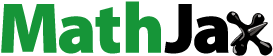
ABSTRACT
A 42-day experiment assessed the effects of phytase and a multicarbohydrase complex containing α-galactosidase (CAG) on broiler performance, processing yield, and nutrient digestibility. Exactly 2250-day-old male broilers were distributed in 90 floor pens and assigned to 1 of 9 treatments (10 replicates). A positive control sufficient in phosphorus (PC-P), a PC sufficient in metabolizable energy (PC-E), and a negative control (NC) with less phosphorus, calcium, and metabolizable energy were formulated. Manufactured using the NC, six additional treatments contained 500 or 1500 FTU/kg of phytase combined with 0, 0.1, or 0.2 g/kg of CAG. Broiler performance was determined on days 14, 28, and 42. Nutrient digestibility and processing yield was determined on days 42 and 43, respectively. Day 1–42 FCR was reduced and fillet yield increased when broilers consumed a 0.2 g/kg CAG diet instead of 0.1 g/kg (P < 0.05). Treatments supplemented with phytase alone or combined with CAG had a 42-day BW comparable to the PC-E and were heavier than the NC except for broilers fed 1500 FTU/kg of phytase and 0.1 g/kg of CAG (P < 0.05). Phytase supplementation alone or in combination with CAG in nutrient deficient diets can improve broiler growth and nutrient utilization.
Introduction
The beneficial impact of exogenous enzymes in monogastric nutrition has been extensively documented (Bedford Citation2000; Adeola and Cowieson Citation2011; Slominski Citation2011; Woyengo and Nyachoti Citation2011). However, further understanding of recently developed enzyme complexes and their influence on broiler production is needed. Phytases and non-starch polysaccharide (NSP) degrading enzymes are used commonly in the poultry industry, as they significantly reduce feed cost while maintaining or enhancing bird performance.
Phosphorus is a macro mineral whose main role is bone formation, nonetheless, it is also required for energy, carbohydrate, amino acid (AA), and fat metabolism, muscle coordination, blood chemistry, and transportation of fatty acids (Leeson and Summers Citation2001a; Humer et al. Citation2015). However, the majority of phosphorus in plant-based feedstuffs is bound to phytate. Phytate is an anti-nutritional factor (ANF) that can reduce broiler performance by chelating essential nutrients and reducing their bioavailability (Fernandes et al. Citation2019), or by stimulating gastric hypersecretion of hydrochloric acid and pepsin which could increase endogenous loss of protein (Cowieson et al. Citation2004; Selle et al. Citation2009).
Phytase (myo-inositol hexakisphosphate phosphohydrolase) catalyses the enzymatic break down of phytate (myo-inositol 1,2,3,4,5,6 hexakis dihydrogen phosphate) liberating inorganic phosphorus (Pallauf and Rimbach Citation1997). In addition, the hydrolytic phosphate splitting of phytic acid (IP6) to lower inositol phosphate esters (IP5 to IP1) can reduce its affinity for various nutrients (Pallauf and Rimbach Citation1997; Harland and Narula Citation1999). Phytase supplementation increases phytate-bound phosphorus utilization of plant-based feedstuffs (Humer et al. Citation2015; Fernandes et al. Citation2019). In addition, phytase can have an energy sparing effect that ranges from 0 to 120 kcal/kg of metabolizable energy (ME) depending on dietary energy, concentration of dietary phytate, phytase inclusion rate, and its intrinsic properties (Ravindran et al. Citation2006; Adeola and Cowieson Citation2011). Indeed, supplementation of phytase above 1500 or 2500 FTU/kg of the diet (Adeola and Cowieson Citation2011; Bedford and Rousseau Citation2017) can further increase ME liberation (Fernandes et al. Citation2019; Leyva-Jimenez et al. Citation2019).
Insoluble NSP can induce a cage-like effect and inhibit the digestion of carbohydrates, lipids, and proteins by reducing their exposure to enzymatic activity (Meng and Slominski Citation2005; Cowieson Citation2010; De Keyser et al. Citation2016). In addition, soluble NSP can have a high water-holding capacity which can decrease diffusion rates of substrates and endogenous enzymes and lead to increased intestinal viscosity, insufficient nutrient utilization, and sticky droppings (Bedford Citation2000; Slominski Citation2011). Insoluble and soluble arabinoxylans are among the most abundant NSP of corn cell wall structures. Thus, most commercial multicarbohydrase complexes contain xylanase as their key enzyme (Slominski Citation2011). However, there has been increasing interest in assessing other NSP degrading enzymes.
More recently, the efficacy of α-galactosidase has been investigated in broiler nutrition. Enzymes with α-galactosidase activity can hydrolyse raffinose family oligosaccharides (RFO), including stachyose and raffinose, which are α-1,6-galactosyl extensions of sucrose (Jackson Citation2010; Zhao et al. Citation2018). Soybean meal is a primary target for exogenous α-galactosidase activity as it contains between 6% and 12% stachyose and raffinose (Kidd et al. Citation2001a; Leeson and Summers Citation2001b; Jackson Citation2010) and monogastric animals lack intestinal production of α-galactosidase to fully metabolize these oligosaccharides (Gitzelmann and Auricchio Citation1965). Llamas-Moya et al. (Citation2021) conducted a meta-analysis compiling data from 78 independent broiler studies that utilized a multicarbohydrase containing α-galactosidase activity and determined that this enzyme strategy improved broiler performance and increased apparent metabolizable energy utilization by an average of 54 kcal/kg. In addition, previous research has shown that supplementing a combination of exogenous enzymes can have positive effects on broiler performance (Schramm et al. Citation2017; Lee et al. Citation2018; Wang et al. Citation2019). Cowieson (Citation2010) proposed that phytase and xylanase in combination could improve ileal digestible energy (IDE) of broilers by approximately 120 kcal/kg. Overall, diets supplemented with phytase and a carbohydrase have been shown to improve broiler performance (Wang et al. Citation2019; Ennis et al. Citation2020) and nutrient digestibility (Schramm et al. Citation2017; Mohiti-Asli et al. Citation2020).
Research investigating the efficacy of a multicarbohydrase containing α-galactosidase combined with varying levels of phytase on broiler performance, processing weight and yield, and nutrient digestibility is limited. Therefore, the objective of the current study was to evaluate the effects of phytase and a multicarbohydrase containing α-galactosidase (CAG) and their interactions on broiler performance, processing yields, and nutrient digestibility when fed a diet reduced in available phosphorus, calcium, and ME from 1 to 42 days of age.
Materials and methods
Animal care
This experiment was conducted at the Auburn University Charles C. Miller, Jr. Poultry Research and Education Center. All procedures involving live birds were approved by the Auburn University Institutional Animal Care and Use Committee (IACUC; PRN 2021-3850).
Bird management
A total of 2250 male day-old broiler chicks (YPM x Ross 708; Aviagen North America, Huntsville, AL) were obtained from a commercial hatchery. Upon arrival, chicks were weighed and distributed randomly among 90 floor pens (25 birds/pen; 0.11 m2/bird) in an environmentally controlled house equipped with exhaust fans, stir fans, forced-air heaters, evaporative-cooling pads, and an electronic control panel to manage temperature and ventilation. All floor pens contained fresh litter consisting of pine shavings, nipple drinkers (5 nipples/pen), and a hanging pan feeder. Feed and water were provided ad libitum. The lighting programme consisted of 24L:0D from 1 to 7 days, 21L:3D from 7 to 10 days, 20L:4D from 10 to 42 days of age. Environmental temperature was maintained at 33°C on day 1 and was gradually reduced to 18.3°C by 42 days of age. Birds and feed were weighed at 1, 14, 28, and 42 days of age to determine body weight (BW), feed intake (FI), and feed conversion ratio (FCR). Mortality and housing conditions were recorded daily and FCR was calculated including the weights of the mortalities.
Feed formulation, manufacture, and experimental design
Broilers were fed a three-phase feeding programme with no antibiotics. Broilers were provided 0.64 kg of starter feed (crumbles) from 1 to 14 days, 1.73 kg of grower feed (pellets) from 14 to 28 days, and ad libitum finisher feed (pellets) from 28 to 42 days of age. For all feeding phases, positive control (PC) and negative control (NC) diets were formulated following Aviagen recommendations for Ross 708 male broilers (Aviagen Citation2019a) and formulated at 96% of the AA recommendations (). A reduced AA density was used to account for the longer feeding time for each phase compared to Ross nutrition specifications (Aviagen Citation2019a).
Table 1. Ingredient and nutrient composition of dietary negative control (NC), positive control for energy (PC-E), and PC for phosphorus (PC-P) fed to YPM x Ross 708 male broilers from 1 to 42 days of age.
Each pen was randomly assigned to 1 of 9 treatments with 10 replicates. Three control treatments were used in this study, which consisted of a PC for phosphorus (PC-P), PC for energy (PC-E; industry standard), and a NC. Positive control for phosphorus met phosphorus requirements through the addition of inorganic phosphorus sources, and the PC-E was formulated to meet all ME recommendations and had a higher energy density (+100 kcal/kg feed) than all the other treatments. Additionally, the PC-E contained 500 FTU/kg (FTU = phytase units) of phytase as it was also formulated to contain 0.20% less calcium (Ca) and available phosphorus (P) compared to the PC-P. The NC diet was formulated to contain 0.20% less calcium and available phosphorus compared to the PC-P and 100 kcal/kg less ME compared to the PC-E at each feeding phase. The supplementation of phytase was expected to increase calcium and available phosphorus by 0.20%, while the supplementation of CAG was expected to increase energy utilization when included in the NC.
Six additional treatments were manufactured using the NC with different combinations of phytase and CAG. Three treatments were supplemented with 500 FTU/kg and inclusion of either 0, 0.1, or 0.2 g/kg of CAG, and three treatments supplemented with 1500 FTU/kg of phytase and inclusion of either 0, 0.1, or 0.2 g/kg of CAG. Enzyme activity of the phytase product was 10,000 FTU/g (Optiphos Plus, Huvepharma Inc., Peachtree City, GA). Additionally, the CAG was characterized as containing 3 major activities, which included α-galactosidase derived from Saccharomyces cerevisiae and carbohydrases (i.e. glucanase, cellulase, and xylanase) derived from Aspergillus niger and Trichoderma citrinoviride (AlphaGal 280P, Kerry, Ireland). This multienzyme system contained a minimum α-galactosidase activity of 8 and 300 U/g of xylanase activity.
Whole corn was ground with a hammermill (Model 11.5 × 38, Roskamp Champion, Waterloo, IA) equipped with a 4.76-mm screen. Phytase and CAG enzymes were premixed with 4.54 kg of ground corn using a countertop mixer (Model A-200, The Hobart Mfg. Co., Troy, OH), prior to their addition to the whole batch of feed. Feed ingredients were blended for 150 s (30 s dry cycle and 120 s wet cycle) using a twin shaft mixer (Model 726, Scott Equipment Co., New Prague, MN) to produce the mash diets. During grower and finisher feed manufacture, poultry fat was equally added in the mixer for all diets while the remaining fat was applied post pelleting using a liquid coating system (Mistcoater, Model no. 5TMX, APEC, Lake Odessa, MI). This was performed to account for the different energy levels among treatments and reduce pellet durability variations between treatments with different poultry fat inclusion. Diets were conditioned at 80°C for 40 s and pelleted through a 4.0-mm pellet die with an effective thickness of 36-mm using a pellet mill (Model 1112-4, California Pellet Mill Co., Crawfordsville, IN). Pellets were dried and cooled using a counter-flow pellet cooler (Model CC0909, California Pellet Mill Co., Crawfordsville, IN). Starter feed was crumbled in a crumbler with manual roll adjustment (Model 624SS, California Pellet Mill Co., Crawfordsville, IN).
During feed manufacture, feed samples were collected at evenly spaced intervals, and mixed to form a composite sample for subsequent nutrient and enzyme analysis. Crude protein (CP; AOAC official method 990.03), crude fat (aether extract; AOCS Standard Procedure Am 5-04), and minerals (modified procedure using Wolf et al. (Citation2003) and CEM Application Notes for Acid Digestion) were analysed by a commercial laboratory (Dairy One Forage Laboratory, Ithaca, NY). Gross energy (GE) of feed was determined by the Central Analytical Lab and Water Quality Lab (Fayetteville, AR). Feed samples were also sent to an external laboratory (Huvepharma BIOVET laboratory, Peshtera, Bulgaria) for phytase analysis using the ISO 30024:2009 (Animal feeding stuffs – Determination of phytase activity) method. Further enzyme analysis was conducted by an external laboratory (Kerry Global Technology and Innovation Centre, Naas, Ireland) for α-galactosidase activity using proprietary standard analytical methods.
Measurements
Broilers and feed were weighed on days 1, 14, 28, and 42 to determine BW, FI, and FCR. Birds were inspected twice daily and room temperature, bird condition, mortality, and availability of feed and water were monitored during each inspection. The BW of mortalities was used to adjust FCR.
An indigestible marker (0.5% titanium dioxide, TiO2) was added to the finisher feed (28–42 days of age) for determination of apparent ileal nutrient digestibility. On day 42, 5 birds per pen were selected randomly and euthanized by CO2 asphyxiation and cervical dislocation in accordance with American Veterinary Medical Association Guidelines (AVMA Citation2020) to collect ileal digesta and evaluate digestibility of protein, energy, fat, and minerals. Ileal digesta was collected starting 2 cm posterior from the Meckel’s diverticulum to 2 cm anterior from the ileal-cecal junction by gently squeezing out the ileal contents to provide sufficient sample (> 10 g freeze dried) for nutrient digestibility analysis. While squeezing out the ileal content, distilled water was used to aid in the removal of digesta. Ileal contents were pooled within individual pens. After collection, pooled ileal samples were stored on ice, transported to the laboratory, and frozen at −20°C until analysis. Upon analysis, ileal digesta samples were lyophilized in a Virtis Genesis Pilot Lyophilizer (SP Industries, Warminster, PA) and then ground using an electric coffee grinder (Capresso 560.4 Infinity, Montvale, NJ) on the finest setting. Thereafter, samples were sent to a commercial laboratory (Dairy One Forage Laboratory, Ithaca, NY) to be analysed for CP, aether extract, and minerals using methods mentioned previously. Additionally, feed and ileal samples were sent to the Central Analytical Lab and Water Quality Lab (Fayetteville, AR) for GE analysis. Single 0.75 g samples of feed and ileal digesta were analysed for GE using an isoperibol oxygen bomb calorimeter (model no. 6200, Parr Instruments, Moline, IA) standardized with benzoic acid. Titanium dioxide concentration of feed and ileal digesta was determined through procedures established by Short et al. (Citation1996). Apparent ileal digestibility (AID) of protein, energy, fat, and minerals were determined using an equation adapted from Stein et al. (Citation2007):
where (Nutrient/TiO2) = ratio of protein, energy, fat, or minerals to TiO2 in diet or ileal digesta. Percent GE digestibility values obtained from this equation were multiplied by the GE content of feed to determine apparent ileal digestible energy (AIDE) in units of kcal/kg on a dry matter basis (Gautier and Rochell Citation2020).
At 43 days of age, 10 birds per pen were selected randomly, wing banded, marked with a non-toxic food-grade dye, and processed for the determination of carcass characteristics. Feed was removed from each pen 12 h prior to processing. However, all birds had ad libitum access to drinking water during the feed removal period. The selected birds were placed in coops and transported to a processing plant. Birds were shackled, stunned electrically, exsanguinated, scalded, plucked, and eviscerated manually. After processing, carcasses were static chilled in slush ice (4°C) for 3 h before chilled carcass weights were determined. After chilling, carcasses were placed on a rack to allow water to drip for 3 min and then were weighed for determination of carcass weight and yield. Thereafter, carcasses were stored in slush ice for 16 h. Afterwards, all carcasses were deboned utilizing stationary cones to determine total breast fillet (Pectoralis major), tenders (Pectoralis minor), wings, and legs weight and their corresponding yield. Carcass yield was calculated relative to 43-day live weight, while breast, tenders, wings, and legs yield were calculated relative to chilled carcass weight.
Statistical analyses
Data were analysed as a randomized complete block design using pen location as blocking factor. Each treatment was represented by 10 replicate pens with pen considered as the experimental unit. Processing yield and nutrient digestibility data were subjected to arcsine transformation before statistical analysis. Data were analysed as a one-way ANOVA using the GLM procedure of JMP software (SAS Citation2010). Least square means among the 9 treatments were compared using Tukey’s HSD procedure with statistical significance considered at P ≤ 0.05. Tendencies were considered at 0.05 < P < 0.10.
In addition, data were analysed as a 2 × 3 factorial with 2 levels of phytase (500 and 1500 FTU/kg) and 3 levels of CAG (0, 0.1, and 0.2 g/kg) to evaluate the main effects of each enzyme and their interactions. Excluded from this factorial analysis were the NC, PC-E, and PC-P diets. Data were analysed using the GLM procedure of JMP software (SAS Citation2010). Least square means were compared using Tukey’s HSD procedure with statistical significance considered at P ≤ 0.05. Tendencies were considered at 0.05 < P < 0.10. Independent orthogonal contrasts were performed for grouped comparison between enzyme supplemented diets (all NC + 500 FTU/kg and all NC + 1500 FTU/kg) and the NC, PC-E, and PC-P.
Results
Feed and enzyme analysis
Diets without phytase supplementation had less than 90 FTU/kg across all feeding phases. Phytase recoveries for diets containing 500 FTU/kg were between 83% to 118%, 97% to 129%, and 96% to 125% during starter, grower, and finisher diets, respectively. Additionally, phytase recoveries for diets containing 1500 FTU/kg during starter, grower, and finisher periods were between 99% to 113%, 91% to 98%, and 85% to 109%, respectively. As for CAG analysis, diets without CAG supplementation had 0 U/kg across the different feeding phases. Enzyme recovery for diets containing 0.1 g/kg of CAG were not determined because the dose rate was below the assay’s limits of detection. However, CAG recovery for diets containing 0.2 g/kg were an average of 101, 111, and 102% for starter, grower, and finisher diets, respectively.
Broiler performance
Treatment effects on broiler performance. Dietary treatments influenced broiler performance during the entire experimental period (). Broilers fed the NC had the lowest day 14 BW (P < 0.001) compared to the other treatments, but similar to the PC-P. On day 28, NC broilers had the lowest BW compared to the remaining treatments (P < 0.001). On day 42, broilers fed the PC-E were 7.5 and 3.9% heavier than birds fed the NC and NC + 1500 FTU/kg of phytase and 0.1 g/kg of CAG, respectively (P < 0.001). Feed intake was influenced between day 1–14 (P = 0.025) and 1–28 (P = 0.041); however, only a tendency was observed between day 1–42 (P = 0.051). Feed conversion ratio was improved between day 1–14 when broilers were fed the PC-E, NC + 500 FTU/kg of phytase and 0.2 g/kg of CAG, or 1500 FTU/kg of phytase (regardless of the CAG level) compared to broilers fed the NC (P < 0.001). Broilers fed the PC-E had approximately a 7-point lower FCR from day 1–28 compared to broilers fed the NC (P < 0.001). Additionally, broilers fed diets containing 1500 FTU/kg of phytase and either 0, 0.1, or 0.2 g/kg of CAG had a similar FCR compared to the PC-E from 1 to 28 days. Broilers fed the PC-E had 5-points improved FCR compared to NC from 1 to 42 d. Furthermore, broilers fed a diet with 500 or 1500 FTU/kg of phytase in combination with 0.2 g/kg of CAG had similar day 1–42 FCR compared to broilers fed the PC-E, and a 1.6- and 3.0-point lower FCR compared to the non-CAG supplemented groups, respectively.
Table 2. Performance of YPM x Ross 708 male broilers provided diets varying in phytase and a multicarbohydrase containing α-galactosidase (CAG) from 1 to 42 days of age.
P-values of orthogonal contrasts for broiler performance are shown in . When grouped, broilers fed a nutrient reduced diet with 500 FTU/kg of phytase and CAG (0, 0.1, or 0.2 g/kg) had a higher BW and FI during the entire study with a lower FCR from d 1–14 and 1–28 compared to the NC (P < 0.05). As for broilers fed a nutrient reduced diet with 1500 FTU/kg of phytase and CAG (0, 0.1, or 0.2 g/kg), they had higher BW during the entire study, tended to have a higher FI from d 1–14 and 1–42, and had a lower FCR from 1 to 14 and 1–28 compared to the NC (P < 0.05). Broilers fed the PC-E had a higher d 42 BW and a lower FCR from d 1–28 and 1–42 compared to broilers in the 500 and 1500 FTU/kg enzyme supplemented groups (P < 0.05). Additionally, broilers consuming the PC-E had a lower d 1–14 FCR compared to the 500 FTU/kg enzyme supplemented groups (P < 0.05). Broilers in the 500 FTU/kg enzyme supplemented groups had a higher d 14 BW and d 1–14 FI compared to broilers fed the PC-P (P < 0.05). As for the 1500 FTU/kg enzyme supplemented groups, they had a higher d 14 BW and lower FCR throughout the entire study compared to the PC-P group (P < 0.05). Lastly, broilers fed the PC-P had a higher FI from d 1–42 compared to the 1500 FTU/kg enzyme supplemented groups (P < 0.05).
Table 3. P-valuesTable Footnote1 of orthogonal contrasts for broiler performance of YPM x Ross 708 male broilers provided diets varying in phytaseTable Footnote2 and a multicarbohydrase containing α-galactosidase (CAG)Table Footnote3.
Main effects and interactions on broiler performance. shows the main effects and interactions of phytase and CAG on broiler performance. There were no interactions between phytase and CAG on broiler performance (P > 0.05). Broilers fed 500 or 1500 FTU/kg of phytase had similar BW during the entire experimental period (P > 0.05). Day 1–14 and 1–42 FI and FCR were not influenced by phytase supplementation (P > 0.05). However, between day 1–28, FI was 2.2% higher when broilers were fed diets with 500 FTU/kg of phytase (P = 0.014), whereas FCR was 2-points lower when broilers were fed diets with 1500 FTU/kg (P < 0.001). From day 1–42, broilers fed diets with 1500 FTU/kg of phytase tended (P = 0.078) to have lower FCR (1.539 vs. 1.554 g:g) compared to broilers fed diets with 500 FTU/kg of phytase. Inclusion of CAG at 0, 0.1, or 0.2 g/kg did not influence BW or FI during the entire grow out. However, broilers fed 0.2 g/kg of CAG had lower FCR (P = 0.018) than birds fed diets with 0 or 0.1 g/kg of CAG.
Table 4. Performance of YPM x Ross 708 male broilers provided diets varying in phytase at 500 or 1500 FTU/kg and a non-starch polysaccharide degrading enzyme (CAG) at 0, 0.1, or 0.2 g/kg from 1 to 42 days of age analysed as a 2 × 3 factorial (phytase x CAG).
Processing weight and yield
Treatment effects on broiler processing weight and yield. shows the treatment effects on processing characteristics of broilers reared to 42 days of age. The experimental treatments did not influence chilled carcass yield, tender yield, wing yield, or leg weight and yield (P > 0.05). Broilers fed the PC-E had higher (P < 0.001) chilled carcass weight compared to broilers fed the NC (2832 vs. 2699 g).
Table 5. Carcass and breast meat yields of YPM x Ross 708 male broilers provided diets varying in phytase and a multicarbohydrase containing α-galactosidase (CAG) from 1 to 42 days of age.
Fillet yield was increased 3.4 and 2.7% when broilers were fed the NC + 1500 FTU/kg of phytase and 0.2 g/kg of CAG compared to broilers fed the NC and PC-E, respectively (30.5 vs. 29.5 and 29.7%; P < 0.001). Additionally, broilers fed the NC + 1500 FTU/kg of phytase or NC + 500 FTU/kg of phytase and 0.2 g/kg of CAG had higher fillet yields compared to broilers fed the NC (P < 0.001). Tender weight was increased 8.4 and 7.7% when broilers consumed the NC + 500 FTU/kg of phytase and 0.2 g/kg of CAG and NC + 1500 FTU/kg of phytase and 0.1 g/kg of CAG, respectively, compared to the NC (168 and 167 vs. 155 g; P = 0.009). Broilers that consumed the NC had 4% lower wing weights (P = 0.018) compared to broilers fed the PC-E, NC + 500 FTU/kg of phytase and 0.2 g/kg of CAG, or NC + 1500 FTU/kg of phytase with the other treatments being intermediate.
P-values of orthogonal contrasts for processing weight and yield are shown in . When grouped, broilers fed a reduced nutrient diet with 500 FTU/kg of phytase and CAG (0, 0.1, or 0.2 g/kg) and broilers fed a reduced nutrient diet with 1500 FTU/kg of phytase and CAG (0, 0.1, or 0.2 g/kg) had a higher chilled carcass weight, fillet weight, fillet yield, tender weight, wing weight, and leg weight compared to broilers fed the NC diet (P < 0.05). When compared to the PC-E, both the 500 and 1500 FTU/kg enzyme supplemented groups had higher fillet yields with the 1500 FTU/kg group also having a higher leg yield (P < 0.05). There were no processing weight and yield differences between the PC-P and either enzyme supplemented groups (P > 0.05).
Table 6. P-valuesTable Footnote1 of orthogonal contrasts for carcass and breast meat yields of YPM x Ross 708 male broilers provided diets varying in phytaseTable Footnote2 and a multicarbohydrase containing α-galactosidase (CAG)Table Footnote3.
Main effects and interactions on processing weight and yield. Main effects and interactions of phytase and CAG on processing characteristics of broilers can be observed in . There were no phytase main effects observed between 500 or 1500 FTU/kg for carcass or breast meat yields (P > 0.05). Main effects of CAG were not observed between 0, 0.1, and 0.2 g/kg for chilled carcass, tender, wing, or leg weight and yield (P > 0.05). However, CAG did influence fillet weight and yield. Broilers fed 0.2 g/kg of CAG had 2.9 and 1.5% higher fillet weight (859 vs. 835 g; P = 0.030) and yield (30.5 vs. 30.1%; P = 0.045), respectively, compared to broilers fed 0.1 g/kg. There were no interactions observed between phytase and CAG on broiler carcass and breast meat characteristics (P > 0.05).
Table 7. Carcass and breast meat yields of YPM x Ross 708 male broilers provided diets varying in phytase at 500 or 1500 FTU/kg and a non-starch polysaccharide degrading enzyme (CAG) at 0, 0.1, or 0.2 g/kg from 1 to 42 days of age analysed as a 2 × 3 factorial (phytase x CAG).
Nutrient digestibility
Treatment effects on nutrient digestibility. shows the treatment effects on broiler nutrient digestibility. Broilers that consumed a diet with 0.2 g/kg of CAG with either 500 or 1500 FTU/kg of phytase had a 4.7 and 6.9% higher CP digestibility compared to the NC (P = 0.002), respectively. In addition, broilers fed the PC-E or PC-P had around a 5% increase in CP digestibility compared to the NC (P = 0.002). Broilers fed the NC + 500 FTU/kg of phytase and NC + 500 FTU/kg phytase and 0.2 g/kg of CAG had higher fat digestibility compared to broilers fed the NC + 1500 FTU/kg of phytase and 0.1 g/kg of CAG (93.2 and 92.3 vs. 85.7%; P < 0.001). Calcium digestibility was increased 34.9, 23.5, 27.2, and 26.2% when broilers were fed the NC + 500 FTU/kg of phytase, NC + 500 FTU/kg of phytase and 0.1 g/kg of CAG, NC + 1500 FTU/kg of phytase, and NC + 1500 FTU/kg of phytase and 0.2 g/kg of CAG, respectively, compared to broilers fed the PC-P (P < 0.001). Broilers fed the NC had a 29.4% higher calcium digestibility compared to broilers fed the PC-P. Phosphorus digestibility was lowest in broilers fed NC (47.4%) and PC-P (55.9%) (P < 0.001), which were the diets that did not contain phytase. In addition, broilers fed a diet with 500 or 1500 FTU/kg of phytase had an average of 24.4 and 34.8% higher phosphorus digestibility than the PC-P, respectively (P < 0.05). Regardless of CAG supplementation, phosphorus digestibility was improved an average of 46.7 and 59.1% when broilers were fed a diet with 500 or 1500 FTU/kg of phytase compared to the NC, respectively (P < 0.001). Broilers fed diets supplemented with 1500 FTU/kg of phytase and 0.2 g/kg of CAG had higher (P < 0.05) AIDE than NC and PC-P treatments (3585 vs. 3254 and 3416 kcal/kg, respectively). A higher AIDE was observed when broilers were fed the PC-P compared to the NC (P < 0.05) although both diets had similar ME and no enzyme supplementation. Additionally, broilers fed a diet with exogenous enzyme supplementation (phytase alone or combined with CAG) had comparable AIDE to broilers fed the PC-E (P > 0.05).
Table 8. Apparent ileal nutrient digestibility of YPM x Ross 708 male broilers provided diets varying in phytase and a multicarbohydrase containing α-galactosidase (CAG) from 28 to 42 days of age.
P-values of orthogonal contrasts for nutrient digestibility are shown in . When grouped, broilers fed a reduced nutrient diet with 500 FTU/kg of phytase and CAG (0, 0.1, or 0.2 g/kg) had a higher CP, fat, and phosphorus digestibility, and AIDE compared to broilers fed the NC (P < 0.05). As for broilers fed a reduced nutrient diet with 1500 FTU/kg of phytase and CAG (0, 0.1, or 0.2 g/kg), they had a higher CP digestibility, phosphorus digestibility, and AIDE compared to the NC group (P < 0.05). Broilers fed the NC had a higher calcium digestibility compared to broilers fed the 1500 FTU/kg enzyme supplemented treatments (P < 0.05). When compared to the PC-E, broilers fed the 500 FTU/kg enzyme supplemented treatments had a higher calcium digestibility (P < 0.05). Broilers fed the PC-E tended to have a higher fat digestibility compared to the 1500 FTU/kg enzyme supplemented treatments, whereas broilers fed the 1500 FTU/kg enzyme supplemented treatments tended to have a higher calcium and phosphorus digestibility (0.05 < P < 0.10). When compared to the PC-P, both the 500 and 1500 FTU/kg enzyme supplemented groups had a higher calcium digestibility, phosphorus digestibility, and AIDE with the 500 FTU/kg group also having a higher fat digestibility (P < 0.05).
Table 9. P-valuesTable Footnote1 of orthogonal contrasts for apparent ileal nutrient digestibility of YPM x Ross 708 male broilers provided diets varying in phytaseTable Footnote2 and a multicarbohydrase containing α-galactosidase (CAG)Table Footnote3.
Main effects and interactions on nutrient digestibility. shows the main effects and interactions of phytase and CAG on nutrient digestibility of broilers. No main effects between 500 and 1500 FTU/kg of phytase were observed for CP and calcium digestibility or AIDE (P > 0.05). Fat digestibility was increased 4% when broilers were fed 500 FTU/kg compared to 1500 FTU/kg (92.0 vs. 88.4%; P = 0.002). Broilers fed 1500 FTU/kg of phytase; however, had an 8.2% higher phosphorus digestibility compared to broilers fed 500 FTU/kg (75.8 vs. 70.0%; P < 0.001). The level of CAG did not influence CP digestibility, phosphorus digestibility, or AIDE (P > 0.05). Fat digestibility increased 4.6 and 4.9% when broilers were fed 0 or 0.2 g/kg of CAG compared to broilers fed 0.1 g/kg (P = 0.007). A 16% increase in calcium digestibility was observed when broilers were fed diets without CAG compared to broilers fed diets with 0.1 g/kg (P = 0.007). Phytase x CAG interactions were not observed for CP, fat, and phosphorus digestibility (P > 0.05). However, there was an interaction for calcium digestibility () and AIDE (). Calcium digestibility was improved by 29.7% when broilers were only fed 500 FTU/kg of phytase compared to broilers fed a combination of 1500 FTU/kg and 0.1 g/kg of CAG (P = 0.047). Lastly, there was a significant interaction for AIDE except Tukey’s HSD was unable to separate the means. Broilers fed a combination of 1500 FTU/kg of phytase and 0.1 g/kg of CAG had the lowest AIDE (3463 kcal/kg) compared to remaining enzyme combinations (P = 0.042).
Figure 1. Interaction effects of varying inclusion rates of phytase (Optiphos Plus1) at 500 or 1500 FTU/kg and a non-starch polysaccharide degrading enzyme (AlphaGal 280P2) at 0, 0.1, or 0.2 g/kg fed to YPM x Ross 708 male broilers on calcium digestibility from 28 to 42 days of age. Notes: P = 0.047. Standard error of the mean = 2.13%. a,bMeans with different superscripts differ significantly (P < 0.05). 1OptiPhos Plus (Huvepharma Inc., Peachtree City, GA) included at 500, or 1500 FTU/kg of diet. 2AlphaGal 280P (Kerry, Ireland) included at 0, 0.1 and 0.2 g supplement/kg of diet.
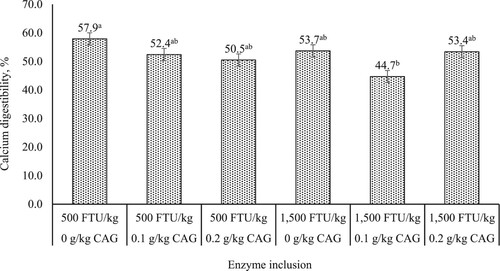
Figure 2. Interaction effects of varying inclusion rates of phytase (Optiphos Plus1) at 500 or 1500 FTU/kg and a non-starch polysaccharide degrading enzyme (AlphaGal 280P2) at 0, 0.1, or 0.2 g/kg fed to YPM x Ross 708 male broilers on apparent ileal digestible energy3 (AIDE) from 28 to 42 days of age. Notes: P = 0.042 (overall model was significantly different; however, Tukey’s HSD was unable to separate the means). Standard error of the mean = 30 kcal/kg. 1OptiPhos Plus (Huvepharma Inc., Peachtree City, GA) included at 500, or 1500 FTU/kg of diet. 2AlphaGal 280P (Kerry, Ireland) included at 0, 0.1 and 0.2 g supplement/kg of diet. 3On a dry matter basis.
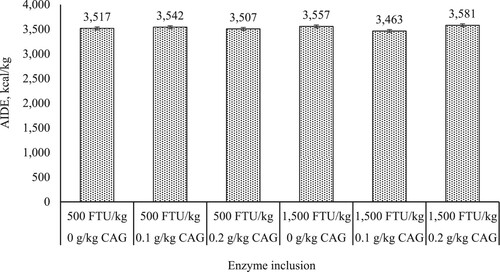
Table 10. Apparent ileal nutrient digestibility of YPM x Ross 708 male broilers provided diets varying in phytase at 500 or 1500 FTU/kg and a non-starch polysaccharide degrading enzyme (CAG) at 0, 0.1, or 0.2 g/kg from 28 to 42 days of age analysed as a 2 × 3 factorial (phytase x CAG).
Discussion
Broiler performance
Treatment effects on broiler performance. Broilers fed the NC diet without enzyme supplementation had depressed growth performance compared to remaining treatments. However, broilers fed the NC from 1 to 42 days of age had a 13.1 and 14.0% higher BW and FI, respectively, with around a 1-point reduction in FCR compared to the YPM x Ross 708 performance objectives (Aviagen Citation2019b). This indicates that broilers in this experiment were able to efficiently utilize nutrients in the available phosphorus, calcium, and ME reduced diet.
Supplementation of multiple exogenous enzymes has been suggested to have synergistic effects on broiler growth (Ennis et al. Citation2020). Commonly, phytase and NSP degrading enzymes have been combined to increase broiler performance by reducing anti-nutritional factors of plant-based feed ingredients (e.g. phytate and NSP) and by increasing availability of otherwise bound nutrients (Adeola and Cowieson Citation2011; Slominski Citation2011). More specifically, NSP are found in the cell wall and surround a portion of phytate molecules that are located within plant cells (Woyengo and Nyachoti Citation2011). Non-starch polysaccharide degrading enzymes can degrade the aleurone layer making it more permeable, which increases the surface area of exposed phytate and improves phytase accessibility (Woyengo and Nyachoti Citation2011). Increased cell wall permeability of the aleurone layer where phytic acid is stored could lead to synergistic effects when both phytase and NSP degrading enzymes are supplemented in the diet (Parkkonen et al. Citation1997; Adeola and Cowieson Citation2011). In agreement with the present study, Ennis et al. (Citation2020) reported an improvement on BW of broilers fed a nutrient deficient diet supplemented with a combination of 1500 FTU/kg phytase and xylanase, to a level comparable with broilers fed a nutrient sufficient diet from 1 to 44 days of age. Similarly, Wang et al. (Citation2019) reported that broilers fed 500 FTU/kg of phytase and a multicarbohydrase had an 8% higher body weight gain (BWG) compared to broilers fed exclusively 500 FTU/kg of phytase from 1 to 21 days of age.
Regarding FI, similar findings were reported by Woyengo et al. (Citation2010) where broilers reared from 1 to 21 days of age had lower FI when fed a nutrient reduced diet compared to broilers fed a diet supplemented with phytase and multicarbohydrase. In contrast, other researchers did not report an influence on FI when combining phytase and NSP degrading enzymes. Karimi et al. (Citation2013) fed broilers varying combinations of phytase and xylanase during an 18-d study and reported no influence on FI; however, they did observe a difference when enzymes were fed separately. Mohiti-Asli et al. (Citation2020) observed no difference in average daily FI when broilers were fed a PC, NC, or a diet supplemented with phytase and a multicarbohydrase with α-galactosidase, xylanase, and β-glucanase activities during a 42-day experiment.
The effect of the combination of exogenous enzymes on FCR has been evaluated by various research groups. Poernama et al. (Citation2021) observed an improvement in FCR when broilers were fed diets containing phytase and a multicarbohydrase (containing xylanase and β-glucanase activities) over broilers fed diets containing phytase alone. Similarly, Lee et al. (Citation2018) observed a 12-point improvement in FCR when broilers were fed diets containing a combination of xylanase and 2500 FTU/kg of phytase compared to broilers fed a diet with lower or no enzyme supplementation between 1 and 42 days of age. On the contrary, Ennis et al. (Citation2020) observed an increase on FCR of at least 2 points when broilers were provided a diet reduced in calcium (0.145%), available phosphorus (0.145%), and AME (100 kcal/kg) supplemented with phytase and NSP degrading enzymes in comparison to broilers fed an energy sufficient diet from 1 to 55 days of age. However, broilers fed 1500 FTU/kg of phytase and xylanase had a similar day 1–44 FCR compared to broilers fed the energy sufficient diet.
Main effects and interactions on broiler performance. Broiler performance can be improved with the supplementation of phytase by reducing anti-nutritional effects of phytate and increasing nutrient availability (Pallauf and Rimbach Citation1997; Humer et al. Citation2015; Fernandes et al. Citation2019). Several studies have reported performance benefits of phytase supplementation in broiler diets (Scholey et al. Citation2018; Sommerfeld et al. Citation2018; Broch et al. Citation2020). However, in the present study, BW was not influenced by increasing rates of phytase. Similarly, Gulizia et al. (Citation2022) reported similar BW and FCR between broilers fed diets with 500, 1000, and 2000 FTU/kg of phytase during a 49-d study. Nonetheless, Karimi et al. (Citation2013) fed broilers 500, 1000, 1500, and 2000 FTU/kg of phytase and reported an increase in BW with increasing inclusion rates of phytase. In the present study, broilers fed a diet with 500 FTU/kg of phytase had a higher d 1–28 FI compared to broilers fed a diet with 1500 FTU/kg. Previous research indicates that FI is not consistently influenced by different phytase inclusion rates (Walk et al. Citation2014; Walters et al. Citation2019; Ennis et al. Citation2020). During early growth periods, broilers can have a higher FI with lower inclusion rates of phytase (Ennis et al. Citation2020), higher FI with higher inclusion rates of phytase (Walters et al. Citation2019), or their FI can remain unchanged (Walk et al. Citation2014).
Llamas-Moya et al. (Citation2020) fed broilers a diet supplemented with CAG (0.2 g/kg) from 1 to 35 days of age and reported a 3.2% and 6-point improvement in BW and FCR, respectively, compared to broilers fed diets without enzyme supplementation. However, they did not observe an effect from CAG on FI, which agrees with the present study. Early research conducted by Kidd et al. (Citation2001b) indicated that broilers fed a diet supplemented with α-galactosidase from 1 to 42 days of age had improved FCR compared to broilers fed diets without enzyme supplementation. However, Jasek et al. (Citation2018) observed no differences on broiler performance between broilers fed a CAG supplemented diet or no enzyme supplementation from 1 to 21 days of age.
Processing weight and yield
Treatment effects on broiler processing weight and yield. Overall, treatment effects on processing weights can be attributed to BW differences between the NC and remaining groups. As observed on day 42, broilers that consumed the NC had the lowest BW and FI compared to the PC-E, PC-P, and all diets supplemented with exogenous enzymes. However, fillet yield was improved when both exogenous enzymes were supplemented at the highest rate compared to broilers fed the PC-E, which had the heaviest day 42 BW. These results might have been caused by an enhancement on AA digestibility due the supplementation of diets with exogenous enzymes. Jasek et al. (Citation2018) evaluated ileal AA digestibility of broilers fed a CAG supplemented diet, and utilized the same rate of CAG inclusion (0.2 g/kg) as in the present study. They observed a 3.8% increase in total ileal AA digestibility when broilers consumed the CAG supplemented diet compared to a non-CAG diet. More specifically, they observed an improvement in methionine, lysine, and threonine digestibility which are the first, second, and third limiting AA in poultry. Additionally, a systematic review of 24 published papers (Cowieson et al. Citation2017) reported that phytase inclusion independent of dose rate improved total AA digestibility in broilers by 4.1% with lysine, methionine, and threonine digestibility increasing by 3.4, 1.3, and 6.0%, respectively. Lysine plays an integral role in breast muscle deposition while sufficient methionine and threonine is needed to support optimal growth and carcass yields (Tesseraud et al. Citation1996; Ojano-Dirain and Waldroup Citation2002). In addition, methionine is involved in body protein and synthesis of substances such as creatine (Ojano-Dirain and Waldroup Citation2002; da Silva et al. Citation2009).
Main effects and interactions on processing weight and yield. In contrast to the present study, Smith et al. (Citation2019) observed a 2.1% improvement in breast meat yield when broilers were fed a diet containing 1500 FTU/kg of phytase compared to 500 FTU/kg. As for CAG inclusion, Llamas-Moya et al. (Citation2020) reported a 2.7% increase in breast meat yield when broilers were fed a 100 kcal/kg reduced diet with CAG supplementation compared to non-CAG supplemented groups.
Nutrient digestibility
Treatment effects on nutrient digestibility. Similar to the present study, Schramm et al. (Citation2017) reported that broilers fed 1000 FTU/kg of phytase and either 100 or 150 FXU/kg of xylanase had the highest ileal digestible CP (83.1%) compared to broilers fed a diet with a lower inclusion of xylanase and 1000 FTU/kg of phytase, xylanase alone, or no enzyme supplementation. Mohiti-Asli et al. (Citation2020) fed broilers a combination of phytase and a multicarbohydrase with α-galactosidase, xylanase, and β-glucanase activities. They supplemented exogenous enzymes in a NC diet, which only accounted for phytase matrix values and a low nutrient NC diet, which accounted for both phytase and multicarbohydrase matrix values. Crude protein digestibility increased when broilers were fed a NC diet with both phytase and multicarbohydrase supplementation. However, in contrast to the present study, they observed similar CP digestibility when broilers were fed a nutrient deficient diet and a low nutrient NC diet supplemented with both phytase and a multicarbohydrase.
Broilers in the present study had the highest CP digestibility when fed a diet with phytase and 0.2 g/kg of CAG. Phytase inclusion could have mitigated gastric hypersecretion induced by phytate and reduced endogenous loss of protein from mucins or digestive enzymes (Cowieson et al. Citation2009), whereas xylanase activity by the CAG could have increased gastric retention. Furthermore, phytase activity could be improved by xylanase through the increase of accessibility to stored phytate or by prolonging gastric retention time via the ileal break mechanism (Cowieson and Masey O’Neill Citation2013; Schramm et al. Citation2017). Xylanase facilitates the breakdown of complex NSP producing xylo-oligosaccharides, which can be partially fermented by cecal and intestinal microbiota and produce volatile fatty acids (VFA) (Cowieson and Masey O’Neill Citation2013). These VFA could yield some energetic value for the bird or stimulate secretion of peptide YY, which can delay gastric emptying, increase exposure to endogenous and exogenous enzymes, and lead to further nutrient digestibility (Adrian et al. Citation1985; Cowieson and Masey O’Neill Citation2013).
Juanpere et al. (Citation2005) reported that broilers fed either a phytase, glycosidase, or a combination of both enzymes had an improved lipid digestibility coefficient compared to broilers fed a NC. As for the present study, fat digestibility was higher when broilers consumed a diet with 500 FTU/kg of phytase compared to 1500 FTU/kg, regardless of CAG supplementation.
Reduction on fat digestibility could be due to higher calcium released from phytate molecules when higher levels of phytase were included in the diet. Calcium can negatively influence fat digestion through formation of metallic soaps (Ravindran et al. Citation2000; Tancharoenrat Citation2012). Indigestible metallic soaps can be formed when calcium-phytate complexes bind with fatty acids reducing mineral and fat utilization (Ravindran et al. Citation2000; Selle et al. Citation2009; Tancharoenrat and Ravindran Citation2014). Furthermore, an influx of dietary calcium or calcium released from the calcium-phytate complex in the gastrointestinal tract (GIT) lumen can decrease fat digestion and absorption through formation of soluble or insoluble soaps (Atteh and Leeson Citation1983; Tancharoenrat and Ravindran Citation2014).
Walters et al. (Citation2019) also observed a 33.5% increase in calcium digestibility when broilers consumed a NC compared to broilers fed a phosphorus and calcium sufficient diet at 28 days of age. Kim et al. (Citation2021) observed the highest AID of calcium in broilers that consumed a diet with a multi-enzyme complex (activity: amylase, protease, mannanase, and xylanase) combined with phytase. However, in the present study, broilers fed diets with 500 FTU/kg of phytase and no inclusion of CAG had the highest calcium digestibility. Additionally, Lu et al. (Citation2013) reported a 49% reduction in calcium digestibility when broilers were fed a diet combined with xylanase, β-glucanase, and phytase compared to broilers fed a nutrient sufficient control diet on day 21. In contrast, they did not observe a difference for day 42 AID calcium when broilers were fed diets with varying rates of NSP degrading enzymes and phytase.
Wang et al. (Citation2021) and Woyengo et al. (Citation2010) reported improved ileal phosphorus digestibility when broilers were fed a combination of phytase and a multicarbohydrase in comparison to broilers fed a diet with sufficient calcium and available phosphorus. Overall, broilers fed a diet with 500 or 1500 FTU/kg of phytase had an average phosphorus digestibility of 70 and 76%, respectively.
A possible contribution to the AIDE differences between the PC-P and NC is that the PC-P had approximately 1.3 times more poultry oil inclusion in the finisher feed compared to the NC. Increased dietary fat could improve efficiency of consumed energy and slow passage rate of the GIT allowing for further nutrient digestion (Baião and Lara Citation2005). As for the similar AIDE values observed between the exogenous enzyme supplemented diets and the PC-E, non-starch polysaccharide structures in diets primarily composed of corn and soybean meal (SBM) could have approximately 400–450 kcal/kg of undigested energy (Cowieson Citation2010). Therefore, the use of NSP degrading enzymes is needed to utilize these energy baring compounds. Regardless of phytase inclusion, broilers fed 0.1 or 0.2 g/kg of CAG had an average improvement of 253 and 292 kcal/kg, respectively, in AIDE compared to the NC. Similarly, Schramm et al. (Citation2017) observed a 300 kcal/kg improvement in apparent ME when broilers were fed 1000 FTU/kg of phytase combined with either 100 or 150 FXU/kg of xylanase compared to broilers fed a diet with only phytase or no enzyme supplementation. Wang et al. (Citation2021) also observed an average increase of 194 kcal/kg in apparent ME when broilers were fed a combination of phytase and a multicarbohydrase compared to broilers fed a 75 kcal/kg ME reduced NC diet. In contrast, Juanpere et al. (Citation2005) did not observe an increase in apparent ME when broilers were fed a combination of phytase and a glycosidase.
Depending on the dietary energy and phytase inclusion, Adeola and Cowieson (Citation2011) suggested that phytase can have an energy sparing effect that ranges from 0 to 120 kcal/kg of ME. In the current study, broilers fed either 500 or 1500 FTU/kg of phytase alone had 247 and 290 kcal/kg more AIDE compared to the NC, respectively. Additionally, improved energy utilization of broilers fed the NC + 1500 FTU/kg of phytase and 0.2 g/kg of CAG could be due to α-galactosidase’s ability to hydrolyse raffinose and stachyose. This ANF reduction could lead to increased nutrient digestibility by reducing digesta viscosity or through hydrolysis of oligosaccharides into monosaccharides (Graham et al. Citation2002). Moreover, the CAG’s xylanase activity could enhance availability of starch by reducing nutrient encapsulation caused by complex NSP (Slominski et al. Citation1993; Jasek et al. Citation2018).
Main effects and interactions on nutrient digestibility. Olukosi et al. (Citation2020) fed broilers 500, 1500, or 3000 FTU/kg of phytase and reported no difference in calcium digestibility at 7 and 28 days of age. Similar to the present study, Walters et al. (Citation2019) observed an increase in ileal phosphorus digestibility with increasing dose rates of phytase. Although AIDE was not influenced by CAG supplementation in the present study, Jasek et al. (Citation2018) reported that broilers fed 0.2 g/kg of a multicarbohydrase (α-galactosidase and xylanase activity) from 1 to 21 days of age had around a 90 kcal/kg improvement in AIDE compared to broilers fed a non-enzyme supplemented diet.
The dietary supplementation of phytase and a multicarbohydrase containing α-galactosidase ameliorated negative effects induced by nutrient reduced diets during a 42-day broiler grow out. When individually assessed, phytase supplemented at 500 FTU/kg increased fat digestibility, whereas 1500 FTU/kg reduced FCR and increased phosphorus digestibility. As for CAG inclusion, supplementation at 0.2 g/kg decreased FCR and increased fillet yield. When compared to nutrient reduced diets, the individual supplementation and combination of these exogenous enzymes improved BW, fillet yield, CP digestibility, phosphorus digestibility, and AIDE. Overall, the combination of phytase and CAG can be an alternative to increase broiler performance, processing measurements, and nutrient digestibility in corn-SBM diets with reduced levels of ME, available phosphorus, and calcium. Further research is warranted to determine optimal inclusion rates of phytase and a multicarbohydrase complex containing α-galactosidase to improve broiler growth and nutrient utilization.
Disclosure statement
The authors declare that the research was conducted in the absence of any commercial or financial relationships that could be construed as a potential conflict of interest. T.D. and S.L.M are employees of Kerry, but authors declare that their role in this project was not directional in the discussion of the results and findings from any commercial angle.
Data availability statement
All data are contained within the article.
Additional information
Funding
References
- Adeola O, Cowieson AJ. 2011. Opportunities and challenges in using exogenous enzymes to improve nonruminant animal production. J Anim Sci. 89:3189–3218. doi:10.2527/jas.2010-3715.
- Adrian TE, Ferri GL, Bacarese-Hamilton AJ, Fuessl HS, Polak JM, Bloom SR. 1985. Human distribution and release of a putative new gut hormone, peptide YY. Gastroenterology. 89:1070–1077. doi:10.1016/0016-5085(85)90211-2.
- American Veterinary Medical Association. 2020. AVMA guidelines for the euthanasia of animals. Schaumburg (IL): American Veterinary Medical Association.
- Atteh JO, Leeson S. 1983. Effects of dietary fatty acids and calcium levels on performance and mineral metabolism of broiler chickens. Poult Sci. 62:2412–2419. doi:10.3382/ps.0622412.
- Aviagen. 2019a. Ross broiler: nutrition specifications. Huntsville (AL): Aviagen.
- Aviagen. 2019b. Yield plus x Ross 708: performance objectives. Huntsville (AL): Aviagen.
- Baião NC, Lara LJC. 2005. Oil and fat in broiler nutrition. Braz J Poult Sci. 7:129–141. doi:10.1590/S1516-635X2005000300001.
- Bedford M, Rousseau X. 2017. Recent findings regarding calcium and phytase in poultry nutrition. Anim Prod Sci. 57:2311–2316. doi:10.1071/AN17349.
- Bedford MR. 2000. Exogenous enzymes in monogastric nutrition – their current value and future benefits. Anim Feed Sci Technol. 86:1–13. doi:10.1016/S0377-8401(00)00155-3.
- Broch J, Dos Santos EC, Damasceno JL, Nesello PO, de Souza C, Eyng C, Pesti GM, Nunes RV. 2020. Phytase and phytate interactions on broilers’ diet at 21 days of age. J Appl Poult Res. 29:240–250. doi:10.1016/j.japr.2019.10.010.
- Cowieson AJ. 2010. Strategic selection of exogenous enzymes for corn/soy-based poultry diets. J Poult Sci. 47:1–7. doi:10.2141/jpsa.009045.
- Cowieson AJ, Acamovic T, Bedford MR. 2004. The effects of phytase and phytic acid on the loss of endogenous amino acids and minerals from broiler chickens. Br Poult Sci. 45:101–108. doi:10.1080/00071660410001668923.
- Cowieson AJ, Bedford MR, Selle PH. 2009. Phytate and microbial phytase: implications for endogenous nitrogen losses and nutrient availability. World’s Poult Sci J. 65:401–418. doi:10.1017/S0043933909000294.
- Cowieson AJ, Masey O’Neill HV. 2013. Effects of exogenous xylanase on performance, nutrient digestibility and caecal thermal profiles of broilers given wheat-based diets. Br Poult Sci. 54:346–354. doi:10.1080/00071668.2013.780200.
- Cowieson AJ, Ruckebusch JP, Sorbara JOB, Wilson JW, Guggenbuhl P, Roos FF. 2017. A systematic view on the effect of phytase on ileal amino acid digestibility in broilers. Anim Feed Sci Technol. 225:182–194. doi:10.1016/j.anifeedsci.2017.01.008.
- da Silva RP, Nissim I, Brosnan ME, Brosnan JT. 2009. Creatine synthesis: hepatic metabolism of guanidinoacetate and creatine in the rat in vitro and in vivo. Am J Physiol Endocrinol Metab. 296:E256–261. doi:10.1152/ajpendo.90547.2008.
- De Keyser K, Kuterna L, Kaczmarek S, Rutkowski A, Vanderbeke E. 2016. High dosing NSP enzymes for total protein and digestible amino acid reformulation in a wheat/corn/soybean meal diet in broilers. J Appl Poult Res. 25:239–246. doi:10.3382/japr/pfw006.
- Ennis CE, Jackson M, Gutierrez O, Cantley S, Wamsley KGS. 2020. Phytase and carbohydrase inclusion strategies to explore synergy within low-energy diets to optimize 56-day male broiler performance and processing. J Appl Poult Res. 29:1045–1067. doi:10.1016/j.japr.2020.09.013.
- Fernandes JIM, Horn D, Ronconi EJ, Buzim R, Lima FK, Pazdiora DA. 2019. Effects of phytase superdosing on digestibility and bone integrity of broilers. J Appl Poult Res. 28:390–398. doi:10.3382/japr/pfz001.
- Gautier AE, Rochell SJ. 2020. Influence of coccidiosis vaccination on nutrient utilization of corn, soybean meal and distilled dried grains with solubles in broilers. Poult Sci. 99:3540–3549. doi:10.1016/j.psj.2020.03.035.
- Gitzelmann R, Auricchio S. 1965. The handling of soya alpha-galactosides by a normal and a galactosemic child. Pediatrics. 36:231–235.
- Graham KK, Kerley MS, Firman JD, Allee GL. 2002. The effect of enzyme treatment of soybean meal on oligosaccharide disappearance and chick growth performance. Poult Sci. 81:1014–1019. doi:10.1093/ps/81.7.1014.
- Gulizia JP, Rueda MS, Ovi FK, Bonilla SM, Prasad R, Jackson ME, Gutierrez O, Pacheco WJ. 2022. Evaluate the effect of a commercial heat stable phytase on broiler performance, tibia ash, and mineral excretion from 1 to 49 days of age assessed using nutrient reduced diets. J Appl Poult Res. 31:100276. doi:10.1016/j.japr.2022.100276.
- Harland BF, Narula G. 1999. Food phytate and its hydrolysis products. Nutr Res. 19:947–961. doi:10.1016/S0271-5317(99)00055-X.
- Humer E, Schwarz C, Schedle K. 2015. Phytate in pig and poultry nutrition. J Anim Physiol Anim Nutr. 99:605–625. doi:10.1111/jpn.12258.
- Jackson ME. 2010. Mannanase, alpha-galactosidase and pectinase. In: Bedford MR, Partridge G, editors. Enzymes in farm animal nutrition. 2nd ed. Oxfordshire: CAB International; p. 54–84.
- Jasek A, Latham RE, Mañón A, Llamas-Moya S, Adhikari R, Poureslami R, Lee JT. 2018. Impact of a multicarbohydrase containing α-galactosidase and xylanase on ileal digestible energy, crude protein digestibility, and ileal amino acid digestibility in broiler chickens. Poult Sci. 97:3149–3155. doi:10.3382/ps/pey193.
- Juanpere J, Pérez-Vendrell AM, Angulo E, Brufau J. 2005. Assessment of potential interactions between phytase and glycosidase enzyme supplementation on nutrient digestibility in broilers. Poult Sci. 84:571–580. doi:10.1093/ps/84.4.571.
- Karimi A, Coto C, Mussini F, Goodgame S, Lu C, Yuan J, Bedford MR, Waldroup PW. 2013. Interactions between phytase and xylanase enzymes in male broiler chicks fed phosphorus-deficient diets from 1 to 18 days of age. Poult Sci. 92:1818–1823. doi:10.3382/ps.2012-02818.
- Kidd MT, Morgan GW Jr., Price CJ. 2001a. Enzyme supplementation to corn and soybean meal diets for broilers. J Appl Poult Res. 10:65–70. doi:10.1093/japr/10.1.65.
- Kidd MT, Morgan GW Jr., Zumwalt CD. 2001b. α-Galactosidase enzyme supplementation to corn and soybean meal broiler diets. J Appl Poult Res. 10:186–193. doi:10.1093/japr/10.2.186.
- Kim M, Ingale SL, Hosseindoust A, Choi Y, Kim KY, Chae B. 2021. Synergistic effect of exogenous multi-enzyme and phytase on growth performance, nutrients digestibility, blood metabolites, intestinal microflora and morphology in broilers fed corn-wheat-soybean meal diets. Anim Biosci. 34:1365–1374. doi:10.5713/ab.20.0663.
- Lee SA, Dunne J, Febery E, Brearley CA, Mottram T, Bedford MR. 2018. Exogenous phytase and xylanase exhibit opposing effects on real-time gizzard pH in broiler chickens. Br Poult Sci. 59:568–578. doi:10.1080/00071668.2018.1496403.
- Leeson S, Summers JD. 2001a. Minerals. In: Leeson S, editor. Scott’s nutrition of the chicken. 4th rev. ed. Guelph: University Books; p. 331–420.
- Leeson S, Summers JD. 2001b. Digestion and nutrient availability. In: Leeson S, editor. Scott’s nutrition of the chicken. 4th rev. ed. Guelph: University Books; p. 1–33.
- Leyva-Jimenez H, Alsadwi AM, Gardner K, Voltura E, Bailey CA. 2019. Evaluation of high dietary phytase supplementation on performance, bone mineralization, and apparent ileal digestible energy of growing broilers. Poult Sci. 98:811–819. doi:10.3382/ps/pey389.
- Llamas-Moya S, Girdler CP, Shalash SMM, Atta AM, Gharib HB, Morsy EA, Salim HM, Awaad MHH, Elmenawey M. 2020. Effect of a multicarbohydrase containing α-galactosidase enzyme on the performance, carcass yield, and humoral immunity of broilers fed corn-soybean meal-based diets of varying energy density. J Appl Poult Res. 29:142–151. doi:10.1016/j.japr.2019.10.001.
- Llamas-Moya S, Higgins NF, Adhikari R, Lawlor PG, Lacey S. 2021. Effect of multicarbohydrase enzymes containing α-galactosidase on the growth and apparent metabolizable energy digestibility of broiler chickens: a meta-analysis. Anim Feed Sci Technol. 277:114949. doi:10.1016/j.anifeedsci.2021.114949.
- Lu H, Adedokun SA, Preynat A, Legrand-Defretin V, Geraert PA, Adeola O, Ajuwon KM. 2013. Impact of exogenous carbohydrases and phytase on growth performance and nutrient digestibility in broilers. Can J Anim Sci. 93:243–249. doi:10.4141/cjas2012-138.
- Meng X, Slominski BA. 2005. Nutritive values of corn, soybean meal, canola meal, and peas for broiler chickens as affected by a multicarbohydrase preparation of cell wall degrading enzymes. Poult Sci. 84:1242–1251. doi:10.1093/ps/84.8.1242.
- Mohiti-Asli M, Ghanaatparast-Rashti M, Akbarian P, Mousavi SN. 2020. Effects of a combination of phytase and multi-carbohydrase enzymes in low-density corn-soybean meal based diets on growth performance and ileal nutrients digestibility of male broilers. Ital J Anim Sci. 19:1533–1541. doi:10.1080/1828051X.2020.1857311.
- Ojano-Dirain CP, Waldroup PW. 2002. Evaluation of lysine, methionine and threonine needs of broilers three to six week of age under moderate temperature stress. Int J Poult Sci. 1:16–21. doi:10.3923/ijps.2002.16.21.
- Olukosi OA, González-Ortiz G, Whitfield H, Bedford MR. 2020. Comparative aspects of phytase and xylanase effects on performance, mineral digestibility, and ileal phytate degradation in broilers and turkeys. Poult Sci. 99:1528–1539. doi:10.1016/j.psj.2019.11.018.
- Pallauf J, Rimbach G. 1997. Nutritional significance of phytic acid and phytase. Arch Anim Nutr. 50:301–319. doi:10.1080/17450399709386141.
- Parkkonen R, Tervilӓ-Wilo A, Hopeakoski-Nurminen M, Morgan A, Poutanen K, Autio K. 1997. Changes in wheat micro structure following in vitro digestion. Acta Agric Scand B Soil and Plant Sci. 47:43–47. doi:10.1080/09064719709362437.
- Poernama F, Wibowo TA, Liu YG. 2021. The effect of feeding phytase alone or in combination with nonstarch polysaccharides-degrading enzymes on broiler performance, bone mineralization, and carcass traits. J Appl Poult Res. 30:100134. doi:10.1016/j.japr.2020.100134.
- Ravindran V, Cabahug S, Ravindran G, Selle PH, Bryden WL. 2000. Response of broiler chickens to microbial phytase supplementation as influenced by dietary phytic acid and non-phytate phosphorus levels. II. Effects on apparent metabolisable energy, nutrient digestibility and nutrient retention. Br Poult Sci. 41:193–200. doi:10.1080/00071660050022263.
- Ravindran V, Morel PC, Partridge GG, Hruby M, Sands JS. 2006. Influence of an Escherichia coli-derived phytase on nutrient utilization in broiler starters fed diets containing varying concentrations of phytic acid. Poult Sci. 85:82–89. doi:10.1093/ps/85.1.82.
- Scholey DV, Morgan NK, Riemensperger A, Hardy R, Burton EJ. 2018. Effect of supplementation of phytase to diets low in inorganic phosphorus on growth performance and mineralization of broilers. Poult Sci. 97:2435–2440. doi:10.3382/ps/pey088.
- Schramm VG, Durau JF, Barrilli LNE, Sorbara JOB, Cowieson AJ, Félix AP, Maiorka A. 2017. Interaction between xylanase and phytase on the digestibility of corn and a corn/soy diet for broiler chickens. Poult Sci. 96:1204–1211. doi:10.3382/ps/pew356.
- Selle PH, Cowieson AJ, Ravindran V. 2009. Consequences of calcium interactions with phytate and phytase for poultry and pigs. Livest Sci. 124:126–141. doi:10.1016/j.livsci.2009.01.006.
- Short FJ, Gorton P, Wiseman J, Boorman KN. 1996. Determination of titanium dioxide added as an inert marker in chicken digestibility studies. Anim Feed Sci Technol. 59:215–221. doi:10.1016/0377-8401(95)00916-7.
- Slominski BA. 2011. Recent advances in research on enzymes for poultry diets. Poult Sci. 90:2013–2023. doi:10.3382/ps.2011-01372.
- Slominski BA, Guenter W, Campbell LD. 1993. New approach to water-soluble carbohydrate determination as a tool for evaluation of plant cell wall degrading enzymes. J Agric Food Chem. 41:2304–2308. doi:10.1021/jf00036a016.
- Smith KA, Wyatt CL, Lee JT. 2019. Evaluation of increasing levels of phytase in diets containing variable levels of amino acids on male broiler performance and processing yields. J Appl Poult Res. 28:253–262. doi:10.3382/japr/pfy065.
- Sommerfeld V, Schollenberger M, Kühn I, Rodehutscord M. 2018. Interactive effects of phosphorus, calcium, and phytase supplements on products of phytate degradation in the digestive tract of broiler chickens. Poult Sci. 97:1177–1188. doi:10.3382/ps/pex404.
- Statistical Analysis System. Institute Inc. 2010. Using JMP 9. Cary, NC: SAS Institute.
- Stein HH, Fuller MF, Moughan PJ, Sève B, Mosenthin R, Jansman AJM, Fernández JA, de Lange CFM. 2007. Definition of apparent, true and standardized ileal digestibility of amino acids in pigs. Livest Sci. 109:282–285. doi:10.1016/j.livsci.2007.01.019.
- Tancharoenrat P. 2012. Factors influencing fat digestion in poultry. PhD diss. Massey University, Palmerston North, New Zealand.
- Tancharoenrat P, Ravindran V. 2014. Influence of tallow and calcium concentrations on the performance and energy and nutrient utilization in broiler starters. Poult Sci. 93:1453–1462. doi:10.3382/ps.2013-03817.
- Tesseraud S, Maaa N, Peresson R, Chagneau AM. 1996. Relative responses of protein turnover in three different skeletal muscles to dietary lysine deficiency in chicks. Br Poult Sci. 37:641–650. doi:10.1080/00071669608417893.
- Walk CL, Santos TT, Bedford MR. 2014. Influence of superdoses of a novel microbial phytase on growth performance, tibia ash, and gizzard phytate and inositol in young broilers. Poult Sci. 93:1172–1177. doi:10.3382/ps.2013-03571.
- Walters HG, Coelho M, Coufal CD, Lee JT. 2019. Effects of increasing phytase inclusion levels on broiler performance, nutrient digestibility, and bone mineralization in low-phosphorus diets. J Appl Poult Res. 28:1210–1225. doi:10.3382/japr/pfz087.
- Wang J, Patterson R, Kim WK. 2019. Effects of extra-dosing phytase in combination with multi-carbohydrase on growth performance and bone mineralization using dual-energy x-ray absorptiometry in broilers. J Appl Poult Res. 28:722–728. doi:10.3382/japr/pfz024.
- Wang J, Patterson R, Kim WK. 2021. Effects of phytase and multicarbohydrase on growth performance, bone mineralization, and nutrient digestibility in broilers fed a nutritionally reduced diet. J Appl Poult Res. 30:100146. doi:10.1016/j.japr.2021.100146.
- Wolf A, Watson M, Wolf N. 2003. Digestion and dissolution methods for P, K, Ca, Mg, and trace elements. In: Peters J, editor. Recommended methods of manure analysis. Madison (WI): University of Wisconsin Extension Publication; p. 30–39.
- Woyengo TA, Nyachoti CM. 2011. Review: supplementation of phytase and carbohydrases to diets for poultry. Can J Anim Sci. 91:177–192. doi:10.4141/cjas10081.
- Woyengo TA, Slominski BA, Jones RO. 2010. Growth performance and nutrient utilization of broiler chickens fed diets supplemented with phytase alone or in combination with citric acid and multicarbohydrase. Poult Sci. 89:2221–2229. doi:10.3382/ps.2010-00832.
- Zhao R, Zhao R, Tu Y, Zhang X, Deng L, Chen X. 2018. A novel α-galactosidase from the thermophilic probiotic Bacillus coagulans with remarkable protease-resistance and high hydrolytic activity. PLoS One. 13:e0197067. doi:10.1371/journal.pone.0197067.