ABSTRACT
In this study, Tembusu virus (TMUV) 1080905 isolate was isolated from 29-week-old breeder Pekin ducks in Taiwan in 2019. Clinical history showed that the clinical features were a temporary decrease in egg production, decline of feed uptake, watery diarrhea, nervous signs and sporadic mortality. Necropsy findings were congestion, hyperemia and hemorrhage of the ovary follicles, swollen spleen and deposits of fibrinous exudate on the pericardium and the liver capsule. The polyprotein gene of TMUV 1080905 was 10,278 nucleotides long, encoded 3425 amino acids and exhibited 99.3% nucleotide similarity and 99.7% amino acid similarity with Taiwanese mosquito-derived isolate TMUV TP1906. Our phylogenetic analysis revealed that the Taiwanese TMUVs were grouped together with Malaysian TMUV (chicken-derived Sitiawan virus and the TMUV prototype strain MM1775). To our knowledge, this is the first report of duck-derived TMUV in Taiwan. Further studies of the pathogenicity and host range of the TMUV 1080905 are required.
Research Highlights
First identification of Tembusu virus in Pekin ducks in Taiwan.
Investigation of the phylogeny of TMUV 1080905 isolate from Pekin ducks in Taiwan.
Introduction
Tembusu virus (TMUV) is a member of the Ntaya group, which belongs to the genus Flavivirus of the family Flaviviridae. TMUV was first identified from Culex tritaeniorhynchus mosquitoes in Kuala Lumpur, Malaysia, and designated as TMUV MM1775 in 1955 (Platt et al. Citation1975), and TMUV isolates have since been occasionally reported in Malaysia and Thailand (Pandey et al. Citation1999). In 2000, a chicken-origin TMUV strain named Sitiawan virus (STWV) was isolated in Malaysia and it could cause encephalitis and retarded growth in broiler chicks (Kono et al. Citation2000). Since 2010, an epidemic of severe egg drop syndrome caused by a variant TMUV, duck TMUV, has been reported on either egg-laying farms or breeder duck farms in China (Yan et al. Citation2011; Su et al., Citation2011). The disease caused by duck TMUV has spread to duck farms in Malaysia and Thailand (Homonnay et al. Citation2014; Thontiravong et al. Citation2015; Ninvilai et al. Citation2018). Moreover, a novel TMUV, strain TP1906, was identified from Culex annulus mosquitoes in 2019 in Taiwan (Peng et al. Citation2020).
In addition to the severe egg drop syndrome, duck TMUV causes decreased appetite, depression, retarded growth, diarrhea and neurological dysfunction. It has a morbidity rate of 90%–100% and a mortality rate of 10%–30% (Yan et al. Citation2011; Su et al., Citation2011; Homonnay et al. Citation2014; Thontiravong et al. Citation2015). Previous reports demonstrate that duck TMUV also causes similar clinical symptoms in chickens and geese and that the virus has also been isolated from mosquitoes, pigeons and sparrows (Liu et al. Citation2012a, Citation2012b; Tang et al. Citation2013; Yu et al. Citation2018).
Like other flaviviruses, the TMUV has a genome of approximately 11 kb composed of single-stranded, positive-sense RNA encoding three structure proteins (capsid protein (C), pre-membrane protein (prM) and envelope protein (E)), as well as seven nonstructural proteins (NS1, NS2A, NS2B, NS3, NS4A, 2k-NS4B and NS5) (Liu et al. Citation2012b). The structural proteins are involved in the formation of the viral particle, and attachment to and entry into the host cells, whereas the nonstructural proteins participate in viral replication, assembly, proteolysis, maturation, and host immunity regulation (Roby et al. Citation2015).
Phylogenetically, Ninvilai et al. (Citation2019) divided TMUVs into two lineages, duck TMUV lineage and TMUV lineage, and they further divided the duck TMUV lineage into three distinct clusters: Cluster 1, Cluster 2 (composed of subclusters 2.1 and 2.2) and Cluster 3. TMUV MM1775, STWV and TMUV-TP1906 are grouped into the TMUV lineage. In the duck TMUV lineage, Cluster 1 consists of the duck TMUV strains from Malaysia and Thailand, and cluster 2 contains the duck TMUV strains from Thailand and China (Peng et al. Citation2020).
In 2019, concurrently with the discovery of mosquito-derived TP1906, a TMUV was identified from a diseased Pekin duck flock in Taiwan. In the present study, we describe the clinical manifestation of the infected Pekin ducks in the flock and characteristics of the virus strain. To the best of our knowledge, this is the first report of duck-derived TMUV in Taiwan.
Materials and methods
Background of the case
Due to declining egg production and sporadic deaths, three carcasses of 29-week-old breeder Pekin ducks were submitted to the Animal Health Research Institute, Taiwan, for necropsy on September 4, 2019. The duck farm was located in Yunlin County in the central-western Taiwan and was raising 650 breeder Pekin ducks (29 -weeks old), 120 breeder Muscovy ducks (29 -weeks old) and 350 broiler Muscovy ducks (17 -weeks old). The breeder ducks were reared in cages and the broiler ducks on the floor. In addition, the flocks of different breeds were housed in separate buildings.
Gross lesions of the necropsied ducks were recorded. Tissue samples of the heart, liver, spleen, lung, kidney and trachea from each submitted duck were used for detection of viral nucleic acids and virus isolation.
Detection of viral nucleic acids
The tissues from the carcasses were homogenized individually and then centrifugated for ten minutes. After the centrifugation, the supernatant of each homogenized tissue was subjected to nucleic acid extraction. Nucleic acid was extracted from the supernatant using the MagNA Pure Compact Nucleic Acid Isolation Kit I (Roche Diagnostics, Mannheim, Germany). For initial detection of the TMUV, a reverse transcription polymerase chain reaction (RT–PCR) with primers T2F/T2R described by Huang et al. (Citation2018) was used to amplify a 716-bp DNA fragment of the E gene of the TMUV. To obtain more precise results, two primers (BYD2113F, 5′-TGGAGAAACTGGCAAGAAGT-3′; BYD2543R, 5′- TCCCTCTTTCCAAGGTATGG-3′) designed on the basis of Taiwanese TMUV sequences were used for RT–PCR to amplify a 431-bp DNA fragment of the NS5 gene. The 30-μL reaction mixture of the later RT–PCR consisted of 7.76 μL of sterile dH2O, 15 μL of Quick Taq HS DyeMix (Toyobo Co., Ltd., Osaka, Japan), 0.3 μM each of forward and reverse primer, 1.2 U AMV reverse transcriptase (Promega Corp., Madison, Wisconsin, USA), 1.2 U RNasin ribonuclease inhibitor (Promega Corp.), and 5 μL of the extracted RNA. The amplification programme consisted of an initial step of 42°C for 30 min and 94°C for 4 min, followed by 35 cycles of 94°C for 50 sec, 58°C for 40 sec and 72°C for 50 sec, and a final elongation step at 72°C for 7 min. To determine the nucleotide sequences of the PCR products with expected size, 3700XL DNA analyzer was used (Applied Biosystems, Life Technologies, Carlsbad, California, USA) by a commercial sequencing service (Mission Biotech, Taipei, Taiwan).
In addition, to identify possible infections with other viral pathogens, PCRs, RT-PCRs, and real-time RT-PCRs were performed for detecting avian influenza virus (AIV) (Spackman et al. Citation2002), goose parvovirus (GPV) (Chang et al. Citation2000), anatid herpesvirus 1 (AnHV-1) (Hansen et al. Citation2000), avian orthoreovirus (ARV) (Zhang et al. Citation2006), Newcastle disease virus (NDV) (Wise et al. Citation2004) and waterfowl circovirus (WCV) (Chen et al. Citation2006).
Virus isolation and electron microscopy
Tissue samples of kidney collected from the carcasses were homogenized individually in phosphate-buffered saline and clarified by centrifugation at 3000 rpm for 10 min, and the obtained supernatant was then filtered through a 0.45 μm membrane filter. The aliquots (200 μL) of the filtered tissue homogenate were inoculated into the allantoic cavity of two 9-day-old embryonated TMUV-free Muscovy duck eggs. The eggs were then incubated at 37°C and observed twice daily for six days. Embryos that died within the first 24 hours of inoculation were discarded. The embryos that died after 24 hours or survived till six days after inoculation were chilled at 4–8°C overnight and their allantoic fluid was harvested.
Presence of TMUV in the allantoic fluid was examined by the aforementioned RT–PCR and by negative staining electron microscopy. For electron microscopy, the allantoic fluid was centrifuged at 3000 rpm for 10 minutes. The supernatant was collected for ultracentrifugation by Airfuge Air-Driven ultracentrifuge (Beckman Coulter Inc., Brea, California, USA) at 90,000 rpm for 10 min. The sediment was resuspended in ddH2O and mixed with an equal volume of sodium phosphotungstate (2%). A drop of the mixture was placed on a glow-discharged carbon-coated copper grid, and surplus fluid was removed with filter papers. The grid was air-dried and observed with a JEOL JEM-1400 transmission electron microscope (JEOL Ltd, Tokyo, Japan) operated at 60 or 80 kV.
Nucleotide sequencing for full-length viral genome
Sequencing of the complete polyprotein gene of the Pekin duck derived TMUV was attempted using primers () designed according to the sequences of the TMUV STWV and MM1775 (GenBank accession numbers JX477686 and MH414569, respectively). The nucleic acids extracted from TMUV isolated from the kidney tissue were used as the RNA source for these RT-PCRs. Reaction mixtures for each amplification were prepared as aforementioned. The amplification programme consisted of an initial step of 42°C for 30 min and 94°C for 4 min, followed by 35 cycles of 94°C for 50 sec, 50°C for 50 sec and 72°C for 90 sec, and a final elongation step at 72°C for 7 min. RT–PCR products were purified using a Qiagen PCR purification kit (Qiagen, Hilden, Germany) and cloned into the pCR2.1-TOPO vector (Invitrogen, Carlsbad, California, USA) according to the manufacturer’s instructions. The cloned inserts were sequenced as described previously. Each nucleotide position was sequenced at least three times.
Table 1. Primers used for nucleotide sequencing of full-length Tembusu virus polyprotein gene.
Phylogenetic analysis
The nucleotide sequences obtained by the RT-PCRs were assembled in the SeqMan programme within the bioinformatic analysis software DNASTAR (Lasergene Inc., Madison, Wisconsin, USA). The whole nucleotide sequences of the polyprotein gene of the TMUVs were aligned by multiple alignment using fast Fourier transform, or MAFFT, programme version 7 (Katoh and Standley Citation2013) web service (Kuraku et al. Citation2013). Phylogenetic relationships among the viral isolates were analyzed by the maximum likelihood method using the Tamura-Nei model (Tamura and Nei Citation1993) in Molecular Evolutionary Genetics Analysis version X (MEGA X; Kumar et al. Citation2018). The reliability of the analysis was evaluated by a bootstrap test with 1000 replications. The sequence of Ntaya virus (GenBank accession number JX236040) was used as the outgroup. In addition, the similarity of the polyprotein gene and its encoded protein of TMUV isolates was calculated using the MegAlign programme in the bioinformatic analysis software DNASTAR.
Results
Clinical observation and gross findings
The breeder Pekin ducks of the reported farm showed an obvious decrease in egg production (15% within 12 days) from 22 August 2019, () and sporadic mortality (0–3 ducks per day). Other clinical signs included decline of feed uptake, watery diarrhea and nervous signs. The lowest egg production rate was recorded on the 12th day after the onset of disease, and then production gradually returned to normal (). The ducks which were not able to lay eggs were eliminated by the owner. As of the 22nd day after the onset of disease, Pekin ducks no longer died. The final mortality of the breeder Pekin ducks was 3%, and 5% of the ducks were unable to drop eggs at all. The breeder Muscovy ducks and the broiler Muscovy ducks in the same farm showed no clinical symptoms.
Figure 1. Egg production rate (line) and the number of daily deaths (vertical bars) of the breeder Pekin ducks in the farm infected with Tembusu virus. A decrease in egg production was first observed on August 22, 2019. Carcasses of diseased ducks were submitted for disease investigation on September 4. Without intensive intervention, the drop in egg production and mortality recovered gradually after mid-September.
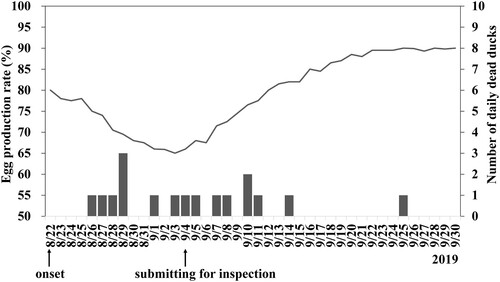
All of the submitted Pekin ducks showed congestion, hyperemia and hemorrhage of the ovary follicles. In addition, swollen spleen and deposits of fibrinous exudate on the pericardium and the liver capsule possibly due to egg peritonitis were also observed. No obvious lesions were observed in other visceral organs.
Detection of viral nucleic acids
TMUV RNA was present in all the sampled organs of one of the three Pekin ducks, as detected by the RT–PCR targeting E gene and NS5 gene. The PCRs, RT-PCRs and real-time RT-PCRs for the detection ofAIV, GPV, AnHV-1, ARV, NDV and WCV gave negative results.
Virus isolation and electron microscopy
To isolate the causative agent, the homogenate of the kidney tissue was inoculated into the allantoic cavity of 9-day-old embryonated Muscovy duck eggs. The embryos of inoculated eggs died 4–6 days post-inoculation, and TMUV RNA was detected in their allantoic fluid by the RT–PCR. The isolate was designated as TMUV 1080905.
Observed by negative staining electron microscopy, spherical and enveloped particles with diameters of 45–50 nm appeared in the allantoic fluid of the second passage of the embryonated eggs ().
Sequence comparison and phylogenetic analysis
The polyprotein gene sequence of TMUV 1080905 was submitted to the GenBank database under the accession number MW922032. The length of full-length polyprotein gene was 10,278 nucleotides, encoding 3425 amino acids, and was composed of capsid gene (C; 360 bp), pre-membrane protein gene (prM; 501 bp), envelope gene (E; 1,503 bp), nonstructural protein 1 gene (NS1; 1056 bp), NS2A gene (681 bp), NS2B gene (393 bp), NS3 gene (1857 bp), NS4A gene (378 bp), 2K gene (69 bp), NS4B gene (762 bp) and NS5 gene (2718 bp). The phylogenetic analysis based on the polyprotein gene suggested that that the TMUVs were divided into four distinct clusters: Cluster 1, Cluster 2 (2.1 and 2.2), Cluster 3 and Cluster 4 (). The isolate TMUV 1080905 was grouped into Cluster 4 and was most closely related to the TMUV-TP1906 isolate with 99.3% nucleotide identity. Moreover, the similarities of polyprotein between TMUV 1080905 and the other TMUVs of Cluster 4 (TP1906, STWV and MM1775) and TMUVs of Clusters 1–3 was 91.2%–99.3% and 86.4%–88.0%, respectively, at the nucleotide level and 98.6%–99.7% and 95.6%–97.1%, respectively, at the amino acid level (). The comparison of the polyprotein sequence of the TMUV 1080905 and TP1906 isolates (Peng et al. Citation2020) is shown in . There were 68 nucleotide and 10 amino acid differences between 1080905 and TP1906. The corresponding open reading frames between 1080905 and TP1906 showed 98.5%–99.7% identity at the nucleotide level and 97.7%–100% identity at the amino acid level. Furthermore, amino acid sequences of the C, NS1, NS2A, NS3 and 2K-NS4B of the two isolates were identical.
Figure 3. Phylogenetic analysis of the Tebmusu virus (TMUV) isolate TMUV 1080905 and 47 selected reference TMUV strains and duck TMUV based on the polyprotein gene (10,287 bp). The phylogenetic tree was constructed using the maximum likelihood method based on the Tamura-Nei model (Tamura and Nei Citation1993). Only bootstrap values (after 1000 replicates) over 70% are indicated at each branch point as a percentage. For each virus strain, strain name, host, year of isolation or detection and GenBank accession number are shown.
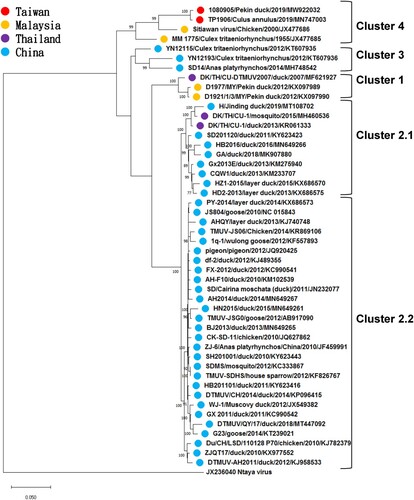
Table 2. The similarity of polyprotein gene and its encoded protein of Taiwanese Tembusu virus isolate TMUV 1080905 against other Tembusu viruses.
Table 3. Sequence comparisons of polyprotein of Tembusu virus isolates 1080905 and TP1906.
Discussion
This article is the first report of a TMUV identified in diseased breeder Pekin ducks in Taiwan. Our observations on clinical signs and gross findings are in agreement with previously reported findings of TMUV infections in China, Malaysia and Thailand (Su et al. Citation2011; Homonnay et al. Citation2014; Thontiravong et al. Citation2015; Zhang et al. Citation2017). Furthermore, virus isolation, molecular detection, electron microscopy and genetic characterization supported the association between the clinical findings and the identified virus. Therefore, the isolated TMUV from Pekin ducks for the first time in Taiwan was the causative agent of the drop in egg production and mortality.
The clinical signs and gross lesions observed in the TMUV-infected Pekin duck flock in Taiwan were similar to the TMUV infections reported previously in China, Thailand and Malaysia. Previous reports indicate that infection with TMUV in ducks is mainly characterized by various degrees of decreased egg production, ranging from 20% to 60% and occasionally as high as 90%, and infected ducks may show clinical signs including decline in feed uptake, diarrhea, ataxia, lameness and paralysis (Cao et al. Citation2011; Su et al. Citation2011; Liu et al. Citation2013; Homonnay et al. Citation2014; Thontiravong et al. Citation2015; Zhang et al. Citation2017; Ninvilai et al. Citation2018). The mortality of the affected flocks ranges from 10% to 30% depending on the management conditions and secondary bacterial infection of the infected flocks (Su et al. Citation2011; Thontiravong et al. Citation2015; Zhang et al. Citation2017; Ninvilai et al. Citation2018). The gross lesions of infected ducks include severe ovarian haemorrhage, ovaritis and regression, and splenic enlargement is occasionally observed (Su et al. Citation2011; Thontiravong et al. Citation2015; Zhang et al. Citation2017; Ninvilai et al. Citation2018). In the present study, the clinical signs of the affected Pekin duck flock, as reported by the owner, were a temporary drop in egg production (15% within 12 days), decline of feed uptake, watery diarrhea and nervous signs clinically. Ovarian congestion, hyperemia and hemorrhage were the most noticeable gross findings among the affected Pekin ducks. Accordingly, with the supporting virological evidence, we believe that TMUV was the etiological agent of the case. Further animal experiments may be required to clarify whether the differences in the degrees of decreasing egg production and mortality can be ascribed to the pathogenicity of the virus strain or the management methods of the flock owner in this study (cage feeding of the breeder ducks and elimination strategy).
Phylogenetic analysis of the present study revealed that the phylogeny of the TMUVs was host-independent. Earlier studies showed that the clustering of TMUVs in their phylogenetic trees was consistent with geographic distribution of the viruses (Lei et al. Citation2017; Ninvilai et al. Citation2018; Yu et al. Citation2018) and the analyzed TMUVs were divided, on the basis of the hosts which the isolates came from, into two lineages: TMUV and duck TMUV (Ninvilai et al. Citation2019). Nevertheless, following the increasing number of reported TMUV genomic sequences, the genetic closeness was no longer paralleled by the hosts from which TMUVs were isolated. Also, Lei et al. (Citation2017) suggest a freedom of species barrier among the TMUVs. Our analysis further supported this idea by demonstrating that the hosts of the duck TMUV lineage were various and not limited to ducks and that no specific cluster indicating a specific host was found (). Furthermore, according to the updated taxonomy released by the International Committee on Taxonomy of Viruses, various TMUV strains, including STWV (Kono et al. Citation2000), BYD virus (Su et al. Citation2011), Perak virus (Homonnay et al. Citation2014), duck egg drop syndrome virus (Su et al. Citation2011; Liu et al. Citation2012b) and duck TMUV (Cao et al. Citation2011; Yan et al. Citation2011; Su et al. Citation2011) belong to the single species Tembusu virus. Conclusively, current findings have evidenced that TMUV is a single species of a variety of animal hosts, ranging from avian species to mosquitos.
Yan et al. (Citation2018) report that the amino acid residue 156-Ser in the E protein is responsible for TMUV tropism and transmission in ducks. Sun et al. (Citation2020) report that the Thr-to-Lys mutation of residue 367 in the E protein plays a predominant role in viral cell adaption and virulence attenuation in ducks. Both specific residues were present in the isolate TMUV 1080905, and this isolate demonstrated a relatively lower pathogenicity, as showed a temporary decreasing egg production and sporadic deaths in this outbreak. Whether and how these residues affect the TMUV’s virulence deserves further investigation.
Taiwanese isolates were genetically close to southeastern Asian isolates. The isolate discovered in the present study, TMUV 1080905, and the isolate TMUV TP1906 were first identified from ducks and mosquitoes, respectively, by different laboratories in Taiwan in the autumn of 2019 (Peng et al. Citation2020). The two Taiwanese TMUVs were almost identical to each other (99.3% nucleotide identity; ). Moreover, both of them were most similar to the MM1775 and STWV isolated from Malaysia, rather than the TMUVs from mainland China, and formed a new branch: Cluster 4 (). Lei et al. (Citation2017) has divided TMUVs into the Chinese mainland TMUV lineage and Southeast Asian TMUV lineage. Our phylogenetic analysis showed that the Taiwanese TMUVs belonged to the Southeast Asian TMUV lineage, implying that the TMUV in Taiwan may have been introduced not directly from China but from distant southeastern Asian countries. A previous study by Lei et al. hypothesized that the TMUV originated in Malaysia, where this virus was first identified, spread northwards to Thailand, later moved gradually northeastward to Shandong Province in eastern China, and then throughout the Chinese mainland (Lei et al. Citation2017). If the introduction of TMUV to Taiwan was not the case, then carrying and spreading of the virus by migratory birds through the East Asian–Australasian flyway, as in the case of the spread of AIV and Japanese encephalitis virus (Cheng et al. Citation2010; Gao et al. Citation2013), may be possible (Peng et al. Citation2020). On the other hand, due to the uncertain mode of transmission, human population movements and regional trade may be another explanation.
In summary, this study reports for the first time the identification and phylogenetic description of the TMUV 1080905 from Pekin ducks in Taiwan. This virus showed a high nucleotide and amino acid homology with the Taiwanese mosquito-derived TMUV TP1906, and the isolate was also grouped together with Malaysia TMUV, MM1775 and STWV. Further studies of the pathogenicity and host range of the 1080905 are required.
Ethical statement
This study does not involve animal experiments.
Acknowledgments
The authors thank Dr. Chieh-Hao Wu from the Animal Health Research Institute, Taiwan for her assistance in electron microscopy.
Disclosure statement
No potential conflict of interest was reported by the author(s).
References
- Cao ZZ, Zhang C, Liu YH, Ye WC, Han JW, Ma GM, Zhang DD, Xu F, Gao XH, Tang Y, et al. 2011. Emergence of Tembusu virus in ducks, China. Emerg Infect Dis. 17:1873–1875.
- Chang PC, Shien JH, Wang MS, Shieh HK. 2000. Phylogenetic analysis of parvoviruses isolated in Taiwan from ducks and geese. Avian Pathol. 29:45–49.
- Chen CL, Wang PX, Lee MS, Shien JH, Shien HK, Ou SJ, Chen CH, Chang PC. 2006. Development of a polymerase chain reaction procedure for detection and differentiation of duck and goose circovirus. Avian Dis. 50:92–95.
- Cheng MC, Lee MS, Ho YH, Chyi WL, Wang CH. 2010. Avian influenza monitoring in migrating in Taiwan during 1998–2007. Avian Dis. 54:109–114.
- Gao X, Liu H, Wang H, Fu S, Guo Z, Liang G. 2013. Southernmost Asia is the source of Japanese encephalitis virus (genotype 1) diversity from which the viruses disperse and evolve throughout Asia. PLoS Negl Trop Dis. 19:e2459.
- Hansen WR, Nashold SW, Docherty DE, Brown SE, Knudson DL. 2000. Diagnosis of duck plague in waterfowl by polymerase chain reaction. Avian Dis. 44:266–274.
- Homonnay ZG, Kovács EW, Bányai K, Albert M, Fehér E, Mató T, Tatár-Kis T, Palya V. 2014. Tembusu-like flavivirus (Perak virus) as the cause of neurological disease outbreaks in young Pekin ducks. Avian Pathol. 43:552–560.
- Huang X, Qiu H, Peng X, Zhao W, Lu X, Mo K, Yan Y, Liao M, Zhou J. 2018. Molecular analysis and serological survey of Tembusu virus infection in Zhejiang, China, 2010–2016. Arch Virol. 163:3225–3234.
- Katoh K, Standley DM. 2013. MAFFT multiple sequence alignment software version 7: improvements in performance and usability. Mol Biol Evol. 30:772–780.
- Kono Y, Tsukamoto K, Abd Hamid M, Darus A, Lian TC, Sam LS, Yok CN, Di KB, Lim KT, Yamaguchi S, Narita M. 2000. Encephalitis and retarded growth of chicks caused by Sitiawan virus, a new isolate belonging to the genus Flavivirus. Am J Trop Med Hyg. 63:94–101.
- Kumar S, Stecher G, Li M, Knyaz C, Tamura K. 2018. MEGA x: molecular evolutionary genetics analysis across computing platforms. Mol Biol Evol. 35:1547–1549.
- Kuraku S, Zmasek CM, Nishimura O, Katoh K. 2013. Aleaves facilitates on-demand exploration of metazoan gene family trees on MAFFT sequence alignment server with enhanced interactivity. Nucleic Acids Res. 41:W22–W28.
- Lei W, Guo X, Fu S, Feng Y, Tao X, Gao X, Song J, Yang Z, Zhou H, Liang G. 2017. The genetic characteristics and evolution of Tembusu virus. Vet Microbiol. 201:32–41.
- Liu M, Chen S, Chen Y, Liu C, Chen S, Yin X, Li G, Zhang Y. 2012a. Adapted Tembusu-like virus in chickens and geese in China. J Clin Microbiol. 50:2807–2809.
- Liu P, Lu H, Li S, Moureau G, Deng YQ, Wang Y, Zhang L, Jiang T, de Lamballerie X, Qin CF, et al. 2012b. Genomic and antigenic characterization of the newly emerging Chinese duck egg-drop syndrome flavivirus: genomic comparison with Tembusu and Sitiawan viruses. J Gen Virol. 93:2158–2170.
- Liu P, Lu H, Li S, Wu Y, Gao GF, Su J. 2013. Duck egg drop syndrome virus: an emerging Tembusu-related flavivirus in China. Science China Life Sciences. 56:701–710.
- Ninvilai P, Nonthabenjawan N, Limcharoen B, Tunterak W, Oraveerakul K, Banlunara W, Amonsin A, Thontiravong A. 2018. The presence of duck Tembusu virus in Thailand since 2007: a retrospective study. Transbound Emerg Dis. 65:1208–1216.
- Ninvilai P, Tunterak W, Oraveerakul K, Amonsin A, Thontiravong A. 2019. Genetic characterization of duck Tembusu virus in Thailand, 2015–2017: identification of a novel cluster. Transbound Emerg Dis. 66:1982–1992.
- Pandey BD, Karabatsos N, Cropp B, Tagaki M, Tsuda Y, Ichinose A, Igarashi A. 1999. Identification of a flavivirus isolated from mosquitos in Chiang Mai Thailand. Southeast Asian J Trop Med Public Health. 30:161–165.
- Peng SH, Su CL, Chang MC, Hu HC, Yang SL, Shu PY. 2020. Genome analysis of a novel Tembusu virus in Taiwan. Viruses. 12:567.
- Platt GS, Way HJ, Bowen ET, Simpson DI, Hill MN, Kamath S, Bendell PJ, Heathcote OH. 1975. Arbovirus infections in sarawak, October 1968–February 1970 Tembusu and Sindbis virus isolations from mosquitoes. Ann Trop Med Parasitol. 69:65–71.
- Roby JA, Setoh YX, Hall RA, Khromykh AA. 2015. Post-translational regulation and modifications of flavivirus structural proteins. J Gen Virol. 96(Pt 7):1551.
- Spackman E, Senne DA, Myers TJ, Bulaga LL, Garber LP, Perdue ML, Lohman K, Daum LT, Suarez DL. 2002. Development of a real-time reverse transcriptase PCR assay for type A influenza virus and the avian H5 and H7 hemagglutinin subtypes. J Clin Microbiol. 40:3256–3260.
- Su J, Li S, Hu X, Yu X, Wang Y, Liu P, Lu X, Zhang G, Hu X, Liu D, et al. 2011. Duck egg-drop syndrome caused by BYD virus, a new Tembusu-related flavivirus. PLoS One. 6:e18106.
- Sun M, Zhang L, Cao Y, Wang J, Yu Z, Sun X, Liu F, Li Z, Liu P, Su J. 2020. Basic amino acid substitution at residue 367 of the envelope protein of Tembusu virus plays a critical role in pathogenesis. J Virol. 94:e02011–e02019.
- Tamura K, Nei M. 1993. Estimation of the number of nucleotide substitutions in the control region of mitochondrial DNA in humans and chimpanzees. Mol Biol Evol. 10:512–526.
- Tang Y, Diao Y, Yu C, Gao X, Ju X, Xue C, Liu X, Ge P, Qu J, Zhang D. 2013. Characterization of a Tembusu virus isolated from naturally infected house sparrows (Passer domesticus) in Northern China. Transbound Emerg Dis. 60:152–158.
- Thontiravong A, Ninvilai P, Tunterak W, Nonthabenjawan N, Chaiyavong S, Angkabkingkaew K, Mungkundar C, Phuengpho W, Oraveerakul K, Amonsin A. 2015. Tembusu-related flavivirus in ducks, Thailand. Emerg Infect Dis. 21:2164–2167.
- Wise MG, Suarez DL, Seal BS, Pedersen JC, Senne DA, King DJ, Kapczynski DR, Spackman E. 2004. Development of a real-time reverse–transcription PCR for detection of Newcastle disease virus RNA in clinical samples. J Clin Microbiol. 42:329–338.
- Yan D, Shi Y, Wang H, Li G, Li X, Wang B, Su X, Wang J, Teng Q, Yang J, et al. 2018. A single mutation at position 156 in the envelope protein of Tembusu virus is responsible for virus tissue tropism and transmissibility in ducks. J Virol. 92:e00427–18.
- Yan P, Zhao Y, Zhang X, Xu D, Dai X, Teng Q, Yan L, Zhou J, Ji X, Zhang S, et al. 2011. An infectious disease of ducks caused by a newly emerged Tembusu virus strain in mainland China. Virology. 417:1–8.
- Yu G, Lin Y, Tang Y, Diao Y. 2018. Evolution of Tembusu virus in ducks, chickens, geese, sparrows, and mosquitoes in northern China. Viruses. 10:485.
- Zhang W, Chen S, Mahalingam S, Wang M, Cheng A. 2017. An updated review of avian-origin Tembusu virus: a newly emerging avian Flavivirus. J Gen Virol. 98:2413–2420.
- Zhang Y, Liu M, Shuidong O, Hu QL, Guo DC, Chen HY, Han Z. 2006. Detection and identification of avian, duck, and goose reoviruses by RT-PCR: goose and duck reoviruses are part of the same genogroup in the genus Orthoreovirus. Arch Virol. 151:1525–1538.