ABSTRACT
Two experiments (Exps.) were conducted to examine the interactions among methane inhibitors on 24 h in vitro methane production using monensin (MON), fumaric acid (FA), and bromochloromethane (BCM). Donor goats and in vitro fermentation incubations were both treated with a 50:50 concentrate:forage diet (DM basis). In Exp. 1, MON (0, 60, or 120 mg/kg DM) and FA (0, 3.44, or 6.89 mM) were used to examine their two-way interaction, and no interaction was found on methane production (P > 0.05). In Exp. 2, MON (0 or 60 mg/kg DM), FA (0 or 6.89 mM), and BCM (0 or 3.08 μM) were used to examine their three-way interaction, and no three-way interaction was observed (P > 0.05). The correlation was significant under the MON and FA treatments without BCM (r = 0.55, P < 0.05), and under the MON, FA, and BCM treatments, the correlation was also significant (r = 0.59, P < 0.05). Collectively, no two- or three-way interactions were observed among MON, FA, and BCM. Regression analyses showed that MON, FA, and BCM were independent from each other, and the inhibitory effects of the three methane inhibitors on methanogenesis to a certain range are additive.
Introduction
Methane, a greenhouse gas, has contributed mainly to climate warming. During the past few decades, the contribution of ruminants to methane emissions has increased rapidly, accounting for half of all noncarbon dioxide greenhouse gas emissions from the agriculture (Dangal et al. Citation2017). In addition, methane produced in the rumen accounts for, on average, 6.4% of gross energy loss to the ruminants (Ranga Niroshan Appuhamy et al. Citation2013). As a consequence, reducing methanogenesis in ruminants is of great significance and interest and is accompanied by the application of many methane inhibitors (Hristov et al. Citation2013).
Owing to different inhibitory mechanisms on methanogenesis, all methane inhibitors can be divided into three types: microflora modifying inhibitors (MMIs), hydrogen receptors (HRs), and enzymatic inhibitors (EIs). MMIs, which can inhibit gram-positive bacteria and protozoa, decrease substrates for methanogenesis (Hook et al. Citation2010; Kumar et al. Citation2013). Monensin (MON), saponins, and tannins are considered MMIs. HRs are well known as a hydrogen sink in the rumen (Hristov et al. Citation2013), and compete with methanogens for the utilization of metabolic hydrogen (Castillo et al. Citation2004; Li et al. Citation2009), resulting in a decrease in the proportion of acetate and an increase in the proportion of propionate in the rumen (Hook et al. Citation2010). Unsaturated fats, nitrates, and organic acids such as fumaric acid (FA) are HRs (Castillo et al. Citation2004). As EIs, halogenated methane analogs, including bromochloromethane (BCM) (Shima et al. Citation2002), 2-bromoethanesulfonate (Lee et al. Citation2009), and 3-nitroxypropanol (Pitta et al. Citation2018), inhibit the enzymatic activities needed in methanogenesis and decrease methane emissions. An increasing number of studies have focused on the combination of these inhibitors owing to their different modes of action in the rumen to maximize methane mitigation ability (Patra and Yu Citation2015; Capelari et al. Citation2018; Vyas et al. Citation2018). Some binary combination studies have been performed among these three types of inhibitors: MMIs and HRs (Callaway and Martin Citation1996; Capelari et al. Citation2018), HRs and EIs (Choi et al. Citation2004; Ebrahimi et al. Citation2011), and MMIs and EIs (Romero-Pérez et al. Citation2017; Vyas et al. Citation2018).
However, no study has investigated the impacts of the three-way interaction of these three types of methane inhibitors. The hypothesis of this research was that no interactions (two- and/or three-way) exist among the three types of methane inhibitors owing to the different inhibitory mechanisms. Accordingly, the objective of the present study was to examine the interactions among three typical and functionally well-defined methane inhibitors (MON, FA, and BCM) after 24 h of in vitro methane production and fermentation.
Materials and methods
Experimental design
Exp. 1. Effect of MON and FA on in vitro methane production and fermentation
In this experiment (Exp. 1), MON (Monensin Premix, 20%; Shandong Shengli Co., Ltd, Jinan, Shandong Province, China) and FA (F19353, 99%; Sigma–Aldrich Crop., St. Louis, Missouri, USA) were added to a medium grain diet (concentrate and ground alfalfa hay; 50:50) to examine the two-way interaction between MON and FA on in vitro methane production and fermentation. The MON at levels of 0, 60, and 120 mg/kg dry matter (DM) and FA at levels of 0, 3.44, and 6.89 mmol were used in the present experiment, and each experimental run was carried out with eight duplicates.
Exp. 2. Effect of MON, FA, and BCM on in vitro methane production and fermentation
The aim of the present experiment was to examine the three-way interaction among MON, FA, and BCM on in vitro methanogenesis and fermentation. The levels of 0 and 60 mg/kg DM for MON and the levels of 0 and 6.89 mmol for FA were used. The BCM (457493, 99%; Sigma–Aldrich Corp., St. Louis, Missouri, USA) was added at 0 and 3.08 μmol. In the present experiment, the same medium grain diet as Exp. 1 was used and carried out with six duplicates in each experimental run.
Fermentation preparation
All procedures in this work were reviewed and approved by the Northwest A&F University Animal Care and Use Committee. Ruminal inocula were collected from three rumen-fistulated goats before morning feeding, and the inocula were used for the in vitro incubations. These goats were offered a diet of a total of 2.4 kg DM daily (provided 1.2 kg twice at 08:00 and 20:00). The diet contained 50% pelleted concentrate and 50% pelleted alfalfa hay, and the concentrate was composed of 25% soybean meal, 70% ground corn, and 5% premix. All goats had been adapted to this diet prior to the experiment for 14 days.
Ruminal contents were moved to an insulated container and then transported to the laboratory immediately (within 5 min). The samples from the three donor goats were mixed (1:1:1, v/v) and then blended using a Waring blender (Fisher 14-509-1) under a carbon dioxide atmosphere (20 s) to detach the bacteria and protozoa from the feed particles. The blended samples were then strained through glass wool and 4 layers of cheesecloth and used as ruminal inocula for subsequent fermentation, followed by combination with a buffer solution. The buffer solution, reducing solution, and micro- and macromineral solutions were prepared as previously described by Goering and Van Soest (Citation1970) and as a ruminal buffer solution. These ruminal buffer solutions (1100 mL) and the ruminal inocula (200 mL) were combined and warmed, and then 50 mL was added to fermentation vessels (260 mL) to ferment at 39℃ for 24 h. Feed samples (600 mg) were weighed and placed in the sealed fermentation vessels equipped with a gas sampling port and automatic pressure transducers (Ankom Technology, Macedon, New York, USA). The control fermentation vessels (without additives) and blank fermentation vessels (without feed or additives) in Exps. 1 and 2, were both supplemented with 50 mL of the ruminal buffer solution.
Collection and analysis of samples
For each incubation in the vessel, gas pressure was monitored using the previously mentioned automated pressure transducer system (Xu et al. Citation2010). At termination of the incubation, gas samples were drawn into sampling syringes and transferred into a vacuum test tube (Vacutainer, Becton Dickinson, Franklin Lakes, New Jersey, USA), and then gas chromatography (7820A, Agilent Technologies, Santa Clara, California, USA) was used to analyze the methane content (Xu et al. Citation2010). The fermentation substrates were then analyzed for pH immediately with a pH meter (Model PHS-2F, Shanghai Precision and Scientific Instrument CO., LTD, Shanghai, China). An aliquot of the fermentation substrates (1.5 mL) was combined with 25% metaphosphoric acid (0.3 mL) and then centrifuged for 15 min (39,000 ×g, 4°C). The supernatant (1 mL) was transferred into vials and then capped and analyzed for total volatile fatty acid (VFA) concentrations (including acetate, butyrate, propionate, valerate, isobutyrate, and isovalerate) by gas chromatography. The gas chromatography was fitted with an autosampler, a flame-ionization detector, and a 30 m × 0.32 mm × 0.25 µm capillary column (DB-FFAP, Agilent Technologies, Santa Clara, California, USA). The concentrations of individual VFAs were summed to obtain the total VFA (TVFA) concentration.
Regression analysis between the predicted methane and observed methane production
Regression analysis between the predicted methane production (calculated based on the production of VFAs) and the observed methane production was conducted in the current study to investigate whether the MON-, FA- and BCM-induced reduction in methanogenesis was caused by changes in VFA production. The predicted methane production relative to VFA concentration (mmol/mol VFA) was estimated using the following equation (Castro Montoya et al. Citation2011):
Methane (mmol/mol VFA) = 0.45 × acetate+ 0.4 × butyrate − 0.275 × propionate.
The units of methane, acetate, butyrate, and propionate are all mmol relative to VFA (mol), and VFAs include production of acetate, butyrate, and propionate. The observed methane production relative to VFA concentration (mmol/mol VFA) was presented in the current study and calculated from: methane production (mL)/22.4/(TVFA × 50 mL/1000).
Statistical analysis
The data were analyzed by a two-factor complete random test design, and the GLM of SPSS13.0 was selected in Exp. 1. The experimental factors included the main effects of MON and FA and their interactions. In Exp. 2, the data were analyzed by a three-factor complete random test design, and the GLM of SPSS13.0 was selected. The main effects of MON, FA, and BCM and their interactions were investigated. Regression analysis was performed by a linear regression model in SPSS13.0. The factors that predicted methane and observed methane production were included in the linear regression model. Significance was declared at P values of ≤ 0.05, and tendencies were declared at P values of ≤ 0.10.
Results
Exp. 1. Effect of MON and FA on in vitro methane production and fermentation
There were no interactions (P > 0.05) between MON and FA on fermentation pH, total gas production, methane production, methane percentage in total gas production, TVFA concentration, or VFA profiles as shown in .
Table 1. The effects of MON and FA on in vitro methane production and fermentation (Exp. 1).
MON had no effect on the total gas production, fermentation pH, or TVFA concentration (P > 0.05) and tended to decrease methane production (P = 0.100) and the methane percentage in total gas production (P = 0.059). FA increased gas production (P < 0.05), decreased fermentation pH and methane percentage in total gas production (P < 0.05), and did not affect TVFA concentrations and methane production (P > 0.05). MON decreased the acetate proportion (P < 0.05), increased the propionate proportion (P < 0.01), and decreased the acetate:propionate ratio (A:P ratio) (P < 0.01). FA decreased the acetate proportion and A:P ratio (P < 0.01), and increased the propionate proportion (P < 0.05). Neither MON nor FA affected the proportion of butyrate, valerate, isobutyrate, or isovalerate (P > 0.05).
Exp. 2. Effect of MON, FA, and BCM on in vitro methane production and fermentation
For all fermentation parameters, there were no two-way interactions among MON, FA, and BCM (P > 0.05) or three-way interactions (P > 0.05) on in vitro methane production and fermentation ().
Table 2. The effects of MON, FA, and BCM on in vitro methane production and fermentation (Exp. 2).
In , MON tended to decrease fermentation pH (P = 0.081) and did not decrease the methane percentage in total gas production or methane production (P > 0.05). MON had the same effects on other parameters (total gas production, TVFA concentration, VFA profiles, and A:P ratio) as Exp. 1. For FA, all fermentation parameters were affected similarly to those in Exp. 1, except for the methane percentage in the total gas production, which only tended to decrease with FA (P = 0.055). BCM decreased methane production and methane percentage in total gas production (P < 0.05) and had no effect on total gas production, pH, TVFA concentrations or the A:P ratio (P > 0.05). BCM did not affect VFA profiles but increased the valerate proportion (P < 0.01).
Regression analysis between the predicted methane and observed methane production
A and B shows the relationship between the predicted methane and observed methane production in Exp. 1 and 2, respectively. In Exp. 1, a significant correlation between the predicted methane and observed methane production under the MON and FA treatments was found (r = 0.46, P < 0.001). In Exp. 2, a significant correlation was shown for the predicted methane and observed methane production with the treatment of MON and FA without BCM (r = 0.55, P = 0.012), and a significant correlation between the predicted methane and observed methane production with the fermentation of MON, FA, and BCM treatment was also observed (r = 0.57, P = 0.006).
Figure 1. Linear regression between the predicted methane and observed methane production. A (Exp. 1): y = 0.68x + 21.23 (r = 0.46, P < 0.001). B (Exp. 2): ‘NO’ indicates treatment without BCM (dotted line); y = 0.73x-0.82 (r = 0.55, P = 0.012); ‘BCM’ indicates treatment with BCM (solid line), y = 0.66x-4.22 (r = 0.59, P = 0.006).
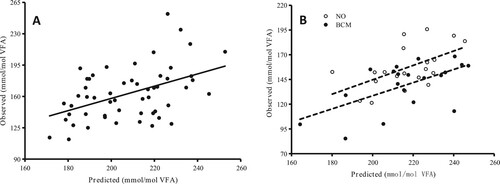
Discussion
MON, FA, and BCM are different types of methane inhibitors and represent MMIs, HRs, and EIs, respectively. In the present study, MON decreased methanogenesis and influenced the proportions of acetate and propionate. These results were in agreement with previous studies (Ponce et al. Citation2012; Wischer et al. Citation2013). FA has shown the effect of depressing methanogenesis, and the increasing propionate proportion at the expense of decreasing acetate proportion, so decreased the A:P ratio, both of which were in line with the results of previous studies (Newbold et al. Citation2005; Hook et al. Citation2010). In the current investigation, methane production decreased by 32.1% with the addition of BCM at 3.08 μmol, in contrast with the control, which was in line with the results of previous studies. Goel et al. (Citation2009) reported that in vitro ruminal fermentation with the addition of BCM at 5 and 10 μmol decreased methane production by 77.9% and 93.0%, respectively. The results of our preliminary study showed that the addition of BCM at 3.08 and 15.40 μmol inhibited methane production by 22.0% and 94.0%, respectively (data not shown). It was not our intent to inhibit methanogenesis solely by BCM when investigating its possible interaction with MON and FA; therefore, a low dose of BCM was chosen in the current study. More importantly, BCM was shown to be toxic at a high level of addition.
MON and FA were added together resulted in greater methane inhibition than when utilized individually, and their additive effect was observed on all ruminal fermentation parameters in the present study. Since the two compounds were representatives of two types of methane inhibitors, it was likely that the MMIs and HRs operate independent from each other. The result was supported by the study of Callaway and Martin (Citation1996), which showed no interactions between MON and fumarate on methane production, total gas production, TVFA, VFA profiles, and A:P ratio when MON and fumarate were fermented in combinations in vitro. Other studies for the two types of methane inhibitord used saponin and nitrate (Patra and Yu Citation2015), MON and calcium propionate (Osorio-Teran et al. Citation2017), and encapsulated nitrate and sodium MON (Capelari et al. Citation2018), and all studies showed the same results as those of the current study.
Despite the widespread use of the three types of methane inhibitors in ruminant diets, previous studies have not explored their possible three-way interaction effect on methane inhibition. According to the two experimental results, we established regression analysis between observed methane production and predicted methane production based on the production of acetate, butyrate, and propionate (Castro Montoya et al. Citation2011). A (Exp. 1) shows a significant correlation between observed methane and predicted methane production for all groups. Therefore, it’s likely that both the inhibiting effects of MON and FA on methanogenesis were by the mechanism of changing VFA profiles. More specifically, MON decreased the generation of VFAs in substrates by inhibiting gram-positive bacteria and protozoa and thereby decreased acetate production and increased propionate production (Hook et al. Citation2010; Ellis et al. Citation2012; Kumar et al. Citation2013), and FA competed with methanogens for the utilization of metabolic hydrogen and thus decreased acetate production and increased propionate production (Castillo et al. Citation2004; Li et al. Citation2009; Hook et al. Citation2010). According to the results of Exp. 2, regression analyses both showed a significant correction between observed methane and predicted methane production for MON and FA, without or with BCM (B). However, the two regressions in B show that the observed methane with BCM was always lower than that without BCM at the same predicted methane level (based on the production of acetate, butyrate, and propionate) in the experimental range in the present study. Indeed, it is well known that BCM inhibits the enzymatic activities needed in methanogenesis (Shima et al. Citation2002). Therefore, BCM did not affect VFA production when inhibiting methanogenesis in the present study, and the mechanism of BCM-induced methane inhibition was different from that of MON and FA, which changed the VFA profiles.
Currently, there are few relevant in vivo studies based on binary or tertiary combinations of the three types of methane inhibitors. A study in beef cattle showed that MON and 3-nitrooxypropanol acted independently in animals fed high-forage or high-grain diets (Vyas et al. Citation2018). This study confirmed that in vivo research had the same results as the current study. Performing in vivo and in vitro experiments simultaneously with respect to methanogenesis inhibition gives that the in vitro results better resemble what happens in the rumen than in the whole animal (Hatew et al. Citation2015; Jonker et al. Citation2016). In vitro experimental approaches offer the opportunity to evaluate multiple additives alone or in combination over a wide range of supplementation levels (Yáñez-Ruiz et al. Citation2016), and they can eliminate the influence of the whole body on the rumen, such as the absorption of VFAs. Future research on in vivo binary or tertiary combinations, especially direct in vitro-in vivo comparisons, should be conducted. Furthermore, binary or tertiary combinations of the three types of methane inhibitors to inhibit methanogenesis should be widely used, since the combinations eliminate dietary interference from ruminant diets or other variations (Patra and Yu Citation2015).
Conclusions
In the present study, there were no two- or three- way interactions among MON, FA, and BCM in decreasing in vitro methane production. Thus, the inhibitory effects of the three types of methane inhibitors on methanogenesis to a certain range were additive. Correlations between the predicted and observed methane production under MON and FA treatment without or with BCM treatment were both significant. More practical strategies with binary and tertiary combinations of the three types of methane inhibitors might be promising.
Acknowledgements
The study was supported by the funds from the Science and Technology Major Project of Inner Mongolia (2020ZD0004).
Disclosure statement
No potential conflict of interest was reported by the author(s).
Additional information
Funding
References
- Callaway TR, Martin SA. 1996. Effects of organic acid and monensin treatment on in vitro mixed ruminal microorganism fermentation of cracked corn. J. Anim. Sci. 74:1982–1989.
- Capelari M, Johnson KA, Latack B, Roth J, Powers W. 2018. The effect of encapsulated nitrate and monensin on ruminal fermentation using a semi-continuous culture system. J. Anim. Sci. 96:3446–3459.
- Castillo C, Benedito JL, Méndez J, Pereira V, López-Alonso M, Miranda M, Hernández J. 2004. Organic acids as a substitute for monensin in diets for beef cattle. Anim. Feed Sci.Technol. 115:101–116.
- Castro Montoya J, Bhagwat AM, Peiren N, Campeneere SD, Baets BD, Fievez V. 2011. Relationships between odd- and branched-chain fatty acid profiles in milk and calculated enteric methane proportion for lactating dairy cattle. Anim. Feed Sci. Technol. 166–167:596–602.
- Choi NJ, Lee SY, Sung HG, Lee SC, Ha JK. 2004. Effects of halogenated compounds, organic acids and unsaturated fatty acids on in vitro methane production and fermentation characteristics. Asian-aust. J. Anim. Sci. 17:1255–1259.
- Dangal SRS, Tian H, Zhang B, Pan S, Lu C, Yang J. 2017. Methane emission from global livestock sector during 1890-2014: magnitude, trends and spatiotemporal patterns. Glob Chang Biol. 23:4147–4161.
- Ebrahimi SH, Mohini M, Singhal KK, Miri VH, Tyagi AK. 2011. Evaluation of complementary effects of 9,10-anthraquinone and fumaric acid on methanogenesis and ruminal fermentation in vitro. Arch Anim Nutr. 65:267–277.
- Ellis JL, Dijkstra J, Bannink A, Kebreab E, Hook SE, Archibeque S, France J. 2012. Quantifying the effect of monensin dose on the rumen volatile fatty acid profile in high-grain-fed beef cattle. J. Anim. Sci. 90:2717–2726.
- Goel G, Makkar HPS, Becker K. 2009. Inhibition of methanogens by bromochloromethane: effects on microbial communities and rumen fermentation using batch and continuous fermentations. Br. J. Nutr. 101:1484–1492.
- Goering HK, Van Soest PJ. 1970. Forage fiber analysis (Apparatus, Reagents, Procedures and Some Applications). Agric Handbook No. 379, US Govt. Printing off., Washington, DC.
- Hatew B, Cone JW, Pellikaan WF, Podesta SC, Bannink A, Hendriks WH, Dijkstra J. 2015. Relationship between in vitro and in vivo methane production measured simultaneously with different dietary starch sources and starch levels in dairy cattle. Anim. Feed Sci. Technol. 202:20–31.
- Hook SE, Wright André-Denis G, Mcbride BW. 2010. Methanogens: methane producers of the rumen and mitigation strategies. Archaea. 2010:1–11.
- Hristov AN, Joonpyo O, Firkins JL, Dijkstra J, Kebreab E, Waghorn G, Makkar HPS, Adesogan AT, Yang W, Lee C, et al. 2013. SPECIAL TOPICS – mitigation of methane and nitrous oxide emissions from animal operations: I. A review of enteric methane mitigation options. J. Anim. Sci. 91:5045–5069.
- Jonker A, Lowe K, Kittelmann S, Janssen PH, Ledgard S, Pacheco D. 2016. Methane emissions changed nonlinearly with graded substitution of alfalfa silage with corn silage and corn grain in the diet of sheep and relation with rumen fermentation characteristics in vivo and in vitro. J. Anim. Sci. 91:5045–5069.
- Kumar S, Choudhury PK, Carro MD, Griffith GW, Dagar SS, Puniya M, Calabro S, Ravella SR, Dhewa T, Upadhyay RC, et al. 2013. New aspects and strategies for methane mitigation from ruminants. Appl Microbiol Biotechnol. 98:31–44.
- Lee SY, Yang SH, Lee WS, Kim HS, Shin DE, Ha JK. 2009. Effect of 2-bromoethanesulfonic acid on in vitro fermentation characteristics and methanogen population. Asian-aust. J. Anim. Sci. 22:42–48.
- Li XZ, Choi SH, Jin GL, Yan CG, Song MK. 2009. Linolenic acid in association with malate or fumarate increased CLA production and reduced methane generation by rumen microbes. Asian-aust. J. Anim. Sci. 22:819–826.
- Newbold CJ, López S, Nelson N, Ouda JO, Wallace RJ, Moss AR. 2005. Propionate precursors and other metabolic intermediates as possible alternative electron acceptors to methanogenesis in ruminal fermentation in vitro. Br. J. Nutr. 94:27–35.
- Osorio-Teran AI, Mendoza-Martínez GD, Miranda-Romero LA, Martínez-Gomez D, Hernández-García PA, Martínez-García JA. 2017. Effect of calcium propionate and monensin on in vitro digestibility and gas production. Rev. Bras. Zootecn. 46:348–353.
- Patra AK, Yu Z. 2015. Effects of garlic oil, nitrate, saponin and their combinations supplemented to different substrates on in vitro fermentation, ruminal methanogenesis, and abundance and diversity of microbial populations. J. Appl. Microbiol. 119:127–138.
- Pitta DW, Nagaraju I, Linda B, Bonnie V, Graeme A. 2018. Symposium review: understanding diet–microbe interactions to enhance productivity of dairy cows. J. Dairy Sci. 101:1–19.
- Ponce CH, Smith DR, Branine ME, Hubbert ME, Galyean ML. 2012. Effects of type of ionophore and carrier on in vitro ruminal dry matter disappearance, gas production, and fermentation end products of a concentrate substrate. Anim. Feed Sci. Technol. 171:223–229.
- Ranga Niroshan Appuhamy JAD, Strathe AB, Jayasundara S, Wagner-Riddle C, Dijkstra J, France J, Kebreab E. 2013. Anti-methanogenic effects of monensin in dairy and beef cattle: A meta-analysis. J. Dairy Sci. 96:5161–5173.
- Romero-Pérez A, Okine EK, Guan LL, Duval SM, Kindermann M, Beauchemin KA. 2017. Rapid communication: evaluation of methane inhibitor 3-nitrooxypropanol and monensin in a high-grain diet using the rumen simulation technique (rusitec). J. Anim. Sci. 95:4072–4077.
- Shima S, Warkentin E, Thauer RK, Ermler U. 2002. Structure and function of enzymes involved in the methanogenic pathway utilizing carbon dioxide and molecular hydrogen. J. Biosci. Bioeng. 93:519–530.
- Vyas D, Alemu AW, Mcginn SM, Duval SM, Kindermann M, Beauchemin KA. 2018. The combined effects of supplementing monensin and 3-nitrooxypropanol on methane emissions, growth rate, and feed conversion efficiency in beef cattle fed high forage and high grain diets. J. Anim. Sci. 96:2923–2938.
- Wischer G, Boguhn J, Steingaβ H, Schollenberger M, Hartung K, Rodehutscord M. 2013. Effect of monensin on in vitro fermentation of silages and microbial protein synthesis. Arch. Anim. Nutr. 67:219–234.
- Xu M, Rinker M, McLeod KR, Harmon DL. 2010. Yucca schidigera extract decreases in vitro methane production in a variety of forages and diets. Anim. Feed Sci. Technol. 159:18–26.
- Yáñez-Ruiz DR, Bannink A, Dijkstra J, Kebreab E, Morgavi DP, O’Kiely P, Reynolds CK, Schwarm A, Shingfield K, Yu Z, Hristov AN. 2016. Design, implementation and interpretation of in vitro batch culture experiments to assess enteric methane mitigation in ruminants–a review. Anim. Feed Sci.Technol. 216:1–18.