ABSTRACT
Co-culture is mimicked in vivo condition, which is influenced by adjacent cells. This study aimed to determine different myogenic genes in mono- and co-culture of satellite cells and fibroblasts. Fibroblasts and satellite cells were isolated from the piglet’s thigh muscles. The expression of PAX-7, MyoD, MyoG, and PAX-3 was downregulated in 24 h co-culture satellite cells than in monoculture. The expression of Myf-5 and CAV-1 was upregulated in 24 h co-culture satellite cells than in 24 h monoculture. The expression of PAX-7, MyoD, and Myf-5 was downregulated in 24 and 48 h co-culture fibroblasts than 24 h monoculture. The expression of MyoG and PAX-3 was upregulated in 24 and 48 h co-culture than 48 h monoculture fibroblasts. The Cyto-C was downregulated in 24 and 48 h co-culture fibroblast than 24 h monoculture. The expression of MyoG and CAV-1 was upregulated in 48 h incubation period co-culture fibroblasts than 24 h monoculture. The MyoD and CAV-1 expression were upregulated in 24 h co-culture than 24 h satellite cells and fibroblasts. The Bcl-2 and BAX were downregulated in 48 h co-culture than 24 h. This result recommended that the satellite cells and fibroblasts interaction enhance the myogenesis marker expression without cellular morphology change.
GRAPHICAL ABSTRACT
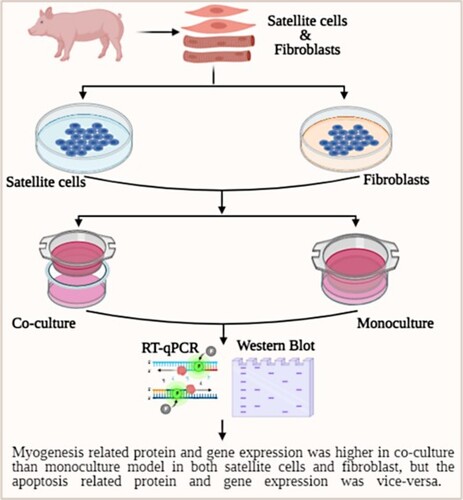
Introduction
Satellite cells are primary stem cells that can repair injured muscles (Almada and Wagers Citation2016). Generally, satellite cells can differentiate themselves from other cells by expressing transcription factor paired box protein 7 (PAX-7) (Seale et al. Citation2000, Citation2004). Previous studies have reported that PAX-7 can maintain satellite cells (Kuang et al. Citation2006; Relaix et al. Citation2006) without any contributions to satellite cell regeneration (Lepper et al. Citation2009). Interestingly, transplanted satellite cells can regenerate muscle fibres in an in vivo study (Collins et al. Citation2005). Satellite cells are influenced by their surroundings. Previous studies have reported that the proliferation and differentiation of satellite cells are regulated by macrophages (Christov et al. Citation2007; Cornelison Citation2008; Tidball and Villalta Citation2010). Generally, satellite cells are inactive in a sound condition, although they become active at injured muscles for repairing (Hill et al. Citation2003). Therefore, satellite cells can be defined as muscle primary stem cells that are capable of self-renewal. Fibroblasts are cells that can produce extracellular matrix (ECM; Spatz Citation2004). They play a crucial role in the infrastructure and remodelling of tissues and organs (Yeo et al. Citation2018). Moreover, these cells can repair damaged tissues and maintain the skeleton structure by ECM (Tomasek et al. Citation2002; Parsonage et al. Citation2005). A previous study has reported that fibroblasts might have therapeutic applications for wound healing (Philippeos et al. Citation2018). In addition, fibroblasts can influence the satellite cells to repair injured muscle by increasing the number of satellite cells (Mendias Citation2017).
Transcriptional factor analysis characterizes different gene expressions in various muscles from different breeds, which is phenotypically different (Guo et al. Citation2015). Such analysis can also help us understand the genetic mechanism that contributes to myogenesis (He and Liu Citation2013). Generally, myogenesis is regulated by myogenic regulatory factors (MRFs) known to modulate the process of new muscle formation and wound healing (Braun and Gautel Citation2011). Several genes contribute to the differentiation and growth of muscle cells. Among these genes, Myf-5, MyoD, MRF4/MYF6, and MyoG mostly affect myogenesis (Eng et al. Citation2013). The expression of Cyto-C can enhance mitochondrial respiration and function (Baldelli et al. Citation2014). Therefore, the expression of Cyto-C is increased during myogenic differentiation (Remels et al. Citation2010). When cell receives get any stimulation, then Cyto-C expression can regulate apoptosis (Ow et al. Citation2008). The PAX family has a total of nine members. They have a common paired-box domain for binding to a specific DNA. The PAX-3 and PAX-7 are present in skeletal muscles with overlapping functions (Buckingham and Relaix Citation2007). Although PAX-3 has a critical role in fetal and embryonic development, its function in the postnatal stage remains unclear (Young and Wagers Citation2010). Interestingly, PAX-7 can substitute for PAX-3 for muscle formation (Relaix et al. Citation2004). However, during the postnatal stage, PAX-3 cannot substitute for PAX-7 (Lagha et al. Citation2008). The CAV-1 is the main component of caveolae. It regulates oxidative stress (Mougeolle et al. Citation2015). Although CAV-1 is expressed in satellite cells, it is not expressed in mature muscles where it inhibits cell proliferation and cell cycle (Volonte et al. Citation2005).
A lesser amount of the cell differentiation process induces apoptosis. A recent study has reported that differentiation can reduce the apoptosis of mouse embryonic stem cells (Zhang et al. Citation2020). Moreover, Bcl-2 family proteins can regulate apoptosis, neuronal activity, autophagy, calcium, and other mechanisms of healthy cells (Hardwick and Soane Citation2013). Additionally, Bcl-2 can prevent cell apoptosis by interacting with apoptotic factors (Gross and Katz Citation2017). A pro-apoptotic protein BAX in mitochondria has been found to be able to regulate apoptosis (Westphal et al. Citation2011).
The co-culture of cells can better mimic the in vivo environment than the monoculture of cells (Kämpfer et al. Citation2017). A previous study has reported that co-culture can stimulate the in vivo environment by cell–cell interaction better than monoculture (Miki et al. Citation2012). Here, we observed interactions between satellite cells and fibroblast isolated from one-day-old Jeju black pig. Additionally, we observed that the Jeju black pig satellite cells and fibroblast influenced each other for myogenesis and apoptosis-related gene and protein expression, as well as cellular morphology.
Materials and methods
Reagents
Some important reagents were essential for cell isolation and cell culture. These reagents included high glucose Dulbecco’s Modified Eagle Medium (DMEM-HG; Gibco, cat. no. 11965092), fetal bovine serum (FBS; Gibco, cat. no. 10270106), 2-[4-(2-hydroxyethyl)-1-piperazine]-1-ethanesulfonic acid buffer (HEPES buffer, 1M; Gibco, cat. no. 15630080), phosphate-buffered saline (PBS; LPS Solution, cat. no. CBP007B), protease (Sigma, cat. no. P8811, 3.5 U mg−1), collagenase (Sigma, cat. no. C7657), and 100× penicillin–streptomycin (10,000 U mL−1) (Invitrogen, cat. no. 15140-122).
Reagents preparation
All reagents were prepared very carefully. Antibiotic (1%), PBS (10%), and distilled water (89%) were mixed to prepare washing solution. PBS (10%), antibiotic (1%), HEPS (4.2%), and distilled water (84.8%) were mixed to prepare mincing solution. Protease powder (75 mg), 10 × PBS (5 mL), HEPES (2.1 mL), and distilled water (42.9 mL) were mixed together to prepare protease solution. Collagenase type XI (1.5 mg), DMEM-HG (1 mL), and FBS (5%) were mixed together to prepare collagenase solution. Proliferation solution (1% antibiotic and 20% FBS in DMEM-HG) was prepared and stored at 4°C.
Isolation of fibroblast and satellite cells
Fibroblasts and satellite cells were isolated from thigh muscles of one-day-old Jeju black pig under the animal biotechnology department of Jeonbuk National University (Park et al. Citation2021) following a previous method (Hasan Siddiqui, Sivakumar, et al. Citation2020; Siddiqui, Kang, et al. Citation2020) with little changes. Briefly, muscles were collected from the thigh of the pig’s hind leg. Collected muscles were immediately transferred into a sterile collection tube containing cold PBS. Collected tissues were transferred into a new sterile Petri dish in a cell culture hood and washed three to four times with four volumes of cold PBS. These muscles were washed three times with washing solution to wash out blood vessels, adipose tissues, and other debris. Muscles were then minced with sharp scissors in the mincing solution. These minced muscles were digested with a protease solution for 1 h at 37°C. After protease digestion, pellet and supernatant were separated by centrifuging at 200g for 5 min at 4°C. The collected pellet was digested with collagenase solution for 1 h at 37°C. After collagenase digestion, cell suspension was filtrated with 100 and 70 μm strainers and centrifuged at 2000g for 10 min at 4°C to collect the supernatant. The pellet was then subjected to protease digestion followed by filtration and centrifugation. Both supernatants were combined and centrifuged at 2000g for 5 min at 4°C. The pellet was suspended with a proliferation solution. After filtrating, this cell suspension with a 40 μm strainer, the filtrate was centrifuged at 700g for 10 min at 4°C. The cell pellet was mixed with cell proliferation solution and seeded into a 75 mm cell culture flask. After 1 h of incubation at 37°C, satellite cells in the supernatant were separated from fibroblasts attached to the bottom of the cell culture flask.
Cell culture and experimental design
Primary pig fibroblast and satellite cells were cultured in DMEM-HG media containing 10% FBS and 1% antibiotics in a 5% CO2 incubator at 37°C. We accomplished our all experiments using cells within 3–6 cell passages. The total experiment was grouped by satellite cells and fibroblasts. Furthermore, the fibroblasts and satellite cells were again grouped by monoculture and co-culture with three replications (n = 3; ). The incubation time was 24 or 48 h for both satellite cells and fibroblast. Cell culture medium was changed every 24 h for each group.
RNA extraction and qRT-PCR
Expression levels of MyoG, MyoD, Myf-5, PAX-3, PAX-7, CAV-1, and Cyto-C genes in fibroblasts and satellite cells of pig were analysed by qRT-PCR. Both cells (satellite cells and fibroblasts) (2 × 105 cells mL−1) were seeded into 6-well plate and 0.4 μm pore size co-culture plates (SPL life science, Gyeonggi-do, Korea) for monoculture and co-culture individually with 2 mL complete medium. The mRNA was isolated from mono- and co-cultured of both satellite cells and fibroblasts after experimental incubation time.
Primer sequences of targeted genes and reference gene (glyceraldehyde 3-phosphate dehydrogenase, GAPDH) used for this experiment is presented in . Total RNA was extracted from each group using Trizol (Invitrogen, NY, USA). The concentration of RNA was determined based on absorbance at 230 nm and the quality of RNA was determined based on absorbance 260/280 nm using a Nanodrop spectrophotometer (Thermo Fisher Scientific, Waltham, Massachusetts, USA). One microgram of total RNA was used for reverse transcription with an iScript cDNA synthesis kit (Bio-Rad, CA, USA) following the manufacturer’s instructions. Targeted gene expression level was analysed by qRT-PCR using SYBR Green® Supermix (TOYOBO, Osaka, Japan) on a CFX96 Real-Time PCR Detection System (Bio-Rad). PCR program was a denaturation step at 95°C for 30 s, 40 cycles of 95°C for 5 s and 58°C for 5 s, followed by a melting step at temperature of 65–95°C for 5 s. Fold changes of gene expression levels were calculated as described in our previous study (Hasan Siddiqui, Kang, et al. Citation2020; Siddiqui, Subramaniyan, et al. Citation2020). The relative gene expression was determined as fold change using the 2-ΔΔCt method (Livak and Schmittgen Citation2001).
Table 1. Primer sequences, amplicon size of different genes for RT-qPCR analysis.
Protein extraction and western blotting
Myogenesis and apoptotic marker can indicate muscle synthesis and cell apoptosis. Western blotting was performed to analyse Bcl-2, BAX, CAV-1, PAX-7, and MyoD protein expression levels in monoculture and co-culture pig fibroblasts and satellite cells. Both cells (satellite cells and fibroblasts) (2 × 105 cells mL−1) were seeded into 6-well plate and 0.4 μm pore size co-culture plates (SPL life science, Gyeonggi-do, Korea) for monoculture and co-culture individually with 2 mL complete medium. Total proteins were isolated from fibroblasts and satellite cells using a protease inhibitor (Invitrogen, NY, USA) mixed with RIPA buffer (150 mM sodium chloride, 1% Triton X-100, 0.1% SDS, 1% sodium deoxycholate, 50 mM tris-HCl, pH 7.5, 2 mM EDTA). DC protein assay kit (Bio-Rad, CA, USA) was used to measure protein concentration. Protein samples (30 μg) were subjected to 12% Bis-Tris gel (Bio-Rad, CA, USA) electrophoresis (SDS-PAGE). Proteins were then transferred to nitrocellulose membranes. After blocking with 5% skim milk for 1 h at room temperature, membranes were incubated with primary antibody at 4°C overnight. After overnight incubation, membranes were washed with Tris buffer solution containing 0.5% Tween-20 (TBST) three times (10 min each wash). Thereafter, membranes were incubated with HRP-conjugated respective secondary antibody for 1 h at room temperature. To visualize protein bands, the chemiluminescent substrate (Invitrogen, NY, USA) was used. An iBright CL1000 was used for image taking. All targeted protein levels were normalized against GAPDH. All primary antibodies including GAPDH (Enzo Life Science, NY, USA) were diluted with blocking buffer at 1:2500 dilution. The secondary antibody (Enzo Life Science, NY, USA) was diluted with blocking buffer at 1:5000 dilutions.
Acridine orange (AO) and ethidium bromide (EtBr) staining
Pig monoculture and co-culture fibroblasts and satellite cells (2 × 105) were seeded in each confocal and co-culture dish and 0.4 μm pore size co-culture plates (SPL life science, Gyeonggi-do, Korea) with 2 mL of complete media. After respective incubation time, cells were washed with PBS. For fixation of cells, methanol:acetic acid (3:1) was added into each dish followed by incubation at room temperature for 30 min. After incubation, cells were washed with ice cold PBS. Cells were then dual stained with acridine orange/ethidium bromide (AO/EtBr) (100 μg mL−1 AO and 100 μg mL−1 EtBr) (Sigma, St. Louis, MO, USA) at room temperature for 10 min in a dark room. Thereafter, excessive stain was washed with ice cold PBS. Next, mounting media (Vector Laboratories, CA, USA) was added to protect cells from drying. Image was taken using a fluorescence microscope (ZEISS LSM 880 Airyscan, Carl Zeissa, Jena, Germany).
Statistical analyses
All data of this experiment were analysed using SAS version 9.4 (SAS Institute Inc., Cary, NC, USA). Data are presented as mean ± standard deviation (SD) from three replications for each experimental group. Duncan’s multiple range test followed by the analysis of variance (ANOVA) was used to compare gene and protein expression levels among different groups of mono- and co-culture fibroblasts and satellite cells.
Results
Myogenic genes expression in mono- and co-culture porcine skeletal muscle cells
Results of PAX-7, PAX-3, MyoD, MyoG, Myf-5, and CAV-1 mRNA expression levels in mono- and co-culture porcine satellite cells and fibroblasts are shown in . In satellite cells, the expression levels of MyoD, PAX-7, and PAX-3 were downregulated in co-culture satellite cells at both 24 and 48 h incubation ((a)). Moreover, the expression of MyoD and PAX-7 was significantly (p < 0.05) decreased in 48 h incubation than 24 h incubation time in both mono- and co-culture satellite cells. The expression of MyoG was upregulated in monoculture satellite cells at 48 h incubation. The expression of Myf-5 was upregulated in co-culture satellite cells at 24 h incubation. In addition, the CAV-1 mRNA expression was upregulated in co-culture satellite cells at both 24 and 48 h incubation, and 48 h incubation of monoculture satellite cells.
Figure 2. The expression of the myogenesis-related mRNA (PAX-7, MyoD, MyoG, PAX-3, Myf-5, and CAV-1) in the mono- and co-culture porcine fibroblast and satellite cells (n = 3). (a) Jeju black pig satellite. Here, S-24: monoculture satellite cells 24 h incubation; S-48: monoculture satellite cells 48 h incubation; F/S-24: co-culture satellite cells 24 h incubation; and F/S-48: co-culture satellite cells 48 h incubation. (b) Jeju black pig fibroblast. Here, F-24: monoculture fibroblast cells 24 h incubation; F-48: monoculture fibroblast cells 48 h incubation; S/F-24: co-culture fibroblast cells 24 h incubation; and S/F-48: Co-culture fibroblast cells 48 h incubation. a–dPAX-7, MyoD, MyoG, PAX-3, Myf-5, and CAV-1 in the columns with different superscript letters differ significantly (p < 0.05) among the groups. Passage number of both cells was 4 in this experiment.
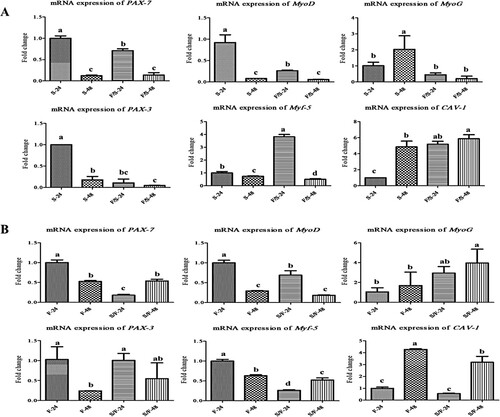
In fibroblast, the expression levels of MyoD, PAX-7, and Myf-5 were downregulated in co-culture fibroblast at both 24 and 48 h incubation ((b)). Moreover, the expression of MyoD was significantly (p < 0.05) decreased in 48 h incubation than 24 h incubation time in both mono- and co-culture fibroblast. The mRNA expression of MyoG was upregulated in co-culture fibroblast at both 24 and 48 h incubation. The mRNA expression of PAX-3 was downregulated in monoculture of fibroblast at 48 h incubation. The mRNA expression of CAV-1 was upregulated at 48 h incubation in both mono- and co-culture fibroblast.
Expression of apoptosis genes in mono- and co-culture porcine skeletal muscle cells
The mRNA expression levels of Cyto-C in mono- and co-culture fibroblast cells and satellite cells were analysed to determine apoptosis (). In satellite cells, the mRNA expression of Cyto-C was not significantly different among the experimental groups ((a)). In fibroblast, the mRNA expression of Cyto-C was downregulated in co-culture ((b)).
Figure 3. The expression of the apoptosis-related mRNA in the mono- and co-culture porcine fibroblast and satellite cells (n = 3). (a) Jeju black pig satellite cells. Here, S-24: monoculture satellite cells 24 h incubation; S-48: monoculture satellite cells 48 h incubation; F/S-24: co-culture satellite cells 24 h incubation; and F/S-48: co-culture satellite cells 48 h incubation. (b) Jeju black pig fibroblast. Here, F-24: monoculture fibroblast cells 24 h incubation; F-48: monoculture fibroblast cells 48 h incubation; S/F-24: co-culture fibroblast cells 24 h incubation; and S/F-48: co-culture fibroblast cells 48 h incubation. a-cThe mean relative expression of Cyto-C in the columns with different superscript letters differ significantly (p < 0.05) among the groups. Passage number of both cells was 4 in this experiment.
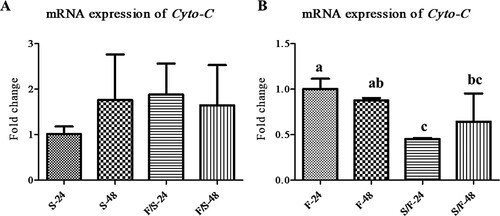
The fold changes of mRNA expression related to myogenesis and apoptosis in fibroblast and satellite cells interaction
The fold changes of mRNA expression related to myogenesis and apoptosis in fibroblasts and satellite cells interaction were visualized by heat map (). In satellite cells, the expression of Myf-5 was upregulated in co-culture at 24 h incubation ((a)). The expression of MyoG was upregulated in monoculture at 48 h incubation. The mRNA expression of CAV-1 was higher at 48 h incubation in monoculture, as well as higher expression was found at both 24 and 48 h incubation time in co-culture. The expression of Cyto-C was upregulated in co-culture at both incubations and monoculture at 48 h incubation.
Figure 4. Heat map generated from fold change of myogenesis and apoptosis-related mRNA expression. (a) Jeju black pig satellite cells. Here, S-24: monoculture satellite cells 24 h incubation; S-48: monoculture satellite cells 48 h incubation; F/S-24: co-culture satellite cells 24 h incubation; and F/S-48: co-culture satellite cells 48 h incubation. (b) Jeju black pig fibroblast. Here, F-24: monoculture fibroblast cells 24 h incubation; F-48: monoculture fibroblast cells 48 h incubation; S/F-24: co-culture fibroblast cells 24 h incubation; and S/F-48: co-culture fibroblast cells 48 h incubation. The pink colour represents higher expression and the red colour represents the lower expression of mRNA.
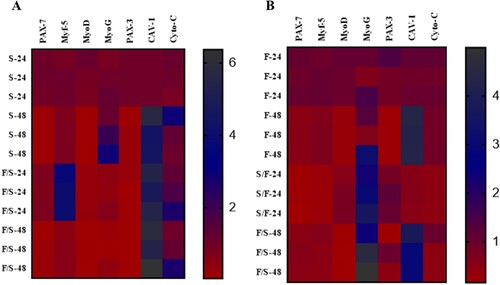
In fibroblasts, the expression of MyoG was upregulated in co-culture at both 24 and 48 h incubation, as well as monoculture at 48 h incubation ((b)). The mRNA expression of CAV-1 was upregulated at 48 h incubation in monoculture, as well as higher expression was found at 48 h incubation time in co-culture.
Myogenic proteins expression in mono- and co-culture porcine skeletal muscle cells
In satellite cells, no remarkable change in PAX-7 protein expression occurred in any group ((a)). However, MyoD protein expression was significantly (p < 0.05) increased in co-culture at 24 h incubation compared to other groups. Moreover, the expression of MyoD was upregulated in co-culture at both incubations compared to monoculture. The protein expression of CAV-1 was upregulated in co-culture at 24 h incubation.
Figure 5. The expression of the myogenesis-related proteins (PAX-7, MyoD, and CAV-1) in the mono- and co-culture porcine fibroblast and satellite cells (n = 3). (a) Jeju black pig satellite cells. Here, S-24: monoculture satellite cells 24 h incubation; S-48: monoculture satellite cells 48 h incubation; F/S-24: co-culture satellite cells 24 h incubation; and F/S-48: co-culture satellite cells 48 h incubation. (b) Jeju black pig fibroblast. Here, F-24: monoculture fibroblast cells 24 h incubation; F-48: monoculture fibroblast cells 48 h incubation; S/F-24: co-culture fibroblast cells 24 h incubation; and S/F-48: co-culture fibroblast cells 48 h incubation. (c) Western blot analysis results. a–dBcl-2 and BAX in the columns with different superscript letters differ significantly (p < 0.05) among the groups. Passage number of both cells was 5 in this experiment.
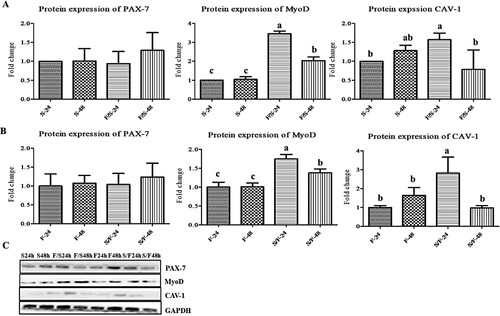
In fibroblast, no significant change in PAX-7 protein expression occurred in any group ((b)). However, MyoD protein expression was significantly (p < 0.05) increased in co-culture at 24 h incubation compared to other groups. Furthermore, the expression of MyoD was upregulated in co-culture at both incubations. The protein expression of CAV-1 was upregulated in co-culture at 24 h incubation. The bands of western blots of myogenesis-related proteins analysis of pig satellite cells and fibroblast have been presented together ((c)).
Expression levels of apoptosis-related proteins in mono- and co-cultured porcine skeletal muscle cells
In satellite cells, the protein expression levels of Bcl-2 in co-culture at 24 incubations was significantly (p < 0.05) decreased compared to monoculture at 24 h incubation. The protein expression level of MyoD was downregulated in co-culture satellite cells at both 24 and 48 h incubation ((a)). Moreover, the expression of MyoD was significantly (p < 0.05) decreased in 48 h incubation than 24 h incubation time in both mono-and co-culture satellite cells.
Figure 6. The expression of the apoptosis-related proteins (Bcl-2 and BAX) in the mono- and co-culture porcine fibroblast and satellite cells (n = 3). (a) Jeju black pig satellite cells. Here, S-24: monoculture satellite cells 24 h incubation; S-48: monoculture satellite cells 48 h incubation; F/S-24: co-culture satellite cells 24 h incubation; and F/S-48: co-culture satellite cells 48 h incubation. (b) Jeju black pig fibroblast. Here, F-24: monoculture fibroblast cells 24 h incubation; F-48: monoculture fibroblast cells 48 h incubation; S/F-24: co-culture fibroblast cells 24 h incubation; and S/F-48: co-culture fibroblast cells 48 h incubation. (c) Western blot analysis results. a–dBcl-2 and BAX in the columns with different superscript letters differ significantly (p < 0.05) among the groups. Passage number of both cells was 5 in this experiment.
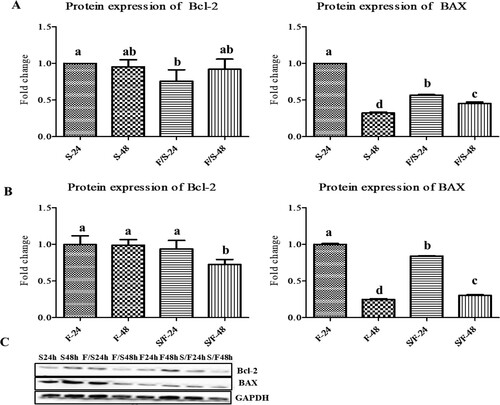
In fibroblast, the protein expression of Bcl-2 was significantly (p < 0.05) decreased at 48 h incubation co-culture than other groups. The protein expression level of MyoD was downregulated in co-culture satellite cells at both 24 and 48 h incubation ((b)). Moreover, the expression of MyoD was significantly (p < 0.05) decreased in 48 h incubation than 24 h incubation time in both mono- and co-culture fibroblast. The bands of western blots of apoptosis-related proteins analysis of pig satellite cells and fibroblast have been presented together ((c)).
Cell–cell interaction regulates cellular morphology
Effects of co-culture on apoptotic morphological changes were examined (). In satellite cells, there were no morphological changes among all the groups ((a)). In fibroblasts, there were also no morphological changes among all the groups ((b)). Hence, the co-culture system did not affect cell morphology.
Figure 7. Morphological features in the mono- and co-culture porcine using AO/EtBr double staining with confocal microscopy (n = 3). (a) Satellite cells. Here, S-24: monoculture satellite cells 24 h incubation; S-48: monoculture satellite cells 48 h incubation; F/S-24: co-culture satellite cells 24 h incubation; and F/S-48: co-culture satellite cells 48 h incubation. (b) fibroblasts. Here, F-24: monoculture fibroblast cells 24 h incubation; F-48: monoculture fibroblast cells 48 h incubation; S/F-24: co-culture fibroblast cells 24 h incubation; and S/F-48: co-culture fibroblast cells 48 h incubation. Passage number of both cells were 6 in this experiment.
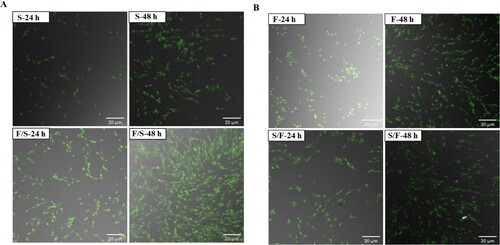
Discussion
Satellite cells have a multipotent character which plays a pivotal role in postnatal muscle growth and muscle regeneration after injury (Seale and Rudnicki Citation2000; Murphy et al. Citation2011). Fibroblasts also play a crucial role in muscle regeneration and fibrosis (Murphy et al. Citation2011). Moreover, fibroblasts also play an important role that produces collagen and superoxide dismutase (SOD) for protecting cells from oxidative stress (Maiorano et al. Citation2007). Both satellite cells and fibroblasts can influence each other for muscle regeneration. A previous study has reported that injured satellite cells cannot repair muscle cells or dysregulated fibroblasts, and vice versa (Murphy et al. Citation2011). However, injured fibroblasts can alter the functions of satellite cells and enhance the differentiation of premature satellite cells (Murphy et al. Citation2011).
Satellite cells as primary stem cells can regenerate the muscle cells. It has been demonstrated that PAX-7 can influence myogenesis by regulating satellite cells’ function (von Maltzahn et al. Citation2013). A previous study has reported that PAX-3 along with PAX-7 can regulate satellite cells proliferation, differentiation, and regeneration. Therefore, these two transcription factors are known as satellite cells markers (Berberoglu et al. Citation2017). Moreover, fibroblasts-synthesized collagen can lead to a notable increase of PAX-7, resulting in better repair and survival of cells (Urciuolo et al. Citation2013). Our results revealed that PAX-7 protein expression was not significantly different among experimental groups. On the other hand, PAX-7 gene expression was notably increased in monoculture satellite cells and fibroblast at 24 h incubation than other groups. PAX-3 gene expression was significantly increased in monoculture satellite cells at 24 h incubation than co-culture fibroblasts at 24 h incubation. The co-culture fibroblast showed significantly higher expression of PAX-7 at 48 h than 24 h incubation. These results suggest that PAX-3 and PAX-7 are markers of satellite cells and that fibroblasts can influence satellite cells.
Generally, MyoD and Myf-5 are highly expressed during the proliferation and differentiation of myoblasts. MyoD is remarkably related to the cell cycle of myoblast. It indicates the opening of cell differentiation (Liu et al. Citation2000). Activated Myf-5 can regulate skeletal myogenesis (Francetic and Li Citation2011). When MyoG as another transcriptional factor is activated, it can influence the cell differentiation process in the mature stage (Hernández-Hernández et al. Citation2017). A previous study has reported that MyoD and Myf-5 can be activated independently. Interestingly, MyoD can act as a substitute for Myf-5 (Rudnicki et al. Citation1993). It has been reported that MyoD, Myf-5, MyoG, and PAX-7 transcriptional factors are remarkably increased in co-culture C2C12 cells than in monoculture C2C12 cells at 48 h after incubation (Muthuraman Citation2014). Results of this study demonstrated that MyoD protein expression was increased in co-culture fibroblasts and satellite cells compared to that in monoculture cells. However, MyoD gene expression was higher at 24 h after incubation in monoculture satellite cells. The Myf-5 gene expression was remarkably upregulated at 24 h in co-culture satellite cells. On the other hand, MyoG gene expression was upregulated in monoculture satellite cells at 48 h. Our results are consistent with those results of Conerly et al. (Citation2016). They reported that MyoD and Myf-5 transcription factors could indicate skeletal muscle cell differentiation (Conerly et al. Citation2016). It has been reported that MyoG can enhance myocyte fusion to maintain muscle homeostasis (Ganassi et al. Citation2018). We will search the mechanism of why muscle cells markers (PAX-7 and MyoD) gene expression significantly increased at 24 h incubation time in porcine monoculture fibroblasts.
The expression of CAV-1 is related to the status of tumour transformation. A previous study has reported that the expression of CAV-1 can indicate the level of cancer and prognosis of patients (Liu et al. Citation2014). Moreover, CAV-1 has been recognized as a marker for the development of tumours (Goetz et al. Citation2008; Quest et al. Citation2008). Our results revealed that CAV-1 protein expression was upregulated in co-culture fibroblasts at 24 h. In addition, CAV-1 gene expression was upregulated at 48 h in co-culture satellite cells. It has been reported that CAV-1 can enhance chemoresistance by preventing the activation of the intrinsic apoptosis pathway (Chatterjee et al. Citation2015). Cells face death due to different stress conditions. Mitochondria can release Cyto-C, a key marker for the initiation of the apoptosis process, into the cytoplasm. Mitochondrial Cyto-C levels are rapidly decreased. However, caspase-3 and caspase-9 are activated, leading to initial apoptosis. Our data indicate that Cyto-C expression is not notably changed in co-culture satellite cells and fibroblasts. However, BAX is pro-apoptotic protein that can promote cell death by inducing Cyto-C (Jürgensmeier et al. Citation1998). In contrast, the BAX and Bcl-2 have absolutely opposite functions. A previous study has reported that Bcl-2 can increase cell number, whereas BAX is responsible for cell death (Gaumer et al. Citation2000). Our data revealed that apoptosis was not notably induced in monoculture and/or co-culture satellite cells and fibroblasts.
Results of this study showed that pig's satellite cells and fibroblasts regulate each other myogenesis and apoptosis-related gene and protein expression. It has been reported that co-cultured satellite cells and fibroblasts show more active and cell cycle activity even in exogenous stress compared to monoculture (Siddiqui et al. Citation2021). Our results revealed that Jeju black pig fibroblasts and satellite cells co-culture increase the myogenesis-related protein and reduce the apoptosis-related proteins. Moreover, these data indicate that the co-culture of fibroblasts and satellite cells does not influence cellular morphology. These data recommended that fibroblasts and satellite cells interaction may be used to muscle differentiation and assist wound healing acceleration of Jeju black pig in in vitro study. Further study needs to observation Jeju black pig muscle growth and development in in vivo study.
Disclosure statement
No potential conflict of interest was reported by the author(s).
Additional information
Funding
References
- Almada AE, Wagers AJ. 2016. Molecular circuitry of stem cell fate in skeletal muscle regeneration, ageing and disease. Nat Rev Mol Cell Biol. 17(5):267–279.
- Baldelli S, Lettieri Barbato D, Tatulli G, Aquilano K, Ciriolo MR. 2014. The role of nNOS and PGC-1α in skeletal muscle cells. J Cell Sci. 127(Pt 22):4813–4820.
- Berberoglu MA, Gallagher TL, Morrow ZT, Talbot JC, Hromowyk KJ, Tenente IM, Langenau DM, Amacher SL. 2017. Satellite-like cells contribute to pax7-dependent skeletal muscle repair in adult zebrafish. Dev Biol. 424(2):162–180.
- Braun T, Gautel M. 2011. Transcriptional mechanisms regulating skeletal muscle differentiation, growth and homeostasis. Nat Rev Mol Cell Biol. 12(6):349–361.
- Buckingham M, Relaix F. 2007. The role of Pax genes in the development of tissues and organs: Pax3 and Pax7 regulate muscle progenitor cell functions. Annu Rev Cell Dev Biol. 23:645–673.
- Chatterjee M, Ben-Josef E, Thomas DG, Morgan MA, Zalupski MM, Khan G, Andrew Robinson C, Griffith KA, Chen CS, Ludwig T, et al. 2015. Caveolin-1 is associated with tumor progression and confers a multi-modality resistance phenotype in pancreatic cancer. Sci Rep. 5:10867.
- Christov C, Chrétien F, Abou-Khalil R, Bassez G, Vallet G, Authier F-J, Bassaglia Y, Shinin V, Tajbakhsh S, Chazaud B, et al. 2007. Muscle satellite cells and endothelial cells: close neighbors and privileged partners. Mol Biol Cell. 18(4):1397–1409.
- Collins CA, Olsen I, Zammit PS, Heslop L, Petrie A, Partridge TA, Morgan JE. 2005. Stem cell function, self-renewal, and behavioral heterogeneity of cells from the adult muscle satellite cell niche. Cell. 122(2):289–301.
- Conerly ML, Yao Z, Zhong JW, Groudine M, Tapscott SJ. 2016. Distinct activities of Myf5 and MyoD indicate separate roles in skeletal muscle lineage specification and differentiation. Dev Cell. 36(4):375–385.
- Cornelison DDW. 2008. Context matters: in vivo and in vitro influences on muscle satellite cell activity. J Cell Biochem. 105(3):663–669.
- Eng D, Ma H-Y, Gross MK, Kioussi C. 2013. Gene networks during skeletal myogenesis. ISRN Dev Biol. 2013:348704.
- Francetic T, Li Q. 2011. Skeletal myogenesis and Myf5 activation. Transcription. 2(3):109–114.
- Ganassi M, Badodi S, Ortuste Quiroga HP, Zammit PS, Hinits Y, Hughes SM. 2018. Myogenin promotes myocyte fusion to balance fibre number and size. Nat Commun. 9(1):4232.
- Gaumer S, Guénal I, Brun S, Théodore L, Mignotte B. 2000. Bcl-2 and Bax mammalian regulators of apoptosis are functional in Drosophila. Cell Death Differ. 7(9):804–814.
- Goetz JG, Lajoie P, Wiseman SM, Nabi IR. 2008. Caveolin-1 in tumor progression: the good, the bad and the ugly. Cancer Metastasis Rev. 27(4):715–735.
- Gross A, Katz SG. 2017. Non-apoptotic functions of BCL-2 family proteins. Cell Death Differ. 24(8):1348–1358.
- Guo B, Greenwood PL, Cafe LM, Zhou G, Zhang W, Dalrymple BP. 2015. Transcriptome analysis of cattle muscle identifies potential markers for skeletal muscle growth rate and major cell types. BMC Genomics. 16(1):177.
- Hardwick JM, Soane L. 2013. Multiple functions of BCL-2 family proteins. Cold Spring Harb Perspect Biol. 5(2):a008722.
- Hasan Siddiqui S, Kang D, Park J, Choi HW, Shim K. 2020. Acute heat stress induces the differential expression of heat shock proteins in different sections of the small intestine of chickens based on exposure duration. Animals. 10(7):1234.
- Hasan Siddiqui S, Sivakumar S, Kang D, Park J, Khan M, Choi H, Shim K. 2020. Direct exposure to mild heat stress stimulates cell viability and heat shock protein expression in primary cultured broiler fibroblasts. Cell Stress Chaperones. 25(6):1033–1043.
- He H, Liu X. 2013. Characterization of transcriptional complexity during longissimus muscle development in bovines using high-throughput sequencing. PLoS ONE. 8(6):e64356–e64356.
- Hernández-Hernández JM, García-González EG, Brun CE, Rudnicki MA. 2017. The myogenic regulatory factors, determinants of muscle development, cell identity and regeneration. Semin Cell Dev Biol. 72:10–18.
- Hill M, Wernig A, Goldspink G. 2003. Muscle satellite (stem) cell activation during local tissue injury and repair. J Anat. 203(1):89–99.
- Jürgensmeier JM, Xie Z, Deveraux Q, Ellerby L, Bredesen D, Reed JC. 1998. Bax directly induces release of cytochrome c from isolated mitochondria. Proc Natl Acad Sci USA. 95(9):4997.
- Kämpfer AAM, Urbán P, Gioria S, Kanase N, Stone V, Kinsner-Ovaskainen A. 2017. Development of an in vitro co-culture model to mimic the human intestine in healthy and diseased state. Toxicol In Vitro. 45(Pt 1):31–43.
- Kuang S, Chargé SB, Seale P, Huh M, Rudnicki MA. 2006. Distinct roles for Pax7 and Pax3 in adult regenerative myogenesis. J Cell Biol. 172(1):103–113.
- Lagha M, Sato T, Bajard L, Daubas P, Esner M, Montarras D, Relaix F, Buckingham M. 2008. Regulation of skeletal muscle stem cell behavior by Pax3 and Pax7. Cold Spring Harb Symp Quant Biol. 73:307–315.
- Lepper C, Conway SJ, Fan C-M. 2009. Adult satellite cells and embryonic muscle progenitors have distinct genetic requirements. Nature. 460(7255):627–631.
- Liu C j, Wang H, Zhao Z, Lu Yu S, Meyer Y B, Chatterjee J, Deschamps G, Roe S, Lengyel B A. 2000. MyoD-dependent induction during myoblast differentiation of p204, a protein also inducible by interferon. Mol Cell Biol. 20(18):7024–7036.
- Liu L, Xu HX, Wang WQ, Wu CT, Chen T, Qin Y, Liu C, Xu J, Long J, Zhang B, et al. 2014. Cavin-1 is essential for the tumor-promoting effect of caveolin-1 and enhances its prognostic potency in pancreatic cancer. Oncogene. 33(21):2728–2736.
- Livak KJ, Schmittgen TD. 2001. Analysis of relative gene expression data using real-time quantitative PCR and the 2(-Delta Delta C(T)) method. Methods. 25(4):402–408.
- Maiorano G, Cavone C, McCormick RJ, Ciarlariello A, Gambacorta M, Manchisi A. 2007. The effect of dietary energy and vitamin E administration on performance and intramuscular collagen properties of lambs. Meat Sci. 76(1):182–188.
- Mendias CL. 2017. Fibroblasts take the centre stage in human skeletal muscle regeneration. J Physiol. 595(15):5005.
- Miki Y, Ono K, Hata S, Suzuki T, Kumamoto H, Sasano H. 2012. The advantages of co-culture over mono cell culture in simulating in vivo environment. J Steroid Biochem Mol Biol. 131(3):68–75.
- Mougeolle A, Poussard S, Decossas M, Lamaze C, Lambert O, Dargelos E. 2015. Oxidative stress induces Caveolin 1 degradation and impairs caveolae functions in skeletal muscle cells. PLoS ONE. 10(3):e0122654.
- Murphy MM, Lawson JA, Mathew SJ, Hutcheson DA, Kardon G. 2011. Satellite cells, connective tissue fibroblasts and their interactions are crucial for muscle regeneration. Development. 138(17):3625–3637.
- Muthuraman P. 2014. Effect of coculturing on the myogenic and adipogenic marker gene expression. Appl Biochem Biotechnol. 173(2):571–578.
- Ow YP, Green DR, Hao Z, Mak TW. 2008. Cytochrome c: functions beyond respiration. Nat Rev Mol Cell Biol. 9(7):532–542.
- Park J, Lee J, Song K-D, Kim S-J, Kim DC, Lee SC, Son YJ, Choi HW, Shim K. 2021. Growth factors improve the proliferation of Jeju black pig muscle cells by regulating myogenic differentiation 1 and growth-related genes. Anim Biosci. 34(8):1392–1402.
- Parsonage G, Filer AD, Haworth O, Nash GB, Rainger GE, Salmon M, Buckley CD. 2005. A stromal address code defined by fibroblasts. Trends Immunol. 26(3):150–156.
- Philippeos C, Telerman SB, Oulès B, Pisco AO, Shaw TJ, Elgueta R, Lombardi G, Driskell RR, Soldin M, Lynch MD, et al. 2018. Spatial and single-cell transcriptional profiling identifies functionally distinct human dermal fibroblast subpopulations. J Invest Dermatol. 138(4):811–825.
- Quest AF, Gutierrez-Pajares JL, Torres VA. 2008. Caveolin-1: an ambiguous partner in cell signalling and cancer. J Cell Mol Med. 12(4):1130–1150.
- Relaix F, Montarras D, Zaffran S, Gayraud-Morel B, Rocancourt D, Tajbakhsh S, Mansouri A, Cumano A, Buckingham M. 2006. Pax3 and Pax7 have distinct and overlapping functions in adult muscle progenitor cells. J Cell Biol. 172(1):91–102.
- Relaix F, Rocancourt D, Mansouri A, Buckingham M. 2004. Divergent functions of murine Pax3 and Pax7 in limb muscle development. Genes Dev. 18(9):1088–1105.
- Remels AH, Langen RC, Schrauwen P, Schaart G, Schols AM, Gosker HR. 2010. Regulation of mitochondrial biogenesis during myogenesis. Mol Cell Endocrinol. 315(1-2):113–120.
- Rudnicki MA, Schnegelsberg PN, Stead RH, Braun T, Arnold HH, Jaenisch R. 1993. MyoD or Myf-5 is required for the formation of skeletal muscle. Cell. 75(7):1351–1359.
- Seale P, Ishibashi J, Scimè A, Rudnicki MA. 2004. Pax7 is necessary and sufficient for the myogenic specification of CD45+:Sca1 + stem cells from injured muscle. PLoS Biol. 2(5):E130–E130.
- Seale P, Rudnicki MA. 2000. A new look at the origin, function, and “stem-cell” status of muscle satellite cells. Dev Biol. 218(2):115–124.
- Seale P, Sabourin LA, Girgis-Gabardo A, Mansouri A, Gruss P, Rudnicki MA. 2000. Pax7 is required for the specification of myogenic satellite cells. Cell. 102(6):777–786.
- Siddiqui SH, Kang D, Park J, Khan M, Shim K. 2020. Chronic heat stress regulates the relation between heat shock protein and immunity in broiler small intestine. Sci Rep. 10(1):18872.
- Siddiqui SH, Park J, Kang D, Khan M, Shim K. 2021. Cortisol differentially affects the viability and myogenesis of mono- and co-cultured porcine gluteal muscles satellite cells and fibroblasts. Tissue and Cell. 73:101615.
- Siddiqui SH, Subramaniyan SA, Kang D, Park J, Khan M, Shim K. 2020. Modulatory effect of heat stress on viability of primary cultured chicken satellite cells and expression of heat shock proteins ex vivo. Anim Biotechnol. 32(6):774–785.
- Spatz MA. 2004. Genetics home reference. J Med Libr Assoc. 92(2):282–283.
- Tidball JG, Villalta SA. 2010. Regulatory interactions between muscle and the immune system during muscle regeneration. Am J Physiol Regul Integr Comp Physiol. 298(5):R1173–R1187.
- Tomasek JJ, Gabbiani G, Hinz B, Chaponnier C, Brown RA. 2002. Myofibroblasts and mechano-regulation of connective tissue remodelling. Nat Rev Mol Cell Biol. 3(5):349–363.
- Urciuolo A, Quarta M, Morbidoni V, Gattazzo F, Molon S, Grumati P, Montemurro F, Tedesco FS, Blaauw B, Cossu G, et al. 2013. Collagen VI regulates satellite cell self-renewal and muscle regeneration. Nat Commun. 4:1964.
- Volonte D, Liu Y, Galbiati F. 2005. The modulation of caveolin-1 expression controls satellite cell activation during muscle repair. FASEB J. 19(2):237–239.
- von Maltzahn J, Jones AE, Parks RJ, Rudnicki MA. 2013. Pax7 is critical for the normal function of satellite cells in adult skeletal muscle. Proc Natl Acad Sci USA. 110(41):16474.
- Westphal D, Dewson G, Czabotar PE, Kluck RM. 2011. Molecular biology of Bax and Bak activation and action. Biochim Biophys Acta (BBA) Mol Cell Res. 1813(4):521–531.
- Yeo S-Y, Lee K-W, Shin D, An S, Cho K-H, Kim S-H. 2018. A positive feedback loop bi-stably activates fibroblasts. Nat Commun. 9(1):3016.
- Young AP, Wagers AJ. 2010. Pax3 induces differentiation of juvenile skeletal muscle stem cells without transcriptional upregulation of canonical myogenic regulatory factors. J Cell Sci. 123(15):2632.
- Zhang W, Tian Y, Gao Q, Li X, Li Y, Zhang J, Yao C, Wang Y, Wang H, Zhao Y, et al. 2020. Inhibition of apoptosis reduces diploidization of haploid mouse embryonic stem cells during differentiation. Stem Cell Rep. 15(1):185–197.