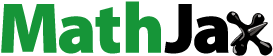
ABSTRACT
Intensive fish farms are often affected by different organisms that produce infectious diseases. To control this situation, antibiotics have been used with negative repercussions for the environment and health. As an alternative to this, probiotics are used that show more effective and respectful results with the environment. The aim of this project is to obtain new potentially probiotic strains against one of the most relevant pathogens of marine aquaculture, vibrios. These bacterial strains were isolated from the gills and intestines of European seabass, meagre and common sole. Later, were evaluated in vitro against 6 pathogenic strains of the genus Vibrio to demonstrate the production of antagonistic effects, production of antibacterial substances, resistance to bile, resistance to pH gradients, adhesion and growth to mucus, competition for nutrients and hydrophobicity. A total of 156 bacterial strains were isolated, but only 7 strains of the genera Alcaligenes, Pseudomonas, Shewanella and Proteus, showed excellent in vitro results to be considered as candidates to be reevaluated by in vivo tests, to determine their harmlessness and protective effect after challenge, and elucidating in future studies their use as possible probiotic strains for aquaculture, highlighting the results obtained with the strain Alcaligenes faecalis subsp. faecalis-1.
Introduction
Aquaculture has been considered as the fastest growing food production system in the world, however, it has been affected by the onset of infectious diseases, that suppose a limitation to the expansion of this sector because many of these pathogens are part of the normal flora of the water (Pillay Citation2004; Austin and Austin Citation2012; Sharifuzzaman and Austin Citation2017). The importance of aquaculture products will increase as a result of illegal and overfishing in the world and the increasing demand for seafood with the ever-increasing population (Kesarcodi-Watson et al. Citation2008). In southern Europe, the culture of European seabass (Dicentrarchus labrax), gilthead seabream (Sparus aurata, Linnaeus, 1758) and common sole (Solea solea) are of high importance.
Growth of aquaculture industry is hampered by unpredictable mortality, many of them caused by pathogenic microorganisms, with a less importance of non-infectious diseases (Ibrahem Citation2015). Bacterial diseases are very common in intensive aquaculture producing a huge economic loss (Sica et al. Citation2012). However, a major drawback in aquaculture is the sudden outbreak of diseases, especially those caused by different species of genus Vibrio, which are considered as major pathogenic bacteria in aquaculture (Bergh et al. Citation2001). The disease caused by pathogenic species of the Genus Vibrio affects different species of farmed marine fish of commercial interest throughout the world and is widely responsible for mortality in cultured aquaculture systems (Chen et al. Citation2000).
Antibiotics are the first option to farmers to control infectious diseases, due to rapid action and availability. However, despite of being an effective strategy in the beginning, the negative effects on environmental and public health justifying the need of developing new strategies (Hagi and Hoshino Citation2009). In order for the aquaculture sector to expand, it is necessary to find alternatives to antibiotics, safe and effective (Abdelkhalek et al. Citation2017; Yan et al. Citation2017). Due to this reason, the European Union placed restrictions on antibiotic use in aquaculture and to solve this problem, research has been focused on alternative environmentally friendly methods to control disease (Sorroza et al. Citation2012).
The use of probiotics is gaining interest in the aquaculture industry as an environmentally friendly management alternative to the use of antibiotics and other antimicrobials for disease prevention and reduce the incidence of fish diseases (Etyemez and Balcázar Citation2016). Most probiotics used in aquaculture are lactic acid bacteria or bacterial strains that belong to the genus Vibrio, Bacillus and Pseudomonas (Balcázar et al. Citation2007). These have been tested in food or added to water, and the most studied aspect has been on the improvement in animal health (Gatesoupe Citation1999). Today, there are many commercial probiotics available for mono or multi-strain bacteria (Van Doan et al. Citation2017). When selecting a new microorganism for testing as an effective probiotic, a number of properties need to be considered. To colonize the gastrointestinal tract, potential probiotics should express high tolerance to acid and bile, have the ability to adhere to intestinal surfaces and, immune modulation (Hagi and Hoshino Citation2009; Sica et al. Citation2012). Among them, potential probiotics should provide protection through the creation of a hostile environment for pathogens by the production of inhibitory compounds and by competing for adhesion sites (Etyemez and Balcázar Citation2016). To date, a wide range of bacteria proposed for their application as probiotics (Kesarcodi-Watson et al. Citation2008). However, this area is not so explored and a few probiotics are commercially available to market (Banerjee and Ray Citation2017). The use of probiotics is regarded as a very promising strategy and their wide acceptance for use in aquaculture is evidently shown in the number of research studies published over the last 10 years (Lazado and Caipang Citation2014). The aim of the present work has been to carry out the in vitro evaluation of bacterial strains isolated from the gut and gills of seabass, meagre and common sole, looking for those showing wider activity against different pathogen species of the genus Vibrio in marine aquaculture.
Materials and methods
Ethics statement
The experimental procedure with fish collected in this study fulfils the requirements contained in the Directive 2010/63/EU of the European Parliament and of the Council of September 22, 2010, on the protection of animals used for scientific purposes.
Sampling and isolation of potential probiotic strains
A total of 12 European seabass (Dicentrarchus labrax), 19 meagre (Argyrosomus regius) and 12 common sole (Solea solea) were slaughtered by immersion in anaesthetic solution with clove oil and sacrificed in liquid ice and kept in refrigeration conditions. The intestinal content of each fish (one-gram amounts) was homogenized in phosphate buffered saline (PBS), and serial dilutions (1/10–1/1000) were spread on Brain Heart Infusion Agar (BHIA), Marine Agar (MA) and Blood Agar (BA) for 48 h at 25°C. In addition, gill samples were taken directly by means of a sowing handle and spread in the same culture media. All isolates strains were tested for antagonistics effects as potential probiotic strains.
Antagonistic effect of potential probiotic strains against Vibrio
The production of antagonistic effect was analysed using different species and strains of the genus Vibrio () following the method described previously by Austin et al. (Citation1992). Vibrio strains and potential probiotic strains were grown in Brain Heart Infusion Broth (BHIB) for 24 h at 25°C to obtain an exponential phase culture. Then, 100 μl of different pathogen strains (107 CFU/ml) were spread on BHIA and BA, and each isolated strain of the fish was put into the plate. Inoculated plates were incubated at 25°C for a maximum of 48 h until a halo of inhibition is observed. Strains with positive halo production were identified by MALDI-TOF mass spectrometry system (Autoflex III, Bruker Daltonics GmbH) (Croxatto et al. Citation2012; Bizzini and Greub Citation2010).
Table 1. Pathogenic strains used in testing antagonistic effect.
Production of antibacterial substances of probiotics against pathogens
In order to determine the production of antibacterial substances in bacterial supernatants we followed the well difusión method described by Nikoskelainen et al. (Citation2001) with modifications (Kim and Austin Citation2008). Potential probiotic strains were cultured in BHIB 24 h at 22°C, centrifuged at 2000 g (Sigma, 4-16KS) for 10 min and the supernatants sterilized through 0.45 μm-pore-size filters and lyophilized using a freeze dryer (Telstar, Cryodos-50) for 18 h. The freeze-dried supernatant was re-suspended in 100 µl of PBS (10 times concentrated) to be challenged against pathogens. Vibrio strains were grown in BHIB overnight and transferred evenly onto TSA and BA plates. Then, 10 µl of lyophilized sample was added to each well, made with sterile Pasteur pipette, and the inhibition zone was observed after incubation for 24 h.
Fish bile and pH resistance
Fish bile was obtained from sea bass (average 400 g body weight) with a previous 24 h fast. Once sacrificed with oil of clove and immersion in liquid ice, we proceed to the extraction of the biliary secretion by direct puncture under sterile conditions with a fine needle. To analyse the resistance to fish bile, a 100 μl aliquot of fresh bile of seabass was added to 900 μl of each potential probiotic strain analysed at 107 CFU/ml. In order to determine the resistance at acid pH, 100 μl of same concentrations of strains tested was added 100 ml of PBS with a pH range 3–7. In both test, samples were incubated for 90 min at 22°C and serially diluted in PBS and determined by plate counting on TSA (Nikoskelainen et al. Citation2001).
Surface characteristics: hydrophobicity
The hydrophobicity is used as an indicator of the ability of the bacteria to adhere to tissues, and was analysed by the microbial adhesion to hydrocarbons method (Ocaña et al. Citation1999). 600 μl of xylene (Panreac) is added to 2.4 ml of bacterial suspension with absorbance from 0.4 to 0.6 (OD 600) and vortexed vigorously for 90 s. They were kept still to allow the immiscible solvent and aqueous phase to separate for 30 min. The aqueous layer was carefully removed, transferred to clean tubes and measured the absorbance by spectrophotometry (Selecta, V-1100). The percent of hydrophobicity (H%) was obtained from the following formula:
The cellular hydrophobicity is classified as high (71–100%), medium (36–70%) and low (0–35%).
Adhesion in intestinal and skin mucus
Intestinal and skin mucus was isolated from healthy sea bass of 400 g of average body weight. All mucus was centrifuged twice at 12,000 g for 5 min to remove particulate and cellular material, and adjusted to 0.5 mg/ml protein in PBS by Bradford Protein assay. The percentage of adhesion to intestinal and skin mucus was evaluated following the methodology described by Etyemez and Balcázar (Citation2016). Briefly, 100 µl of each mucus was deposited in polystyrene plates overnight at 4°C. The wells were washed twice with sterile PBS and 100 µl of a bacterial suspension of each potentially probiotic strain is added at a concentration of 10 9 CFU/ml in PBS and then incubated for 1 h at 22°C. The plates were washed to release the bacteria that remained attached to the polystyrene plate, 0.5% Triton X-100 was added to each well for 5 min. Adhesion was expressed as the percentage of bacteria recovered after adhesion in relation to the number of bacteria initially added to each well.
Growth in intestinal and skin mucus
The intestinal and skin mucus isolated from sea bass was diluted in sterile PBS to a final protein concentration of 0.5 mg/ml. Then, 3 ml of diluted mucus was added to 10 ml of an overnight culture of each potentially probiotic strain, and incubated at 22°C for 22 h in a shaking incubator. Samples with BHIB and PBS were used as negative controls. The growth rate of each strain in intestinal and skin mucus, BHIB and PBS was measured by monitoring the optical density at 540 nm and serial dilutions on TSA (Olsson et al. Citation1992).
Inhibition of growth in co-culture
Potential probiotics and Vibrio strains were cultured overnight in trypticasein soybean medium (TSB), centrifuged at 2000 g for 10 min, performing two washes with sterile PBS. Bacterial suspension was adjusted by spectrophotometry at 0.5 absorbance at 600 nm. Then, 100 μl of each bacterial suspension (probiotic and pathogen), were mixed in 1ml of TSB medium and incubated at 22°C for 48 h, and serially diluted in PBS and determined by plate counting on TSA (Nikoskelainen et al. Citation2001).
Statistical analysis
All experiments were carried out in triplicated using the univariate general model. Data were analysed by two-way analysis of variance (ANOVA) using the statistical package SPSS for Windows version 22.0 (SPSS). Differences were considered statistically significant when p < 0.05.
Results
A total of 156 bacterial strains were recovered from the intestinal gut and gills of the different species of fish sampled, but only 7 strains showed inhibitory effect against at least, one of the pathogens tested of the genus Vibrio. shows the identification of these potential probiotic strains by the MALDI-TOF mass spectrometry system, as well as their profiles of growth inhibition against the vibrio strains analysed. Of the 7 strains that have presented inhibition of growth against pathogens used, the strain Alcaligenes faecalis subsp. faecalis-1 is the only one that has an inhibitory effect against all the vibrio strains tested. Conversely, the strains Proteus penneri-1 and Proteus penneri-2 only have an inhibitory effect against a single strain, Vibrio anguillarum 4347.
Table 2. In vitro antagonistic effect and identification of potential probiotic strains isolated from in seabass, meagre and common sole against Vibrio.
Only the strain Shewanella putrefaciens produced a zone of inhibition around the well on BHIA by well diffusion method against the tested pathogens where it showed an antagonistic effect, showing that the inhibitory effect was due to the production of antimicrobial substances in its metabolism.
As the pH decreases, the bacterial viability is reduced (), showing values below 50% at pH 3, except for the strain Proteus penneri-1 that shows a viability of 63.5%. The viability of strains Proteus penneri-1 and Alcaligenes faecalis subsp. faecalis-1 are not affected significantly (P < 0.05) by the action of bile, showing survival rates of 99.2% and 95.7%, respectively. The other probiotics tested are affected significantly by the action of the bile, showing values below 50%.
Table 3. Percentage of survival to pH gradients tested for potential probiotic strains isolated from seabass, meagre and common sole.
A total of 4/7 probiotic strains have hydrophobic capacity. The strains Shewanella putrefaciens and Pseudomonas viridiflava shows high hydrophobicity, with values of 86% and 79%, respectively. The strains Alcaligenes faecalis subsp. faecalis-1 and Alcaligenes faecalis subsp. faecalis-2 shows medium hydrophobicity (38% and 68%). The other potential probiotic strains tested show hydrophilic capacity.
All strains tested have the ability to use sea bass intestinal and skin mucus as a source of nutrients, producing a statistically significant growth after 24 h of incubation with increments greater than 1 logarithm, highlighting the Alcaligenes faecalis subsp. faecalis-1 and Proteus penneri-1. In the adhesion test, the strain Alcaligenes faecalis subsp. faecalis-3 shows the highest adhesion in the intestinal mucus (16.8%) and in the skin mucus (26.08%). The rest of the strains have an average adherence under 10%.
All potential probiotic strains tested produced a decrease in the growth of vibrio strains analysed in the test of inhibition of growth in co-culture after 24 h of incubation, but this decrease was only statistically significant for the probiotic Proteus penneri-2 against Vibrio anguillarum 4347 (78.07%) and Alcaligenes faecalis subsp. faecalis-1 against Vibrio alginolyticus (40.27%). The rest of the probiotic strains tested produce a decrease in the growth in a range between 20 and 30%.
Discussion
Use of living organisms that may show an antagonistic effect is a very useful tool to prevent against different pathogens of aquaculture (Hjelm et al. Citation2004; Makridis et al. Citation2005). Probiotics have been used in terrestrial animals and in humans for many years (Irianto and Austin Citation2002). Nowadays, the application of probiotics is used as a control method and also as a new mechanism of natural prevention against diseases of fish caused by pathogenic bacteria (Nikoskelainen et al. Citation2001).
Three relevant fish species for marine aquaculture of Spain, seabass (D. labrax), meagre (A. regius) and common sole (S. solea) were used in this work with the aim of isolate and selecting different strains with probiotic activity. The evaluation and characterization of the potential probiotic strains were carried out through the analysis of its mechanism of action in vitro.
The fact that the isolated strains produces an inhibitory effect on the growth of different marine fish pathogens of the genus Vibrio is the method that allowed us the initial preselection, to continue later with a battery of complementary tests, methodology indicated by previous works (Pan et al. Citation2008). A total of 7/156 isolates showed inhibitory capacity against different vibrio strains, highlighting the strain Alcaligenes faecalis subsp. faecalis-1, which produced inhibition of bacterial growth of all pathogenic strains analysed (). Production of antibacterial substances allows us to determine if the inhibitory effect on pathogens observed in previous tests, is produced by extracellular substances. In our study, only 1/7 isolated strains analysed (Shewanella putrefaciens) produced extracellular substances able of inhibiting all fish pathogens tested. Previous studies have suggested that the inhibitory effects could be caused by the production of volatile organic acid compounds and bacteriocins (Balcázar et al. Citation2007).
In this study, the effect of bile and pH as a prior step to adhesion were evaluated, trying to simulate the passage of the bacteria through the gastrointestinal tract for subsequent colonization in the intestines (Robertson et al. Citation2000; Irianto and Austin Citation2002; Nikoskelainen et al. Citation2003), in order its long-term effect was observed, or add it continuously in the diet (Duwat et al. Citation2000; Nikoskelainen et al. Citation2001). The viability of our potential probiotic strains analysed has been affected by the action of sea bass bile. However, the real concentration of bile in fish is unknown, therefore an overestimation has been made, using 10% fresh bile, a much higher concentration than used in the assays with humans (3%) (Sorroza et al. Citation2012; Nikoskelainen et al. Citation2001). Low pH values affect the viability of the strains analysed, showing rates below 50% at pH 3, except the Proteus penneri-1 (63.5%), but this does not mean that these strains are unable to survive and colonize the intestine. We must consider that the strains are not directly exposed to stomach secretions since the bacteria will mix with food, and in addition, other elements are involved in digestion, such as the number of daily food intakes (Nikolopoulou et al. Citation2011; Nikoskelainen et al. Citation2001). In fact, the tolerance limit to acid medium is not always a previous condition of bacterial strains to be selected as probiotics.
Selected probiotics are able to increase their initial concentration, after 24 h of incubation using intestinal and skin mucus of sea bass as the sole source of nutrients. This means that the probiotic could be kept in the intestine and colonize (Ringø et al. Citation2010). The capacity of adhesion to the mucosa and its subsequent growth in the mucus of the preselected probiotics is a very important property to enable colonization and persistence in the intestinal tract (Collado et al. Citation2007; Verschuere et al. Citation2000). We analysed the adhesion of the strains both to the intestinal mucus and to the mucus of skin of sea bass, showing very high values for the strain Alcaligenes faecalis subsp. faecalis-3, and values below 10% for the rest of the strains. The hydrophobicity of the cell surface is related with the adherence of microorganisms beneficial to the intestinal tract of fish (Vázquez-Juárez et al. Citation1994), and in our study, 5/7 strains showed hydrophobicity activity.
Before beginning with subsequent studies, it is absolutely necessary to have a good enough collection of new bacterial strains showing some particular probiotic effect in order to test all together at the same conditions by in vivo studies. Here is where the importance of the present study lies.
In conclusion, in this work we have performed a screening in different marine species of fish with interest for aquaculture, looking for bacterial strains with possible probiotic effect. By in vitro tests 7 strains were selected with inhibitory activity against different pathogenic strains of the genus Vibrio, which could be suitable to carry out the in vivo studies. We also highlight the results obtained with strain Alcaligenes faecalis subsp. faecalis-1 isolated from meagre, which has a marked inhibitory effect against all the vibrio strains analysed, and shows the adequate conditions for adhesion and colonization in fish intestines.
Disclosure statement
No potential conflict of interest was reported by the author(s).
Additional information
Funding
References
- Abdelkhalek NK, Eissa IA, Ahmed E, Kilany OE, El-Adl M, Dawood MAO, Hassan AM, Abdel-Daim MM. 2017. Protective role of dietary Spirulina platensis against diazinon-induced Oxidative damage in Nile tilapia; Oreochromis niloticus. Environ Toxicol Pharmacol. 54:99–104. DOI:10.1016/j.etap.2017.07.002.
- Austin B, Austin DA. 2012. Bacterial fish pathogens: disease of farmed and wild animals, 5th ed. Dordrecht: Springer.
- Austin B, Baudet E, Stobie M. 1992. Inhibition of bacterial fish pathogens by Tetraselmis suecica. J Fish Dis. 15(1):55–61. DOI:10.1111/j.1365-2761.1992.tb00636.x.
- Balcázar JL, Vendrell D, De Blas I, Ruiz-Zarzuela I, Gironés O, Múzquiz JL. 2007. In vitro competitive adhesion and production of antagonistic compounds by lactic acid bacteria against fish pathogens. Vet Microbiol. 122(3-4):373–380. DOI:10.1016/j.vetmic.2007.01.023.
- Banerjee G, Ray AK. 2017. The advancement of probiotics research and its application in fish farming industries. Res Vet Sci. 115:66–77. DOI:10.1016/j.rvsc.2017.01.016.
- Bergh O, Nilsen F, Samuelsen O. 2001. Diseases, prophylaxis and treatment of the Atlantic halibut, Hippoglossus hippoglossus: a review. Dis Aquat Org. 48:57–74. DOI:10.3354/dao048057.
- Bizzini A, Greub G. 2010. Matrix-assisted laser desorption ionization time-of-flight mass spectrometry, a revolution in clinical microbial identification. Clin Microbiol Infect. 16:1614–1619. DOI:10.1111/j.1469-0691.2010.03311.x.
- Chen F, Liu P, Lee K. 2000. Lethal attribute of serine protease secreted by Vibrio alginolyticus strains in Kuruma prawn. Penaeus Japonicus. Zeitschrift für Naturforschung C. 55(1–2):94–99. DOI:10.1515/znc-2000-1-218.
- Collado MC, Grześkowiak L, Salminen S. 2007. Probiotic strains and their combination inhibit in vitro adhesion of pathogens to pig intestinal mucosa. Curr Microbiol. 55(3):260–265. DOI:10.1007/s00284-007-0144-8.
- Croxatto A, Prod’hom G, Greub G. 2012. Applications of MALDI-TOF mass spectrometry in clinical diagnostic microbiology. FEMS Microbiol Rev. 36:380–407. DOI:10.1111/j.1574-6976.2011.00298.x.
- Duwat P, Cesselin B, Sourice S, Gruss A. 2000. Lactococcus lactis, a bacterial model for stress responses and survival. Int J Food Microbiol. 55(1–3):83–86. DOI:10.1016/s0168-1605(00)00179-3.
- Etyemez M, Balcázar JL. 2016. Isolation and characterization of bacteria with antibacterial properties from Nile tilapia (Oreochromis niloticus). Res Vet Sci. 105:62–64. DOI:10.1016/j.rvsc.2016.01.019.
- Gatesoupe F. 1999. The use of probiotics in aquaculture. Aquaculture. 180(1-2):147–165. DOI:10.1016/S0044-8486(99)00187-8.
- Hagi T, Hoshino T. 2009. Screening and characterization of potential probiotic lactic acid bacteria from cultured common carp intestine. Biosci, Biotechnol, Biochem. 73(7):1479–1483. DOI:10.1271/bbb.80746.
- Hjelm M, Bergh Ø, Riaza A, Nielsen J, Melchiorsen J, Jensen S, Gram L. 2004. Selection and identification of autochthonous potential probiotic bacteria from turbot larvae (Scophthalmus maximus) rearing units. Syst Appl Microbiol. 27(3):360–371. DOI:10.1078/0723-2020-00256.
- Ibrahem MD. 2015. Evolution of probiotics in aquatic world: potential effects, the current status in Egypt and recent prospectives. J Adv Res. 6(6):765–791. DOI:10.1016/j.jare.2013.12.004.
- Irianto A, Austin B. 2002. Use of probiotics to control furunculosis in rainbow trout, Oncorhynchus mykiss (Walbaum). J Fish Dis. 25(6):333–342. DOI:10.1046/j.1365-2761.2003.00414.x.
- Kesarcodi-Watson A, Kaspar H, Lategan MJ, Gibson L. 2008. Probiotics in aquaculture: The need, principles and mechanisms of action and screening processes. Aquaculture. 274(1):1–14. DOI:10.1016/j.aquaculture.2007.11.019.
- Kim D, Austin B. 2008. Characterization of probiotic carnobacteria isolated from rainbow trout (Oncorhynchus mykiss) intestine. Lett Appl Microbiol. 47(3):141–147. DOI:10.1111/j.1472-765X.2008.02401.x.
- Lazado CC, Caipang CMA. 2014. Mucosal immunity and probiotics in fish. Fish Shellfish Immunol. 39(1):78–89. DOI:10.1016/j.fsi.2014.04.015.
- Makridis P, Martins S, Vercauteren T, Van Driessche K, Decamp O, Dinis M. 2005. Evaluation of candidate probiotic strains for gilthead sea bream larvae (Sparus aurata) using an in vivo approach. Lett Appl Microbiol. 40(4):274–277. DOI:10.1111/j.1472-765X.2005.01676.x.
- Nikolopoulou D, Moutou K, Fountoulaki E, Venou B, Adamidou S, Alexis M. 2011. Patterns of gastric evacuation, digesta characteristics and pH changes along the gastrointestinal tract of gilthead sea bream (Sparus aurata L.) and European sea bass (Dicentrarchus labrax L.). Comp Biochem Physiol A Mol Integr Physiol. 158(4):406–414. DOI:10.1016/j.cbpa.2010.11.021.
- Nikoskelainen S, Ouwehand AC, Bylund G, Salminen S, Lilius E. 2003. Immune enhancement in rainbow trout (Oncorhynchus mykiss) by potential probiotic bacteria (Lactobacillus rhamnosus). Fish Shellfish Immunol. 15(5):443–452. DOI:10.1016/s1050-4648(03)00023-8.
- Nikoskelainen S, Salminen S, Bylund G, Ouwehand AC. 2001. Characterization of the properties of human- and dairy-derived probiotics for prevention of infectious diseases in fish. Appl Environ Microbiol. 67(6):2430–2435. DOI:10.1128/AEM.67.6.2430-2435.2001.
- Ocaña VS, Bru E, Ruiz Holgado AAP, Nader-Macias ME. 1999. Surface characteristics of lactobacilli isolated from human vagina. J Gen Appl Microbiol. 45(5):203–212. DOI:10.2323/jgam.45.203.
- Olsson JC, Westerdahl A, Conway PL, Kjelleberg S. 1992. Intestinal colonization potential of turbot (Scophthalmus maximus)- and dab (Limanda limanda)-associated bacteria with inhibitory effects against Vibrio anguillarum. Appl Environ Microbiol. 58(2):551–556.
- Pan X, Wu T, Zhang L, Song Z, Tang H, Zhao Z. 2008. In vitro evaluation on adherence and antimicrobial properties of a candidate probiotic Clostridium butyricum CB2 for farmed fish. J Appl Microbiol. 105(5):1623–1629. DOI:10.1111/j.1365-2672.2008.03885.x.
- Pillay TVR. 2004. Pathogens in the aquatic environment. In: Pillay T.V.R, editor. Aquaculture and the environment. Oxford: Blackwell; p. 92–96.
- Ringø E, Løvmo L, Kristiansen M, Bakken Y, Salinas I, Myklebust R, Mayhew TM. 2010. Lactic acid bacteria vs. pathogens in the gastrointestinal tract of fish: a review. Aquac Res. 41(4):451–467. DOI:10.1111/j.1365-2109.2009.02339.x.
- Robertson P, O’Dowd C, Burrells C, Williams P, Austin B. 2000. Use of Carnobacterium sp. as a probiotic for Atlantic salmon (Salmo salar L.) and rainbow trout (Oncorhynchus mykiss, Walbaum). Aquaculture. 185(3–4):235–243. DOI:10.1016/S0044-8486(99)00349-X.
- Sharifuzzaman SM, Austin B. 2017. Probiotics for disease control in aquaculture. In: Austin B., Newaj-Fyzul A, editors. Diagnosis and control of diseases of fish and shellfish. John Wiley; p. 189–222.
- Sica MG, Brugnoni LI, Marucci PL, Cubitto MA. 2012. Characterization of probiotic properties of lactic acid bacteria isolated from an estuarine environment for application in rainbow trout (Oncorhynchus mykiss, Walbaum) farming. Antonie van Leeuwenhoek. 101(4):869–879. DOI:10.1007/s10482-012-9703-5.
- Sorroza L, Padilla D, Acosta F, Román L, Grasso V, Vega J, Real F. 2012. Characterization of the probiotic strain Vagococcus fluvialis in the protection of European sea bass (Dicentrarchus labrax) against vibriosis by Vibrio anguillarum. Vet Microbiol. 155(2–4):369–373. DOI:10.1016/j.vetmic.2011.09.013.
- Van Doan H, Hoseinifar SH, Dawood MAO, Chitmanat C, Tayyamath K. 2017. Effects of Cordyceps militaris spent mushroom substrate and Lactobacillus plantarum on mucosal, serum immunology and growth performance of Nile tilapia (Oreochromis niloticus). Fish Shellfish Immunol. 70:87–94. DOI:10.1016/j.fsi.2017.09.002.
- Vázquez-Juárez R, Andlid T, Gustafsson L. 1994. Cell surface hydrophobicity and its relation to adhesion of yeasts isolated from fish gut. Colloids Surf B. 2(1–3):199–208. DOI:10.1016/0927-7765(94)80035-9.
- Verschuere L, Rombaut G, Sorgeloos P, Verstraete W. 2000. Probiotic bacteria as biological control agents in aquaculture. Microbiol Mol Biol Rev. 64(4):655–671. DOI:10.1128/mmbr.64.4.655-671.2000.
- Yan J, Guo C, Dawood MAO, Gao J. 2017. Effects of dietary chitosan on growth, lipid metabolism, immune response and antioxidant-related gene expression in Misgurnus anguillicaudatus. Benefic Microbes. 8:439–449. DOI:10.3920/BM2016.0177.