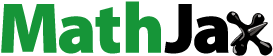
ABSTRACT
The objective of this research was to investigate the effects of nitrate, chitosan extract or shrimp shell meal on in vitro degradability and gas production kinetics. The study was arranged in a 2 × 7 factorial arrangement in a completely randomized design (CRD). Factor A was two ratios of roughage (R) to concentrate (C) ratio, R:C ratios at 60:40 and 40:60 (R:C), and the other factors were as several kinds and levels of additives (Non: non supplementation; Low-Ni: supplementation with 7 mg of KNO3; High-Ni: supplementation with 14 mg of KNO3; Low-CE: supplementation with 1.6 mg of chitosan extract (CE); High-CE: supplementation with 4.8 mg of chitosan extract; Low-SSM: supplementation with 8 mg of shrimp shell meal (SSM) and High-SSM: supplementation with 24 mg of SSM). Cumulative gas production was significantly decreased in the supplemented group. When increasing the level of a concentrate feed, propionate (C3) and total volatile fatty acid were enhanced, while acetate (C2) and C2 to C3 ratios were reduced (P < 0.05). The C3 and total VFA concentrations were increased with the supplementation of nitrate, chitosan extract and shrimp shell meal. CH4 production by nitrate, chitosan extract and shrimp shell meal addition was found to be lower than that of the control treatment.
1. Introduction
The production of methane through enteric fermentation is of worldwide concern because of its contribution to the accumulation of greenhouse gases in the atmosphere and the waste of dietary energy for ruminants (Johnson and Johnson Citation1995; Boadi et al. Citation2004). Mitigation of CH4 emission by the inhibition of ruminal methanogens, would in turn increase the feed utilization for ruminants and have significant economical and environmental benefits (Benchaar and Greahead Citation2011; Wanapat et al. Citation2012). Therefore, developing feeding strategies for ruminants with methane mitigation is desirable and the inhibition of methanogenesis has long been considered as one of the strategies to improve ruminant productivity.
Enteric methane production in ruminants can be mitigated by removing rumen methanogens, decreasing hydrogen production, or providing an alternative hydrogen-sink (Joblin Citation1999; Ellis et al. Citation2008). It was concluded that methanogens were usually capable of hydrogen utilization than other microbes. McAllister and Newbold (Citation2008) also reported that the reduction of an alternative electron acceptor has to be energetically more favourable than the production of methane from CO2, in order to decrease methane emission. Nitrate is an electron-sink, shown to suppress the production of CH4 in sheep (Takahashi and Young Citation1991; Sar et al. Citation2005). Leng (Citation2014) reported that using nitrate as a feed component replacing urea has a dual role as an electronic-sink for H2 produced by fermentation and that ammonia produced is the preferred source of fermentable nitrogen in diets having a low content of crude protein. Thus, it is logical to test whether nitrate can be used to lower methane production.
Chitosan extract (CE), is a natural biopolymer derived through the deacetylation of chitin, a major component of the shells of crustaceans. CE has received much attention for diverse potential applications in medicine and food preservation because of its against bacteria and anti-microbial properties (Jeon et al. Citation2002). Moreover, CE may provide an alternative to anti-microbial growth promoters in the feeds (Goiri et al. Citation2009). Benefits observed in vitro seem to be caused by changes in rumen fermentation, in particular, by increased C3 and reduced methane production, which in turn lead to energetically more efficient fermentation patterns, and also by decreased ruminal protein disappearance (Goiri et al. Citation2009). Hence, the aim of this study was to investigate the effect of the levels of nitrate, chitosan extract or shrimp shell meal on different roughage and concentrate ratio-based diets by using in vitro gas production technique.
2. Materials and methods
The experimental design and procedures were approved by the Khon Kaen University Animal Ethics Committee (KKU28/2560).
2.1. Dietary treatments and experimental design
This study was conducted using an in vitro gas fermentation technique at various incubation time intervals. The experiment design was a 2 × 7 factorial arrangement in a completely randomized design (CRD). Factor A was 2 levels of R:C ratio (60:40, 40:60) and factor B was 7 levels of additives (Non: non supplementation; Low-N: supplementation with 7 mg of KNO3; High-N: supplementation with 14 mg of KNO3; Low-CE: supplementation with 4.8 mg of chitosan extract; Low-SSM: supplementation with 4.8 mg of shrimp shell meal and High-SSM: supplementation with 24 mg of shrimp shell meal). Substrates and additives were ground and weighed (total substrate mixture 200 mg of DM) into 50 ml bottles for various time incubations. Experimental ingredients and nutritive values are illustrated in .
Table 1. Ingredients and chemical composition of the diet used in the experiment.
Rice straw (RS) was pre-treated by using 2.0% urea + 2.0% calcium hydroxide or lime-solution (ULTRS) in 100 ml of water. A mixture of urea-lime solution was sprinkled on rice straw bales, well covered with plastic sheets and ensiled for at least 10 days before feeding. As reported in , the concentrate mixture was formulated using locally available feed ingredients. The ULTRS and concentrate mixtures were dried in a forced-air oven at 50°C and ground to pass through a screen (1 mm) size to chemically analyze for the nutritive values of the feedstuffs (dry matter, DM, organic matter, OM, and crude protein, CP) using the standard method (AOAC Citation1990). The fibre contents (neutral detergent fibre, NDF and acid detergent fibre, ADF) were analyzed by using the method of Van Soest et al. (Citation1991). Chitin was determined according to Stelmock et al. (Citation1985).
2.2. Rumen fluid and substrate sources
Two, rumen-fistulated dairy steers were used as rumen fluid donors. Each animal was fed with a concentrate (14.1% CP) at 0.5% BW, while ULTRS was fed ad libitum. Before the morning feeding, rumen fluid (1 L) was collected using a suction pump. The experimental diet was weighed and mixed into a 50 ml bottle for each mixed substrate (200 mg). All bottles were tightly well sealed. The sample was mixed with the prepared artificial saliva solution (2 ml:1 ml) and was incubated at 39°C under continuous CO2 flushing and placed in a shaking water bath (Makkar et al. Citation1999). The rumen fluid medium of 40 ml was mixed with the substrate in each bottle and then incubated at 39°C in a shaking water bath, following the method of Blummel and Orskov (Citation1998).
2.3. In vitro gas production and fermentation characteristic kinetics
Gas production was subsequently measured at intervals following the respective incubations at 0, 1, 2, 4, 6, 8, 12, 24, 48, 72, and 96 h. Cumulative production of data was fitted to the Orskov and McDonal (Citation1979) model, as follows:
where y = gas produced at time ‘t’, a = gas production from the immediately soluble fraction, b = gas production from the insoluble fraction, c = gas production rate constant for the insoluble fraction (b), t = incubation time, and (a + b) = the potential extent of gas production.
The substrate-rumen fluid mixture was sampled at 4 and 8 h post-incubation to measure the pH. The samples were then divided into two parts; the first portion was used for a total direct count of rumen protozoa by fixing with formalin (10%) in a sterilized saline solution (0.9%). The rumen protozoa counts were enumerated by using a haemacytometer (Galyen Citation1989). The last portion was centrifuged at 16,000 × g for 15 min before the supernatant was taken and stored at −20°C before NH3-N analysis (Kjeltech Auto 1030 Analyzer, Tecator, Sweden). The VFA production was chemically analyzed using high performance liquid chromatography (HPLC) [instruments by controller water model 600E, water model 484 UV detector, column Nova-Pak C18, column size 3.9 mm × 300 mm, mobile phase 10 mM H2PO4 (pH 2.5)] according to Samuel et al. (Citation1997). Methane production was estimated using the equation of Moss et al. (Citation2000): CH4 production = 0.45(acetic acid) − 0.275 (propionic acid) + 0.4(butyric acid). The inocula after inoculation were filtered through pre-weighed Gooch crucibles, and residual dry matter was estimated. The percent loss in weight was determined and presented as in vitro dry matter degradability (IVDMD). The dried feed sample and residue left above were ashed at 550°C, for the determination of in vitro organic matter degradability (IVOMD) (Tilley and Terry Citation1963).
2.4. Statistical analysis
All data were prepared for the analysis of variance (ANOVA) according to the General Linear Model of SAS (Citation2013). The following model was used:
Y = observation, μ = overall mean, Aj = factor A effect (A = R: C ratio) Bk = factor B effect (levels of additives), ABjk = interaction effect and Eijk = error. Multiple comparisons among treatment means were performed by Duncan’s New Multiple Range Test (DMRT). Differences among means with P < 0.05 were accepted as representing statistically significant differences.
3. Results and discussion
3.1. Chemical composition of the experimental feeds
presents the feed ingredients and their chemical compositions. The concentrate was formulated using available and common feed resources, namely, cassava chip, rice bran, coconut meal, palm kernel meal, urea, molasses, salt mineral premix, and sulfur. Rice straw is a crop-residue that is abundantly available in the tropic. Despite the low nutritive value and high level of fibre and lignin, it was fed to ruminants, especially during the long dry season, it has been used as a main roughage source. It can be chemically treated with 2% of urea and calcium hydroxide (lime) to enhance digestibility and DM intake. Straw quality improvement with a lime solution with minimal urea concentration was beneficial and economical. The cost of lime is cheaper than urea. Rice straw was treated with 2% of urea and lime under this experiment and contained 5.8% CP and fibre (71.4% NDF and 51.3% ADF), respectively. The result was similar to the finding of Phesatcha et al. (Citation2020), who showed that RS treated with lime and urea significantly increased DM and CP intake, nutrient digestibility, as well as VFA production. It was promising to treat with the urea-lime solution to enhance the nutritive value of RS, as a major roughage source.
3.2. In vitro gas production kinetics
Cumulative gas production for each substrate treatment for each time of measurement is presented as gas production and kinetics of gas production, and are reported in . Increasing the levels of concentrate affected total in vitro gas production volume at 96 h after subsequent incubation (P < 0.001), gas production volume from the immediately soluble fraction (a) (P < 0.001), gas production from the insoluble fraction (b) (P < 0.001), gas production rate (c) (P > 0.05) and the potential extent of gas production (a + b) (P < 0.001). These findings indicated that the inclusion of a high proportion of concentrate resulted in an increasing rate and extent of fermentation of the inoculums. These results agreed with Anantasook and Wanapat (Citation2012) who reported that cumulative gas production was higher when the R:C ratio decreased from 70:30 to 30:70.
Table 2. Effects of nitrate (N), chitosan extract (CE) and shrimp shell meal (SSM) on in vitro gas production kinetics.
Feed additives supplementation also impacted the total cumulative gas volume (P < 0.05). Cumulative gas production in respective treatments was the highest on treatment with Low-CE and the lowest was at High-N addition. In the rumen, continuous microbial fermentation is contingent on the reoxidation of cofactors generated when organic matter is fermented to volatile fatty acids (VFA) with the synthesis of microbial cells. Nitrate can fully replace CO2 as an electron acceptor and will generate another reduced product, such as nitrate reduced to nitrite and then to ammonia (Leng Citation2014). The reactions by which electrons are transferred to produce methane or ammonia, therefore, hydrogen sinks in the rumen, were trapped and resulted in lower rumen gas production.
Chitosan has antimicrobial activity against different groups of microorganisms (Goiri et al. Citation2009; Benhabiles et al. Citation2012) that produce enzymes to hydrolyze the forage and that results in the reduction of total gas production and decrease in IVDMD and IVDOM. The results of the present study were also in agreement when supplemented with high levels of chitosan extract and shrimp shell meal on in vitro fermentation.
Under this present study, there were no significant interactive effects between the factor R:C ratio and supplementation of chitosan extract, nitrate and shrimp shell meal. With an increasing level of the concentrate ratio, in vitro dry matter degradability (IVDMD) and in vitro organic matter degradability (IVOMD) under this investigation was significantly enhanced. These data were similar to those reported by Kang et al. (Citation2017). This result could be due to the improvement of rumen microbial growth which could reflect on the degradation of feed activity. In addition, IVDMD and IVOMD were decreased with the addition of high chitosan extract and high shrimp shell.
3.4. Effect on ruminal ammonia-nitrogen (NH3-N) concentration
shows the data of the R:C ratio and supplementation of nitrate, chitosan extract and shrimp shell meal on NH3-N concentration. No interactions were obtained between the factor of R:C ratio and supplementation of nitrate, chitosan extract and shrimp shell meal on rumen NH3-N profile under this study. The NH3-N data were from 11.1 to 15.8 mg/dl for all treatments. Ruminal pH is a major factor in rumen ecology; hence, it could be maintained at a normal range (pH 6.3-6.7) to support suitable rumen fermentation and microbial population. Ruminants can generally buffer a ruminal pH of 6.3-7.0 when fed with a high level of roughage (Paula and Broderick Citation2020).
Table 3. Effects of nitrate (N), chitosan extract (CE) and shrimp shell meal (SSM) on in vitro degradability, pH, NH3-N and protozoa population.
As presented, the R:C ratio of 40:60 has attributed to the highest level of rumen NH3-N. This result could be supported by a high level of concentrate which could supply microbial activity to produce high NH3-N concentration than the data with a low ratio of concentrate mixture (Phesatcha et al. Citation2020). As stated by Wanapat and Pimpa (Citation1999), NH3-N concentration from 15 to 30 mg/dl would improve voluntary dry matter intake, nutrient degradability, and microbial protein synthesis, consequently. Furthermore, the addition of nitrate, chitosan extract and shrimp shell meal significantly lowered the rumen NH3-N concentration (P < 0.05). This decrease could be enhanced by a higher speed of absorption of NH3-N into the microbial protein process. Thus, a higher rumen by-pass would be expected.
3.5. Rumen volatile fatty acids and methane production
As shown in , no interactive result was found between the R:C ratio and feed additive supplementation on total VFA and individual VFA concentrations. The total VFA concentrations ranged from 45.2 to 55.8 mM; this result was similar to the values reported by Wang et al. (Citation2016). When the level of the concentrate mixture was increased, total VFA and C3 were enhanced, while the concentrations of rumen C2, and C2 to C3 ratios were subsequently reduced (P < 0.05). The data of nutrient degradability were increased, as well as total VFA and C3 proportion were significantly impacted, while the proportion and ratio of C2, C4 and C2 to C3 were subsequently decreased, respectively. This result was favourably supported by a concentrate addition, which composited highly degradable starch. A concentrate mixture containing high starch would result in more C3 production (Kang et al. Citation2017).
Table 4. Effects of nitrate (N), chitosan extract (CE) and shrimp shell meal (SSM) on in vitro volatile fatty acids (VFA) and methane (CH4) production.
No interactive result was found between the R:C ratio and supplementation of nitrate, chitosan extract and shrimp shell meal as related to the rumen CH4 production (). As shown, methane production was mitigated with the extent of an increased level of concentrate in total diets, particularly, having the R:C ratio at 40:60; these results were in agreement with the data reported earlier (Kang et al. Citation2017). This result could be due to an effect of a high concentration of C3 which was initiated by a reduction in the production of CH4. H2 from the CH4 pathway was then captured for C3 synthesis. Tong et al. (Citation2020) found that the supplementation of chitosan extract significantly increased the propionate proportion and markedly decreased the acetate proportion in in vitro gas production.
Under this experiment, CH4 production for nitrate, chitosan extract and shrimp shell meal supplementation resulted in lower volume than those in the control treatment. Goiri et al. (Citation2009) revealed that reduced rumen CH4 production by chitosan extract supplementation could be attributed to C3 production enhancement, which required the metabolic of hydrogen, and thus, reduced methanogenesis. Belanche et al. (Citation2016) showed that by simplifying the structure of the ruminal bacterial culture, chitosan extract inhibits methane production. By increasing rumen C3 and decreasing the C2 to C3 ratio, chitosan extract changed the rumen fermentation pattern. The reduction of gram positive bacteria could explain increased propionate production (Zanferari et al. Citation2018). When chitosan is used as a feed additive, changes in the molar proportions of VFA in the rumen were related to the efficiency of the use of metabolizable energy for growth (Kirwan et al. Citation2021 ).
4. Conclusions and recommendations
There were significant differences between R:C and among the supplementation sources. Apparently, there were no interactive effects. Among the three supplementation sources, shrimp shell meal showed the highest enhancement of rumen C3 concentration and the lowest protozoal population and methane production. However, further in vivo experiments should be conducted in order to elucidate more relevant data for further implementation.
Acknowledgments
Sincere thanks are extended to the Tropical Feed Resource Research and Development Center (TROFREC), Faculty of Agriculture, Khon Kaen University and the graduate students of Animal Science for their support of research facility and assistances.
Disclosure statement
No potential conflict of interest was reported by the author(s).
Additional information
Funding
References
- Anantasook N, Wanapat M. 2012. Influence or rain tree pod meal supplementation on rice straw based diets using in vitro gas fermentation technique. Asian-Australas. J. Anim. Sci. 25(3):325–334.
- AOAC. 1990. Official methods of analyses, 15th edn. Arlington, VA: Assoc. Offic. Anal. Chem.
- Belanche A, Pinloche E, Preskett D, Newbold CJ. 2016. Effects and mode of action of chitosan and ivy fruit saponins on the microbiome, fermentation and methanogenesis in the rumen simulation technique. FEMS Microbiol Ecol. 92:1–12.
- Benchaar C, Greahead D. 2011. Essential oils and opportunities to mitigate enteric methane emissions from ruminants. Anim Feed Sci Technol. 166-167:338–355.
- Benhabiles MS, Salah R, Lounici H, Drouiche N, Goosen MFA, Mameri N. 2012. Antibacterial activity of chitin, chitosan and its oligomers prepared from shrimp shell waste. Food Hydrocoll. 29:48–56.
- Blummel, M, Orskov, ER. 1993. Comparison of in vitro gas production and nylon bag degradability of roughage in predicting feed intake in cattle. Anim. Feed Sci. Technol. 40:109–119.
- Boadi D, Benchaar C, Chiquette J, Masse D. 2004. Mitigation strategies to reduce enteric methane emissions from dairy cows: update review. Can. J. Anim. Sci. 84:319–335.
- Ellis JL, Dijkstra J, Kebreab E, Bannink A, Odongo NE, McBride BW, France J. 2008. Aspects of rumen microbiology central to mechanistic modelling of methane production in cattle. J. Agric. Sci. 146:213–233.
- Galyen M. 1989. Laboratory procedures in animal nutrition research. Las Cruces, NM: New Mexico State University.
- Goiri I, Garcia-Rodriguez A, Oregui LM. 2009. Effect of chitosans on in vitro rumen digestion and fermentation of maize silage. Anim. Feed Sci. Technol. 148:276–287.
- Jeon YI, Kamil JYVA, Shahidi F. 2002. Chitosan as an edible invisible film for quality preservation of herring and Atlantic cod. J. Agric. Food Chem. 20:5167–5178.
- Joblin KN. 1999. Ruminal acetogens and their potential to lower ruminant methane emissions. Aust. J. Agric. Res. 50:1307–1314.
- Johnson KA, Johnson DE. 1995. Methane emissions from cattle. J. Anim. Sci. 73:2483–2492.
- Kang S, Wanapat M, Phesatcha K, Norrapoke T, Foiklang S, Ampapon T, Phesatcha B. 2017. Using krabok (Irvingia malayana) seed oil and Flimingia macrophylla leaf meal as a rumen enhancer in an in vitro gas production system. Anim. Prod. Sci. 57:327–333.
- Kirwan SF, Pierce KM, Serra E, McDonald M, Rajauria G, Boland TM. 2021. Effect of chitosan inclusion and dietary crude protein level on nutrient intake and digestibility, ruminal fermentation, and N excretion in beef heifers offered a grass silage based diet. Animals (Basel). 11:171.
- Leng RA. 2014. Interaction between microbial consortia in biofilm: a paradigm shift in rumen microbial ecology and enteric methane mitigation. Anim. Prod. Sci. 54:519–543.
- Makkar HPS, Blummel M, Becker K. 1995. In vitro effects and interaction between tannins and saponins and fate of tannins in the rumen. J. Sci. Food Agric. 69:481–493.
- McAllister TA, Newbold CJ. 2008. Redirecting rumen fermentation to reduce methanogenesis. Aust. J. Exp. Agric. 48:7–13.
- Moss AR, Jouany JP, Newbold J. 2000. Methane production by ruminants: its contribution to global warming. Ann. Zootech. 49:231–253.
- Orskov ER, McDonal I. 1979. The estimation of protein degradability in the rumen from incubation measurements weighted according to rate of passage. J. Agric. Sci. 92:499–503.
- Paula EM, Broderick GA. 2020. Effects of replacing soybean meal with canola meal for lactating dairy cows fed 3 different ratios of alfalfa to corn silage. J. Dairy Sci. 103(2):1463–1471.
- Phesatcha K, Phesatcha B, Wanapat M, Cherdthong A. 2020. Roughage to concentrate ratio and Saccharomyces cerevisiae inclusion could modulate feed digestion and in vitro ruminal fermentation. Vet. Sci. 7(151):1–13.
- Samuel M, Sagathevan S, Thomas J, Mathen G. 1997. An HPLC method for estimation of volatile fatty acids in ruminal fluid. Indian J. Anim. Sci. 67:805–807.
- Sar C, Mwenya B, Pen B, Takaura K, Morikawa R, Tsujimoto A, Kuwaki K, Isogai N, Shinzato I, Asakura Y, et al. 2005. Effect of ruminal administration of Escherichia coli wild type or a genetically modified strain with enhanced high nitrite reductase activity on methane emission and nitrate toxicity in nitrate-infused sheep. Br. J. Nutr. 94:691–697.
- SAS. 2013. User's guide: statistic, version 6, 12th ed.. Cary, NC: SAS Inst. Inc.
- Stelmock RL, Husby FH, Brundage AL. 1985. Application of Van Soest acid detergent fiber method for analysis of shellfish chitin. J. Dairy Sci. 68:1502–1506.
- Takahashi J, Young BA. 1991. Prophylactic effect of L-cysteine on nitrate-induced alterations in respiratory exchange and metabolic rate in sheep. Anim. Feed Sci. Technol. 35:105–113.
- Tilley JMA, Terry RA. 1963. A two stage technique for in vitro digestion of forage crops. J. Br. Grassl. Soc. 18:104–111.
- Tong JJ, Zhang H, Wang J, Yun L, Mao SY, Xiong BH, Jiang LS. 2020. Effect of different molecular weight of chitosan on methane production and bacterial community structure in vitro. J. Integr. Agric. 19(6):1644–1655.
- Van Soest PJ, Robertson JB, Lewis BA. 1991. Methods for dietary fiber neutral detergent fiber, and non-starch polysaccharides in relation to animal nutrition. J. Dairy Sci. 74:3583–3597.
- Wanapat M, Kongmun P, Poungchompu O, Cherdthong A, Khejornsart P, Pilajun R, Kaenpakdee S. 2012. Effects of plants containing secondary compounds and plant oils on rumen fermentation and ecology. Trop. Anim. Health. Prod. 44:399–405.
- Wanapat M, Pimpa O. 1999. Effect of ruminal NH3-N levels on ruminal fermentation, purine derivatives, digestibility and rice straw intake in swamp buffaloes. Asian-Australas. J. Anim. Sci. 12(6):904–907.
- Wang Z, He Z, Beauchemin KA, Tang S, Zhou C, Han X, Wang M, Kang J, Odongo NE, Tan Z. 2016. Evaluation of different yeast species for improving in vitro fermentation of cereal straws. Asian-Australas. J. Anim. Sci. 29:230–240.
- Zanferari F, Vendramini THA, Rentas MF, Gardinal R, Calomeni GD, Mesquita LG, Takiya CS. 2018. Effects of chitosan and whole raw soybeans on ruminal fermentation and bacterial populations, and milk fatty acid profile in dairy cows. J. Dairy Sci. 101:10939–10952.