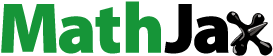
ABSTRACT
Colostrum in the milk bulk tank could affect cheese milk and the characteristics of the final product, leading to multiple problems in industrial processes. The objective of this study was to evaluate colostrum concentrations (0%, 1, 5% and 10%) in cheese milk subjected to two discontinuous heat treatments (56°C for 1 h or 63°C for 30 min) on the physicochemical characteristics, cheese-making properties, and the effectiveness of pasteurization. All physicochemical parameters were modified by colostrum , and some of them were varied due to the thermal treatment. Milk rennet coagulation characteristics were no different among the thermal treatments applied when colostrum (up to 10%) was added to the milk. Both thermal treatments had the same effectiveness in reducing total plate count in raw milk without colostrum; however, when colostrum was added, the treatment at 63°C for 30 min proved to be more efficient.
Introduction
Microorganisms can be introduced into milk at any stage in the production to consumption chain (Sharma et al. Citation2014). Recently, Alegbeleye et al. (Citation2018) listed the primary sources and routes of microorganisms in milk, such as commensal microflora, diseases, mastitis, environmental contamination, and post-pasteurization contamination. These authors also summarized the microbiological hazards associated with the consumption of raw milk, such as relatively benign symptoms to more severe complications, even long-term, and chronic complications. These risks can be extended to the consumption of non-well pasteurized milk or low-efficiency pasteurization process.
Colostrum is the mammary secretion obtained after partum, which provides nutrients and passive immunity to the newborn. Abd El-Fattah et al. (Citation2012), Sánchez-Macías et al. (Citation2014) and Tsioulpas et al. (Citation2007), working with bovine, goat, and buffalo colostrum, respectively, reported extreme differences in the physical and technological parameters between colostrum and mature milk. Colostrum is obtained in large quantities. It is considered nutraceutical and contains plenty of physiologically active components, including immunoglobulins (Igs), lactoferrin, lysozyme, cytokines, growth factors, hormones, oligosaccharides, and lipid components (Pakkanen and Aalto Citation1997; Korhonen Citation2009; Borad and Singh Citation2018). The main difference between mature milk and colostrum is the level of IgG concentration (0.72–0.90 versus 32–212 mg mL−1 of bovine immunoglobulin G, respectively) (Gapper et al. Citation2007; Godden et al. Citation2019). Moreover, colostrum presents a higher microbial count than mature milk, which could increase a load of bacteria if colostrum is added to the milk bulk tank (Godden et al. Citation2019).
To address food security concerns, many countries, through governments, regulatory authorities, and public health practitioners, require undergoing processing techniques to treat raw milk to eliminate pathogenic microorganisms. These significantly reduce spoilage microflora and inactivate enzymes, to enhance the shelf-life of dairy products. These techniques include thermization, pasteurization, sterilization, ultraviolet treatment, high pressures, microwave treatment, membrane processing, and microfiltration, among others (Sarkar Citation2015; Bucci et al. Citation2018). However, scientific evidence shows that pasteurization is the primary, incontrovertible safeguard for ensuring milk quality (Alegbeleye et al. Citation2018).
Low-temperature-long-time pasteurization or holder method is a typical batch method where milk is placed in an open vat and heated to 63°C for 30 min (Gedam et al. Citation2007). This is considered the standard minimum time-temperature combination recognized worldwide. It has less impact on milk than higher temperatures and, therefore better retains of raw milk constituents while inactivating relevant pathogens (Angulo et al. Citation2009). However, in many countries and regions raw milk is used for dairy products processing and consumers’ increased consumption of raw milk or raw dairy products is the trend (Verraes et al. Citation2015).
Heat treatment adversely affects the bioactivity of colostrum immunoglobulins. Researchers have found the onset of thermal unfolding of Igs starting at 62.6°C, depending on the pH of colostrum, and complete denaturation at 89°C (Lindström et al. Citation1994; Singh and Havea Citation2003). Moreover, it is established that Igs are vulnerable to heating above 65°C (Indyk et al. Citation2008; Borad et al. Citation2019), causing an increase in viscosity and gelation. The thermo-labile nature of colostrum Igs and other bioactive compounds is well established and depends on temperature, treatment time, pH, and compositional factors (McGrath et al. Citation2016; Borad and Singh Citation2018; Morales-delaNuez et al. Citation2020). Abd El-Fattah et al. (Citation2014) reported that commercial pasteurization time-temperature combinations (63°C for 30 min or 72°C for 15 s) significantly reduce IgG, IgM, IGF-1 and lactoferrin, and increase the viscosity of colostrum compared with 60°C for 60 min treatment. However, Steinbach et al. (Citation1981) and Trujillo et al. (Citation2007) did not observe differences in IgG concentration in colostrum after pasteurization at 65°C for 30 min or 56°C for 60 min.
There is scarce literature about the effects of colostrum on the effectiveness of milk pasteurization and cheese milk quality. In this study, we used the lowest colostrum heat treatment found in the literature (56°C for 60 min) that reduces the bacterial load but affects minimally Igs concentration (Argüello et al. Citation2003; Morales-delaNuez et al. Citation2020), and conventional milk batch low-temperature-long time pasteurization (LTLT, 63°C for 30 min). McGrath et al. (Citation2016) observed that the duration of mammary secretion classified as colostrum varies according to the literature from immediately after parturition to 5–7 days post-partum, which is considered unmarketable and often excluded from bulk milk collection for milk processing. The introduction of colostrum to the milk bulk tank is also possible due to bad farming practices, intentionally or unintentionally. Its composition and high antimicrobial compounds leadsto multiple problems and may affect the industrial and fermentation processes (Marnila and Korhonen Citation2002; McGrath et al. Citation2016). Even this could interfere with the effectiveness of the pasteurization of milk. For this reason, we proposed a study to evaluate the effects of two heat treatments applied to milk containing 0%, 1%, 5%, and 10% of colostrum on physicochemical characteristics, pasteurization effectiveness and cheese-making properties.
Material and methods
Colostrum and milk handling
Colostrum and mature milk were obtained from a Holstein dairy farm (Chimborazo Province, Ecuador) using mechanical milking. Animals had good health, including low somatic cells count, and did not receive veterinary treatment before or during the experimental period. First-milking bovine colostrum of ten multiparous Holstein cattle was procured and frozen (−26°C) to create a colostrum bank. Thawed colostrum at 4°C was pooled to create a unique 80-L batch to ensure uniformity of the colostrum sample used in experimental trials. On the other hand, fresh mature milk (20 L) from a bulk tank milk was collected from the same Holstein dairy farm (with 32 animals in their middle lactation) when needed for each batch.
For colostrum and mature milk collection, proximate composition, physicochemical characteristics, colour, total plate count and IgG quantification were estimated to ensure the quality of the samples.
Experimental design and processing of milk and colostrum
The present experiment was conducted at the laboratories of the Engineering Faculty of the Universidad Nacional de Chimborazo (Riobamba, Ecuador). To study the influence of different concentrations of colostrum in mature milk on the pasteurization effectiveness and cheese milk quality, colostrum was added to milk in different concentrations (0%, 1%, 5% and 10%) until it became 3L. Each group was subdivided into three subgroups of 1L: the first one was treated at 56°C for 60 min (LT56), the second one at 63°C for 30 min (LT63), and the third one received no thermal treatment (Raw). Thermal treatment was performed in 2L sterilized bottles in a water bath, using an extra bottle to control the internal temperature of the milk during heating. Time started when the experimental temperature was reached. When the time was reached, milk was cooled down and analysed at 20°C. Samples were taken and frozen (−80°C) for later IgG quantification. Each treatment was carried out in triplicate.
Physicochemical characteristics of colostrum and milk
Proximate composition (fat, protein, lactose, total solids, and non-fat solids) of colostrum and milk samples was estimated using a Milkoscan 6000 FT (Foss Electric, Hillerød, Denmark). Titratable acidity (percentage of lactic acid) of the samples was measured according to Sánchez-Macías et al. (Citation2014), and the ethanol stability was determined according to Tsioulpas et al. (Citation2007). The buffering capacity was evaluated by adding 100 μL of 0.5 M HCl every 30 s in a 100-mL of colostrum or milk sample, and the final buffering capacity was counted when a constant pH of 4.5 was reached (Huppertz et al. Citation2004). Milk density and pH were determined using a lactodensimeter (Alla France, Chemillé, France) and a digital pH-meter (Hach, Gel-filled, USA), respectively. A Minolta colorimeter CR-400 (Illuminant D65, Konica Minolta, Osaka, Japan) was used to determine lightness (L*), yellow index (b*), and red index (a*) for each sample. For determining total colour differences among milk samples, ΔE* was calculated as . The final values of ΔE* were compared with a range established by the Zmeškal scale (Nedomová et al. Citation2017). All these parameters were measured in triplicate.
Cheese-making properties
LT56 and LT63 milk samples with 0%, 1%, 5%, and 10% of colostrum, heat-treated or raw were coagulated with commercial recombinant chymosin (CHY-MAXs extra; EC 3.4.23.4, isozyme B, 600 IMCU/mL, Chr. Hansen Inc., Barcelona, Spain) at 0.035% (v/v) and 32°C. Milk coagulation characteristics were analysed for rennet clotting time (RCT, min), rate of curd firming (RCF, Δ%T/min), curd firmness at 30, 45 and 60 min (F30, F45 and F60, Δ%T), using a near-infrared optical device (Optigraph Ysebaert SA, Frépillon, France). Also, the potential cheese yield (CY, %) and curd draining capacity (CD, %) were assessed according to Calvo and Balcones (Citation1998) and Calvo and Espinoza (Citation1999), respectively, using the same chymosin concentration described above.
Microbiological analysis and IgG quantification
To assess the total plate count, samples were serially diluted in a saline solution (0.9% of NaCl) and then plated on 3M™ Petrifilm Aerobic Count plates (3M Microbiology, St. Paul, MN, USA) for aerobic total plate count (TPC). All plates were incubated at 32°C, and the colonies were enumerated after 48 h. The obtained results were expressed in log colony-forming units per mL of sample (log cfu mL−1). The inactivation ratios were expressed as cell survival percentage (N/N0 × 100), where N is the number of cells in the heat-treated sample and N0 is the initial number of cells in the untreated sample.
To determine colostrum and milk IgG concentrations, commercial ELISA sets (Bethyl Laboratories, Montgomery, TX, USA) were used. Purified bovine IgG was used as the standard reference. Samples were individually analysed in duplicate, and results were expressed as milligrams of IgG per millilitre.
Statistical analysis
Statistical analyses were performed using the SAS program (V.9, SAS Institute Inc., Cary, NC). The ANOVA procedure compared compositional, physicochemical properties, cheese-making properties and TPC between mature milk and colostrum samples. The ANOVA procedure for repeated measurements evaluated the effect of different concentrations of colostrum in milk and heat treatments on proximate composition, physicochemical characteristics, cheese-making properties, and bacterial inactivation. Significantly different means were identified using the Tukey test (P < 0.05).
Results and discussion
Raw colostrum and milk characteristics
summarizes the proximate composition, physicochemical parameters, total plate count, andIgG quantification of raw colostrum and mature milk used in the experiment. The results showed that the composition of colostrum differs markedly from mature milk for all analysed parameters. The values of fat, protein, non-fat solids, and total solid as well as density and lactic acid content, were higher in colostrum than in milk, while lactose content and pH were lower. Likewise, a markedly higher buffering capacity was found in colostrum, as seen by other authors (Borad et al. Citation2019). These results are according to the literature when comparing colostrum to mature milk (Marnila and Korhonen Citation2002; Madsen et al. Citation2004; Kehoe et al. Citation2007; Tsioulpas et al. Citation2007; Jeong et al. Citation2009; Sánchez-Macías et al. Citation2014).
Table 1. Physicochemical and microbiological characteristics of colostrum and milk.
The total plate count in colostrum was higher (5.46 log cfu mL−1) than in milk (3.67 log cfu mL−1). Morrill et al. (Citation2012) found that up to 60% of colostrum produced in USA dairy farms does not meet the minimum bacteriological standards for milk (TPC of <100,000 cfu mL−1). The values of TPC found in our study for colostrum are according to or like those found in the literature. For example, Donahue et al. (Citation2012) reported that TPC ranged from 5.0 to –5.9 log cfu mL−1 in fresh colostrum from six large commercial dairy farms in the USA. Gelsinger et al. (Citation2014) reported 4.59 log cfu mL−1 in raw colostrum, while Elizondo-Salazar et al. (Citation2010) reported 4.60 log cfu mL−1.
The higher TPC values in colostrum than in mature milk can represent an increased load of microorganisms if colostrum is added to the milk bulk tank. Elizondo-Salazar et al. (Citation2010) reported the presence of bacteria in naturally contaminated colostrum such as environmental streptococci (4.23 log cfu mL−1), Staphylococcus aureus (4.31 log cfu mL−1), and coliform count (4.31 log cfu mL−1) among others. In addition, Elizondo-Salazar et al. (2010) and Elizondo-Salazar et al. (Citation2010) reported that disease-causing pathogens that may be transmitted to dairy calves through colostral secretions include Mycobacterium avium subsp. paratuberculosis, Salmonella spp., Mycoplasma spp., Listeria monocytogenes, Campylobacter spp., Mycobacterium bovis, and Escherichia coli (also pathogenic bacteria relevant to human health risks). For this reason, and considering different types of microorganisms in colostrum, their presence in milk bulk tank can be a contamination factor that requires an adequate milk heat treatment to ensure its sanitation.
The IgG concentration was also significantly higher in colostrum (61.15 mg mL−1) than in mature milk (1.32 mg mL−1). Different authors have found that albumins and Igs represent higher concentration (6 g L−1) in colostrum than mature milk (0.5 y 0.09 g L−1, respectively) (Smolenski et al. Citation2007; Zhang et al. Citation2011), making up to 70–80% of the total protein in colostrum (Larson Citation1992).
These results reflect the biological differences between colostrum and mature milk, which could influence the technological characteristics of milk for cheese processing if colostrum is added to cheese milk, according to different authors (Rynne et al. Citation2004; McGrath et al. Citation2016).
Physicochemical characteristics of milks containing colostrum
The proximate composition of the milk containing 0%, 1%, 5% and 10% of colostrum, raw or pasteurized, is summarized in . As was expected, as colostrum increased in the milk, percentages of fat, protein, total solids, and non-fat solids were increased, while lactose contents decreased. These results are according to those found by other authors in transitional milk from colostrum to milk (Tsioulpas et al. Citation2007; Romero et al. Citation2014; Sánchez-Macías et al. Citation2014). According to Bogahawaththa et al. (Citation2017), a high protein (mainly whey protein) and mineral contents might render milk unsuitable for food processing operations, such as ultra-high temperature processes, and incompliant with the requirements regarding milk composition for processing (Marnila and Korhonen Citation2002; Tsioulpas et al. Citation2007; Sánchez-Macías et al. Citation2014).
Table 2. Composition of raw or pasteurized milk containing 0%, 1%, 5% and 10% of colostrum treated at 56°C for 1 h (LT56) or at 63°C for 30 min (LT63).
The physicochemical characteristics of raw and thermally treated milk samples containing colostrum concentrations up to 10% are detailed in and , respectively. As the concentration of colostrum was increased up to 10%, acidity and density also increased in milk. However, pH decreased significantly when the colostrum concentration in milk was 10%. These results are according to those found in transitional milk from colostrum to milk (Tsioulpas et al. Citation2007; Romero et al. Citation2014; Sánchez-Macías et al. Citation2014).
Table 3. Physicochemical characteristic of raw or pasteurized milk containing 0%, 1%, 5% and 10% of colostrum treated at 56°C for 1 h (LT56) or at 63°C for 30 min (LT63).
Table 4. Instrumental colour analysis of raw or pasteurized milk containing 0, 1, 5 and 10% of colostrum treated at 56°C for 1 h (LT56) and 63°C for 30 min (LT63).
An important characteristic of milk is its buffering capacity, i.e. resistance to changes in pH on addition of acid or base. The salts in mature milk (especially soluble calcium phosphate, citrate, and bicarbonate) and acidic and basic amino acid side-chains of proteins are mainly responsible for the buffering capacity (Fox et al. Citation2015). The buffering capacity of milk was not affected by colostrum up to 10%. Ethanol stability values (near to 70% or higher) were not affected by colostrum or heat treatment.
After the heat treatment of milk containing 0%, 1%, 5%, or 10% of colostrum, density and pH values decreased in all cases, while acidity values increased. Pasteurization causes some change in pH due to the loss of CO2 and precipitation of calcium phosphate (Fox et al. Citation2015). Heat treatments can result in a decrease on pH due to the thermal oxidation of lactose to various organic acids, hydrolysis of organic phosphate, among others, which accounts for 50% and 30% of the pH decrease, respectively (Singh Citation2004). This fact could explain the acidity increase and pH decrease of milk after heat treatment in this work.
Literature shows that acid–base equilibria in milk are influenced by processing operations, such as pasteurization above 100°C (Fox et al. Citation2015). However, in this experiment, neither the milk buffering capacity nor the alcohol stability was affected by the heat treatment, possibly because the pasteurization temperatures were low (56°C and 63°C).
L* and a* values were similar in mature milk and colostrum samples, but the yellow index (b*) differed markedly between colostrum (24.42 ± 12.17) and milk (13.73 ± 2.00) (). summarizes the instrumental colour parameters of milk samples containing 0%, 1%, 5% and 10% of colostrum. L* values were not affected by colostrum in milk or the pasteurization process. Red index values (greenish, negative values) were also not affected by colostrum presence; however, the values increased as pasteurization temperature was higher. The b* values of raw milk increased when the colostrum was added up to 5%. However, these differences due to colostrum disappeared when milk was pasteurized. On the other hand, the values of b* in raw milk and with 1% of colostrum were not affected by the pasteurization process, while this parameter decreased after LT56 and LT63 treatments in milks with 5% and 10% of colostrum. According to the criteria of Nedomová et al. (Citation2017), when 1% of colostrum was added to milk, the difference in colour related to the raw milk was considered lightly perceptible, while adding 5–10% colostrum resulted in higher differences for raw milk (middle differences). On the other hand, colour differences decreased with the pasteurization process, and these differences were lower as pasteurization temperature increased.
The probable reason for differences in colour data can be the higher carotenoid content of colostrum than in mature milk (Kehoe et al. Citation2007), which varies from yellow to orange and deep red-orange as the concentration of carotenoids increases (Antone et al. Citation2015).
Cheese-making properties of milk
As colostrum exhibits some extreme physical properties, this could be an important issue for the dairy industry because it could affect milk processability when it is present in milk bulk tank, as suggested by Sánchez-Macías et al. (Citation2014) and Tsioulpas et al. (Citation2007). As reported in scientific literature, casein content in milk is double than in colostrum, while the latter has reduced proportions of αS-caseins and elevated proportions of к-casein (Sobczuk-Szul et al. Citation2013). Alteration of protein content and the proportion of the individual proteins in milk could alter the quality of the milk after heat treatment, and consequently the cheese-making properties, the effectiveness of pasteurization and the quality of the final product. However, according to the obtained results (), there were no statistical differences in the rennet coagulation properties for RCT, RCF, F30, F45 and F60 parameters between non-added colostrum milk and those containing 1–10% of colostrum treated at 56°C for 1 h or at 63°C for 30 min. Furthermore, CY values were higher in LT63 milk samples than in their LT56 counterparts, while CD values were lower, but there were no statistical differences for these two parameters when colostrum was present in milk up to 10%.
Table 5. Cheese-making properties of raw or pasteurized milk containing 0, 1, 5 and 10% of colostrum treated at 56°C for 1 h (LT56) or at 63°C for 30 min (LT63).
According to Singh and Fox (Citation1985, Citation1986), heating at a pH value less than 6.7 results in a greater quantity of denatured whey proteins associated with casein micelles. During the rennet coagulation process, a weak curd structure and higher cheese yield could result from the incorporation of denatured whey proteins, mainly due to the interactions of these proteins with casein micelles after pasteurization (Singh and Waungana Citation2001). On the other hand, according to Bogahawaththa et al. (Citation2017), the main whey proteins (IgG, β-lactoglobulin, α-lactalbumin and bovine serum albumin) are heat sensitive with various thermal denaturation extensions, depending on the nature of the heat treatment, the individual physicochemical characteristics of these proteins and the influence of the interprotein interactions, generally through the interchange reactions between free sulphhydryl groups and disulphide bonds of proteins (Wijayanti et al. Citation2014; Bogahawaththa et al. Citation2017). Therefore, increasing the level of whey protein denaturation (by elevating the pasteurization temperature) could impair the rennet coagulation properties of milk when it is pasteurized above 72°C (Guinee Citation2021).
Pasteurization has either a positive influence (Salwa and Galal Citation2002; San Martín-González et al. Citation2007) or no effect on cheese yield (Drake et al. Citation1997). Cheese yield depends almost entirely on the moisture content of the cheese, fat and casein contents of milk, and the retention of each during cheese making (Guinee et al. Citation2007; De Marchi et al. Citation2008). It was expected that the intensity of heat treatment could affect the denaturation of whey proteins, which could be incorporated into cheese curd, resulting in a higher cheese yield when LT63 treatment was applied compared to LT56 treatment. The lower level of CD observed in curds from milk LT63-treated could be explained by the higher water retention of these curds due to the incorporation of a high extent of denatured whey proteins (Guinee Citation2021) compared to curds from milk LT56-treated.
Microbiological characteristics
shows the mean values of TPC of each milk sample and the pasteurization effectiveness through the bacterial reduction in percentage. TPC in raw milk was 3.67 log cfu mL−1. As colostrum concentration was higher in raw milk, TPC was increasing, and it was statistically different when 5% of colostrum was included in milk (4.41 log cfu mL−1). Both thermal treatments had similar bacterial reduction (99.81–99.90% of reduction) on raw milk (0% colostrum addition). However, the effectiveness of these discontinuous heat treatments diminished when 1, 5 y 10% of colostrum was included in milk (from 99.9% to 94.59% in LT56; and from 99.81% to 98.88% in LT63, when 10% of colostrum was added). These values are similar to others reported in the literature for LTLT treatment, such as those found by Liu et al. (Citation2020). These authors compared heat conventional and non-conventional treatment on milk and observed that thermal treatment at 63°C for 30 min resulted in a near three-log cycle reduction (99.85%) in total bacterial count (from 5.08 in raw milk to 2.26 log cfu mL−1 in pasteurized milk).
Table 6. Means values of total bacterial counts (log cfu mL−1) and bacterial reduction (%) of raw or pasteurized milk containing 0, 1, 5 and 10% of colostrum treated at 56°C for 1 h (LT56) or at 63°C for 30 min (LT63).
Johnson et al. (Citation2007) demonstrated that a heat treatment performed at 60°C for 30 min could significantly reduce pathogenic bacteria in colostrum. Elizondo-Salazar et al. (Citation2010) found that most bacterial groups require approximately 30 min or less at 60°C to destroy 90% of its populations in colostrum; however, environmental streptococci require at least 65 min at 57°C or 45 min at 63°C. Similarly, Donahue et al. (Citation2012) reported that bath heat treatment of colostrum at 60°C for 60 min significantly decreased coliform counts and TPC by 2.25 log and did not affect native IgG concentration.
In this study, after the heat treatment was carried out, the bacterial load decreased between 94.6 and 99.9% (from 1.3 to 3.1 log CFU mL−1 microbial reduction, as shown in ), depending on the presence of colostrum, which is considered a very good reduction. However, as reported by Elizondo-Salazar et al. (Citation2010), colostrum requires a longer time or temperature than the standard treatment at 63°C for 30 min, to eliminate a greater burden of environmental streptococci. These microorganisms are usually present in milk or colostrum due to environmental contamination, so it is necessary to maintain good hygiene and control, both udders and during the milking process, to reduce the contamination of this pathogen.
Figure 1. Microbial reduction (log cfu mL−1) of milk containing 0%, 1%, 5% and 10% of colostrum after heat treatment at 56°C for 1 h (LT56) or at 63°C for 30 min (LT63).
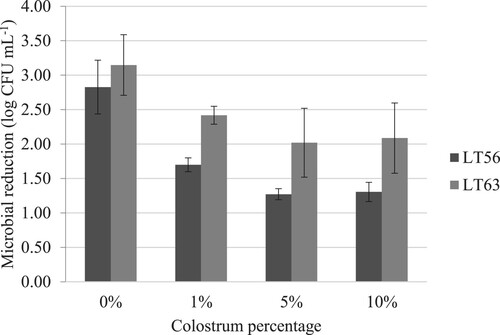
Because of these results, when the dairy industry suspects milk fraud with colostrum or when transitional milk is used, the conventional LTLT pasteurization process (63°C for 30 min) must be used to ensure the safety of their products. Various researchers (Boor and Murphy Citation2002; Barbano et al. Citation2006) have shown the poor quality of finished dairy products due to a higher bacteria content in raw milk. Current traditional methods of pasteurization have been effective in reducing bacterial load by as much as 5.0 log cfu mL−1 (Guan et al. Citation2005).
It is also necessary to mention that the high content of antimicrobial components in colostrum could affect the fermentation process (Marnila and Korhonen Citation2002; McGrath et al. Citation2016), or even the residual microbial content during the life of the products during storage. For this reason, it is necessary to further studies in the future to evaluate the presence of colostrum in milk on processed products during different storage periods.
Conclusions
The presence of colostrum up to 10% can modify the proximate composition and physicochemical characteristics of milk, which could impair the milk’s ability to be processed in the cheese industry. Cheese-making properties of milk are not affected by colostrum presence up to 10%, contrary to the expected. However, the heat treatment at 63°C for 30 min results in milk with higher values for CY and lower CD values, respectively, when compared with milk heat-treated at 56°C for 60.
Microbiological analysis results show the effectiveness of the two discontinuous thermal treatments (63°C for 30 min and 56°C for 60 min) reducing the TPC used in milk processing for the cheese industry. However, the first heat treatment proves to be more efficient for reducing the TPC than the second when colostrum is present in milk as low as 1%.
Because of these results, the conventional HTLT treatment of 63°C for 30 min is recommended when the presence of colostrum is suspected to ensure the safety of dairy products. However, it is necessary for further experiments in the future to evaluate the presence of colostrum on processed products during their storage periods.
As a recommendation for milk producers, the incidence of colostrum in milk bulk tank may be minimized by adopting appropriate operation and maintenance of milking equipment and contemporary dairy husbandry practices.
Acknowledgements
The authors are grateful to all members of the Research Group ‘Producción Animal e Industrialización’ from Universidad Nacional de Chimborazo.
Disclosure statement
No potential conflict of interest was reported by the author(s).
Additional information
Funding
References
- Abd El-Fattah AM, Abd Rabo FHR, EL-Dieb SM, Elkashef HAS. 2012. Changes in composition of colostrum of Egyptian buffaloes and Holstein cows. BMC Vet Res. 8. doi:10.1186/1746-6148-8-19.
- Abd El-Fattah AM, Abd Rabo FHR, El-Dieb SM, Elkashef HAS. 2014. Preservation methods of buffalo and bovine colostrum as a source of bioactive components. Int Dairy J. 39(1). doi:10.1016/j.idairyj.2014.04.008.
- Alegbeleye OO, Guimarães JT, Cruz AG, Sant’Ana AS. 2018. Hazards of a ‘healthy’ trend? An appraisal of the risks of raw milk consumption and the potential of novel treatment technologies to serve as alternatives to pasteurization. Trends Food Sci Technol. 82: 148–166. doi:10.1016/j.tifs.2018.10.007.
- Angulo FJ, LeJeune JT, Rajala-Schultz PJ. 2009. Unpasteurized milk: a continued public health threat. Clin Infect Dis. 48(1):93–100.
- Antone U, Zagorska J, Sterna V, Jemeljanovs A, Berzins A, Ikauniece D. 2015. Effects of dairy cow diet supplementation with carrots on milk composition, concentration of cow blood serum carotenes, and butter oil fat-soluble antioxidative substances. Agron Res. 13(4):879–891.
- Argüello A, Castro N, Capote J, Ginés R, Acosta F, López JL. 2003. Effects of refrigeration, freezing-thawing and pasteurization on IgG goat colostrum preservation. Small Ruminant Res. 48(2):135–139. doi:10.1016/S0921-4488(02)00277-8.
- Barbano DM, Ma Y, Santos MV. 2006. Influence of raw milk quality on fluid milk shelf life. J Dairy Sci. 89(Suppl 1). doi:10.3168/jds.s0022-0302(06)72360-8.
- Bogahawaththa D, Chandrapala J, Vasiljevic T. 2017. Thermal denaturation of bovine immunoglobulin G and its association with other whey proteins. Food Hydrocolloids. 72: 350–357. doi:10.1016/j.foodhyd.2017.06.017.
- Boor KJ, Murphy SC. 2002. Microbiology of market milks. In: R. K. Robinson, editor. Dairy microbiology handbook. 3rd ed. New York (NY): John Wiley and Sons, Inc; p. 91–122.
- Borad SG, Singh AK. 2018. Colostrum immunoglobulins: processing, preservation and application aspects. Int Dairy J. 85: 201–210. doi:10.1016/j.idairyj.2018.05.016.
- Borad SG, Singh AK, Kapila S, Behare P, Arora S, Sabikhi L. 2019. Influence of unit operations on immunoglobulins and thermal stability of colostrum fractions. Int Dairy J. 93:85–91. doi:10.1016/j.idairyj.2019.02.007.
- Bucci AJ, Van Hekken DL, Tunick MH, Renye JA, Tomasula PM. 2018. The effects of microfluidization on the physical, microbial, chemical, and coagulation properties of milk. J Dairy Sci. 101(8):6990–7001. doi:10.3168/jds.2017-13907.
- Calvo MM, Balcones E. 1998. Influence of heat treatment on rennet clotting properties of mixtures of cow’s, ewe’s, and goat’s milk and on cheese yield. J Agric Food Chem. 46(8):2957–2962. doi:10.1021/jf9801623.
- Calvo MM, Espinoza NA. 1999. Syneresis rate of cow’s, ewe’s, and goat’s curd. effect of thermal treatment and ultrafiltration. J Agric Food Chem. 47(3):883–886. doi:10.1021/jf980783w.
- De Marchi M, Bittante G, Dal Zotto R, Dalvit C, Cassandro M. 2008. Effect of Holstein Friesian and Brown Swiss breeds on quality of milk and cheese. J Dairy Sci. 91(10):4092–4102. doi:10.3168/jds.2007-0788.
- Donahue M, Godden SM, Bey R, Wells S, Oakes JM, Sreevatsan S, Fetrow J. 2012. Heat treatment of colostrum on commercial dairy farms decreases colostrum microbial counts while maintaining colostrum immunoglobulin G concentrations. J Dairy Sci. 95(5):2697–2702. doi:10.3168/jds.2011-5220.
- Drake MA, Harrison SL, Asplund M, Barbosa-Canovas G, Swanson BG. 1997. High pressure treatment of milk and effects on microbiological and sensory quality of cheddar cheese. J Food Sci. 62(4):843–860. doi:10.1111/j.1365-2621.1997.tb15468.x.
- Elizondo-Salazar JA, Jayarao BM, Heinrichs AJ. 2010. Effect of heat treatment of bovine colostrum on bacterial counts, viscosity, and immunoglobulin G concentration. J Dairy Sci. 93(3):961–967. doi:10.3168/jds.2009-2388.
- Fox PF, Uniacke-Lowe T, McSweeney PLH, O’Mahony JA. 2015. Heat-induced changes in milk. In: Dairy chemistry and biochemistry. Cham: Springer; p. 345–375. doi:10.1007/978-3-319-14892-2_9.
- Gapper LW, Copestake DEJ, Otter DE, Indyk HE. 2007. Analysis of bovine immunoglobulin G in milk, colostrum and dietary supplements: a review. Anal Bioanal Chem. 389(1):93–109. doi:10.1007/s00216-007-1391-z.
- Gedam K, Prasad R, Vijay VK. 2007. The study on UHT processing of milk: a versatile option for rural sector. World J Dairy Food Sci. 2(2):49–53.
- Gelsinger SL, Gray SM, Jones CM, Heinrichs AJ. 2014. Heat treatment of colostrum increases immunoglobulin G absorption efficiency in high-, medium-, and low-quality colostrum. J Dairy Sci. 97(4):2355–2360. doi:10.3168/jds.2013-7374.
- Godden SM, Lombard JE, Woolums AR. 2019. Colostrum management for dairy calves. Vet Clin North Am Food Anim. 35(3):535–556. doi:10.1016/j.cvfa.2019.07.005.
- Guan D, Chen H, Hoover DG. 2005. Inactivation of Salmonella typhimurium DT 104 in UHT whole milk by high hydrostatic pressure. Int J Food Microbiol. 104(2): 145–153. doi:10.1016/j.ijfoodmicro.2005.01.014.
- Guinee TP. 2021. Effect of high-temperature treatment of milk and whey protein denaturation on the properties of rennet–curd cheese: A review. Int Dairy J 121. 105095. doi:10.1016/j.idairyj.2021.105095.
- Guinee TP, Mulholland EO, Kelly J, Callaghan DJO. 2007. Effect of protein-to-fat ratio of milk on the composition, manufacturing efficiency, and yield of cheddar cheese. J Dairy Sci. 90(1):110–123. doi:10.3168/jds.S0022-0302(07)72613-9.
- Huppertz T, Fox PF, Kelly AL. 2004. Influence of high pressure treatment on the acidification of bovine milk by lactic acid bacteria. Milchwissenschaft. 59(5–6):246–249.
- Indyk HE, Williams JW, Patel HA. 2008. Analysis of denaturation of bovine IgG by heat and high pressure using an optical biosensor. Int Dairy J. 18(4):359–366. doi:10.1016/j.idairyj.2007.10.004.
- Jeong S-G, Ham J-S, Kim D-H, Ahn C-N, Chae H-S, You Y-M, Jang A, Kwon I-K, Lee S-G. 2009. Physicochemical properties of colostrum by milking time of gyeonggi province. Korean J Food Sci Ani Resour. 29(4):445–456.
- Johnson JL, Godden SM, Molitor T, Ames T, Hagman D. 2007. Effects of feeding heat-treated colostrum on passive transfer of immune and nutritional parameters in neonatal dairy calves. J Dairy Sci. 90(11):5189–5198. doi:10.3168/jds.2007-0219.
- Kehoe SI, Jayarao BM, Heinrichs AJ. 2007. A survey of bovine colostrum composition and colostrum management practices on pennsylvania dairy farms. J Dairy Sci. 90(9):4108–4116. doi:10.3168/jds.2007-0040.
- Korhonen H. 2009. Milk-derived bioactive peptides: from science to applications. J Funct Foods. 1(2):177–187.
- Larson BL. 1992. Immunoglobulins of the mammary secretions. In: Fox PF, editor. Advanced dairy chemistry-1: proteins. 2nd ed. London: Elsevier Applied Science; p. 231–254.
- Lindström P, Paulsson M, Nylander T, Elofsson U, Linkmark-Månsson H. 1994. The effect of heat treatment on bovine immunoglobulins. Milchwissenschaft. 49(2):67–71.
- Liu G, Carøe C, Qin Z, Munk DM, Crafack M, Petersen MA, Ahrné L. 2020. Comparative study on quality of whole milk processed by high hydrostatic pressure or thermal pasteurization treatment. LWT. 127:109370. doi:10.1016/j.lwt.2020.109370.
- Madsen BD, Rasmussen MD, Nielsen MO, Wiking L, Larsen LB. 2004. Physical properties of mammary secretions in relation to chemical changes during transition from colostrum to milk. J Dairy Res. 71(3):263–272. doi:10.1017/S0022029904000263.
- Marnila P, Korhonen H. 2002. Colostrum. Encyclopedia of Dairy Sci. 1:473–478.
- McGrath BA, Fox PF, McSweeney PLH, Kelly AL. 2016. Composition and properties of bovine colostrum: a review. Dairy Sci Technol. 96(2):133–158. doi:10.1007/s13594-015-0258-x.
- Morales-delaNuez A, Hernández-Castellano LE, Moreno-Indias I, Sánchez-Macías D, Argüello A, Castro N. 2020. Use of glycerol and propylene glycol as additives in heat-treated goat colostrum. J Dairy Sci. 103(3):2756–2761. doi:10.3168/jds.2019-17535.
- Morrill KM, Conrad E, Lago A, Campbell J, Quigley J, Tyler H. 2012. Nationwide evaluation of quality and composition of colostrum on dairy farms in the United States. J Dairy Sci. 95(7):3997–4005. doi:10.3168/jds.2011-5174.
- Nedomová Š, Kilián L, Pytel R, Kumbár V. 2017. Effect of ripening time on colour and texture properties in cheese. Potravinarstvo Slovak J Food Sci. 11(1):296–301. doi:10.5219/744.
- Pakkanen R, Aalto J. 1997. Growth factors and antimicrobial factors of bovine colostrum. Int Dairy J. 7(5):285–297. doi:10.1016/S0958-6946(97)00022-8.
- Romero T, Beltrán MC, Pérez-Baena I, Rodríguez M, Molina MP. 2014. Effect of the presence of colostrum on microbial screening methods for antibiotic detection in goats’ milk. Small Ruminant Res. 121(2–3):376–381. doi:10.1016/j.smallrumres.2014.07.007.
- Rynne NM, Beresford TP, Kelly AL, Guinee TP. 2004. Effect of milk pasteurization temperature and in situ whey protein denaturation on the composition, texture and heat-induced functionality of half-fat cheddar cheese. Int Dairy J. 14(11):989–1001. doi:10.1016/j.idairyj.2004.03.010.
- Salwa AA, Galal EA. 2002. Effect of milk pretreatment on the keeping quality of domiati cheese. Pak J Nutr. 1(3):132–136.
- Sánchez-Macías D, Moreno-Indias I, Castro N, Morales-delaNuez A, Argüello A. 2014. From goat colostrum to milk: physical, chemical, and immune evolution from partum to 90 days postpartum. J Dairy Sci. 97(1):10–16. doi:10.3168/jds.2013-6811.
- San Martín-González MF, Rodríguez JJ, Gurram S, Clark S, Swanson BG, Barbosa-Cánovas GV. 2007. Yield, composition and rheological characteristics of cheddar cheese made with high pressure processed milk. LWT - Food Sci Technol. 40(4):697–705. doi:10.1016/j.lwt.2006.03.022.
- Sarkar S. 2015. Microbiological considerations: pasteurized milk. Int J Dairy Sci. 10(5):206–218. doi:10.3923/ijds.2015.206.218.
- Sharma P, Bremer P, Oey I, Everett DW. 2014. Bacterial inactivation in whole milk using pulsed electric field processing. Int Dairy J. 35(1):49–56. doi:10.1016/j.idairyj.2013.10.005.
- Singh H. 2004. Heat stability of milk. Int J Dairy Technol. 57(2–3). doi:10.1111/j.1471-0307.2004.00143.x.
- Singh H, Fox PF. 1985. Heat stability of milk: pH-dependent dissociation of micellar K-casein on heating milk at ultra high temperatures. J Dairy Res. 52:529–538.
- Singh H, Fox PF. 1986. Heat stability of milk: further studies on the pH-dependent dissociation of micellar /c-casein. J Dairy Res. 53:237–248.
- Singh H, Havea P. 2003. Thermal denaturation, aggregation and gelation of whey proteins. In: Fox PF, McSweeney PLH, editors. Advanced dairy chemistry—1 proteins. Boston (MA): Springer; p. 1261–1287.
- Singh H, Waungana A. 2001. Influence of heat treatment of milk on cheesemaking properties. Int Dairy J. 11(4–7). doi:10.1016/S0958-6946(01)00085-1.
- Smolenski G, Haines S, Kwan FYS, Bond J, Farr V, Davis SR, Stelwagen K, Wheeler TT. 2007. Characterisation of host defence proteins in milk using a proteomic approach. J Proteome Res. 6(1):207–215. doi:10.1021/pr0603405.
- Sobczuk-Szul M, Wielgosz-Groth Z, Wroński M, Rzemieniewski A. 2013. Changes in the bioactive protein concentrations in the bovine colostrum of jersey and Polish Holstein-Friesian cows. Turk J Vet Anim Sci. 37(1). doi:10.3906/vet-1107-42.
- Steinbach G, Kreutzer B, Meyer H. 1981. Zum einfluss der erwarmung auf den immunbiologischen wert des rinderkolostrums. Monatsh Veterinarmed. 36:29–31.
- Trujillo AJ, Castro N, Quevedo JM, Argüello A, Capote J, Guamis B. 2007. Effect of heat and high-pressure treatments on microbiological quality and immunoglobulin G stability of caprine colostrum. J Dairy Sci. 90(2):833–839. doi:10.3168/jds.S0022-0302(07)71567-9.
- Tsioulpas A, Grandison AS, Lewis MJ. 2007. Changes in physical properties of bovine milk from the colostrum period to early lactation. J Dairy Sci. 90(11):5012–5017. doi:10.3168/jds.2007-0192.
- Verraes C, Vlaemynck G, Van Weyenberg S, De Zutter L, Daube G, Sindic M, Uyttendaele M, Herman L. 2015. A review of the microbiological hazards of dairy products made from raw milk. Int Dairy J. 50:32–44. doi:10.1016/j.idairyj.2015.05.011.
- Wijayanti HB, Bansal N, Deeth HC. 2014. Stability of whey proteins during thermal processing: a review. Compr Rev Food Sci Food Saf. 13(6):1235–1251. doi:10.1111/1541-4337.12105.
- Zhang LY, Wang JQ, Yang YX, Bu DP, Li SS, Zhou LY. 2011. Comparative proteomic analysis of changes in the bovine whey proteome during the transition from colostrum to milk. Asian-Australas J Anim Sci. 24(2). doi:10.5713/ajas.2011.10122.