ABSTRACT
The aim of this study was to evaluate the growth performance and plasma antioxidant activity of meat goats fed diets containing mangosteen peel powder (MPP; 41.25 g/kg dry matter). Twelve goats (body weight, 23.1 ± 1.49 kg) were fed a total mixed ration for 0–38 days under a severe temperature–humidity index (temperature, 80.96–103.64°F; humidity, 63%). Body weight and samples of feed, rumen fluid, blood, faeces, and urine were analysed. The MPP diet included condensed tannins, flavonoids, and cinnamic acid. Compared to the control group (CTL; no MPP), dietary MPP increased the nutrient digestibility of organic matter and body weight percentage of goats. Total volatile fatty acids, with a higher proportion of acetic acid, were higher in the MPP group; however, pH and NH3-N remained unchanged for both groups. Methane production in the rumen was reduced in the MPP group. Dietary MPP partly improved biochemical indicators such as plasma total protein (PTP) and albumin (ALB), and modulated antioxidant activity by increasing the glutathione peroxidase concentration and 2,2-diphenyl-1-picrylhydrazyl scavenging activity in plasma. These findings indicate that feeding meat goats the proposed MPP diet represents an environmentally friendly strategy for improving growth performance and plasma antioxidant activity under a severe temperature–humidity index.
1. Introduction
Oxidative stress (OS) is a negative effect that occurs when reactive oxygen species (ROS) accumulate to levels that exceed the cellular antioxidant and neutralizing capacity (Samadieh et al. Citation2017). Therefore, OS might lead to reduced animal productivity and immune function (Yuan et al. Citation2007). In tropical countries, the high temperature–humidity index is a major contributor to an increase in ROS, which can lead to OS (Suong, Paengkoum, Schonewille et al. Citation2022). In general, OS can be treated by improving antioxidant enzyme activity via natural or synthetic antioxidant supplements in animal diets; this can reduce the activity of ROS and prevent its conversion to harmful forms (Schogor et al. Citation2013). That is, the superoxide radical is initially reduced by superoxide dismutase (SOD) to hydrogen peroxide (H2O2) and oxygen (O2), then reduced to water and alcohol (Jafari et al. Citation2021).
In recent decades, researchers have investigated antioxidant supplements containing plant secondary metabolites, such as vitamin C or E, phenolics, flavonoids, condensed tannins, and other polyphenols, for their many biological and health-related properties, and revealed their beneficial effects on alleviating OS (Schogor et al. Citation2013; Jafari et al. Citation2021; Peng et al. Citation2022). These phenolic, flavonoid, and condensed tannins can be degraded in the rumen by stimulating the protein, fibre, and fat components of feeds, as well as, by interacting with the rumen microbiome (Hassan et al. Citation2020b; Purba et al. Citation2020d). Enriched antioxidants in the rumen are then transferred to intermediate metabolites (Purba et al. Citation2022). At appropriate supplementation levels, these plant secondary metabolites have antioxidant, antibacterial, protective protein, and enteric rumen methane mitigation functions, resulting in enhanced animal performance as well as positive effects on the environment (Vasta et al. Citation2019; Purba et al. Citation2020a; Hassan et al. Citation2020b). Nevertheless, the supplementation of different plant species and forms, as well as the use of different methods of extraction, purification, and quantification, can lead to highly distinct biological roles (e.g. antioxidant activity, animal nutrition, or production) due to the complex chemical matrices of plant secondary metabolites. For example, Peng et al. (Citation2016) reported that the dietary supplementation of condensed tannins from purple prairie clover in total mixed rations modulated the nutrient digestibility, blood metabolites, ruminal fermentation, and growth performance of lambs. Tian et al. (Citation2018) included anthocyanin from the flavonoid group of purple corn (Zea mays L.) in dairy goat diets, which led to increased 2,2-diphenyl-1-picrylhydrazyl scavenging activity in response to greater antioxidant activity. However, Purba et al. (Citation2020d) reported that combining condensed tannins and their synergistic compounds of flavonoids, essential oils, and cinnamic acids obtained from Piper betle L. leaves further increased their individual multifunctional biological properties, with flavonoids becoming a major molecule in the selection of target sites through their hydrophobic interactions. Consequently, dietary antioxidant supplements enriched with plant secondary metabolites from other plant species and forms such as roots, fruits, and skin should also be considered.
Mangosteen (Garcinia mangostana L.) is a tropical fruit native to Southeast Asian countries that has become a major agricultural product with high commercial value (Aizat et al. Citation2019). In recent years, the whole fruit and fruit peel of mangosteen have garnered particular interest, exhibiting several benefits for treating human health issues (Kaur et al. Citation2020). Some of the important constituents of mangosteen peel include phenolics, condensed tannins, flavonoids, and cinnamic acids (Zadernowski et al. Citation2009; Ghasemzadeh et al. Citation2018). Notably, these compounds are responsible for antioxidant activity (Purba et al. Citation2021a). Furthermore, the functional properties of mangosteen peel as rumen modifier supplements have also been explored in livestock. For example, mangosteen peel can modulate rumen fermentation and reduce methane emissions with no negative effects in swamp buffaloes (Wanapat et al. Citation2014) and dairy steers (Foiklang et al. Citation2016); therefore, it has a positive impact on the environment. However, to the best of our knowledge, there have been no reports on the antioxidant activity of mangosteen peel in small ruminants. In order to fill this gap, we hypothesize that mangosteen peel powder (MPP) will increase the plasma antioxidant activity of meat goats without negatively affecting growth performance or rumen fermentation. Therefore, the objective of this study was to evaluate the growth performance and plasma antioxidant activity of meat goats fed diets containing different levels of MPP under a severe temperature–humidity index.
2. Materials and methods
2.1. Animals, treatment, and experimental design
The National Research Council of Thailand (U1-02632-2559) and the Animal Ethics Committee of the Suranaree University of Technology (SUT 4/2558) approved all experimental procedures. The experiment began on 29 March 2020 and ended on 12 May 2020. Twelve crossbred Thai-native × Anglo-Nubian male goats with an initial average body weight of 23.10 kg (SD 1.49) were obtained from the university farm of Suranaree University of Technology, Thailand, and were allocated to two experimental rations (n = 6) in a randomized complete block design in two repeated periods. The period was 19 days, with five days in the last week, which was set as the time of sample collection. The goats received isonitrogenous and isocaloric experimental rations, that is, a total mixed ration consisting of either 0% MPP (CTL) or 4.125% MPP (). MPP was purchased as a commercial product (Wallawit-herb company, Bangkok, Thailand).
Table 1 . Ingredient and chemical composition of experimental diets (g/kg dry matter; unless otherwise stated).
The experiment was preceded by a seven-day acclimatization period, during which the animals adapted to the selected tropical conditions, i.e. a severe temperature–humidity index (temperature, 80.96–103.64°F; humidity, 63%). An infrared thermometer (Testo 835H1, Testo SE & Co. KGaA, Bangkok, Thailand) was used to measure the temperature and humidity twice daily at 07:00 and 20:00. Goats were then trained in individual metabolic cages (length 2.2 m × width 1.3 m × height 2 m) with a manual feeder and waterer for the 14-day-adaptation period, enabling goats to adapt to the housing set before starting the experiment. The experimental rations were offered ad libitum, twice daily at 09:00 and 17:00, with free access to fresh drinking water. The daily feed intake was calculated by recording feed refusals every 24 h. Each animal was weighed daily before morning feeding during the adaptation period and feeding trial.
2.2. Feed, faeces, and urine samples and analysis
Feed, faeces, and urine were sampled on the five days following the feeding trial in every period. Daily samples of feed and faeces were prepared in dried form (passed through a 1-mm screen) to determine the chemical composition, including dry matter (DM), organic matter (OM), gross energy (GE), crude protein (CP), ether extract (EE), acid detergent fibre (ADF), and neutral detergent fibre (NDF), as described in previous reports (Vorlaphim et al. Citation2021; Paengkoum et al. Citation2021b). The concentrations of condensed tannins, flavonoids, and cinnamic acid in the feed samples were determined by means of liquid chromatography (HPLC system, Agilent Technologies 1260 Infinity, Santa Clara, CA, U.S.A.), as described by Purba et al. (Citation2021a), with a standard calibration provided by Purba and Paengkoum (Citation2019). Daily urine samples were collected by preparing plastic containers. Detailed descriptions of sample collection and nitrogen concentration in urine can be found in a previous study (Paengkoum et al. Citation2017). All analyses were performed in quadruplicate for each treatment in every period.
2.3. Rumen fluid samples and analysis
On the last day of the feeding trial at every period, rumen fluids (∼500 mL) at 0 h (before feeding) and 4 h post-feeding were gathered from the goats using a stomach tube connected to a manual pump following an earlier protocol reported in (Purba et al. Citation2021b). The pH of the collected rumen fluids was checked and subsequently strained through four layers of cheesecloth. The filtered rumen fluids were then divided into three parts. The first part (100 mL) was fixed with 10 mL of a 50% H2SO4 solution and centrifuged at 16,000×g for 15 min to collect the supernatant. The supernatant was then used to analyse the concentration of volatile fatty acids (VFA) via gas chromatography (Agilent 6890 GC, Agilent Technologies, Wilmington, DE, U.S.A.) with a 30 m × 0.25 mm × 0.25 µm column (DB-FFAP), in quadruplicate. Standard calibration, peak detection, and calculation followed the method of Purba et al. (Citation2020d). The second part (100 mL) was centrifuged at 6000×g for 15 min at 4°C, and the supernatant was subsequently measured for ruminal ammonia nitrogen (NH3-N) using the micro-Kjeldahl method [Kjeltec 8100, Hillerød, Denmark, AOAC (Citation2005)], in quadruplicate.
The remaining part was immediately transported to the laboratory to determine methane production using in vitro gas production (Menke and Steingass Citation1988). All incubations were completed in 10 replicates. Methane production at 0, 2, 4, 6, 8, 10, 12, and 24 h post-incubation was measured by a gas chromatographer (Agilent 7890A, Agilent Technologies) following the methods described in an earlier study (Purba et al. Citation2021b). Internal standard calibration, peak detection, and calculation were provided by Purba et al. (Citation2020b). To calculate the residual dry matter, the content of the incubated substrate was individually filtered and collected through pre-weighed Gooch crucibles (Tilley and Terry Citation1963).
2.4. Blood samples and analysis
After collecting rumen fluids, blood samples (10 mL) were collected before the morning feeding (0 h) and 4 h post-feeding from the jugular vein into evacuated tubes containing lithium heparin. A volume of blood (4 mL) was immediately transferred to the Suranaree University of Technology (SUT) hospital to detect plasma biochemical parameters (plasma total protein, PTP; albumin, ALB; glucose, GLU; blood urea nitrogen, BUN). The remaining volume of blood samples was centrifuged at 3000×g for 15 min at 4°C (Sorvall Legend XT/XF Centrifuge Series, Thermo Fisher Scientific, Waltham, MA). Plasma was stored at −80°C to determine the plasma antioxidant activity.
The plasma 2,2-diphenyl-1-picrylhydrazyl (DPPH) scavenging capacity was analysed according to the method of (Tian et al. Citation2019) with a minor modification. In brief, 100 μL of the sample was mixed with 1 mL of DPPH reagent (50 μmol/L, PCode: 101845869, Sigma-Aldrich, Germany) into a 2-mL centrifugal tube, vortexed for 15 s, then stored in a dark room at ambient temperature (27°C) for 35 min and centrifuged at 3000×g for 15 min at 4°C. Then, 200 µL of supernatant was pipetted into a 96-well plate, and the absorbance was determined at 517 nm using a microplate reader. Glutathione peroxidase (GPx), SOD, and total antioxidant capacity (TAC) were detected using commercial kits (Sigma-Aldrich, U.S.A.) in quadruplicate.
2.5. Calculation and statistical analysis
The apparent digestibility was calculated as follows: [Nutrient intake (g/day) − faecal excretion of corresponding nutrient (g/day)]/nutrient intake (g/day) × 100%.
All data (e.g. growth performance including nutrient intake and apparent digestibility, nitrogen balance, rumen fermentation end-products, plasma biochemical indicators, and plasma antioxidant activity) were statistically analysed as a randomized complete block design following the general linear model procedure of the SAS 9.4 (SAS Institute Inc Citation2015). All data were normalized using the Shapiro–Wilk test. Data related to the initial weight of goats and blood parameters at 0 h post-feeding were used as covariates. All data were analysed using Student’s t-test. The least-squares means were reported, and significance was declared at P < 0.05.
All data related to methane production at 0, 2, 4, 6, 8, 10, 12, and 24 h post-incubation were processed as a completely randomized design with repeated measures using the MIXED procedure of SAS 9.4 (SAS Institute Inc Citation2015). Akaike’s information criterion of the mixed model of SAS was used to check the compound symmetry of the covariance structure. The Kolmogorov–Smirnov test was used to check whether data were normally distributed. The statistical significance of the ration effect was tested against the variance of the gas syringe nested within the ration according to the repeated measures design. The least-square means were reported, and significance was declared at P < 0.05. Differences among the mean values of diet and incubation time were calculated by Tukey’s HSD with a probability level of P < 0.05 and 0.05 ≤ P < 0.10.
3. Results
3.1. Mangosteen peel powder increases the nutrient digestibility of organic matter and body weight percentage of meat goats
All goats remained healthy and showed no polydipsia throughout the experiment. The nutrient intake, apparent digestibility, and body weight percentage (%BW) of meat goats are shown in . Dietary supplementation with MPP did not affect the nutrient intake or apparent digestibility, except for the apparent digestibility of OM, which increased after goats were fed the MPP diet (+0.55%; P < 0.05). Compared to CTL, dietary MPP increased the %BW of meat goats (P = 0.005). All goats either maintained or gained weight during the experiment, indicating that all experimental diets (isonitrogenous and isocaloric rations) supplied the nutritional requirements for growing goats.
Table 2. Effect of mangosteen peel powder on nutrient intake, apparent digestibility, and body weight percentage of meat goats.
3.2. Mangosteen peel powder reduces nitrogen excretion in the urine of meat goats
The total nitrogen intake, nitrogen balance, and nitrogen excretion in the faeces and urine of meat goats are shown in . Dietary supplementation with MPP did not affect nitrogen intake or nitrogen excretion in faeces and urine of meat goats.
Table 3. Effect of mangosteen peel powder on total nitrogen intake, nitrogen balance, and nitrogen excretion in the faeces and urine of meat goats.
3.3. Mangosteen peel powder modulates rumen fermentation end-products by maintaining the rumen pH of meat goats
The pH and concentration of rumen fermentation end-products, including VFAs and NH3-N in the rumen of meat goats, are depicted in . Dietary supplementation with MPP did not affect the rumen pH; the range of supplementation-maintained pH was 6.92–7.07. Dietary supplementation with MPP increased the VFA concentration (up to +17%; P = 0.005) compared to dietary supplementation with no MPP (CTL). Compared to CTL, a significant difference was noted only in an increased concentration of acetic acid (up to +29%; P < 0.001), with the concentrations of propionic acid and butyric acid remaining unchanged after the meat goats received the MPP diet. Moreover, dietary supplementation with MPP did not affect the concentration of NH3-N in rumen, which maintained a range of 15.24–16.62 mg/dL (P = 0.37; ).
Figure 1. Levels of pH and rumen fermentation end-products including volatile fatty acids (VFAs) and NH3-N in the rumen of meat goats fed CTL and MPP diets. Values are the means, with standard errors represented by vertical bars. Significantly different values are indicated as follows: ***P < 0.001; **P < 0.01; ns, P > 0.05.
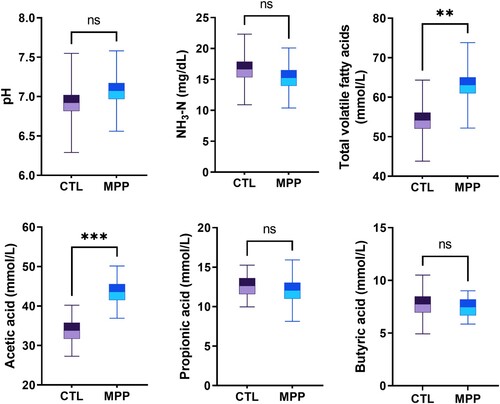
3.4. Mangosteen peel powder increases ruminal methane mitigation
Ruminal methane mitigation was increased after dietary supplementation with MPP (P < 0.02), with a drop 24 h post-incubation after similar methane production from 2 to 12 h of incubation time for both CTL and MPP groups (). No interaction (incubation time × diet) was observed after any duration of incubation (P > 0.11).
Figure 2. In vitro ruminal methane production observed at 0, 2, 4, 6, 8, 10, 12, and 24 h post-incubation of rumen fluids for meat goats fed CTL and MPP diets. Values are the means, with standard errors represented by vertical bars. *P < 0.05 was significant for diet; ns, P > 0.05 was not significant for incubation time or interaction (incubation time × diet).
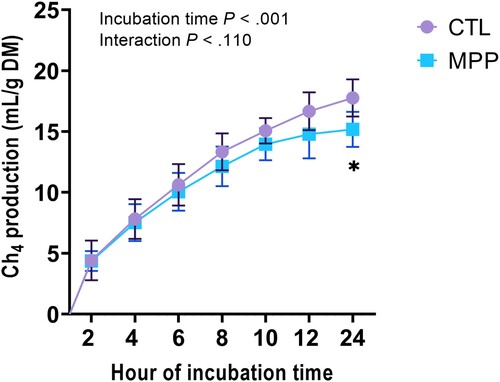
3.5. Mangosteen peel powder improves biochemical indicators and antioxidant activity in the plasma of meat goats
The biochemical indicators and antioxidant activity in the plasma of meat goats are presented in . Dietary supplementation with MPP did not affect the plasma biochemical indicators, including GLU, BUN, or globulin (GLB), except for PTP and ALB (P < 0.01). However, dietary supplementation with MPP increased antioxidant activity in the plasma of meat goats (P < 0.05; ). Compared to CTL, a significant difference was noted only in an increased concentration of DPPH scavenging activity (P < 0.05) and GPx (P < 0.05). A lack of TAC and SOD in the plasma was observed in both CTL and MPP groups, with TAC and SOD ranging from 43.70–44.15 nmol/μL and 54.89–56.91%, respectively ().
Table 4. Effect of mangosteen peel powder on biochemical indicators and antioxidant activity in the plasma of meat goats.
4. Discussion
To the best of our knowledge, this is the first study to assess the effectiveness of incorporating MPP into small ruminant diets to modulate growth performance and plasma antioxidant activity under tropical conditions. Previous studies (Poungchompu et al. Citation2009; Wanapat et al. Citation2014; Foiklang et al. Citation2016; Paengkoum et al. Citation2021a) have used MPP containing only condensed tannins and/or saponin in cattle diets; thus, the effects observed in these studies cannot be attributed to condensed tannins and/or saponin alone due to the synergistic and antagonistic effects from complex matrices such as flavonoids; moreover, other cinnamic acids of MPP have also been reported (Zadernowski et al. Citation2009; Ghasemzadeh et al. Citation2018). Previous reports have investigated synergistic compounds in animal nutrition and physiology (Hassan et al. Citation2020a; Purba et al. Citation2020b, Citation2020d); however, none these compounds were derived from MPP.
Feed characteristics after modification by plant secondary metabolites may be attributed to animal feed intake and animal performance by considering the complexity of chemical compositions therein; therefore, feed intake is one of the most important limiting factors in ruminants (Yang et al. Citation2021). Although MPP consisting of flavonoids and cinnamic acids is capable of providing a pleasant flavour, the condensed tannin content could make this experimental diet bitter and tangy; therefore, this supplementation strategy could result in a slightly low feed intake in meat goats. In the present study, the DM and OM intakes of meat goats were not influenced by a daily total mixed ration containing ∼ 41.25 g of condensed tannins, flavonoids, and cinnamic acid. Thus, the data reported here and in previous reports (Wanapat et al. Citation2014; Foiklang et al. Citation2016; Purba et al. Citation2020d) suggest that the inclusion of MPP did not have a negative effect on the palatability of the experimental diet.
No change was observed in nutrient digestibility parameters in this study, except for OM digestibility. The non-significant effect of MPP on DM, CP, NDF, ADF, and GE agrees with the results of earlier studies (Wanapat et al. Citation2014; Foiklang et al. Citation2016) on cattle fed with MPP (30–100 g/head/day). These studies indicate that a suitable condensed tannin content in the MPP (<50 g/kg DM) is safe for use as a supplement. However, previous experiments supplementing soapberry fruit-mangosteen peel in cow diets under several roughage-to-concentrate ratios suggested that condensed tannins and other oligomer flavonoids could increase the digestibility of DM, CP, and NDF (Poungchompu et al. Citation2009). Moreover, oligomer flavonoids and phenolics present in MPP diets of current study, such as cinnamic acid, have been shown to increase the digestibility of OM. An increase in the digestibility of OM by MPP addition agrees with the results of earlier studies that reported enhanced dietary flavonoids and/or phenolics in small ruminant diets in response to greater stimulation by quercetin (a major regulator in complex plant polyphenols; Berger et al. Citation2015; Purba et al. Citation2020d) on ruminal microorganisms, which increased OM fermentation in the substrate containing abundant fibre sources (Purba et al. Citation2020c). Subsequent hydrolysis and conjugation of enzymes in the small intestine might be influenced by the modulation of a higher digestion rate in the rumen by flavonoids (Suong, Paengkoum, Salem et al. Citation2022). Therefore, the findings of this study revealed that treatment with MPP rich in condensed tannins, flavonoids, and cinnamic acid showed no adverse effects on nutrient intake. As such, MPP could positively affect nutrient digestibility and the activity of synthesizing enzymes in meat goats. The improved OM fermentation may be attributed to the large amount of acetic, propionic, and butyric fractions. In addition, the increased body weight percentage in meat goats receiving an MPP diet could be attributed to higher nutrient digestion or absorption, as well as lower nutrient turnover.
As expected, the higher OM digestion rate observed in this study resulted in a higher total VFA, with a wide range of acetic acid production. Wanapat et al. (Citation2014) reported that MPP supplementation of 100 g/head/day in cattle did not affect the concentration of total VFAs, acetate, or butyrate, which is in contrast to the findings of this study. However, Foiklang et al. (Citation2016) found that a lower concentration of MPP (30 g/head/day in cattle) increased the concentration of total VFAs and reduced the concentration of acetate. These contradictions may be due to the different ability of MPP secondary metabolites to modulate VFA-producing bacteria, especially cellulose and hemicellulose bacteria, which are responsible for the production of VFAs by soluble sugars (Hassan et al. Citation2020b; Purba et al. Citation2020d). In this study, we acknowledge that MPP not only contains condensed tannins and/or saponin, but also oligomer flavonoids and some other cinnamic acids. The data reported here confirm that the notable effects of oligomeric flavonoids and some other cinnamic acids could increase the abundance of VFA-producing bacteria, such as F. succinogenes, R. albus, and B. fibrisolvens (Ma et al. Citation2017; Purba et al. Citation2020d). The increased proportion of acetic acid observed in this study was similar to that reported in a previous study (Yulistiani et al. Citation2015), suggesting the enhanced digestion of structural carbohydrates. However, the NDF and ADF digestibility were similar for CTL and MPP groups in this study. The most likely reason is the ability of the rumen to degrade oligomeric flavonoids and other cinnamic acids through hydrolysis of their glycosides and cleavage of heterocyclic compounds, finally yielding monohydroxyphenolics, phloroglucinol, and acetic acid (McSweeney et al. Citation2001; Purba et al. Citation2020c). Consequently, the increase in acetic acid was caused by the MPP, which did not affect propionic acid or butyric acid concentrations. Notably, a previous study on short-term elevated ambient temperature for the characterization of ruminal VFA dynamics showed shifts in rumen VFA fluxes; the total VFAs were unaffected, but the molar proportions were affected by the thermal environment effect (Bedford et al. Citation2020). Therefore, our findings could corroborate other possible factors, that is, the shifts in rumen VFA fluxes of small ruminants might be related to a higher ambient temperature or climatic conditions, which can be remedied by the supplementation strategy proposed in this study.
Consistent with earlier studies, we observed no change in the ruminal pH of meat goats fed MPP (Wanapat et al. Citation2014; Foiklang et al. Citation2016), with ruminal pH values within the normal range (6.92–7.07). These findings indicate that the microbial rumen seems to have high adaptation ability to the proposed treatment, regardless of the additions or differences in the composition of plant secondary compounds from dietary MPP. However, previous studies have reported conflicting results on the effect of MPP on rumen pH. For example, Poungchompu et al. (Citation2009) reported that the presence of saponin and condensed tannins in MPP with a roughage proportion of 30% of the total diet composition decreased the ruminal pH, whereas Foiklang et al. (Citation2016); Wanapat et al. (Citation2014) reported no change of ruminal pH after using MPP with the full availability of roughage (ad libitium). Notably, saponin can shift the pH in the rumen between concentrate- and roughage-based diets (Patra and Saxena Citation2009). Here, we prepared the experimental diets with at least 67% roughage; however, this result might be attributed to the presence of oligomeric flavonoids. Flavonoids are known to be beneficial during periods of animal stress; they also balance rumen pH in subacute acidosis (Olagaray and Bradford Citation2019; Ku-Vera et al. Citation2020). Owing to the structural similarities of flavonoids in the presence of tannins, cinnamic acid, and other phenolics, they exhibit a substantial balancing effect on rumen pH, which is in agreement with previous reports (Purba et al. Citation2020b, Citation2020c, Citation2020d).
Given this impact, methane mitigation frequently affects the performance of ruminants fed plant secondary metabolites (Ku-Vera et al. Citation2020). In this study, plant secondary metabolites present in the MPP decreased ruminal in vitro methane production, which is similar to previous reports (Poungchompu et al. Citation2009; Wanapat et al. Citation2014). The fermentation of enzymatically structural carbohydrates, starch, and proteins in the rumen for producing metabolic H2, CO2, and VFAs is complex, and these fermentation end-products are further used by rumen methanogens for CH4 synthesis during methanogenesis (Ku-Vera et al. Citation2020). Specifically, more VFAs produced during fermentation yield more CH4. However, MPP negatively affects methanogenesis by inhibiting H2-releasing reactions or removing H2 during carbohydrate fermentation. The observed increase in the proportion of acetic acid, with no change in propionic or butyric acid, agreed with earlier findings (Purba et al. Citation2020b, Citation2020c), indicating that acetic acid production exhibits higher hydrogen consumption. Previously, a weak relationship has been observed between propionic acid and methanogenesis in shifted H2 uptake pathways in ruminant studies based on in vivo and in vitro experiments (Jayanegara et al. Citation2012). Thus, we assumed other possible reasons affecting the decrease in CH4 synthesis. The most likely reason was reported by Greening et al. (Citation2019), who suggested that H2 metabolism is a more complex and widespread trait among rumen microorganisms, which results in various H2 uptake pathways during carbohydrate fermentation. Nitrite reduction (Selenomonas spp.) and acetogenesis (Blautia spp.) are superior to rumen methanogens. Moreover, the increase of plant secondary metabolite supplementation in diets is associated with a decrease in nucleic acid synthesis in protozoa (Newbold et al. Citation2015). Protozoa employ starch, cellulose, hemicellulose, pectin, and soluble sugars to produce VFAs (Ku-Vera et al. Citation2020). On the other hand, quercetin, as the flavonoid content of MPP, can maintain the characteristics of cellulolytic-hemicellulolytic bacteria, with reduced protozoa and methanogen populations (Purba et al. Citation2020b, Citation2020c). MPP may exhibit polyphenol-mediated modulation of gut microbiota to influence methane-suppressing properties. However, it is not clear if these plant secondary metabolites from MPP could directly stimulate Blautia spp.; therefore, further studies are required to better explain the specific bacteria, including Blautia spp., involved in the H2 uptake pathways.
No change in the protein digestibility parameter was observed in this study, which could be attributed to the lack of influence of MPP on ammonia adsorption and modulation of the passage of digesta in the rumen. The non-significant effect of MPP on NH3-N concentration, urinary N, faecal N, and total N excretion, as well as the constant level of retained N as a proportion of N digested, and the constant BUN as a proportion of N distributed in the blood, are all in agreement with previous studies (Poungchompu et al. Citation2009; Wanapat et al. Citation2014) in cattle fed MPP (100 g/head/day). However, Suchitra and Wanapat (Citation2008) reported that feeding lactating dairy cows with MPP at 200 g/head/day decreased protein digestibility and reduced the NH3-N concentration; however, it did not affect BUN concentration. Plant secondary metabolites play a role in protein digestibility, commonly exhibiting a substantial effect on reducing the availability of feed protein for ruminal degradation and ammonia nitrogen release (Makkar Citation2003). A diet-dependent response of bacterial protein synthesis to plant secondary metabolites, including its excretion in faeces or urine, was clearly observed (Patra and Saxena Citation2010). Their data confirmed the observation that lower levels of dietary MPP at 41.25 g/head/day do not modulate protein synthesis; therefore, this amount of MPP supplementation could not achieve the environmental benefits of reducing N excretion either in faeces or urine, as observed in the present study.
Blood biochemical indicators are important parameters for predicting the health status of animals, and dietary nutrient absorption and metabolism can define the characteristics of blood biochemical indicators (Zaitsev et al. Citation2021). For example, GLU and BUN levels are important indicators of energy and nitrogen metabolism in ruminants (Graugnard et al. Citation2012). In this study, however, the lack of change in the apparent digestibility of protein and gross energy was attributed to not only unchanged BUN levels, but also unchanged GLU levels as a result of dietary MPP. The BUN level ranged from 16.27–16.47 mg/dL and the GLU level ranged from 53.63–55.79 mg/dL, which are similar values to those reported in a previous study (Abarghuei et al. Citation2014), suggesting that dietary MPP should be considered safe for animals. In addition, the parameters of PTP, ALB, GLB, and the ratio of ALB to GLB in blood are indicators of immune function in ruminants (Zaitsev et al. Citation2021). A high-temperature environment has been shown to decrease GLU and cholesterol levels (Ribeiro et al. Citation2018). Thus, maintaining stable levels of GLU in the blood is a key function of the liver, which is supported by several extrahepatic tissues and important hormones, such as glucagon, cortisol, thyroid, and insulin hormones (Dukes and Reece Citation2006). Despite the abundance of flavonoids and cinnamic acid, which have a greater ability to modulate blood biochemicals (Chedea et al. Citation2017; Tian et al. Citation2018), dietary MPP contains condensed tannins (approximately 0.62% in a combination of catechin and proanthocyanidins) and increased PTP and ALB levels in meat goat blood, as observed in this study. Oni et al. (Citation2012) found that cassava leaves (containing approximately 1% condensed tannins) offered to meat goats could increase PTP, ALB, and GLB levels in blood. However, Zhang et al. (Citation2019) supplemented 3% of two different condensed tannins (Bayberry and Acacia mangium), and found that neither condensed tannin had an effect on PTP, ALB, GLB, or the ratio of ALB to GLB. Therefore, plant secondary metabolites contained in dietary MPP exhibit a beneficial biology that may improve goat immunity, as indicated by changes of biochemical indicators in the blood. However, more data on the diet-dependent response is required for further extrapolation of these results to practical feeding conditions.
Furthermore, the method for quantifying DPPH scavenging activity has been widely used to evaluate the properties of constituents for free radical scavenging (Szerlauth et al. Citation2019), where SOD acts as the first guard of defence against ROS, and GPx is the second (Wang et al. Citation2018). The increased concentration of enzymatic GPx and DPPH scavenging activity observed in this study was corroborated by previous reports (Tian et al. Citation2018; Szerlauth et al. Citation2019), suggesting that natural antioxidants from plant secondary metabolites could possess reactive hydrogen atoms, which act as reductants for OS. However, increased DPPH scavenging activity and GPx were not accompanied by greater SOD and TAC activity, suggesting that the effect of plant secondary metabolites on antioxidant activity depends on the molecular weight or threshold used in the diets. For example, Di Trana et al. (Citation2015) reported that increased TAC and GPx levels, with no change in SOD, were observed in the blood of goats fed Sulla forage (Sulla coronarium L.) containing 1.23 g of nontannic polyphenols and 2 g of condensed tannin. Liu et al. (Citation2013) reported that increased antioxidant activity for SOD, GPx, and TAC was achieved by supplementation of chestnut wood containing 1.8 g of nontannic polyphenols and 1.4 g of condensed tannin in cow diets. Moreover, Tian et al. (Citation2018) reported that feeding dairy goats with purple-corn stover silage containing 8.75 g of nontannic polyphenols could be attributed to greater SOD antioxidant activity with GPx activity and TAC. Hence, feeding meat goats MPP containing 10.36 g of nontannic polyphenols and 6.21 g of condensed tannins has the practical benefit of improving antioxidant activity in meat goats. Taken together, dietary MPP diet could enhance the growth performance of meat goats by improving antioxidant activity (and, therefore, reducing OS) and enhancing nutrient digestion by increasing fermentable OM.
5. Conclusion
According to this study, we conclude that feeding meat goats a diet consisting of 41.25 g/head/day MPP did not adversely affect feed intake or nutrient digestibility. Condensed tannins, flavonoids, and cinnamic acid present in the MPP modulated the fermentable OM, thereby increasing nutrient digestion, total VFA concentrations, and body weight percentages. Concurrently, these plant secondary metabolites improved biochemical indicators and antioxidant activity in the blood, but did not affect the distribution of N in the blood. In addition, feeding meat goats with MPP resulted in a reduction of enteric methane emissions; however, MPP could not reduce NH3-N concentrations, urinary N, faecal N, or total N excretion. Therefore, MPP represents an environmentally friendly strategy for improving growth performance and plasma antioxidant activity under a severe temperature–humidity index.
Acknowledgements
Authors are truly grateful to all staffs of the Centre of Scientific and Technological Equipment and Section of Goat and Sheep SUT farm, Suranaree University of Technology, Siriwan Phetsombat and Thara Wongdee for use of the research facilities. Authors extend the heartfelt thanks to Nittaya Taethaisong for her assistance with animal care, feeding, and sampling. Chao Ban gratefully acknowledges the SUT-OROG scholarship for supporting study and research. Rayudika Aprilia Patindra Purba gratefully acknowledges the Institute of Research and Development grant (Grant# No. Full-time61/02/2021) as a full-time Doctoral Researcher to conduct research in Suranaree University of Technology.
Disclosure statement
No potential conflict of interest was reported by the author(s).
Additional information
Funding
References
- Abarghuei MJ, Rouzbehan Y, Salem AZM, Zamiri MJ. 2014. Nitrogen balance, blood metabolites and milk fatty acid composition of dairy cows fed pomegranate-peel extract. Livest Sci. 164:72–80.
- Aizat WM, Jamil IN, Ahmad-Hashim FH, Noor NM. 2019. Recent updates on metabolite composition and medicinal benefits of mangosteen plant. PeerJ. 7:e6324.
- AOAC. 2005. Official methods of analysis. Gaitherburg: AOAC International Suite 500.
- Bedford A, Beckett L, Harthan L, Wang C, Jiang N, Schramm H, Guan LL, Daniels KM, Hanigan MD, White RR. 2020. Ruminal volatile fatty acid absorption is affected by elevated ambient temperature. Sci Rep. 10 (1):13092.
- Berger LM, Blank R, Zorn F, Wein S, Metges CC, Wolffram S. 2015. Ruminal degradation of quercetin and its influence on fermentation in ruminants. J Dairy Sci. 98(8):5688–5698.
- Chedea VS, Pelmus RS, Lazar C, Pistol GC, Calin LG, Toma SM, Dragomir C, Taranu I. 2017. Effects of a diet containing dried grape pomace on blood metabolites and milk composition of dairy cows. J Sci Food Agric. 97(8):2516–2523.
- Di Trana A, Bonanno A, Cecchini S, Giorgio D, Di Grigoli A, Claps S. 2015. Effects of Sulla forage (Sulla coronarium L.) on the oxidative status and milk polyphenol content in goats. J Dairy Sci. 98(1):37–46. Epub 2014/12/04.
- Dukes HH, Reece WO. 2006. Fisiologia dos animais domésticos. Rio de Janeiro: Guanabara Koogan.
- Foiklang S, Wanapat M, Norrapoke T. 2016. Effect of grape pomace powder, mangosteen peel powder and monensin on nutrient digestibility, rumen fermentation, nitrogen balance and microbial protein synthesis in dairy steers. Asian Australas J Anim Sci. 29(10):1416–1423.
- Ghasemzadeh A, Jaafar HZE, Baghdadi A, Tayebi-Meigooni A. 2018. Alpha-mangostin-rich extracts from mangosteen pericarp: optimization of green extraction protocol and evaluation of biological activity. Molecules. 23:8.
- Graugnard DE, Bionaz M, Trevisi E, Moyes KM, Salak-Johnson JL, Wallace RL, Drackley JK, Bertoni G, Loor JJ. 2012. Blood immunometabolic indices and polymorphonuclear neutrophil function in peripartum dairy cows are altered by level of dietary energy prepartum. J Dairy Sci. 95(4):1749–1758.
- Greening C, Geier R, Wang CJ, Woods LC, Morales SE, McDonald MJ, Rushton-Green R, Morgan XC, Koike S, Leahy SC, et al. 2019. Diverse hydrogen production and consumption pathways influence methane production in ruminants. ISME J. 13:2617–2632.
- Hassan F, Arshad MA, Ebeid HM, Rehman MS, Khan MS, Shahid S, Yang C. 2020a. Phytogenic additives can modulate rumen microbiome to mediate fermentation kinetics and methanogenesis through exploiting diet–microbe interaction. Front Vet Sci. 7:892.
- Hassan F, Arshad MA, Li M, Rehman MS, Loor JJ, Huang J. 2020b. Potential of mulberry leaf biomass and its flavonoids to improve production and health in ruminants: mechanistic insights and prospects. Animals. 10:11.
- Jafari S, Saleh H, Mirakzehi MT. 2021. Performance, immune response, and oxidative status in broiler chicken fed oxidized oil and Otostgia persica leaf extract. Ital J Anim Sci. 20(1):878–886.
- Jayanegara A, Leiber F, Kreuzer M. 2012. Meta-analysis of the relationship between dietary tannin level and methane formation in ruminants from in vivo and in vitro experiments. J Anim Physiol Anim Nutr. 96(3):365–375.
- Kaur G, Singh A, Dar BN. 2020. Mangosteen (Garcinia mangostana L.). In: Nayik Gulzar Ahmad and Gull Amir, Antioxidants in fruits: properties and health benefits. Singapore: Springer Singapore; p. 83–101.
- Ku-Vera JC, Jiménez-Ocampo R, Valencia-Salazar SS, Montoya-Flores MD, Molina-Botero IC, Arango J, Gómez-Bravo CA, Aguilar-Pérez CF, Solorio-Sánchez FJ. 2020. Role of secondary plant metabolites on enteric methane mitigation in ruminants. Front Vet Sci. 7:584.
- Liu HW, Zhou DW, Li K. 2013. Effects of chestnut tannins on performance and antioxidative status of transition dairy cows. J Dairy Sci. 96(9):5901–5907.
- Ma T, Chen DD, Tu Y, Zhang NF, Si BW, Diao QY. 2017. Dietary supplementation with mulberry leaf flavonoids inhibits methanogenesis in sheep. Anim Sci J. 88(1):72–78.
- Makkar HPS. 2003. Effects and fate of tannins in ruminant animals, adaptation to tannins, and strategies to overcome detrimental effects of feeding tannin-rich feeds. Small Ruminant Res. 49(3):241–256.
- McSweeney CS, Palmer B, McNeill DM, Krause DO. 2001. Microbial interactions with tannins: nutritional consequences for ruminants. Anim Feed Sci Tech. 91(1/2):83–93.
- Menke KH, Steingass H. 1988. Estimation of the energetic feed value obtained from chemical analysis and in vitro gas production using rumen fluid. Anim Res Dev. 28:7–55.
- Newbold CJ, de la Fuente G, Belanche A, Ramos-Morales E, McEwan NR. 2015. The role of ciliate protozoa in the rumen. Front Microbiol. 6:61313–61313.
- Olagaray KE, Bradford BJ. 2019. Plant flavonoids to improve productivity of ruminants – a review. Anim Feed Sci Tech. 251:21–36.
- Oni AO, Arigbede OM, Sowande OS, Anele UY, Oni OO, Onwuka CF, Onifade OS, Yusuf KO, Dele PA, Aderinboye RY. 2012. Haematological and serum biochemical parameters of West African Dwarf goats fed dried cassava leaves-based concentrate diets. Trop Anim Health Prod. 44(3):483–490. Epub 2011/07/12.
- Paengkoum P, Thongpea S, Paengkoum S. 2017. Utilization of concentrate supplements containing varying levels of cassava leaf pellet by growing goats fed a basal diet of pangola hay. Indian J Anim Res. 51(6):1091–1096.
- Paengkoum S, Petlum A, Purba RAP, Paengkoum P. 2021a. Protein-binding affinity of various condensed tannin molecular weights from tropical leaf peel. J Appl Pharm Sci. 11(3):114–120.
- Paengkoum S, Tatsapong P, Taethaisong N, Sorasak T, Purba RAP, Paengkoum P. 2021b. Empirical evaluation and prediction of protein requirements for maintenance and growth of 18–24 months old Thai swamp buffaloes. Animals. 11(5):1405.
- Patra AK, Saxena J. 2009. The effect and mode of action of saponins on the microbial populations and fermentation in the rumen and ruminant production. Nutr Res Rev. 22(2):204–219.
- Patra AK, Saxena J. 2010. A new perspective on the use of plant secondary metabolites to inhibit methanogenesis in the rumen. Phytochemistry. 71(11–12):1198–1222.
- Peng K, Lv X, Zhao H, Chen B, Chen X, Huang W. 2022. Antioxidant and intestinal recovery function of condensed tannins in Lateolabrax maculatus responded to in vivo and in vitro oxidative stress. Aquaculture. 547: 737399.
- Peng K, Shirley DC, Xu Z, Huang Q, McAllister TA, Chaves AV, Acharya S, Liu C, Wang S, Wang Y. 2016. Effect of purple prairie clover (Dalea purpurea Vent.) hay and its condensed tannins on growth performance, wool growth, nutrient digestibility, blood metabolites and ruminal fermentation in lambs fed total mixed rations. Anim Feed Sci Tech. 222:100–110.
- Poungchompu O, Wanapat M, Wachirapakorn C, Wanapat S, Cherdthong A. 2009. Manipulation of ruminal fermentation and methane production by dietary saponins and tannins from mangosteen peel and soapberry fruit. Arch Anim Nutr. 63(5):389–400.
- Purba RAP, Paengkoum P. 2019. Bioanalytical HPLC method of Piper betle L. for quantifying phenolic compound, water-soluble vitamin, and essential oil in five different solvent extracts. J Appl Pharm Sci. 9(5):033–039.
- Purba RAP, Paengkoum P, Paengkoum S. 2020a. The links between supplementary tannin levels and conjugated linoleic acid (CLA) formation in ruminants: A systematic review and meta-analysis. PLoS One. 15(3):e0216187.
- Purba RAP, Paengkoum S, Paengkoum P. 2021a. Development of a simple high-performance liquid chromatography-based method to quantify synergistic compounds and their composition in dried leaf extracts of Piper sarmentosum Robx. Separations. 8(9):152.
- Purba RAP, Paengkoum S, Yuangklang C, Paengkoum P. 2020b. Flavonoids and their aromatic derivatives in Piper betle powder promote in vitro methane mitigation in a variety of diets. Cienc Agrotec. 44:e012420.
- Purba RAP, Paengkoum S, Yuangklang C, Paengkoum P, Salem AZM, Liang JB. 2022. Mammary gene expressions and oxidative indicators in ruminal fluid, blood, milk, and mammary tissue of dairy goats fed a total mixed ration containing piper meal (Piper betle L.). Ital J Anim Sci. 21(1):129–141.
- Purba RAP, Yuangklang C, Paengkoum P. 2020c. Enhanced conjugated linoleic acid and biogas production after ruminal fermentation with Piper betle L. supplementation. Ciênc Rural. 50(7):e20191001.
- Purba RAP, Yuangklang C, Paengkoum S, Paengkoum P. 2020d. Milk fatty acid composition, rumen microbial population and animal performance in response to diets rich in linoleic acid supplemented with Piper betle leaves in Saanen goats. Anim Prod Sci. https://doi.org/10.1071/AN20182
- Purba RAP, Yuangklang C, Paengkoum S, Paengkoum P. 2021b. Piper oil decreases in vitro methane production with shifting ruminal fermentation in a variety of diets. Int J Agric Biol. 25:231–240.
- Ribeiro MN, Ribeiro NL, Bozzi R, Costa RG. 2018. Physiological and biochemical blood variables of goats subjected to heat stress – a review. J Appl Anim Res. 46(1):1036–1041.
- Samadieh H, Mohammadi GR, Maleki M, Borji H, Azizzadeh M, Heidarpour M. 2017. Relationships between oxidative stress, liver, and erythrocyte injury, trace elements and parasite burden in sheep naturally infected with dicrocoelium dendriticum. Iran J Parasitol. 12(1):46–55. Epub 2017/08/02.
- SAS Institute Inc. 2015. Step-by-step programming with base SAS® edition 9.4. Cary, NC: SAS Institute Inc.
- Schogor ALB, Palin M, dos Santos GT, Benchaar C, Lacasse P, Petit HV. 2013. Mammary gene expression and activity of antioxidant enzymes and oxidative indicators in the blood, milk, mammary tissue and ruminal fluid of dairy cows fed flax meal. Br J Nutr. 110(10):1743–1750. Epub 04/12.
- Suchitra K, Wanapat M. 2008. Effects of mangosteen (Garcinia mangostana) peel and sunflower and coconut oil supplementation on rumen fermentation, milk yield and milk composition in lactating dairy cows. Livest Res Rural Dev. 20. http://www.lrrd.org/lrrd20/supplement/such2.htm.
- Suong NTM, Paengkoum S, Salem AZM, Paengkoum P, Purba RAP. 2022. Silage fermentation quality, anthocyanin stability, and in vitro rumen fermentation characteristic of ferrous sulfate heptahydrate-treated black cane (Saccharum sinensis R.). Front Vet Sci. 9:896270.
- Suong NTM, Paengkoum P, Schonewille JT, Purba RAP, Paengkoum P. 2022. Growth performance, blood biochemical indices, rumen bacterial community, and carcass characteristics in goats fed anthocyanin-rich black cane silage. Front Vet Sci. 9:880838.
- Szerlauth A, Muráth S, Viski S, Szilagyi I. 2019. Radical scavenging activity of plant extracts from improved processing. Heliyon. 5:11.
- Tian XZ, Paengkoum P, Paengkoum S, Chumpawadee S, Ban C, Thongpea S. 2019. Purple corn (Zea mays L.) stover silage with abundant anthocyanins transferring anthocyanin composition to the milk and increasing antioxidant status of lactating dairy goats. J Dairy sci. 102(1):413–418.
- Tian XZ, Xin H, Paengkoum P, Paengkoum S, Ban C, Sorasak T. 2018. Effects of anthocyanin-rich purple corn (Zea mays L.) stover silage on nutrient utilization, rumen fermentation, plasma antioxidant capacity, and mammary gland gene expression in dairy goats. J Anim Sci. 97(3):1384–1397.
- Tilley JMA, Terry RA. 1963. A two-stage technique for the in vitro digestion of forage crops. Grass Forage Sci. 18(2):104–111.
- Vasta V, Daghio M, Cappucci A, Buccioni A, Serra A, Viti C, Mele M. 2019. Invited review: plant polyphenols and rumen microbiota responsible for fatty acid biohydrogenation, fiber digestion, and methane emission: experimental evidence and methodological approaches. J Dairy Sci. 102(5):3781–3804.
- Vorlaphim T, Paengkoum P, Purba RAP, Yuangklang C, Paengkoum S, Schonewille JT. 2021. Treatment of rice stubble with Pleurotus ostreatus and urea improves the growth performance in slow-growing goats. Animals. 11(4):1053.
- Wanapat M, Chanthakhoun V, Phesatcha K, Kang S. 2014. Influence of mangosteen peel powder as a source of plant secondary compounds on rumen microorganisms, volatile fatty acids, methane and microbial protein synthesis in swamp buffaloes. Livest Sci. 162:126–133.
- Wang Y, Branicky R, Noë A, Hekimi S. 2018. Superoxide dismutases: dual roles in controlling ros damage and regulating ros signaling. J Cell Biol. 217(6):1915–1928.
- Yang K, Qing Y, Yu Q, Tang X, Chen G, Fang R, Liu H. 2021. By-product feeds: current understanding and future perspectives. Agriculture. 11:3.
- Yuan S, Chen D, Zhang K, Yu B. 2007. Effects of oxidative stress on growth performance, nutrient digestibilities and activities of antioxidative enzymes of weanling pigs. Asian Australas J Anim Sci. 20(10):1600–1605.
- Yulistiani D, Jelan ZA, Liang JB, Yaakub H, Abdullah N. 2015. Effects of supplementation of mulberry (Morus alba) foliage and urea-rice bran as fermentable energy and protein sources in sheep fed urea-treated rice straw based diet. Asian-Australas J Anim Sci. 28(4):494–501.
- Zadernowski R, Czaplicki S, Naczk M. 2009. Phenolic acid profiles of mangosteen fruits (Garcinia mangostana). Food Chem. 112(3):685–689.
- Zaitsev SY, Belous AA, Voronina OA, Rykov RA, Bogolyubova NV. 2021. Correlations between antioxidant and biochemical parameters of blood serum of duroc breed pigs. Animals. 11:8.
- Zhang J, Xu X, Cao Z, Wang Y, Yang H, Azarfar A, Li S. 2019. Effect of different tannin sources on nutrient intake, digestibility, performance, nitrogen utilization, and blood parameters in dairy cows. Animals. 9:8.