ABSTRACT
In this study, we aimed to test whether embryonic development of blastocysts following injection of mouse induced pluripotent stem (iPS) cells can be improved by the supplementation of antioxidants during in vitro maturation. For this, we evaluated the effects of chlorogenic acid (CGA) and insulin–transferrin–selenium (ITS) supplementation either alone or in combination during oocyte in vitro maturation on the quality of porcine blastocysts and the embryonic development after injection of mouse iPS cells. Our results demonstrate that, prior to injection of mouse iPS cells, CGA or ITS supplementation either alone or in combination had no effects on the rates of oocyte maturation, oocyte DNA fragmentation, fertilization, and blastocyst formation. However, the combination of CGA and ITS significantly increased the total cell number in blastocysts compared with the control group (P < 0.05). When mouse iPS cells were injected into porcine blastocysts, CGA supplementation either alone or in combination with ITS had a beneficial effect on the development of interspecies chimera blastocysts. In conclusion, our results indicate that supplementation of maturation medium with a combination of CGA and ITS enhances the ability of porcine oocytes to develop interspecies chimera blastocysts following iPS cell injection.
Introduction
Generation of fully pluripotent stem cell-derived tissues or organs in a chimeric animal can be produced by injecting wild-type pluripotent stem cells into blastocysts derived from a genetically deficient animal (Wu et al. Citation2017; Suchy and Nakauchi Citation2018). Following blastocyst injection, chimeric cell survival and proliferation during embryogenesis are influenced by several factors including variations in systemic contribution of the donor cell cycle and cell culture conditions (Masaki et al. Citation2016; Suchy and Nakauchi Citation2018). In the production of chimeric animals, however, obtaining high-quality blastocysts is the most critical factor for generating live chimeric animals after the transfer of embryos with the injection of pluripotent stem cells (PSCs).
In pigs, the development and quality of embryos produced by the current in vitro production system involving in vitro oocyte maturation, fertilization, and embryo culture are lower than those of in vivo-derived embryos (Kikuchi et al. Citation2008). In vitro maturation (IVM) of oocytes is considered to be the most crucial step for in vitro embryo production (IVP) (Jeong et al. Citation2008). Despite the technical developments in IVM over the years, a major limitation of the in vitro culture system is the imbalance between oxygen concentration and antioxidant protection, resulting in an increase in the generation of reactive oxygen species (ROS), which in turn increase the oxidative stress of oocytes (Agarwal et al. Citation2003; Agarwal et al. Citation2006). The supplementation of chlorogenic acid (CGA), known to have free radical scavenging properties (Gonthier et al. Citation2006; Castro et al. Citation2018), to the maturation medium has shown potential in improving maturation, fertilization and developmental competence of porcine oocytes (Nguyen et al. Citation2017; Nguyen et al. Citation2018). In addition, insulin–transferrin–selenium (ITS) has been routinely used in several IVM systems to promote the growth of oocytes retrieved from sheep (Mondal et al. Citation2015) and mouse (De La Fuente et al. Citation1999). As a polypeptide hormone, insulin promotes the uptake of glucose and amino acids and thus improves the developmental competence of porcine oocytes (Kikuchi et al. Citation1999; Kim et al. Citation2005). Transferrin has a prominent growth-promoting activity that exerts a supportive effect on in vitro embryo development through the binding of iron (Fe2+), making it less toxic (Natsuyama et al. Citation1993). Selenium functions as an essential component of glutathione peroxidase, which protects cells and tissue from free radicals and oxidative stress (Michiels et al. Citation1994). Therefore, the use of CGA and ITS either alone or in combination may not only prevent the adverse effects of oxidative stress during IVM but also improve the quality of the resulting blastocysts.
The present study aimed to examine the effects of CGA and ITS supplementation into the maturation medium on the quality of porcine blastocysts and the development of blastocysts after mouse induced pluripotent stem (iPS) cell injection to produce interspecies chimera.
Materials and methods
General
All procedures were approved by the Animal Research Committee of Tokushima University.
Oocyte collection and in vitro maturation
In vitro maturation was performed according to methods described by Namula et al. (Citation2013) with minor modifications. Briefly, porcine ovaries were obtained from approximately 6-month-old gilts at a local slaughterhouse and were transported within 1 h to the laboratory in physiological saline at 30°C. The follicles on the ovarian surface were sliced using a surgical blade in a sterilized dish under a stereomicroscope and the cumulus oocyte complexes (COCs) were collected. Approximately 50 COCs were then cultured in 500 μL of maturation medium for 22 h in 4-well dishes (Nunc A/S, Roskilde, Denmark) covered with mineral oil (Sigma-Aldrich, St. Louis, MO, U.S.A.). Maturation medium consisting of tissue culture medium 199 with Earle’s salts (TCM 199; Thermo Fisher Scientific, Waltham, MA, U.S.A.) was supplemented with 10% (v/v) porcine follicular fluid, 50 μM sodium pyruvate (Sigma-Aldrich), 2 mg/mL D-sorbitol (Wako Pure Chemical Industries Ltd., Osaka, Japan), 0.6 mM cysteine (Sigma-Aldrich), 10 IU/mL equine chorionic gonadotropin (Asuka Pharmaceutical, Tokyo, Japan), 10 IU/mL human chorionic gonadotropin (Nippon Zenyaku Kogyo, Fukushima, Japan), and 50 μg/mL gentamicin (Sigma-Aldrich). Subsequently, the COCs were transferred into maturation medium without hormone supplementation and cultured for an additional 22 h. COCs were incubated at 39°C in a humidified incubator containing 5% CO2 in air.
Assessment of meiotic status and DNA fragmentation
To assess the meiotic status and DNA fragmentation of oocytes following IVM, some oocytes were fixed at 44 h of culture and analysed using a technique for simultaneous nuclear staining with DAPI (Nacalai Tesque Inc., Kyoto, Japan) and terminal deoxynucleotidyl transferase nick-end labelling (TUNEL) according to the method described by Do et al. (Citation2015). Based on their chromatin configuration, they were classified as ‘germinal vesicle’, ‘condensed chromatin’, ‘metaphase I’, or ‘metaphase II’. Oocytes with diffusely stained cytoplasm characteristic of nonviable cells and those in which chromatin was unidentifiable or not visible were classified as ‘degenerated’ and excluded from DNA damage analysis.
In vitro fertilization and assessment
In vitro fertilization (IVF) was performed according to the method described by Nguyen et al. (Citation2017). Freeze-thawed spermatozoa obtained from a boar were transferred into 6 mL of porcine fertilization medium (PFM; Research Institute for the Functional Peptides Co., Yamagata, Japan) and washed by centrifugation at 500 × g for 5 min. The pelleted spermatozoa were re-suspended in PFM and adjusted to a cell density of 1 × 106 cells/mL. Oocytes were then transferred to the sperm-containing PFM and co-incubated for 5 h at 39°C, in a humidified incubator containing 5% CO2, 5% O2 and 90% N2.
To assess oocyte fertilization, some presumptive zygotes were mounted on glass slides 10 h after insemination and fixed with acetic acid:ethanol (1:3, v/v) for 48–72 h. The fixed zygotes were stained with acetic orcein (1% orcein in 45% acetic acid) and examined by phase contrast microscope. Oocytes containing both female and male pronuclei were considered to be fertilized and were categorized as monospermic or polyspermic depending on the number of swollen sperm heads and/or pronuclei in the cytoplasm (Do et al. Citation2015). The proportion of monospermic fertilization was calculated by dividing the number of monospermic fertilized oocytes by the total number of fertilized oocytes.
In vitro culture and assessment of blastocyst quality
After the co-incubation, the inseminated oocytes were denuded from the cumulus cells and attached spermatozoa by mechanical pipetting. Approximately 50 denuded presumptive zygotes were subsequently cultured in 500 μL PZM-5 (Research Institute for the Functional Peptides Co.) in 4-well dishes. The presumptive zygotes were cultured in vitro at 39°C in a humidified incubator with 5% CO2, 5% O2 and 90% N2. The cleaved embryos were transferred into 500 μL porcine blastocyst medium (PBM; Research Institute for the Functional Peptides Co.) 72 h after insemination, and were cultured for an additional 4 days to evaluate their ability to develop to the blastocyst stage. All embryos were fixed on Day 7 (Day 0 = insemination) and stained with Hoechst 33342 (Sigma-Aldrich). Their cell numbers were counted to assess the quality and development stage of the embryos.
Preparation of mouse iPS cells
The mouse iPS line-iPS-Stm-FB/gfp-99-1 (Aoi et al. Citation2008) which expresses a green fluorescent protein (GFP) was provided by RIKEN Bio Resource Center, Japan. iPS cells were cultured in iPS cell culture medium (ESGRO complete PLUS clonal grade medium, Millipore, Billerica, MA) without feeder cells. The cells were passaged every 3–4 days.
Injection of iPS cells and embryos culture
Blastocysts with an obvious blastocoel were collected on Day 6 and were used for iPS cells injections. The injection was performed in a mixture medium (20 µL drop) comprising of iPS cell culture medium and PBM (1:1, v/v) covered by mineral oil. After the blastocysts were immobilized with a holding pipette, the inner cell mass (ICM) was placed at the 6, 9 or 12 o’clock position (A) and the injection pipette was inserted into the blastocoel through the zona pellucida at the 3 o’clock position. The injection pipette containing about 10 iPS cells was put in the blastocoel of blastocysts. After injection of iPS cells (B), the blastocysts were cultured in the mixture medium containing 5% FBS for another 24 h in 4-well dishes. After 24 h blastocysts that regained their original shape with a fully re-expanded blastocoel and those that had hatched from zona pellucida were classified as ‘re-expanded blastocyst’ (C) and ‘hatched blastocyst’ (D), respectively. At the end of the culture period, the GFP signal was observed using an inverted fluorescence microscope (ECLIPSE Ti-U, Nikon, Tokyo, Japan).
Figure 1. Representative images of porcine blastocysts following injection of mouse induced pluripotent stem (iPS) cells: (A) blastocyst before cell injection; (B) shrunk blastocyst immediately after cell injection; (C) re-expanded blastocyst 24 h after culture; (D) hatched blastocyst 24 h after culture. Green colour represents injected iPS cells. Scale bar indicates 50 μm.
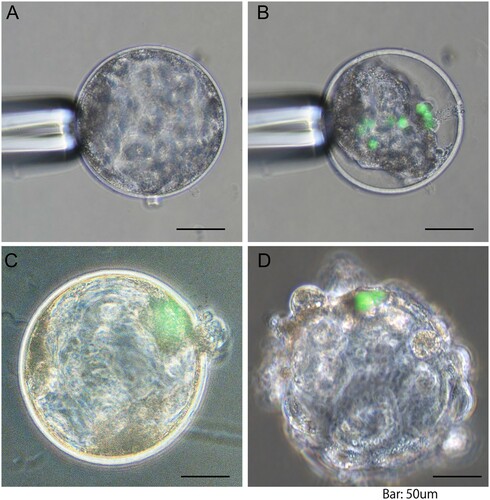
Experimental design
In the first experiment, we evaluated the effects of CGA and ITS supplementation during IVM culture (44 h) on the in vitro maturation (146–152 oocytes per treatment), fertilization (139–152 oocytes per treatment), and development (199–230 oocytes per treatment) of porcine oocytes. COCs were cultured in maturation medium supplemented with 50 µM CGA (C3878, Sigma-Aldrich) dissolved in dimethyl sulphoxide or ITS (1.0 mg/mL insulin, 0.55 mg/mL transferrin, and 0.5 µg/mL selenium, I3146, Sigma-Aldrich), either alone or in combination. As a control, COCs were cultured in maturation medium containing 50 μM β-mercaptoethanol (Wako Pure Chemical Industries Ltd.) instead of ITS and CGA. All of the experiments were repeated four to five times.
In the second experiment, we evaluated the effects of ITS and CGA supplementation during IVM culture on the development of blastocysts (54–64 embryos per treatment) following injection of mouse iPS cells. Mouse iPS cells were injected into the blastocoel of blastocysts collected on Day 6, and their development was examined as described in ‘injection of iPS cells and embryos culture’ section. Blastocysts from COCs matured in the maturation medium containing β-mercaptoethanol instead of CGA and ITS served as the control group. All of the experiments were repeated four times.
Statistical analysis
Experiments were repeated four to five times. Statistical significance was inferred from analysis of variance (ANOVA) tests followed by Fisher’s protected least significant difference (PLSD) tests using STATVIEW (Abacus Concepts, Inc., Berkeley, CA, USA). Percentage data were subjected to arcsin transformation prior to statistical analysis. Differences with a probability value (P) of 0.05 or less were considered statistically significant.
Results
The effects of CGA and ITS supplementation on in vitro maturation, fertilization, and development of porcine oocytes during IVM culture were examined. The supplementation of ITS and CGA either alone or in combination into the maturation medium had no beneficial effects on the rates of maturation and DNA fragmentation of oocytes compared to the control group (A and B; 88.5%–92.1% and 1.3%–3.1%, respectively). Moreover, the rates of total fertilization and monospermic fertilization of oocytes did not differ among the supplementation groups (C and D; 62.2%–69.8% and 91.2%–94.0%, respectively). A significantly higher total cell number in blastocysts was found in the embryos derived from oocytes matured with the combination of CGA and ITS when compared to control and ITS groups (F; 51.5 vs 42.0 and 44.2, P < 0.05). However, no significant differences in the rates of blastocyst formation were observed among the groups (E; 24.2%–29.1%).
Figure 2. Effects of chlorogenic acid (CGA) and ITS supplementation during in vitro maturation on the proportions of oocytes reaching metaphase II (A) and oocytes with DNA fragmented nuclei (B), total fertilization (C), monospermic fertilization (D), blastocyst formation (E) and the total cell number of blastocysts (F). Oocytes matured in the maturation medium containing β-mercaptoethanol but not CGA and ITS served as the control group. All oocytes matured with or without CGA and ITS were used to estimate the proportions of oocytes reaching metaphase II (MII) and having nuclei with fragmented DNA (146–152 oocytes per treatment), and of fertilization (139–152 oocytes per treatment) and embryo development (199–230 oocytes per treatment). All the experiments were repeated four to five times. Each bar represents the mean value ± SEM. Bars with different letters differ significantly (P < 0.05).
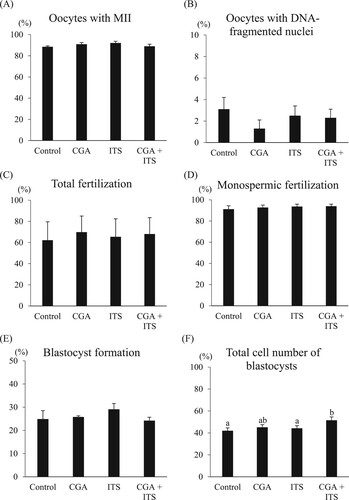
When the effects of CGA and ITS supplementation during in vitro maturation on the development of embryos following injection of mouse iPS cells into blastocysts were examined, there was no significant difference in the rates of re-expanded blastocysts among the treatment groups (). However, the rates of hatched blastocysts derived from oocytes matured with CGA alone and in combination with ITS were significantly higher than those of the other groups.
Table 1. Effects of CGA and ITS supplementation during in vitro maturation on the development of embryos following injection of mouse iPS cells into blastocysts*.
Discussion
There have been many improvements in the in vitro culture system to enable production of high-quality oocytes and embryos. However, the ability of embryos to develop further in vitro remains insufficient when compared to the in vivo system (Kikuchi et al. Citation2008). In the most porcine IVP systems, β-mercaptoethanol is widely used as an antioxidant in porcine IVM cultures to generate high-quality embryos (Funahashi Citation2005; Choe et al. Citation2010). Therefore, the control group used in the present study contained β-mercaptoethanol instead of CGA and ITS in the maturation medium. Our results show that the combined supplementation of CGA and ITS during IVM culture resulted in a higher total cell number in blastocysts compared with β-mercaptoethanol supplementation. These results are supported by our previous finding that CGA supplementation at a concentration of 50 µM during the IVM process is beneficial for porcine IVP system owing to CGA protecting the oocytes from DNA damage caused by oxidative stress (Nguyen et al. Citation2017). The present study also reveals that ITS supplementation in maturation medium did not improve the rates of blastocyst formation and total cell number in blastocysts. Our results are in agreement with those of Shamsuddin et al. (Citation1994) who supplemented ITS in the maturation medium of bovine oocytes and observed that the percentage of cleavage and blastocyst formation was not different from that of the control group. However, interestingly, this study suggests that there is a synergistic effect on the total cell number in blastocysts when a combination of CGA and ITS was used. This synergistic effect may in part be due to enhanced antioxidant defenses by selenium in ITS that has antioxidant activity (Michiels et al. Citation1994). In a previous study, ITS supplementation during IVM has been shown to improve the developmental competence of porcine oocytes (Jeong et al. Citation2008), which is not consistent with the findings of the present study. Moreover, we found that the combined supplementation of CGA and ITS during IVM culture did not improve the oocyte maturation, oocyte quality, fertilization, and blastocyst formation compared with β-mercaptoethanol supplementation. These results indicate that no apparent effects of the combination of CGA and ITS were observed because all treatment groups contained antioxidants such as CGA, ITS, and β-mercaptoethanol. Therefore, further studies will be necessary to clarify the effect of the combination of CGA and ITS by use of IVM medium without any antioxidant supplementation as control group.
To the best of our knowledge, this is the first report showing the beneficial effects of supplementation of CGA in combination with ITS on the hatched rate of interspecies chimera blastocysts. Following mouse iPS cell injection, the blastocysts shrunk instantly due to the creation of holes in zona pellucida, resulting in the loss of blastocoel fluid and decrease in blastocyst developmental competence. The blastocoel fluid plays a crucial role in direct to support cellular processing and blastocyst development (Pedersen and Spindle Citation1980; Jensen et al. Citation2014). However, the interspecies chimera blastocysts could further re-expand, develop, and completely hatch after 24 h of culture. The rate of embryos hatched from zona pellucida has been reported as an indicator of adequacy of culture conditions (Fléchon and Renard Citation1978). In the present study, our results showed that the rate of hatched blastocysts derived from oocytes treated with CGA either alone or in combination with ITS was higher than that in the other groups. This suggests that the combination of CGA and ITS may have a positive effect on the in vitro development of interspecies chimera blastocysts because of the improvement in blastocyst quality. Moreover, considering the improvement of embryonic development by the combination of CGA and ITS, further investigations are needed to clarify whether the supplementation of CGA in combination with ITS during the culture of interspecies chimera blastocysts may increase the rate of hatched blastocysts.
In conclusion, although the CGA and ITS supplementation of porcine oocyte maturation medium did not positively affect the rates of oocyte maturation and fertilization, the total cell number in blastocysts was improved by the combined supplementation of CGA and ITS. Moreover, the development of blastocysts after mouse iPS cells injection also improved when CGA was used either alone or in combination with ITS. Thus, we conclude that the combination of CGA and ITS supplementation in the maturation medium could be beneficial to the porcine IVP system for producing interspecies chimera blastocysts.
Acknowledgements
The authors would like to thank Nippon Food Packer, K. K. Shikoku (Tokushima, Japan) for supplying pig ovaries. This work was supported in part by the Program of Open Innovation Platform with Enterprises, Research Institute and Academia (OPERA) grant number JPMJOP1613 from the Japan Science and Technology Agency (JST), and KAKENHI grant number JP18K12062 and JP19K16014 from the Japan Society for the Promotion of Science (JSPS). We acknowledge Tokushima University for their financial support of the Research Clusters program of Tokushima University.
Disclosure statement
No potential conflict of interest was reported by the author(s).
References
- Agarwal A, Gupta S, Sikka S. 2006. The role of free radicals and antioxidants in reproduction. Curr Opin Obstet Gynecol. 18:325–332.
- Agarwal A, Saleh RA, Bedaiwy MA. 2003. Role of reactive oxygen species in the pathophysiology of human reproduction. Fertil Steril. 79:829–843.
- Aoi T, Yae K, Nakagawa M, Ichisaka T, Okita K, Takahashi K, Chiba T, Yamanaka S. 2008. Generation of pluripotent stem cells from adult mouse liver and stomach cells. Science. 321:699–702.
- Castro A, Oda F, Almeida-Cincotto M, Davanço M, Chiari-Andréo B, Cicarelli R, Peccinini R, Zocolo G, Ribeiro P, Corrêa M. 2018. Green coffee seed residue: A sustainable source of antioxidant compounds. Food Chem. 246:48–57.
- Choe C, Shin YW, Kim EJ, Cho SR, Kim HJ, Choi SH, Han MH, Han J, Son DS, Kang D. 2010. Synergistic effects of glutathione and beta-mercaptoethanol treatment during in vitro maturation of porcine oocytes on early embryonic development in a culture system supplemented with L-cysteine. J Reprod Dev. 56:575–582.
- De La Fuente R, O'Brien MJ, Eppig JJ. 1999. Epidermal growth factor enhances preimplantation developmental competence of maturing mouse oocytes. Hum Reprod. 14:3060–3068.
- Do LT, Luu VV, Morita Y, Taniguchi M, Nii M, Peter AT, Otoi T. 2015. Astaxanthin present in the maturation medium reduces negative effects of heat shock on the developmental competence of porcine oocytes. Reprod Biol. 15:86–93.
- Fléchon J-E, Renard J-P. 1978. A scanning electron microscope study of the hatching of bovine blastocysts in vitro. Reproduction. 53:9-NP.
- Funahashi H. 2005. Effect of beta-mercaptoethanol during in vitro fertilization procedures on sperm penetration into porcine oocytes and the early development in vitro. Reproduction. 130:889–898.
- Gonthier M-P, Remesy C, Scalbert A, Cheynier V, Souquet J-M, Poutanen K, Aura A-M. 2006. Microbial metabolism of caffeic acid and its esters chlorogenic and caftaric acids by human faecal microbiota in vitro. Biomed Pharmacother. 60:536–540.
- Jensen PL, Grøndahl ML, Beck HC, Petersen J, Stroebech L, Christensen ST, Yding Andersen C. 2014. Proteomic analysis of bovine blastocoel fluid and blastocyst cells. Syst Biol Reprod Med. 60:127–135.
- Jeong YW, Hossein MS, Bhandari DP, Kim YW, Kim JH, Park SW, Lee E, Park SM, Jeong YI, Lee JY. 2008. Effects of insulin–transferrin–selenium in defined and porcine follicular fluid supplemented IVM media on porcine IVF and SCNT embryo production. Anim Reprod Sci. 106:13–24.
- Kikuchi K, Kashiwazaki N, Nagai T, Nakai M, Somfai T, Noguchi J, Kaneko H. 2008. Selected aspects of advanced porcine reproductive technology. Reprod Domest Anim. 43(Suppl 2):401–406.
- Kikuchi K, Kashiwazaki N, Noguchi J, Shimada A, Takahashi R, Hirabayashi M, Shino M, Ueda M, Kaneko H. 1999. Developmental competence, after transfer to recipients, of porcine oocytes matured, fertilized, and cultured in vitro. Bio Reprod. 60:336–340.
- Kim S, Lee GS, Lee SH, Kim HS, Jeong YW, Kim JH, Kang SK, Lee BC, Hwang WS. 2005. Embryotropic effect of insulin-like growth factor (IGF)-I and its receptor on development of porcine preimplantation embryos produced by in vitro fertilization and somatic cell nuclear transfer. Mol Reprod Dev. 72:88–97.
- Masaki H, Kato-Itoh M, Takahashi Y, Umino A, Sato H, Ito K, Yanagida A, Nishimura T, Yamaguchi T, Hirabayashi M. 2016. Inhibition of apoptosis overcomes stage-related compatibility barriers to chimera formation in mouse embryos. Cell Stem Cell. 19:587–592.
- Michiels C, Raes M, Toussaint O, Remacle J. 1994. Importance of Se-glutathione peroxidase, catalase, and Cu/Zn-SOD for cell survival against oxidative stress. Free Radic Biol Med. 17:235–248.
- Mondal S, Mor A, Reddy IJ, Nandi S, Parameswaragupta P. 2015. Effect of fibroblast growth factor 2 (FGF2) and insulin transferrin selenium (ITS) on in vitro maturation, fertilization and embryo development in sheep. Braz Arch Biol Technol. 58:521–525.
- Namula Z, Sato Y, Kodama R, Morinaga K, Luu VV, Taniguchi M, Nakai M, Tanihara F, Kikuchi K, Nagai T, Otoi T. 2013. Motility and fertility of boar semen after liquid preservation at 5 degrees C for more than 2 weeks. Anim Sci J. 84:600–606.
- Natsuyama S, Noda Y, Narimoto K, Mori T. 1993. Role of protein supplements in the culture of mouse embryos. Theriogenology. 40:149–157.
- Nguyen TV, Tanihara F, Do L, Sato Y, Taniguchi M, Takagi M, Van Nguyen T, Otoi T. 2017. Chlorogenic acid supplementation during in vitro maturation improves maturation, fertilization and developmental competence of porcine oocytes. Reprod Dom Anim. 52:969–975.
- Nguyen TV, Wittayarat M, Do LTK, Nguyen TV, Nii M, Namula Z, Kunihara T, Tanihara F, Hirata M, Otoi T. 2018. Effects of chlorogenic acid (CGA) supplementation during in vitro maturation culture on the development and quality of porcine embryos with electroporation treatment after in vitro fertilization. Anim Sci J. 89:1207–1213.
- Pedersen RA, Spindle AI. 1980. Role of the blastocoele microenvironment in early mouse embryo differentiation. Nature. 284:550–552.
- Shamsuddin M, Larsson B, Gustafsson H, Rodriguez-Martinez H. 1994. A serum-free, cell-free culture system for development of bovine one-cell embryos up to blastocyst stage with improved viability. Theriogenology. 41:1033–1043.
- Suchy F, Nakauchi H. 2018. Interspecies chimeras. Curr Opin Genet Dev. 52:36–41.
- Wu J, Platero-Luengo A, Sakurai M, Sugawara A, Gil MA, Yamauchi T, Suzuki K, Bogliotti YS, Cuello C, Valencia MM. 2017. Interspecies chimerism with mammalian pluripotent stem cells. Cell. 168:473–486. e415.