonates. Biol Neonate. 89:323–329. doi: 10.1159/000092869
- Hilgendorff A, Reiss I, Ruppert C, Hanfstingl T, Seliger AS, Günther A, Ebsen M, Gortner L. 2006. Positive end-expiratory pressure modifies response to recombinant and natural exogenous surfactant in ventilated immature newborn rabbits. Biol Neonate. 90:210–216. doi: 10.1159/000093820
- Hughes D. 2006. Interpretation of lactate- what is it? Abstracts presented at the North American Veterinary Conference. Ithaca (NY): EUA.
- Jackson JC, MacKenzie AP, Chi EY, Standaert TA, Truog WE, Hodson WA. 1990. Mechanisms for reduced total lung capacity at birth during hyaline membrane disease in premature newborn monkeys. Am Rev Respir Dis. 142:413–419. doi: 10.1164/ajrccm/142.2.413
- Lindeman JH, Van Zoeren-Grobben D, Schrijver J, Speek AJ, Poorthuis BJ, Berger HM. 1989. The total free radical trapping ability of cord blood plasma in preterm and term babies. Pediatr Res. 26:20–24. doi: 10.1203/00006450-198907000-00008
- Lledías F, Rangel P, Hansberg W. 1998. Oxidation of catalase by singlet oxygen. J Biol Chem. 273:10630–10637. doi: 10.1074/jbc.273.17.10630
- Muscedere JG, Mullen JB, Gan K, Slutsky AS. 1994. Tidal ventilation at low airway pressures can augment lung injury. Am J Respir Crit Care Med. 149:1327–1334. doi: 10.1164/ajrccm.149.5.8173774
- Nichi M, Bols PEJ, Züge RM, Barnabe VH, Goovaerts IGF, Barnabe RC, Cortada CNM. 2006. Seasonal variation in semen quality in Bos indicus and Bos Taurus bulls raised under tropical conditions. Theriogenology. 66:822–828. doi: 10.1016/j.theriogenology.2006.01.056
- Nichi M, Goovaerts IG, Cortada CN, Barnabe VH, De Clercq JB, Bols PE. 2007. Roles of lipid peroxidation and cytoplasmic droplets on in vitro fertilization capacity of sperm collected from bovine epididymides stored at 4 and 34 degrees C. Theriogenology. 67:334–340. doi: 10.1016/j.theriogenology.2006.08.002
- Rebello CM, Precioso AR, Mascaretti RS. 2014. A multicenter, randomized, double-blind trial of a new porcine surfactant in premature infants with respiratory distress syndrome. Einstein (Sao Paulo). 12:397–404. doi: 10.1590/S1679-45082014AO3095
- Sato A, Whitsett JA, Scheule RK, Ikegami M. 2010. Surfactant protein D inhibits lung inflammation caused by ventilation in premature newborn lambs. Am J Respir Crit Care Med. 181:1098–1105. doi: 10.1164/rccm.200912-1818OC
- Schmölzer GM, Paz AB, Davis PG, Morley CJ. 2008. Reducing lung injury during resuscitation of pretern infants. J Pediatr. 153:741–745. doi: 10.1016/j.jpeds.2008.08.016
- Schock BC, Sweet DG, Ennis M, Warner JA, Young IS, Halliday HL. 2001. Oxidative stress and increased type IV collagenase levels in bronchoalveolar lavage fluid from newborn babies. Pediatr Res. 50:29–33. doi: 10.1203/00006450-200107000-00008
- Shah S, Tracy M, Smyth J. 2004. Postnatal lactate as an early predictor of short-term outcome after intrapartum asphyxia. J Perinatol. 24:16–20. doi: 10.1038/sj.jp.7211023
- Silva LCG, Regazzi FM, Lúcio CF, Veiga GAL, Angrimani DSR, Vannucchi CI. 2018. Redox, acid-base and clinical analysis of preterm and term neonatal lambs. Anim Reprod. 15:51–55. doi: 10.21451/1984-3143-2017-AR0054
- Souza MH, Elias DO. 2006. Prognostic value of lactic acidosis during infusion. Rev Latinoam Tecnol Extracorpórea. 111:14–17. Article in Portuguese.
- Van Kaam A. 2011. Lung-protective ventilation in neonatology. Neonatology. 99:338–341. doi: 10.1159/000326843
- Vannucchi CI, Rodrigues JA, Silva LCG, Lucio CF, Veiga GAL. 2012. A clinical and hemogasometric survey of neonatal lambs. Small Rumin Res. 108:107–112. doi: 10.1016/j.smallrumres.2012.05.013
- Vento M, Asensi M, Sastre J, Lloret A, García-Sala F, Viña J. 2003. Oxidative stress in asphyxiated term infants resuscitated with 100% oxygen. J Pediatr. 142:240–246. doi: 10.1067/mpd.2003.91