ABSTRACT
This study was designed to assess variation in the development of clinical signs, the associated pathological changes and immune activation following experimental Avibacterium paragallinarum (Av. paragallinarum) infection in chicken and Japanese quail. Thirty-three, 4-week-old chicken and Japanese quail were divided into 3 groups, namely (1) naïve control (unhandled) containing 3 birds in each, (2) ‘sham-inoculated (sterile PBS inoculated) group’ with 9 birds in each and (3) ‘infected group (Av. pargallinarum infected) with 19 birds in each. Birds were inoculated with 1.8 × 108 CFU/mL of Av. paragallinarum, while sham inoculated received sterile PBS without Av. paragallinarum. Daily clinical monitoring; blood and tissue samples were collected on 0, 3, 5 and 12 days post-infection. Haemagglutination inhibition (HI) and serum oxidative index were measured to assess immune activation. Nasal discharges constituted prominent clinical signs in chicken. Japanese quail elaborated quicker, slightly higher seroconversion in the HI test. The observed immunoprotection mediated via oxidative changes (systemic malondialdehyde (MDA) level) indicated an unusual way of protection provided by inflammatory leukocytes. Trans-epithelial migration of KUL01+ cells following the putative release of chemo-attractants by turbinates epithelium was evident. Action by reduced glutathione levels (neutralizing MDA) in chicken, not in Japanese quail, suggests species-specific differences of innate immunity against infection.
1. Introduction
Infectious coryza (IC), a bacterial infection of poultry caused by Avibacterium paragallinarum (syn.Haemophilus paragallinarum), is associated with serous nasal discharge in the upper respiratory tract along with oedema of face and wattle in chicken of all age groups (Blackall and Soriano Citation2008). The infection is prevalent in intensive poultry production systems in developing countries, where stress factors linked to poor environmental conditions and the presence of multiple pathogens occur (Nighot et al. Citation2002). Chicken (Gallus gallus) is the best known host for Avibacterium paragallinarum (Av. paragallinarum), but recent reports confirm its presence in other avian species (Priya et al. Citation2012) including Japanese quail (Thenmozhi and Malmarugan Citation2013; Wahyuni et al. Citation2018).
Various disease conditions involving inflammation are associated with the production of oxidative radicals (reactive oxygen species ROS) leading to lipid peroxidation of polyunsaturated fatty acids in cell membranes (Wang et al. Citation2011). These free radicals are considered detrimental to the structural and functional integrity of an organ, but the host may apply several antioxidant mechanisms to counteract the damage (Deng Citation2010). Infection with Av. paragallinarum in chicken is associated with inflammation of the upper respiratory tract during the initial phase with a subsequent systemic spread and the generation of oxidative free radicals upon chronic exposure. Despite the detrimental effect on host tissue, oxidative stress mechanisms are suggested to play a role in the early immune protection of the host against IC (Boucher et al. Citation2014) and against infectious bronchitis virus (Cao et al. Citation2011) besides coccidian parasites in green finches (Sepp et al. Citation2012). However, the exact role of oxidative stress radicals during disease progression and protection in IC is not completely understood, and it may differ between host species. Different pathogen types may also induce different reactions. Viral infections affecting poultry, such as infectious bronchitis virus and infectious bursal disease, cause pronounced oxidative changes with ROS-mediated tissue architectural changes and damages (Wang et al. Citation2011; Rasoli et al. Citation2015) and some protection. However, no suggestions of protection offered by these reactions were found in chicken with New castle disease and Marek’s disease(Li et al. Citation1999; Guo et al. Citation2000). Almost no information is available regarding oxidative damage in poultry during bacterial infections including IC. Accordingly, the present comparative study was conducted to elucidate oxidant–antioxidant status, infection kinetics, clinical signs, macrophage kinetics and pathological changes at nasal turbinates, conjunctiva and trachea, up to 12 days after experimental infection of Av. paragallinarum in chicken and Japanese quail. In addition, levels of plasma antibody reacting with haemagglutinin, a protein implicated in pathogenesis in birds (Blackall Citation1989), were recorded.
2. Materials and methods
2.1. Birds and their husbandry
Thirty-three-day-old chickens (White leghorn layer, BV-300 strain) and Japanese quails (Coturnix japonica japonica) were purchased from Venkateshwara Hatcheries Ltd., Ludhiana, India, and Central Poultry Development Organization, Chandigarh, India, respectively. The birds were acclimatized for 30 days in an isolated and fumigated room. Antibiotic-free feed and water were provided ad libitum. Strict hygienic measures were adopted to prevent any collateral infections or secondary bacterial infections. To confirm the disease-free status of the birds, two birds from each group were sacrificed on the 25th day of their age and infra-orbital sinus swab was taken in the Haemophilus test medium (HTM) broth (Balouria et al. Citation2019) for incubation in 5–10% CO2 at 37°C for 24 h. Subsequently, samples of incubated broth were streaked onto Haemophilus test medium (HTM) agar plates and blood agar plates with the feeder culture (Staphylococcus aureus) for further incubation (37°C, 24 h), reduced oxygen concentration and 5–10% CO2 in a candle jar. The HTM broth was prepared from Haemophilus test medium agar base (HiMedia Laboratories Pvt Ltd, Mumbai, India) by dissolving the base in distilled water (manufacturer’s instructions). Following filtration (Whattman’s filter paper No.4, GE Healthcare UK Ltd), the filtrate was autoclaved at 15 psi for 15 min at 121°C, then cooled to 45°C followed by the addition of sterile-filtered horse serum @ 1:20, nicotinamide adenine dinucleotide (NAD) (HiMedia Laboratories Pvt Ltd, Mumbai, India) and Haemophilus growth supplement @ 1:100 (HiMedia Laboratories Pvt Ltd, Mumbai, India). The Haemophilus agar plates were prepared similar to the HTM broth except for the filtration step (to avoid removal of the agar base).
2.2. Bacteriology (isolate confirmation and inoculum preparation)
The Av. paragallinarum isolate used in the study was obtained from a field disease outbreak in Ludhiana district in the state of Punjab, India. IC-infected chicken with facial oedema and clear nasal discharges were sacrificed humanely by cervical dislocation and a few loopful of clear exudates from infraorbital sinus were inoculated in HTM broth and agar plates. To further confirm specificity of the pathogen, 10% sheep blood agar with S. aureus was used as the feeder culture. Incubations for all cultures were performed for 24 h at 37°C under reduced oxygen concentration (5–10% CO2) in a candle jar. Evidence of typical dew-drop like colonies around the feeder culture as well as in Haemophilus test medium agar plates with subsequent Gram’s staining was used as initial visual colony identification. The molecular confirmation of isolate identity was essentially made prior to experimental inoculation through the polymerase chain reaction (PCR) amplifying a 500 bp fragment using species-specific primers as described by Chen et al. (Citation1996). Further serovar confirmation was made through multiplex PCR as reported (Sakamoto et al. Citation2012) and was confirmed to be Av. paragallinarum serovar A.
For experimental inoculations, an overnight (∼16 h) grown bacterial culture was centrifuged at 25°C (4000g) for 10 min, and bacterial pellets were washed three times with phosphate-buffered saline (PBS, pH 7.4). Serial ten-fold dilutions were prepared to determine the bacterial cell concentration by the plate count method on Haemophilus test medium agar plates (Balouria et al. Citation2019). The colony-forming units (CFU) of bacteria in PBS wash was 1.8 × 108 CFU/mL, and was used as the inoculum for experimental infection.
2.3. Animal ethics
Ethical approval related to the present work involving chicken and Japanese quail in the experimental trial was obtained from Institutional Animal Ethical Committee (IAEC), GADVASU, Ludhiana, India, and performed according to the regulations and guidelines on animal ethics.
2.4. Experimental design
On the 28th day of their age, both chicken and Japanese quails were randomly subdivided into 3 groups, so as to make 6 subgroups separately (), namely (1) naïve/control group (birds without inoculation) containing 3 birds from each species (which were sacrificed prior to the start of experimental infection, i.e. on the 29th day of their age), (2) ‘sham-inoculated (birds injected with sterile PBS without Av. pargallinarum) group’ containing 9 birds from each species and (3) the ‘Infected group (birds inoculated with PBS containing (Av. pargallinarum) infected chicken or Japanese quail)’ with 19 birds each, respectively. At 30 days of age, each experimental bird from the designated infected group was inoculated with a fixed bacterial concentration of inoculum. Birds in the infected groups were inoculated through the intra-sinus route with PBS-washed bacterial inoculum containing 1.8 × 108 CFU/mL, whereas sham-inoculated birds were inoculated with the same volume of sterile PBS only in respective species. Three randomly birds from each indicated groups were sacrificed at the chosen time points, i.e. 3, 5 and 12 day post-infection.
Table 1. Experimental set-up showing the number of birds in different group(s), volume of inoculum used, route of administration and sampling time point considered.
2.5. Clinical signs development
Following infections and sterile PBS inoculations, birds were carefully observed daily for the development of clinical signs (at least three times a day) for 12 days. The clinical signs were recorded, graded as 1 for mild, 2 for moderate and 3 for severe intensity for each parameter(Balouria Citation2017) () and presented as (i) cumulative observation depicting the total number of birds showing that particular symptoms over a total period of 12 days (i.e. the last day of sampling in both the species) () as well as (ii) total clinical score for each day which is the sum total of scores obtained by multiplying the intensity score by the number of birds showing that particular intensity ().
Table 2. Criteria set for clinical sign evaluation.
Table 3. The clinical signs of infected chicken and Japanese quail inoculated with A. paragallinarum at 4 weeks of age.
Table 4. Total clinical signs score in chicken and Japanese quail at different intervals post – Av. Paragallinarum infection.
2.6. Re-isolation of Av. paragallinarum after infection challenge
Attempts were made to re-isolate Av. paragallinarum from a nasal swab from both chicken and Japanese quail either from the infected or uninfected group on the day of sacrifice, where collected exudate was streaked on an Haemophilus test medium agar (HTM) agar plate and incubated at 37°C under reduced oxygen concentration (5–10% CO2) in a candle jar for 24 h (Zhao et al. Citation2010; Balouria et al. Citation2019).
2.7. Haemagglutination inhibition assay
Heart blood samples without heparin were collected from the intra-cardiac route from three randomly selected birds of each species at 0, 3, 5 or 12 days post-infection. For measuring antibody titres, the HI assay described by Blackall et al. (Citation1990) was applied with slight modification. Briefly, haemagglutinin antigen (HA) was prepared from overnight grown culture of Av. paragallinarum, following centrifugation (@4000× g, for 10 min), washed with PBS and subsequent treatment with hyaluronidase (@200 U/mL) for 2 h at 37°C. Treated cells were washed twice with PBS and used as haemagglutinin antigen for the HI assay. The serum samples from each bird were adsorbed with 1% formalin fixed-chicken red blood cells (RBCs) (1:5) in a 96 well U-bottom micro plate (Tarson Pvt Ltd, India) at 37°C for 1 h. Adsorbed sera samples were diluted two-fold in PBS from 1:2 to 1:256 (each well containing 25 μL of the diluted serum). The same amount of antigen (25 μL, 4 haemeagglutinating HA units) was added to each well. The mixture was shaken well and allowed to stand for 30 min at room temperature. Finally, 50 μL of 1% formaldehyde fixed-chicken RBCs were added to each well and again kept at room temperature (25–28°C) for 15 min. Individual wells and plates were then examined for HI titres. Normal chicken serum was used as a negative control, while serum from known positive birds from our earlier infection study with Av. paragllinarum (Balouria et al. Citation2019) served as a positive control sample (Wambura Citation2010).
2.8. Malondialdehyde (MDA) and reduced glutathione (GSH) level analysis
For MDA determination, the method described by Placer et al. (Citation1966) was used. Haemolysate was first prepared by centrifugation of heparinized heart blood samples at 2500–3000 rpm for 20 min. The pelleted red blood cells were then washed three times with 0.85% normal saline solution and soon followed with continual and slow addition of distilled water with constant stirring until the initial volume of blood was reached. Around 100 μL of haemolysate were added to 1400 μL of Tris-KCl buffer followed by 30 min incubation at 37°C. Around 1500 μL of 0.8% 2-thiobarbituric acid was then added and boiled in a water bath for 10 min and followed by cooling on ice. After cooling, 3 mL of pyridine/η butanol and 1000 μL sodium hydroxide were added to the lysate, which was mixed by shaking and absorbance was recorded at 548 nm using a spectrophotometer (Microprocessor visible spectrophotometer, Macro Scientific works Pvt Ltd, Karnal Road, Delhi). A standard curve for MDA was also prepared using thiobarbituric reactive substances (data not shown). Similarly, the haemolysate from the infected, sham-inoculated and control (naive) groups was pelleted in Tri-chloroacetic acid solution, and the reduced glutathione (GSH) level was quantified by using the 5′5 ′ dithiobis reagent (Hafeman et al. Citation1974). The photometric measurement was carried out at a wavelength of 420 nm. For each of these measurements, duplicate heart blood samples were utilized from three randomly selected birds of each species at 0, 3, 5 or 12 days post-infection.
2.9. Histopathology and immunohistochemistry
Tissue samples (nasal turbinates, conjunctiva and trachea) from both sham-inoculated birds and 3, 12 day post-infected birds from each species were collected in 4% para-formaldehyde–PBS (for 24–48 h) and were subsequently transferred to 70% ethanol until processing (Deshmukh et al. Citation2013; Balouria et al. Citation2019). The tissues were dehydrated through graded alcohol series and xylene and embedded in paraffin where after tissue sections of 3–4 μm thick were cut with a microtome (Leica 2125; Leica Germany) and stored at 4°C until further use. Hematoxylin and eosin (H&E) staining was carried out for histopathology. For immunohistochemistry, a protocol by Rebel et al. (Citation2011) was applied with slight modifications. Slides were deparaffinized and rehydrated in xylene, graded series of ethanol and finally in Tris-buffered saline (TBS) for 2 min. Endogenous peroxidase activity was quenched by incubation with 1.5% hydrogen peroxide in TBS for 10 min, and antigen (KUL01+) for macrophage/dendritic cell was retrieved by incubating slides with Proteinase K solution at 37°C for 10–15 min. To block non-specific binding of antibodies, the slides were incubated in 2% bovine serum albumin (BSA) in TBS at room temperature for 10 min. Slides were then incubated with primary mouse monoclonal antibody (anti chicken KUL01+, Southern Biotech, USA) at 4°C for overnight. In order to improve specific labelling, slides were incubated with the amplifier (HiDef Detection™ Amplifier for mouse and rabbit, Cell Marque, USA) for 10 min and then incubated with the detector (HiDef Detection™ HRP Polymer Detector, Cell Marque, USA) for another 10 min. The bound antibody was then visualized by incubating slides with DAB solution for 10–15 min later the slides were counterstained with Mayer’s haematoxylin (BTL, India) for 15 s and subsequently mounted with water-soluble mounting medium (ImmunoHistomount, Sigma Aldrich, Germany). Slides incubated with 1% BSA instead of primary antibody served as the negative control. For bright field microscopy, the slides were examined using Nikon CFi-l microscope (Nikon Corporation, Japan) and photographs were captured using Nikon DSFi-2 camera. For low magnification (×4) and image capturing, the Olympus BX-51 motorized light microscope (Olympus, Japan) was used.
2.10. Statistical analysis
All statistical analyses were performed with Graph Pad Prism 4.0 software (Graph Pad Software Inc., La Jolla, CA, USA). Results of lipid peroxidation and reduced glutathione assay were evaluated using one-way ANOVA and subsequently assessed through Tukey’s multiple comparison test (Manoharan et al. Citation2005). The data were presented as mean ± SEM. For comparing clinical signs scoring data, Student’s t-test was applied using a probability level of .05. Titres against haemagglutinin (HI assay) were recorded as log2X of the reciprocal of the highest serum dilution, which showed agglutination where the geometric mean of agglutinin titres (GMT) was calculated (Wambura Citation2010).
3. Results
3.1. Clinical signs development
The birds from both the sham-inoculated (sterile PBS inoculated) groups and control (naïve) group were completely free from all clinical symptoms throughout the experiment. The clinical signs appeared at 1 day post infection (DPI) with unilateral and bilateral nasal discharges, characterized by sticking of a low-viscous fluid to the nasal passages ((A)) and continued until the last phase of the observation period with few birds showing such symptoms. Bilateral discharge persisted only up to 3 DPI and the accrued total clinical symptom score peaked at 3 DPI and declined thereafter (). The first evidence of nasal discharge in Japanese quail was noted on 7 and 8 DPI after pressing fingers over nostrils ((B)). A persistent, unilateral, mild to moderate ocular discharge in chicken prevailed at all time points. Ocular discharge was absent in Japanese quail till 10 DPI; however, incidental unilateral occurrence of ocular discharges was noted in a few Japanese quail on 11 and 12 DPI. Some chicken exhibited milder to moderate degree of both infraorbital swelling (i.e. facial swelling) and conjunctival redness either concurrently or as separate clinical entity from 3 till 11 DPI ((C)), whereas Japanese quail never showed these symptoms. They only showed mild unilateral nasal secretions on pressing nostrils ((D)). Among infected chicken and Japanese quail, the chickens were significantly affected (P < .05) (). Overall, nasal discharge, though not statistically significant, constituted the most prominent clinical symptom in the majority of infected chickens.
Figure 1. Development of clinical signs following experimentally induced infectious coryza (Av. paragallinarum serovar A) in birds at various days post-infection (DPI). (A) Chicken showing moderate nasal discharge (score 2) on 6 DPI (arrow); (B) Japanese quail showing mild nasal discharge on pressing (score 1) on 8 DPI (arrow); (C) Chicken showing swelling of supra-orbital sinus (star-shaped mark) along with mild nasal discharge (score 1) on 3 DPI (arrow) and (D) Japanese quail showing mild nasal discharge on pressing (score 1) on 12 DPI (arrow).
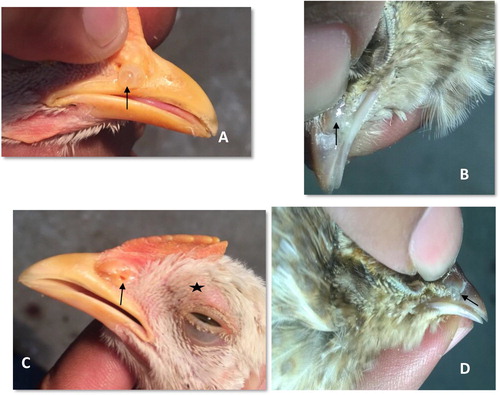
3.2. Agglutinin response to Av. paragallinarum infection
Birds inoculated with Av. paragallinarum (1.8 × 108 CFU/mL) developed agglutination titre; however, the uninfected (naïve control) and sham-inoculated (sterile PBS inoculated) birds did not develop agglutinin titres at any stage of the study (). The infected Japanese quail showed a marginal increase in agglutinin titres (GMT of 1.94 log2 to 2.63 log2) while infected chicken expressed agglutinin titres at a range of GMT 1.85 log2 to 2.40 log2, but the titres were not statistically different between the two species.
Table 5. Agglutination titre(s) in chicken and Japanese quail at 3 and 12 DPI.
3.3. Malondialdehyde (MDA) production and reduced glutathione (GSH) level analysis
Estimation of erythrocytic MDA levels from infected birds showed significant elevations at all time points after infection ((A)). Sterile PBS inoculation in the sham group also stimulated the production of MDA, however the level was considerably less and invariable. Japanese quail had higher MDA levels than chicken at all sampling time points. On the other hand, erythrocytic reduced glutathione level (GSH) did not change in Japanese quail following infection ((B)). A gradual and significant decline in the GSH level in chicken was observed until 12 DPI, but no significant impact of experimental inoculation of either infectious isolate or sterile broth on GSH was observed in either species.
Figure 2. (A) Effects of experimentally induced infectious coryza (Av. paragallinarum serovar A) on lipid peroxidation in chicken and Japanese quail at various intervals. Each data bar represents the mean ± SEM for three birds from the indicated group. Upper case letters indicate a significant difference with respect to naïve control birds of each species; lower case letters indicate a significant difference between groups as determined by Tukey’s multiple comparison tests. The overall significant difference among groups at various time points as analysed by one way ANOVA is indicated as P < .0001. (B) Effects of experimentally induced infectious coryza infection (Av. paragallinarum serovar A) on reduced glutathione levels in chicken and Japanese quail at various intervals. Each data bar represents the mean ± SEM for three birds from the indicated group. Upper case letters indicate significant difference with respect to naïve control birds of each species. Lower case letters with different case indicate a significant difference between groups as determined by Tukey’s multiple comparison tests. The overall significant difference among groups at various time points as analysed by one way ANOVA is indicated as P < .0001.
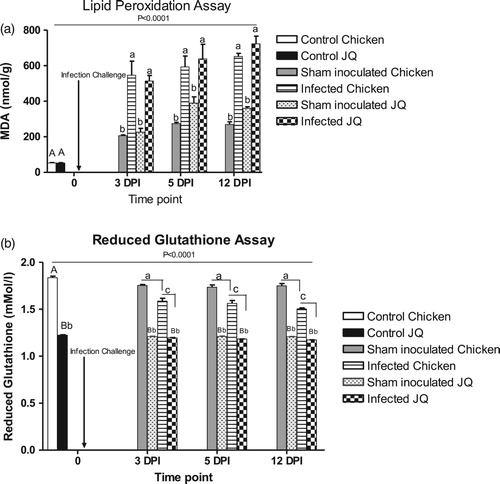
3.4. Pathological observations
Nasal turbinates: At 3 DPI, all the regions of nasal turbinates from chicken exhibited a moderate degree of damage characterized by focal necrosis and few areas of mucosal sloughing with supported heterophilic infiltrations besides severe hyperemic and oedematous changes. In addition, a wide distribution of intermingled KUL01+stained macrophages was also noted within the lamina propria of nasal conchae among all chicken. This change was more predominantly seen in lamina propria of the middle nasal conchae. There was an atrophy of mucous glands particularly of the infraorbital sinus (IOS) noted by their absolute disappearance. The initiation of papillary projections of epithelial lining appeared to begin at IOS and continued up until12 DPI. Bowman’s glands of posterior nasal conchae were spotted with casts of heterophilic leucocytes. In some instances, a number of cells with stellate shape appearance having positive KUL01+immunostaining from lamina propria were found migrating from the baso-lateral region of mucous gland to the epithelial surface, also further to luminal surface, indicating the migration of KUL01+ cells across the mucosal epithelium. Japanese quail showed a milder degree of hyperemia as well as oedematous changes within the mucosal surface. There was only minimal to very mild cellular infiltration particularly of heterophils, which predominated the inflammatory changes at middle nasal conchae, nasal septum and posterior nasal conchae. However, a few KUL01+cells were noted mainly within the sensory epithelium of posterior conchae as well as in the inferior nasal meatus (INM) nearing to the luminal end. At 12 DPI, the pathological damage in chicken was characterized by severe heterophilic infiltrations in the middle as well as in posterior nasal conchae that resulted in shrivelled alveolar mucous glands and atrophy of Bowman’s gland. INM exhibited a continual presence of heterophils in addition to massive accumulation of lymphoid cells. Loss of cilia from entire nasal conchae was predominant by that time. A considerable change in cellular infiltration was noted within the lamina propria of the nasal septum, INM and IOS distinguished by heterophilic infiltration to a moderate number of lymphocytes. Intriguingly, by this time, only few KUL01+cells were noted within the lamina propria of all nasal conchae with evidence of marked trans-epithelial migrations. At 12 DPI Japanese quail revealed lesser damages with limited heterophilic cellular infiltrations mainly within the lamina propria of middle and posterior nasal conchae. Intriguingly, the papillary-like projections of the mucosal epithelium at IOS begin to appear by this stage, which were accompanied by a drastic decline and then almost no KUL01+cells by 12 DPI.
The sham-inoculated birds from both the species did not exhibit any significant tissue changes. Only little alteration noted were hyperaemia of sub-mucosal blood vessels, oedema of lamina propria of middle nasal conchae especially on 3 DPI in two chickens and one Japanese quail, respectively. Similarly, a small amount of KUL01+ cells in the form of spindle- to stellate-shaped cells was noted coinciding with the above tissue changes only to chicken. No changes were noted on 12 DPI. (A–H), 3M-3P, describes various tissue alterations along with KUL01+ cells dynamism following Av. paragallinarum infection at nasal turbinates in both chicken and Japanese quail.
Figure 3. Pathological changes and immunohistochemical detection of KUL01+ cells in nasal and extra nasal tissues of chicken and Japanese quail following Av. paragallinarum infection. (A) Chicken, hyperaemic blood vessels, haemorrhages (multifocal) and oedematous changes in the lamina propria of middle nasal conchae at day 3 post challenge, ×100 (H&E). (B) Japanese quail, focal areas of hypaermic blood vessels within the lamina propria of middle nasal conchae at day 3 post challenge, ×100 (H&E). (C) Chicken, posterior nasal conchae showing Bowman’s glands with casts of heterophilic leucocytes and widespread heterophilic infiltrations at day 3 post challenge, ×100 (H&E). (D) Japanese quail, posterior nasal conchae with mild heterophilic infiltration and some hypaermic blood vessels at day 3 post challenge, ×100 (H&E). (E) Chicken, Posterior nasal conchae with shrivelled Bowman’s gland on day 12 post challenge, ×100 (H&E). (F) Japanese quail, Middle nasal conchae with some presence of heterophilic cells in the lamina propria at day 12 post challenge, ×100 (H&E). (G) Chicken, INM showing massive presence of lymphoid cells infiltration and lymphoid nodule formation, ×100 (H&E). (H) Japanese quail, INM with comparative lesser presence of lymphoid cells and their aggregates to chicken, ×100 (H&E). (I) Chicken, conjuctiva with the presence of normal mucosal folds and fissures prior to infection challenge, × 100 (H&E). (J) Chicken, conjuctiva with loss of mucosal fissures and the presence of marked haemorrhages and loss of lymphoid aggregates at the conjuctiva associated lymphoid tissue (CALT), ×100 (H&E). (K) Chicken, Trachea with intact mucosal epithelium and goblet cells prior to infection challenge, ×100 (H&E). (L) Chicken, trachea revealing crater-like loss of mucosal epithelium at day 3 post challenge, ×100 (H&E). (M) Chicken, middle conchae with multifocal presence of KUL01+cells within the lamina propria at day 3 post challenge, ×400 (IHC). (N) Japanese quail, posterior nasal conchae with few occasional numbers of KUL01+cells within the lamina propria at 3 day post challenge, ×400 (IHC). (O) Chicken, posterior nasal conchae with trans-epithelial migrations of KUL01+ cells at 3 day post challenge, ×400 (IHC). (P) Japanese quail, posterior nasal conchae at some places exhibited few trans-epithelial migrations of KUL01+ cells on day 3 post challenge, ×400 (IHC). (Q) Chicken, conjuctiva with trans-epithelial migration of KUL01+ cells on 12 day post challenge, ×400 (IHC). (R) Chicken, trachea with no presence of KUL01+ cells at 0 day (i.e. prior infection challenge), ×400 (IHC). (S) Chicken, trachea with trans-luminal migration of KUL01+ cells, at 3 day post infection, ×400 (IHC).
Note: Japanese quail showed no to very rare presence of KUL01+ cells at the tracheal epithelium and conjunctival surfaces. Due to poor/loss of tissue architecture after Proteinase K treatment during immunohistochemistry, the comparative photomicrographs becomes unworthy and were not included in the current panel.
Conjuctiva: Pathological alterations in conjunctiva were mainly noted in chicken at both 3 and 12 DPI, but were absent in Japanese quail. (I) explains normal conjuctival epithelium with mucosal folds prior to infection challenge. Lesions were characterized by marked loss of epithelial folds and fissures with severe hyperaemic changes. The conjunctiva-associated lymphoid tissues were dominated with massively extravasated RBCs ((J)). Interestingly, KUL01+cells in chicken conjunctiva were prominently noticed on 12 DPI as several trans-epithelial migrated cells towards the luminal surface ((Q)). However, in Japanese quail these cells were noted in the sub-epithelial connective tissue till 3 DPI without any trans-epithelial migration. No evidence of KUL01+cells were there on 12 DPI. None of the sham-inoculated birds from either of the species had exhibited any specific tissue or cellular changes.
Trachea: (K) explains the normal mucosal epithelium and goblet cells present in the trachea prior to infection challenge. Sloughing of the mucosal epithelium, distorted mucous glands and cellular influxes (heterophils and macrophages) marked the major pathological alterations in chicken at both 3 and 12 DPI ((L)). Japanese quail were affected with minimal sloughing and less cellular infiltration within the lamina propria. Prior to infection challenge, no KUL01+ cells were noted in the propria submucosa of the tracheal epithelium ((R)). Incidentally after infection, the tracheal mucosa of chicken was found to be highly positive for the KUL01+ antigen ((S)), which otherwise was absent in Japanese quail. A cellular change in the form of very mild heterophilic infiltration was only noted in the tracheal mucosal lining confined to 3 DPI in sham-inoculated chicken.
3.5. Re-isolation of Av. pargallinarum
Re-isolation was successfully achieved from nasal swabs taken from chickens sacrificed at 3 and 5 DPI and not on 12 DPI. However, in the case of Japanese quail, re-isolation of organism was only achieved on 3 and 12 DPI. No bacteria (Av. paragallinarum) could be isolated from sham-inoculated (sterile PBS) birds from either of the species at any stage of the experiment.
4. Discussion
Little is known about the oxidative damages during Av. paragallinarum infection in poultry. In the present study, we have shown differences in the development of clinical signs, levels of HI antibodies, oxidant–antioxidant status and tissue damages in the upper respiratory tract following experimental Av. paragallinarum infection of chicken and Japanese quail. These results reveal species-specific differences of host–pathogen interaction during IC and could be useful for the development of control strategies, including vaccine production.
A recent study on host susceptibility to Av. paragallinarum infection demonstrated existence of histo-anatomical differences between chicken and Japanese quail nasal turbinates. Superior, extended impervious coverings by modified squamous epithelial lining spanning the anterior nasal and middle turbinates in Japanese quail were noted (Balouria Citation2017). This observation was further supported by our immuno-co-localization study where significant hindrance to an initial establishment of Av. paragallinarum was observed in Japanese quail (Balouria et al. Citation2019). In the present study humoral components responsible for immune protection were identified, which could suggest improved immune responses and protection in Japanese quail. The first among them is HI titre among infected birds where a slightly better immune resolution was observed in Japanese quail compared to chicken. Previous studies on pathogenicity of Av. paragallinarum in poultry indicated a significant induction of immune responses to the HA antigen (Iritani et al. Citation1980; Kume et al. Citation1980; Sawata et al. Citation1980) that were correlated with protection in birds and correspondingly the host response against a particular antigenic epitope of Av. parallinarum has been largely utilized for the estimation of vaccine-mediated efficacy (Takagi et al. Citation1991; Hsu et al. Citation2007; Garcia et al. Citation2008). In the present study, a similar immune-protection due to the presence of circulatory HI antibodies was noted in Japanese quail as evident by lack of clinical sign development. Surprisingly, the HI titres in chicken, which were not statistically different from Japanese quail, failed to impart a corresponding protection against IC. This suggests that the HI titres produced in chicken may not always be protective. Almost a parallel observation was made by Garcia et al. (Citation2008), where they failed to observe protection even with the detectable presence of HI titres in chicken following single-dose challenge with Av. paragallinarum. Therefore, other protective factors may be involved.
Levels of erythrocytic MDA, a product of lipid peroxidation generated either at a circulatory cell surface or at localized tissue level are largely formed by free radicals, were different between the two bird species in the present study. A gradual rise in MDA levels after infection was observed in both species but with higher production in Japanese quail. This phenomenon could reflect an initial attempt in the host towards neutralization of IC. Previous studies have reported that oxidative radicals formed during the inflammatory process enhanceTh2 cytokine production, which leads to monocyte as well as T-cell differentiation during the infection (Elahi and Matata Citation2008; Frossi et al. Citation2008). We speculate that similar oxidative changes with resultant radicals were produced from activated polymorphonuclear (PMN) cells and macrophages which may have led to lipid peroxidation in our study (Allen et al. Citation1996; Allen Citation1997). Free radicals are toxic to pathogens but will during extended periods with inflammation affect the host physiology leading to reduced appetite and thereby decreased body mass and slow growth (Sepp et al. Citation2012), but in the present study conducted over a limited period no such physiological aberrations were noted in the infected birds. Correspondingly, experimental infection with a coccidian (protozoan) parasite in wild greenfinches (Sepp et al. Citation2012) suggested that immune protection in birds was positively correlated with oxidative damages leading to a decline in infection intensity in immunologically naïve birds, even with mild etio-pathology. Like Japanese quail, the chickens in the present study also exhibited increased MDA levels, but were not immunoprotected thereby supporting, species-specific variability in innate immunity.
Based on the present observations, it can be hypothesized that there exists a conditional imbalance between oxidative and anti-oxidative mechanisms at the tissue or cell surface level (immune cells and erythrocytes) particularly at an early stage of infection. If this balance deviates it can cause cell/tissue injury and clinical symptoms of the disease as noted for chicken in this study.
Erythrocytic-reduced glutathione level was found to be utilized (consumed) gradually in chicken and not in Japanese quail, which further indicates species-specific variations in handling of infection-inflicted tissue damages and subsequent attempt by the host for disease alleviation and protection. Glutathione is used for mitigation of oxidative radicals formed during the inflammatory process (Boucher et al. Citation2014), and our results suggest that it explains the reduced oxygen species level during Av. paragallinarum infection in birds. A possible source of these free radicals may be PMN cells (heterophils) and mononuclear cells, but glutathione seems to be used for neutralization of inflammation-induced ROS activity. Chickens were indeed found to be superfluous with heterophils and mononuclear cells like macrophages (KUL01+ cells) in the areas of infectious assault in a shorter span of time. Almost a similar mechanism was observed by Boucher et al. (Citation2014), where ROS were displayed to be consumed or restricted by GSH level constantly, without being re-synthesized for further usage. Japanese quail did not elaborate any change in their levels of reduced glutathione, which possibly explains a different mechanism for counteracting the effect of MDA-associated oxidative damage.
Our work has also showed a typical migratory pattern of chicken macrophage-like cells (KUL01+ cells) from the lamina propria to the epithelial cell surface at all major areas of nasal turbinates. This observation suggests a putative role of epithelial cells of nasal turbinates (conchae) in inducing chemotaxis for KUL01+cells. Corresponding observations were done by Kannan et al. (Citation2009) who showed alveolar Type II cells in lungs mediate monocyte chemotactic protein-1 release for immediate activation of alveolar macrophages against lipopolysaccharides cascaded attack for improved protection against Pseudomonas aeruginosa. Tissue damages recorded through histopathological examination and immunohistochemistry also confirm a better protection in Japanese quail with minimal cellular infiltrations, unique macrophage participation and minor architectural disintegrity.
5. Conclusion
This study demonstrates the existence of inherent differences in innate immunity between chicken and Japanese quail against IC caused by Av. paragallinarum. There was feeble clinical sign development in Japanese quail with quicker and slightly higher seroconversion (HI titres against HA antigen of Av. paragallinarum) as compared to chicken.
The higher susceptibility of chicken associated with higher infection and more expressed clinical symptoms may be related to excess production of free radicals. The unknown innate mechanisms by which Japanese quail keep infection and inflammation under some control should be ascertained. Further studies are required to explore these species-specific differences at the cellular level.
Disclosure statement
No potential conflict of interest was reported by the author(s).
Additional information
Funding
References
- Allen PC. 1997. Production of free radical species during Eimeria maxima infections in chickens. Poultry Sci. 76:814–821. doi: 10.1093/ps/76.6.814
- Allen PC, Danforth HD, Levander OA. 1996. Diets high in n-3 fatty acids reduce cecal lesion scores in chickens infected with Eimeria tenella. PoultrySci. 75:179–185. doi: 10.3382/ps.0750179
- Balouria A. 2017. Comparative studies on immuno-pathogenetic mechanism of avian infectious coryza in chicken and Japanese quail [M.V.Sc thesis]. Ludhiana: Guru Angad Dev Veterinary and Animal Science University.
- Balouria A, Deshmukh S, Banga HS, Ahmad A, Brar RS, Sodhi S. 2019. Early migration pattern of Avibacterium paragallinarum in the nasal passage of experimentally infected chicken and Japanese quail by immunohistochemistry. Avian Pathol. 48(2):168–177. doi: 10.1080/03079457.2018.1562153
- Blackall PJ. 1989. The avian haemophili. Clin Microbiol Rev. 2:270–277. doi: 10.1128/CMR.2.3.270
- Blackall PJ, Eaves LE, Aus G. 1990. Serotyping of Haemophilus paragallinarum by the page scheme: comparison of the use of agglutination and hemagglutination-inhibition tests. Avian Dis. 34:643–646. doi: 10.2307/1591258
- Blackall PJ, Soriano VE. 2008. Infectious coryza. In: Saif YM, Fadly AM, Glisson JR, McDougald LR, Nolan LK, Swayne DA, editors. Disease of poultry. 12th ed. Ames Iowa State Press, Iowa; p. 789–803.
- Boucher CE, Theron CW, Jansen AC, Bragg RR. 2014. Transcriptional profiling of chicken immunity-related genes during infection with Avibacterium paragallinarum. Vet Immunol Immunopathol. 158:135–142. doi: 10.1016/j.vetimm.2013.12.004
- Cao Z, Han Z, Shao Y, Geng H, Kong X, Liu S. 2011. Proteomic analysis of chicken embryonic trachea and kidney tissues after infection in ovo by avian infectious bronchitis coronavirus. Proteome Sci. 9(1):11. doi 10.1186/1477-5956-9-11.
- Chen X, Miflin JK, Zhang P, Blackall PJ. 1996. Development and application of DNA probes and PCR tests for Haemophilus paragallinarum. Avian Dis. 40:398–407. doi: 10.2307/1592238
- Deng H. 2010. An assessment of the relationship among oxidative stress, adaptive immunity and genetic variations in the chicken [PhD dissertation]. Virginia Polytechnic Institute and State University, Blacksburg, Virginia, USA.
- Deshmukh S, Kania PW, Chettri JK, Skov J, Bojesen AM, Dalsgaard I, Buchmann K. 2013. Insight from molecular, pathological, and immunohistochemical studies on cellular and humoral mechanisms responsible for vaccine-induced protection of rainbow trout against Yersinia ruckeri. Clin Vaccine Immunol. 20(10):1623–1641. doi: 10.1128/CVI.00404-13
- Elahi MMY, Matata BM. 2008. Significance of oxidants and inflammatory mediators in blood of patients undergoing cardiac surgery. J Cardiothor Vasc An. 22:455–467. doi: 10.1053/j.jvca.2007.12.022
- Frossi B, Carli MD, Pieomonte M, Pucillo C. 2008. Oxidative microenvironment exerts an opposite regulatory effect on cytokine production by Th1 and Th2 cells. Mol Immunol. 45(1):58–64. doi: 10.1016/j.molimm.2007.05.008
- Garcia A, Romo F, Ortiz AM, Blackall PJ. 2008. The vaccination-challenge trial: the gold standard test to evaluate the protective efficacy of infectious coryza vaccines. Avian Pathol. 37:183–186. doi: 10.1080/03079450801929941
- Guo DZ, Yang SJ, Wu JG, Li JK. 2000. The level of MDA and activities of SOD in broiler infected with newcastle disease virus. Chin J Prev Vet Med. 22(5):365–367.
- Hafeman DG, Sunde RA, Hoekstra WG. 1974. Effect of dietary selenium on erythrocyte and liver glutathione peroxidase in the rat. J Nutr. 104:580–587. doi: 10.1093/jn/104.5.580
- Hsu YM, Shieh HK, Chen WH, Shiang JH, Chang PC. 2007. Immunogenicity and haemagglutination of recombinant Avibacterium paragallinarum HagA. Vet Microbiol. 122:280–289. doi: 10.1016/j.vetmic.2007.01.014
- Iritani Y, Katagiri K, Arita H. 1980. Purification and properties of Haemophilus paragallinarum hemagglutinin. Am J Vet Res. 41(12):2114–2118.
- Kannan S, Huang H, Seegar D, Aude A, Chen Y, Huang C, Gao H, Li S, Wu M. 2009. Alveolar epithelial type II cells activate alveolar macrophages and mitigate P. aeruginosa infection. PloS One. 4(3):e4891. DOI:10.1371/journal.pone.0004891.
- Kume K, Sawata A, Nakase Y. 1980. Relationship between protective activity and antigen structure of Haemophilus paragallinarum serotypes 1 and 2. Am J Vet Res. 41:97–100.
- Li QZ, Hao YH, Chi YJ. 1999. Studies on principle of variation of active oxygen radical metabolism of MDV artificially infected broiler chicks. JNE Agriculture Uni. 1:50–58.
- Manoharan S, Kolanjiappan K, Suresh K, Panjamurthy K. 2005. Lipid peroxidation and antioxidants status in patients with oral squamous cell carcinoma. Indian J Med Res. 122(6):529–534.
- Nighot PK, Kolte GN, Ghalsasi GR. 2002. Physiopathology of avian respiratory diseases- respiratory diseases are often complex and multi-factorial but understanding the important factors can help in disease control. Poultry International. 41:24–29.
- Placer ZA, Cushman LL, Johnson BC. 1966. Estimation of product of lipid peroxidation (malonyl dialdehyde) in biochemical systems. Anal Biochem. 16:359–364. doi: 10.1016/0003-2697(66)90167-9
- Priya PM, Krishna S, Kumar V, Mini M. 2012. Isolation and characterization of Avibacterium paragallinarum from ornamental birds in Thrissur, Kerala. Int J Life Sci Res. 1(3):87–88.
- Rasoli M, Yeap SW, Tan SW, Roohani K, Kirsteen-Teo YW, Alitheen NB, Rahaman YA, Aini I, Bejo MH, Kaiser P, et al. 2015. Differential modulation of immune response and cytokine profiles in the bursae and spleen of chickens infected with very virulent infectious bursal disease virus. BMC Vet Res. 11:7. DOI:10.1186/s12917-015-0377. doi: 10.1186/s12917-015-0377-x
- Rebel JMJ, Peeters B, Fijten H, Post J, Cornelissen J, Vervelde L. 2011. Highly pathogenic or low pathogenic avian influenza virus subtype H7N1 infection in chicken lungs: small differences in general acute responses. Vet Res. 42:10. DOI:10.1186/1297-9716-42-10.
- Sakamoto R, Kino Y, Sakaguchi M. 2012. Development of a multiplex PCR and PCR-RFLP method for serotyping of Avibacterium paragallinarum. J.Vet Med Sci. 74(2):271–273 doi: 10.1292/jvms.11-0319
- Sawata A, Kume K, Nakase Y. 1980. Biologic and serologic relationships between Page’s and Sawata’s serotypes of Haemophilus paragallinarum. Am J Vet Res. 41:1901–1904.
- Sepp T, Karu U, Jonathan DB, Sild E, Manniste M, Horak P. 2012. Coccidian infection causes oxidative damage in greenfinches. PloS One. 7(5):e36495. Doi:10.1371/journal.pone.0036495.
- Takagi M, Hirayama N, Makie H, Ohta S. 1991. Production, characterization, and protective effect of monoclonal antibodies to Haemophilus paragallinarum serotype A. Vet Microbiol. 27:1311–1314. doi: 10.1016/0378-1135(91)90158-C
- Thenmozhi V, Malmarugan S. 2013. Isolation, identification and antibiogram pattern of Avibacterium paragallinarum from Japanese quail. Tamil Nadu J Vety Ani Sci. 9:253–258.
- Wahyuni AETH, Tabbu CR, Artanto S, Setiawan DCB, Rajaguguk SI. 2018. Isolation, identification and serotyping of Avibacterium paragallinarum from quails in Indonesia with typical infectious coryza disease symptoms. Vet World. 11(4):519–524. doi: 10.14202/vetworld.2018.519-524
- Wambura PN. 2010. Preparation and use of autogenous vaccine from Avibacterium paragallinarum (strain Tan 1-05) in layer chickens. Trop Anim Health Prod. 42:483–486. doi: 10.1007/s11250-009-9447-z
- Wang HF, Zhong X, Shi W, Guo B. 2011. Study of malondialdehyde (MDA) content, superoxide dismutase (SOD) and glutathione peroxidase (GSH-Px) activities in chickens infected with avian infectious bronchitis virus. Afr J Biotechnol. 10:9213–9217. doi: 10.5897/AJB11.782
- Zhao Q, Sun Y, Zhang X, Kong Y, Xie Z, Zhu Y, Zhou E, Jiang S. 2010. Evaluation of two experimental infection models for Avibacterium paragallinarum. Vet Microbiol. 141:68–72. doi: 10.1016/j.vetmic.2009.07.034