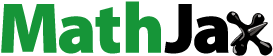
ABSTRACT
Surface temperature recording has shown promise in older pigs and in piglets but only under controlled conditions. The aim of this experiment was to compare surface temperatures using an infrared thermal camera (pointer), verified by the maximum temperature from the thermal images extracted by an algorithm (MATLAB®R2020), at the base, tip of ear, or eye, with rectal temperature under production conditions. The influence of bodyweight (BWC1; ≤0.80 kg, BWC2; 0.81 kg to 1.10 kg and BWC3; >1.10 kg), time post-partum (0 and 24 h) and colostrum intake (CI1; <200 g and CI2, ≥200 g) were investigated. Ear tip pointer temperatures showed low correlations with rectal temperature (r < 0.39; P = 0.20). Both the ear base and eye had moderate correlations (r = 0.53 and 0.55, respectively; P < 0.001 for both) to rectal temperature, and high correlations between pointer and extracted values (r = 0.73 and 0.82, respectively; P < 0.001 for both). At birth, eye temperatures had a moderate correlation with rectal temperature. The extracted eye, but not rectal or ear base, temperatures were higher (P = 0.01) in piglets consuming greater amounts of colostrum. In contrast to research settings, production conditions are highly variable and increase the inaccuracy of the surface temperature recordings. Therefore, overall, surface temperatures were not appropriate for commercial application in neonatal piglets.
Introduction
Body temperature is an important indicator of pig welfare and performance and an appropriate temperature is critical for neonatal piglets as they establish thermoregulation (Tucker et al. Citation2021). The non-invasive use of infrared cameras to record piglet body temperature is a growing area of research and of interest across multiple production animal species (Soerensen and Pedersen Citation2015). Non-contact temperature recording can potentially eliminate the stress often caused by other more invasive methods such as the measurement of rectal temperature, which may also result in the transfer of bacteria and other pathogens to the animal (Godyn and Herbut Citation2017). Currently, it is necessary to restrain neonatal animals to obtain their temperature rectally, which is the gold standard for measuring body temperature of young animals (Dewulf et al. Citation2003). Restraint can cause stress at a critical time for the neonate which can influence the validity of the temperature recorded leading to inaccurate interpretation of temperature changes (Magnani et al. Citation2011; Godyn and Herbut Citation2017). Further, obtaining body temperature using rectal thermometers can be stressful, potentially traumatic and interruptive to suckling, thus potentially disadvantaging the piglets. It has been suggested that infrared thermal technology could be used in pigs to detect surface temperatures (Shao and Xin Citation2008; Brown-Brandl et al. Citation2013; Zhang et al. Citation2019; Jorquera-Chavez et al. Citation2021). If applicable, this technology could be used on farm to detect at risk, lower viability piglets early for appropriate and timely intervention. A risk threshold similar to that suggested with rectal temperature could be established for surface temperature and be applied across production systems. However, most studies testing infrared thermal technologies have been performed with older pigs that have established thermoregulation and under different environmental conditions (Magnani et al. Citation2011). Further, most experiments have been conducted under tightly controlled environmental conditions which are not comparable to those of commercial production and, therefore, further studies are required to validate the use of this technology in commercial conditions and their applicability to the pork industry.
Previously, Chung et al. (Citation2010) suggested that infrared thermometry was a suitable alternative to rectal temperature under the conditions of a germ-free facility with no handling of experimental piglets, while others have also supported the utility of infrared thermometry but in a climate-controlled chamber (Mostaço et al. Citation2015). Under conditions more representative of commercial production, Schmid et al. (Citation2021) found that infrared ear thermometry could be used to assess temperature in pigs but with surface measures at the inner thigh and abdomen being best at estimating core temperature. However, with the need for significant animal manipulation, this provided no advantage over rectal thermometry. It is known that piglet temperatures change rapidly in the first 24 h of life and can be used as an indicator of likely survival (Mount Citation1959; Caldara et al. Citation2014; Sasaki et al. Citation2016; Santiago et al. Citation2019). Therefore, the development of a non-invasive protocol to measure piglet body temperatures in this period could be used commercially to predict early survival chance and allow early intervention for at-risk piglets. Surface temperature recorded at the base of the ear has recently been shown to have a moderate correlation to rectal temperature during the first 24 h, but with the poorest correlations being at birth and 24 h (Tucker et al., unpublished data). We hypothesized that under relatively uncontrolled commercial conditions, piglet surface temperature measured with a handheld infrared camera at birth and 24 h would not be concordant to rectal temperature and thus not appropriate as a replacement in production.
Methods
This experiment was conducted between January and March 2021 in a commercial piggery in southern New South Wales, in accordance with the Australian Code for the Care and Use of Animals for Scientific Purposes (National Health and Medical Research Council, Citation2013).
Animals and housing
The data set included 109 piglets (53 male and 56 female) born to 6 multiparous Large White x Landrace sows (parity 3.45 ± 1.16; 1–4). Total born litter sizes (14.9 ± 2.4) were recorded including born alive and stillborn but excluding mummified piglets. Sows were moved to their farrowing accommodation at 110 ± 1 d of gestation and housed in individual slatted floor farrowing crates (0.5 × 2.0 m). Each crate was equipped with a sow and piglet level nipple drinker, solid floored creep area located to the side of the crate, with a heat lamp positioned centrally over the creep area. The farrowing rooms were semi-enclosed with natural ventilation and a dripper cooling system set to automatically activate at 28°C. Additional portable evaporative coolers were placed in the shed at entry and used until 2 weeks after farrowing to aid in temperature control.
All sows farrowed naturally without induction and no sows required manual assistance. Fostering and piglet processing occurred after the experimental period.
Experimental design
At delivery of each piglet the time of birth was recorded, and the piglet was moved within 5 min of birth to a semi-enclosed plastic container mounted on a scale located behind the farrowing crate. Birth weight of each piglet was recorded, and thermal images were taken to measure the surface temperature at the base of the right ear, tip of the right ear and eye () with minimal additional manipulation (occasional repositioning of the ear was necessary to obtain access to the back of the ear and or eye when covered). All attempts were made to obtain the images without further manipulation or restraint of the piglet. The FLIR® E8 infrared thermal camera (FLIR Systems, Wilsonville, OR, U.S.A.; <0.06°C thermal sensitivity) with an emissivity value set to 0.985 was used at a 30 cm distance (using a generic ruler) from the piglet to record all surface temperatures. All images were saved for further analysis. Rectal temperature was recorded immediately after the images were obtained using a standard digital thermometer (0.3°C sensitivity) with a 32°C minimum limit. Piglets were then tagged and returned to the crate location they were picked up from. At 24 h after birth, piglets were reweighed, new thermal images obtained at all locations, and rectal temperature recorded (following the same procedures performed at birth). Any mortalities between birth and 24 h were recorded.
Figure 1. FLIR® E8 camera examples of thermal images of different surface locations obtained with the FLIR E8 camera, using the pointer. (a) = ear tip. (b) = base of ear. (c) = eye.
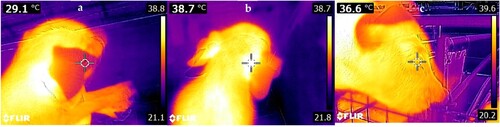
Infrared thermal images were analysed using MATLAB® R2020 (MathWorks Inc. Natick, MA, U.S.A.) and FLIR® Atlas SDK (FLIR Systems, Wilsonville, OR, U.S.A.; Jorquera-Chavez et al. Citation2021). The radiometric information of each image was extracted and the desired region of interest selected, which allowed extraction of the maximum temperature from this region. The algorithm extracted temperature will be referred to as the extracted value throughout this paper and the automatically generated value from the FLIR E8 camera will be referred to as the pointer value.
Statistical analysis
All statistical analyses were performed with SAS version 9.4 (Statistical Analysis Software, Cary, NC, U.S.A.). Level of significance was set at P < 0.05. Some data were manipulated prior to analysis:
The difference in surface temperature two temperature methods were calculated to create a new binomial variable (‘temperature agreeance’); agree = within 1°C, and disagree = ± > 1°C. This rule was applied to the following combinations:
- ear base; extracted - pointer
- eye; extracted – pointer
Piglets were categorized into three groups based on their birth weight: BWC1: ≤0.80 kg; BWC2: 0.81 kg to 1.10 kg; and BWC3: >1.10 kg. Colostrum intake for each piglet was calculated using the equation developed by Devillers et al. (Citation2007):
where CI = colostrum intake (g), W = piglet body weight at 24 h (kg), BW = piglet body weight at birth (kg), t = age (min), and tfs = time elapsed from birth to first sucking (min); tfs was assumed to be 30 min and t was 1440 min (24 h).
Piglets were also categorized into two groups based on their colostrum intake based on 200 g being the recommended minimum amount of colostrum needed (Quesnel et al. Citation2012) (CL1: <200 g, CL2: ≥200 g).
The PROC CORR procedure of SAS was used to estimate the correlation between rectal and surface temperature by both the camera pointer value and extracted values and between pointer and extracted values at respective locations. Pearson correlation coefficients and the respective 95% confidence intervals were the output. Correlation was deemed very high if r2 ≥ 0.90, high if r2 = 0.70–0.89, moderate if r2 = 0.50–0.69, low if r2 = 0.30–0.49, and negligible if r2 < 0.30 (Hue et al. Citation2021).
The effect of time and colostrum intake on ear base and eye temperatures (surface) from both the extracted and pointer were estimated using a mixed model in PROC MIXED, as presented in Equation (1):
(1)The effect of time and colostrum intake on rectal temperatures was estimated using a mixed model in PROC MIXED, as presented in Equation (2):
(2)where ls = litter size. Piglet sex, week born, sow parity, crate lamp on or off and BWC were tested but all were non-significant as covariables or fixed effects (P > 0.10). The outputs for all PROC MIXED procedures were the means and their respective standard errors.
The effect of time on the number of piglets that agreed within 1°C of surface temperature values was estimated using a mixed model in PROC GLIMMIX as presented in Equation (3):
(3)Piglet sex, litter size, week born, sow parity, crate lamp on or off and BWC were tested but all were non-significant as covariables (P > 0.10). The outputs were proportions and their respective 95% confidence intervals are represented in brackets.
Results
Correlation between surface and rectal temperatures
At the base of the ear, both the pointer and extracted surface temperatures were moderately correlated with rectal temperature (r = 0.51 and 0.53, respectively; P < 0.001 for both; (a)). At the tip of the ear, the pointer and the extracted surface temperatures had a negligible correlation with rectal temperature (r = 0.24; P = 0.064 and r = 0.18; P = 0.0004, respectively; (b)). The surface temperature of the eye showed a moderate correlation to rectal temperature for both the pointer and extracted values (r2 = 0.60 and 0.55, respectively; P < 0.001 for both; (c)).
Figure 2. Pearson’s correlation coefficient and respective 95% confidence intervals for Pointer (orange, r2p) and Extracted (blue, r2m) temperatures from thermal images taken using FLIR® E8 infrared thermal camera, between rectal temperature and locations in 109 piglets. (a) presents Ear base temperature, (b) presents Ear Tip temperature and (c) presents Eye temperature, measured by thermal imaging camera.
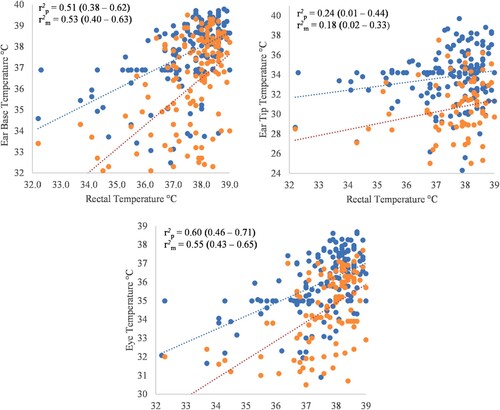
At 0 and 24 h, ear base temperature showed low and negligible correlation to rectal temperature for pointer values (r = 0.31; P = 0.03 and r = 0.22; P = 0.018 respectively) and low for the extracted values (r = 0.38; P = 0.007 and r = 0.30; P = 0.012, respectively: ). Ear tip temperature showed a moderate correlation to rectal temperature only for the pointer value at time 0 (r = 0.36; P = 0.131) and negligible for time 24 h (r = −0.07; P = 0.684) and extracted values at both time points (r = 0.19; P = 0.205 and r = 0.05; P = 0.726, respectively). Eye temperature showed a medium correlation between pointer and rectal temperatures at time 0 (r = 0.499; P = 0.0002) and negligible correlation at time 24 h (r = 0.138; P = 0.29; ). Similarly, the eye-extracted value had low correlation at time 0 (r = 0.47; P = 0.003) and negligible correlation at time 24 h (r = 0.211; P = 0.102). P values for most 24 h comparison of surface and rectal temperature showed non-significant results.
Table 1. Pearson’s correlation coefficient (r; 95% confidence intervals) for correlation between rectal temperature and surface temperature locations for camera generated pointer values and algorithm extracted values across time 0 (birth) and 24 h in 109 piglets. N = number of observations for each time grouping.
Ear tip was not used for further analysis due to its low correlation to rectal temperature and within surface temperature methods.
Pointer vs extracted surface temperatures
Ear base and eye temperatures showed a high correlation between pointer and extracted values (r = 0.73 and 0.82, respectively; P < 0.001 for both; (a,c)), while the ear tip showed a moderate correlation between pointer and extracted values (r = 0.52; P < 0.001; (b)).
Figure 3. Pearson’s correlation coefficient and respective 95% confidence intervals for the relationship between the Extracted temperature and Pointer temperature from thermal images taken using a FLIR® E8 infrared thermal camera at three locations in 109 piglets. (a) presents the base of the ear (purple), (b) presents the tip of the ear (green), (c) presents the eye (red).
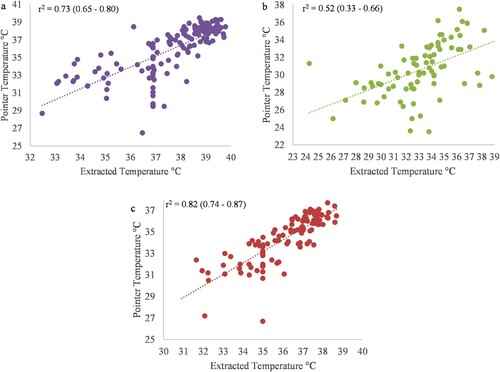
Factors affecting thermal surface temperature
Ear base temperatures were not significantly impacted by colostrum intake category (Extracted, P = 0.27; Pointer, P = 0.76) and were higher (P < 0.001) at 24 h than at birth ().
Table 2. Mean ± SEM for surface temperature extracted and pointer recorded values taken at the base of the ear and eye of 109 piglets between birth (0 h) and 24 h post birth and piglets who consumed less or more than 200 g of colostrum. N = number of piglets per group. Adjusted for litter size.
Pointer surface temperature of the eye was not significantly impacted by colostrum intake category (P = 0.48) but the extracted temperature was significantly higher in those piglets consuming ≥200 g of colostrum (P = 0.01; ). Both temperatures were higher in piglets at 24 h than at birth (P < 0.001).
Rectal temperatures were not significantly affected by colostrum intake (P = 0.29) but tended (P = 0.08) to be higher at 24 h than at birth ().
Table 3. Mean ± SEM for rectal temperature taken via digital thermometer of 109 piglets between birth (0 h) and 24 h post birth and piglets who consumed less than or greater than 200 g of colostrum. N = number of piglets per group. Adjusted for litter size.
Surface temperature agreeance between the pointer-derived value and the extracted value was only significantly impacted by time in one model (); at 24 h, the agreeance at the ear base between extracted and pointer values tended to be lower than at birth (P = 0.08).
Table 4. Proportion (95% confidence intervals) of piglets that temperatures agree within 1°C between pointer and the extracted surface temperatures for ear base and eye across time and colostrum intake categories for 109 piglets.
Discussion
Body temperature is a major indicator of a pig’s health, performance and potential for survival (Brown-Brandl et al. Citation2013; Jorquera-Chavez et al. Citation2020; Weng Citation2020; Jorquera-Chavez et al. Citation2021), and skin or eye surface temperatures have been suggested as appropriate alternatives to rectal temperature, allowing for less manipulation of the piglet at measurement (Soerensen and Pedersen Citation2015). However, this suggested relationship remained to be evaluated under commercial production conditions. Our data show that the base of the ear and the eye were the locations on the piglet that showed the best correlations between the thermal infrared camera pointer and extracted surface temperature values within the conditions of this study. However, their correlation to rectal temperature was still only moderate and thus these methods did not appear to be a good substitute for rectal temperature measurement under production conditions, supporting our hypothesis.
Unlike in previous studies where the thermal temperatures at the base of the ear and eye were reported to be highly correlated with rectal temperature (Soerensen and Pedersen Citation2015), our study showed that this seems not to be always accurate under production conditions. The extracted and pointer values for the base of the ear and eye locations were moderately correlated to rectal temperature. However, thermal temperature of the ear tip was not well correlated with rectal temperature using either pointer or the extracted values. This supports the previous findings by Tabuaciri et al. (Citation2012) who also showed that ear tip temperature had a lower correlation to rectal temperature than ear base of newborn piglets. This Anecdotally, the ear tip was the easiest to consistently access each time, but it was difficult to maintain it in the camera range without piglet manipulation. Other studies using temperature loggers which were directly attached to the tip of the ear showed greater promise and may be more appropriate for this location (Garrido-Izard et al. Citation2019). Production conditions are highly variable, as with this study design the shed was naturally ventilated assisted by a cooling system and the crates equipped with heat lamps which although allows some control on humidity and temperature are vastly different under tightly controlled research conditions. This would explain some of the variation within the results as well as the great differences between previous research outcomes from controlled trials to our study outcomes.
Rectal temperature was poorly correlated with surface temperature when considered with respect to time. In general, ear tip had the lowest correlation values followed by ear base and eye, although the confidence intervals were large for all correlations and, therefore, this variability may have affected the trend. However, the trend is supported by previous suggestions that the eye is the most sensitive to minor changes in temperature. Regardless, the lack of correlation at different time points supports our suggestion that, currently, measuring surface temperature under production conditions is not viable. Furthermore, the P values for our correlations to rectal temperature showed greater occurrence of non-significance in 24 h measures than time 0 regardless of surface location.
The ear base and eye are good thermal locations for surface temperature readings but obtaining clear and consistent images without piglet manipulation seems to be difficult. Our study showed a high correlation between these locations suggesting that base of ear and eye are comparable and could be used when a location e.g. the eye, is covered by an ear. Surface temperature has also been shown to be impacted by soiling, therefore, it would be reasonable to suggest the same or similar effect for wet piglets (Lengling et al. Citation2020). To reduce this effect, piglets could be dried. However, the effect of drying would need to be accounted for (Cooper et al. Citation2019). Further, it has been shown that stress can have a significant effect on the surface temperatures of the ear and eye (Magnani et al. Citation2011; Vinkers et al. Citation2013; Herborn et al. Citation2015) in pigs, among other species. When pigs were placed under a stress test, the temperature at the eye and right ear decreased after the test due to vasoconstriction (Magnani et al. Citation2011). This highlights that even the ‘best’ suggested locations for surface temperature are highly affected by environment and stressors, decreasing their validity under variable production conditions. It should be noted that the majority of pig temperature studies were primarily performed in older pigs of varying ages and reproductive stages with established energy reserves, unlike newborn piglets. In the first 24 h of life neonatal piglets are exposed to high levels of stress, like great environmental changes from in utero to the crate environment and competition for critical resources (Herpin et al. Citation2002; Caldara et al. Citation2014; Muns et al. Citation2016). With the known stress effect on surface temperature readings, it is reasonable to suggest that neonatal piglet surface temperature is less reliable and more effected by stress. Alternatively, it could be assumed that all neonatal piglets are exposed to high levels of stress and thus the effect on surface temperature is similar. However, it is well known that smaller piglets have lower energy reserves and are poorer thermoregulators (Herpin et al. Citation2002; Caldara et al. Citation2014; Cooper et al. Citation2019). Therefore, this subset of piglets is potentially under greater levels of stress and thus their temperature reading could be greatly impacted by stress. Currently, the only method of monitoring stress effects is measuring cortisol in saliva or faecal samples which, in newborn piglets, requires further handling or is impossible until colostrum is absorbed (Martínez-Miró et al. Citation2016; Wolf et al. Citation2020). Therefore, although this cannot be accounted for, it should be considered in all neonatal piglet temperature monitoring studies.
Temperature was significantly higher at 24 h for both ear base and eye extracted and pointer values than at birth. This was expected as, by 24 h, surviving piglets should have recovered from the initial post-natal temperature drop and, therefore, their internal temperature is likely to be higher and more stable. However, at 24 h, a greater number of piglet ear base extracted, and pointer values were not in agreeance than was evident at birth, although it should be noted that the confidence intervals were quite large. Some of this variation may be contributed by an increase in inaccurate location readings due to the piglets being more active at 24 h. It is also possible that some of the piglets have used up most of their energy supply and thus are conserving energy by reducing peripheral blood flow and so reducing the surface temperature (Charneca et al. Citation2010; Devillers et al. Citation2011; Tansey and Johnson Citation2015; Villanueva-Garcíaa et al. Citation2021). However, the constraints of this study did not allow for the activity of the piglets to be measured and accounted for. Piglets that suckled immediately prior to being measured or that were piling under the heat lamp may show surface temperatures higher than those who had not. Further research into the impact of, and methods, to account for piglet behaviour and activity are needed before these methods can truly be considered for production application.
An effect of difference in amount of estimated colostrum consumed was only evident in the eye temperatures, with an effect not being identified in the ear base data, due to it being more influenced by environmental factors than the eye temperature (reviewed by Soerensen and Pedersen Citation2015). Previous studies have shown that factors such as room temperature, heat source location, exposure duration and level, humidity, light exposure, hair coverage/density and distance of camera to pig, all impact the surface temperature and the cameras’ ability to record it (Soerensen and Pedersen Citation2015; Zhang et al. Citation2016; Schmid et al. Citation2021). This could suggest that at 24 h the piglets are affected by a greater variation in, and sources of, factors in their environment than at birth. Our results may also suggest that since, this experiment was performed in summer, less fluctuation between day conditions was likely to occur thus potentially reducing the variation between individual piglet temperatures at birth and 24 h. Additionally, within our study design the piglets were picked up and placed within a designated area to measure their temperatures, minimizing disturbance to the sow and creating a consistent environment for each image (Schmid et al. Citation2021). The piglets were not necessarily restrained during measurement but the movement outside of the crate to take the images would be unlikely to be replicated under production conditions. Therefore, greater environmental variation could occur, which might result in greater difficulty in obtaining repeatable image readings. Under research conditions, it is easier to account for and control for additional factors (Mostaço et al. Citation2015) but in production this is impractical. Further research is required to evaluate systems that potentially reduce variation discussed previously i.e. automatic monitoring systems to record infrared thermal surface temperature (Kammersgaard et al. Citation2013; Lu et al. Citation2018).
Conclusion
Infrared thermal images taken at the ear and eye in neonatal piglets at birth and 24 h did not appear to be an adequate replacement for rectal temperature (measured by conventional digital thermometer) under production conditions. However, ear base and eye infrared thermal temperatures were found to be in moderate to high agreeance at both birth and 24 h suggesting that these locations could be used interchangeably. The use of handheld thermal infrared technology to monitor piglet temperature change seems to be more appropriate for use in research than under production conditions. Future research and use of thermal infrared technology in production should be focused on a system that can automatically account for the changing environmental conditions and piglet activity levels and behaviours.
Author contributions
Conceptualization, B.S.T., M.CJ., R.N.K.; methodology, B.S.T., M.CJ., R.N.K., R.S.M., J.R.C.; validation, B.S.T., M.CJ., J.R.C., R.S.M., R.J.S., R.N.K.; formal analysis, B.S.T., J.R.C., K.R.P.; investigation, B.S.T, M.CJ., resources, R.S.M., R.J.S.; writing – original draft preparation, B.S.T.; writing – review and editing, all authors.; visualization, B.S.T.; supervision, J.R.C, RS.M., R.J.S., R.N.K. All authors have read and agreed to the published version of the manuscript.
Acknowledgements
The authors would like to thank the Rivalea Research and Innovation and farming staff for their assistance.
Data availability statement
Data available from senior author upon request.
Disclosure statement
No potential conflict of interest was reported by the author(s).
Correction Statement
This article has been corrected with minor changes. These changes do not impact the academic content of the article.
Additional information
Funding
References
- Brown-Brandl TM, Eigenberg RA, Purswell JL. 2013. Using thermal imaging as a method of investigating thermal thresholds in finishing pigs. Biostyt Eng. 114(3):327–333. doi:10.1016/j.biosystemseng.2012.11.015.
- Caldara FR, Dos Santos LS, Machado ST, Moi M, de Alencar Naas I, Foppa L, Garcia RG, de Kassia Silva Dos Santos R. 2014. Piglets’ surface temperature change at different weights at birth. Asian-Australas J Anim Sci. 27(3):431–438. doi:10.5713/ajas.2013.13505.
- Charneca R, Nunes J, Le Dividich J. 2010. Body composition and blood parameters of newborn piglets from Alentejano and conventional (large white × landrace) genotype. Span J Agric Res. 8(2):317–325. doi:10.5424/sjar/2010082-1192.
- Chung T-h, Jung W-s, Nam E-h, Kim J-h, Park S-h, Hwang C-Y. 2010. Comparison of rectal and infrared thermometry for obtaining body temperature of gnotobiotic piglets in conventional portable germ-free facility. Asian-Australas J Anim Sci. 23(10):1364–1368. doi:10.5713/ajas.2010.90507.
- Cooper N, Vande Pol KD, Ellis M, Xiong Y, Gates R. 2019. Effect of piglet birth weight and drying on post-natal changes in rectal temperature. J Anim Sci. 97(Supplement_2):4–4. doi:10.1093/jas/skz122.006.
- Devillers N, Farmer C, Le Dividich J, Prunier A. 2007. Variability of colostrum yield and colostrum intake in pigs. Animal. 1(7):1033–1041. doi:10.1017/S175173110700016X.
- Devillers N, Le Dividich J, Prunier A. 2011. Influence of colostrum intake on piglet survival and immunity. Animal. 5(10):1605–1612. doi:10.1017/S175173111100067X.
- Dewulf J, Koenen F, Laevens H, De Kruif A. 2003. Infrared thermometry is not suitable for the detection of fever in pigs. Vlaams Diergeneeskd Tijdschr. 72(5):373–379.
- Garrido-Izard M, Correa E-C, Requejo J-M, Villarroel M, Diezma B. 2019. Phase Space Analysis of Pig Ear Skin Temperature during Air and Road Transport. Appl. Sci. 9(24):5527. doi:10.3390/app9245527.
- Godyn D, Herbut P. 2017. Applications of continuous body temperature measurements in pigs – a review. Ann. Warsaw Unv. of Life Sci. - SGGW. Anim Sci. 56(2):209–220. doi:10.22630/AAS.2017.56.2.22.
- Herborn KA, Graves JL, Jerem P, Evans NP, Nager R, McCafferty DJ, McKeegan DEF. 2015. Skin temperature reveals the intensity of acute stress. Physiol Behav. 152(Pt A):225–230. doi:10.1016/j.physbeh.2015.09.032.
- Herpin P, Damon M, Le Dividich J. 2002. Development of thermoregulation and neonatal survival in pigs. Livest Prod Sci. 78(1):25–45. doi:10.1016/s0301-6226(02)00183-5.
- Hue DT, Williams JL, Petrovski K, Bottema CDK. 2021. Predicting colostrum and calf blood components based on refractometry. J Dairy Res. 88(2):194–200. doi:10.1017/s0022029921000340.
- Jorquera-Chavez M, Fuentes S, Dunshea F, Warner R, Poblete T, Unnithan R, Morrison R, Jongman E. 2021. Using imagery and computer vision as remote monitoring methods for early detection of respiratory disease in pigs. Comput Electron Agric. 187:106283. doi:10.1016/j.compag.2021.106283.
- Jorquera-Chavez M, Fuentes S, Dunshea FR, Warner RD, Poblete T, Morrison RS, Jongman EC. 2020. Remotely sensed imagery for early detection of respiratory disease in pigs: a pilot study. Animals (Basel). 10:3. doi:10.3390/ani10030451.
- Kammersgaard TS, Malmkvist J, Pedersen LJ. 2013. Infrared thermography – a non-invasive tool to evaluate thermal status of neonatal pigs based on surface temperature. Animal. 7(12):2026–2034. doi:10.1017/s1751731113001778.
- Lengling A, Alfert A, Reckels B, Steinhoff-Wagner J, Büscher W. 2020. Feasibility study on the use of infrared thermography to classify fattening pigs into feeding groups according their body composition. Sensors. 20:5221. doi:10.3390/s20185221
- Lu M, He J, Chen C, Okinda C, Shen M, Liu L, Yao W, Norton T, Berckmans D. 2018. An automatic ear base temperature extraction method for top view piglet thermal image. Comput Electron Agric. 155:339–347. doi:10.1016/j.compag.2018.10.030.
- Magnani D, Gatto M, Cafazzo S, Stelletta C, Morgante M, Nanni Costa L. 2011. Difference of surface body temperature in piglets due to the backtest and environmental condition. Animal hygiene and sustainable livestock production. Proceedings of the XVth International Congress of the International Society for Animal Hygiene Vienna, Austria. p. 1029–1032.
- Martínez-Miró S, Tecles F, Ramón M, Escribano D, Hernández F, Madrid J, Orengo J, Martínez-Subiela S, Manteca X, Cerón JJ. 2016. Causes, consequences and biomarkers of stress in swine: an update. BMC Vet Res. 12(1):171–171. doi:10.1186/s12917-016-0791-8.
- Mostaço GM, d KO, Miranda S, d ICF, Condotta S, Salgado DDA. 2015. Determination of piglets’ rectal temperature and respiratory rate through skin surface temperature under climatic chamber conditions. Eng Agricola. 35(6):979–989. doi:10.1590/1809-4430-Eng.Agric.v35n6p979-989/2015.
- Mount LE. 1959. The metabolic rate of the new-born pig in relation to environmental temperature and to age. J Physiol. 147(2):333–345. doi:10.1113/jphysiol.1959.sp006247.
- Muns R, Nuntapaitoon M, Tummaruk P. 2016. Non-infectious causes of pre-weaning mortality in piglets. Livest Sci. 184:46–57. doi:10.1016/j.livsci.2015.11.025.
- National Health and Medical Research Council (Australia). 2013. Australian code for the care and use of animals for scientific purposes Canberra: National Health and Medical Research Council. Canberra: National Health and Medical Research Council. https://www.nhmrc.gov.au/about-us/publications/australian-code-care-and-use-animals-scientific-purposes
- Quesnel H, Farmer C, Devillers N. 2012. Colostrum intake: influence on piglet performance and factors of variation. Livest Sci. 146(2-3):105–114. doi:10.1016/j.livsci.2012.03.010.
- Santiago PR, Martínez-Burnes J, Mayagoitia AL, Ramírez-Necoechea R, Mota-Rojas D. 2019. Relationship of vitality and weight with the temperature of newborn piglets born to sows of different parity. Livest Sci. 220:26–31. doi:10.1016/j.livsci.2018.12.011.
- Sasaki Y, Furusho K, Ushijima R, Tokunaga T, Uemura R, Sueyoshi MLS. 2016. Body surface temperature of suckling piglets measured by infrared thermography and Its association with body weight change. JARQ. 50:361–368. doi:10.6090/jarq.50.361.
- Schmid SM, Büscher W, Steinhoff-Wagner J. 2021. Suitability of different thermometers for measuring body core and skin temperatures in suckling piglets. Animals (Basel). 11(4).doi:10.3390/ani11041004
- Shao B, Xin H. 2008. A real-time computer vision assessment and control of thermal comfort for group-housed pigs. Comput Electron Agric. 62(1):15–21. doi:10.1016/j.compag.2007.09.006.
- Soerensen DD, Pedersen LJ. 2015. Infrared skin temperature measurements for monitoring health in pigs: a review. Acta Vet Scand. 57(1):5–5. doi:10.1186/s13028-015-0094-2.
- Tabuaciri P, Bunter K, Graser H-U. 2012. Thermal imaging as a potential tool for identifying piglets at risk, AGBU Pig Genetics Workshop Notes. Armidale, Australia: Animal Genetics and Breeding Unit, University of New England. p. 23–30.
- Tansey EA, Johnson CD. 2015. Recent advances in thermoregulation. Adv Physiol Educ. 39(3):139–148. doi:10.1152/advan.00126.2014.
- Tucker BS, Craig JR, Morrison RS, Smits RJ, Kirkwood RN. 2021. Piglet viability: a review of identification and pre-weaning management strategies. Animals (Basel). 11(10):2902. doi:10.3390/ani11102902.
- Villanueva-Garcíaa D, Mota-Rojasb D, Martínez-Burnesc J, Mora-Medinae P, Salmerónf C, Gómezb J, Boscatob L, Gutiérrez-Pérezf O, Cruzf V, Reyesb B, González-Lozanof M. 2021. Hypothermia in newly born piglets: mechanisms of thermoregulation and pathophysiology of death. J Anim Behav Biometeorol. 9:1–10. doi:10.31893/jabb.21001.
- Vinkers CH, Penning R, Hellhammer J, Verster JC, Klaessens JH, Olivier B, Kalkman CJ. 2013. The effect of stress on core and peripheral body temperature in humans. Stress. 16(5):520–530. doi:10.3109/10253890.2013.807243.
- Weng R-C. 2020. Variations in the body surface temperature of sows during the post weaning period and its relation to subsequent reproductive performance. Asian-Australas J Anim Sci. 33(7):1138–1147. doi:10.5713/ajas.19.0576.
- Wolf TE, Mangwiro N, Fasina FO, Ganswindt A. 2020. Non-invasive monitoring of adrenocortical function in female domestic pigs using saliva and faeces as sample matrices. PloS one. 15(6):e0234971. doi:10.1371/journal.pone.0234971.
- Zhang K, Jiao L, Zhao X, Dong D. 2016. An instantaneous approach for determining the infrared emissivity of swine surface and the influencing factors. J Therm Biol. 57:78–83. doi:10.1016/j.jtherbio.2016.03.003.
- Zhang Z, Zhang H, Liu T. 2019. Study on body temperature detection of pig based on infrared technology: a review. Artificial Intelligence in Agriculture. 1:14–26. doi:10.1016/j.aiia.2019.02.002.