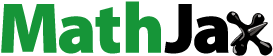
ABSTRACT
The study aimed to assess the impact of mixed silages of biomass sorghum and BRS Capiaçu with cactus pear on the fermentative profile and nutritional value. The silages included forage sorghum cv. Volumax, biomass sorghum cv. ‘BRS 716,’ BRS Capiaçu grass, a mix of 60% biomass sorghum ‘BRS 716’ with 40% Opuntia ficus indica Mill (‘Gigante’) cactus pear (based on fresh matter), and a mix of 60% BRS Capiaçu grass with 40% Opuntia ficus indica Mill (‘Gigante’) cactus pear (based on fresh matter). The experiment followed a randomized experimental design with five treatments and eight repetitions. The highest gas losses (P < 0.01) and lowest dry matter recovery (DMR; P < 0.01) were observed in the BRS Capiaçu grass and BRS Capiaçu grass with cactus pear silages. The lactic acid concentration in the biomass sorghum with cactus pear silage was 49.96% higher than in the Volumax sorghum silage . Volumax sorghum and ‘BRS 716’ biomass silages demonstrated higher DM contents (P < 0.01; mean 33.44%) compared to other silages. There was no significant difference (P = 0.24) among silages regarding the potentially degradable insoluble fraction of the DM, with a mean of 51.75%. The mixed silage of biomass sorghum with cactus pear exhibited lower losses and higher DMR.
Highlights
Incorporation of cactus pear into silage reduces dry matter losses.
Adding cactus pear to sorghum silage enhances lactic acid contents.
Incorporating cactus pear into sorghum silage improves digestibility.
Introduction
Grain or forage ensiling is a widely practiced technique for preserving feed for ruminant animals globally. Traditionally, corn (whole plant) has been the dominant crop used for silage production (Daniel et al. Citation2019). However, its extensive water requirements, especially in regions with water scarcity, such as arid and semiarid areas, have limited its cultivation (McCary et al. Citation2020; Cordeiro et al. Citation2023; Souza et al. Citation2023).
As an alternative to corn, sorghum (Sorghum bicolor L. Moench) has gained popularity in Brazil, where varieties like Volumax are extensively cultivated for both grain and silage production. Sorghum is favoured for its ability to thrive in water-stressed conditions (with average rainfall as low as 500 mm) and high temperatures. Its deep root system enables efficient moisture and nutrient extraction from the soil, allowing it to thrive and yield substantial forage in semiarid regions. Annual dry matter (DM) yields typically range from 10 to 50 t ha−1 (Qu et al. Citation2014; Almeida et al. Citation2019; Dubeux et al. Citation2021; Hora et al. Citation2023), with biomass sorghum varieties like ‘BRS 716’ (Ramos et al. Citation2021; Queiroz et al. Citation2021) exhibiting higher yields and good nutritional value. Sorghum adaptability to diverse soil and climate conditions worldwide further enhances its potential for future use. Dubeux et al. (Citation2021) demonstrated impressive productivity of ‘BRS 716’ biomass sorghum, yielding 36.61 t of DM in a single cutting in a semiarid region of Brazil.
Selecting high-yield forage varieties for silage production can significantly reduce animal feed costs, particularly in intensive production systems. In this regard, BRS Capiaçu grass (Pennisetum purpureum Schum.) has gained prominence in Brazil due to its annual DM productivity exceeding 50 t ha−1 (Monção et al. Citation2020b; Ribas et al. Citation2021). However, silages of biomass sorghum and BRS Capiaçu grass may face quality limitations due to low concentrations of soluble carbohydrates during ensiling—a critical substrate for homofermentative bacteria responsible for lactic acid production (Borreani et al. Citation2018; Amaral et al. Citation2020; Monção et al. Citation2020a; Cordeiro et al. Citation2023).
Conversely, cactus pear (Opuntia or Nopalea) is a cactus species rich in soluble carbohydrates, containing over 200 g kg−1 of DM (Monção et al. Citation2020b; Pastorelli et al. Citation2022). It is extensively cultivated in Brazil's semiarid region, covering over 550,000 ha (Dubeux et al. Citation2021). Cactus pear shows potential for mixed silage production with other tropical forages. However, its high water content, though not entirely free but in mucilage form, can potentially affect fermentation and silage quality. In ruminant feeding, cactus pear is not suitable as a sole feed due to its low fibre and crude protein content, making it a complementary roughage source.
Mixed silages of biomass sorghum, BRS Capiaçu grass, and cactus pear are uncommon in rural areas, leading to knowledge gaps regarding their fermentation profile, DM losses, and nutritional value. This research aims to address these gaps and assess whether including cactus pear in the ensiling process of biomass sorghum (cv. ‘BRS 716’) and BRS Capiaçu grass can improve fermentation and nutritional quality. This approach may promote the production of quantitatively significant forage with adequate nutritional value, alleviating the feed deficit in semiarid regions. Additionally, it may simplify and optimize cactus pear use by reducing the need for frequent cutting when used fresh.
In light of the above, this study aims to evaluate the fermentation profile and nutritional value of silages produced by mixing biomass sorghum ‘BRS 716’ and BRS Capiaçu grass with cactus pear.
Material and methods
Experiment site
The experiment took place at the Experimental Farm of the State University of Montes Claros, situated in the municipality of Janaúba, with geographical coordinates of 15°52'38” S and 43°20'05” W, located in Minas Gerais State, Brazil. According to the Köppen’s classification (Citation1948), the region boasts a tropical savanna climate (Aw), characterized by rainy summers and distinct dry periods during the winter. The average annual rainfall is less than 800 mm, and the mean annual temperature remains around 27°C. The climate can be described as tropical mesothermic, almost megathermic, due to the altitude, and varies from sub-humid to semiarid, with irregular rain events leading to extended periods of drought.
Treatments and experimental design
We employed experimental silos to create silages from the following forage sources: forage sorghum cv. Volumax, biomass sorghum cv. ‘BRS 716,’ BRS Capiaçu grass, a blend of 60% biomass sorghum and 40% ‘Gigante’ cactus pear based on fresh matter, and a blend of 60% BRS Capiaçu grass and 40% ‘Gigante’ cactus pear based on fresh matter. The experimental design was completely randomized, consisting of five treatments, each with eight replications.
Crops and treatment application
All the forages used in this study were cultivated at the Experimental Farm of the State University of Montes Claros. For the sorghum varieties, the soil tillage was performed by one plowing and two harrowings. Soil samples were collected and analyzed 90 days before sowing. During the sowing phase, NPK fertilizer (05-30-10) was applied according to soil analysis recommendations for sorghum crops. Weed and pest control were carried out using an Atrazine-based herbicide and insecticide/fungicide applications. ‘Volumax’ sorghum was harvested at 110 days, while biomass sorghum was harvested 160 days after sowing (Dubeux et al. Citation2021; Souza et al. Citation2021; Queiroz et al. Citation2021).
BRS Capiaçu grass (Pennisetum purpureum Schum) was established in 2016 in a flat area measuring 50 m x 100 m. After uniformly cutting the grass 10 cm above the ground using a manual pruner, maintenance fertilization was applied, consisting of 100 kg ha−1 of N in the form of urea and 60 kg ha−1 of K in the form of KCl through sprinkler fertigation. At harvest, BRS Capiaçu grass reached a height of approximately 3.9 m after 110 days of regrowth (Monção et al. Citation2019).
The ‘Gigante’ cactus pear was planted in 2014 within a 250-m2 area on an eutrophic Red-yellow Latosol (Oxisol in the U.S. Classification). The plants were manually harvested without removing the primary cladode.
The sorghum varieties and BRS Capiaçu grass were processed using a mechanized silage machine (Model JF C90, JF Máquinas, São Paulo, Brazil) equipped with knives set to cut and obtain a 2-cm particle size. Following manual cutting, the cactus pear was processed in an electric forage slicer MC1N (Laboremus®, Campina Grande, Paraíba, Brazil), set at a 2-cm cut without maceration. When ensiling treatments containing cactus pear, all materials were weighed on mechanical scales to ensure a 60% grass (either biomass sorghum or BRS Capiaçu grass) and 40% cactus pear proportion before homogenization.
Silage process
We employed experimental polyvinyl chloride (PVC) silos with known weights, measuring 50 cm in length and 10 cm in diameter. Their bottom contained a 10 cm layer of dry sand (400 g), separated from the forage by plastic foam to measure the produced effluent. The resulting material from each treatment was placed in the silos and compacted using a wooden plunger. For each treatment, we quantified the silage density (550 kg of fresh matter per cubic metre) and deposited approximately 4 kg of chopped material from each fresh forage, as recommended by Sucu et al. (Citation2016). After filling, the silos were sealed with PVC lids equipped with Bunsen-type valves, sealed with tape, and weighed. These silos were stored at room temperature and opened 130 days after ensiling.
Aerobic stability
Aerobic stability was determined by placing a sample of silage (approximately 2 kg) in a mini-silo and maintaining it in a temperature-controlled room with temperatures ranging from 24.5°C to 25.5°C. The temperature of the silage was monitored hourly using a data logger placed in the centre of the mass for nine days. Concurrently, the environmental temperature was recorded hourly using a data logger placed near the mini-silos. Aerobic stability was defined as the number of hours the silage temperature remained stable before increasing by more than 2°C above ambient temperature (Moran et al. Citation1996).
Fermentative losses
Dry matter (DM) losses in the silages, in the form of gases and effluents, were quantified through weight difference, following the method outlined by Jobim et al. (Citation2007). Effluent loss was calculated using EquationEquation 1(1)
(1) .
(1)
(1) Where:
E: effluent production (kg t−1 of fresh mass); Pab: weight of the set (silo + cap + wet sand + foam) at opening (kg); Pen: weight of the set (silo + cap + dry sand + foam) at ensiling (kg); GMfe: fresh mass of forage ensiled (kg).
Gas losses (% DM) were calculated using EquationEquation 2(2)
(2) .
(2)
(2) Where:
G: gas losses (% DM); PCen: weight of the full silo at ensiling (kg); Pen: weight of the set (silo + cap + dry sand + foam) at ensiling (kg); MSen: DM content of the forage at ensiling; PCab: weight of the full silo at opening (kg); MSab: DM content of the forage at opening.
The dry matter recovery (DMR) for each silo was calculated based on the initial and final weights of the DM contents of forage and silage, as per Jobim et al. (Citation2007).
Evaluation of pH, N-NH3, and organic acids
Quantification of N-NH3 and organic acids was conducted on the silage juices obtained using a 16-ton mechanical press (Pryce Citation1969). N-NH3 was measured following the method outlined by Noel and Hambleton (Citation1976). Volatile fatty acids were determined using a liquid chromatographer (Shimadzu® Prominence System model 20A, Kyoto, Japan) equipped with a UV-Vis detector set at 210 nm, an automatic injector calibrated for 5 μL sample volume, and a 300 x Rezex™ ROA-Organic Acid + 7.8 mm column (Phenomenex) maintained at 60°C in an oven. Analytes were diluted in 2.5 mM H2SO4 at a flow rate of 0.6 mL min−1. External standards were used for quantitative calibration purposes.
Chemical composition and ruminal kinetics
A portion of the silage was dried in a forced ventilation oven at 55°C and ground in a knife mill using a 2 mm diameter sieve. A subset was further reground using a 1 mm sieve for laboratory analysis, while the 2 mm diameter particle samples were used for in situ incubation. Samples underwent analysis for dry matter (INCT-CA G-001/1 and G-003/1); crude protein (INCT-CA N-001/2); ether extract (INCT-CA G-004/1); ashes (INCT-CA M-001/2); NDF (INCT-CA F-001/2); ADF (INCT-CA F-003/2), with corrections for ashes (INCT-CA M-002/2) and crude protein (INCT-CA N-004/2); iNDF (INCT-CA F-008/2); insoluble nitrogen compounds in neutral detergent (INND) and acid detergent (INAD); lignin (INCT-CA F-005/2); and non-fibre carbohydrates (Detmann et al. Citation2021). The content of NDFpd was calculated as the difference between NDFap and iNDF. The content of total digestible nutrients (TDN) was estimated according to NRC (Citation2001). provides the in natura chemical composition of the forages used for silage production.
Table 1. Chemical composition of the forages used for silage.
For the rumen kinetics assay, we followed method G-009/1 by Detmann et al. (Citation2021). Two cannulated crossbred steers, with an average body weight of 500 ± 30 kg (mean ± standard deviation), were acclimated for 14 days to a diet containing roughage and concentrate at an 80:20 ratio on a DM basis, with 12% crude protein. The roughage fraction consisted of silage (30% BRS Capiaçu grass silage and 30% biomass sorghum silage) and 20% cactus pear. Water and mineral salt were provided ad libitum. DM intake was estimated at 2.3% of body weight, and the mean pH and rumen ammonia nitrogen at the time of incubation were 6.98 and 13.04 mg dL−1, respectively.
In situ degradability was assessed using 7.5 × 15 cm nonwoven fabric bags (TNT, weight 100) with an approximate porosity of 60 μm, following Casali et al. (Citation2009). The number of samples was determined based on a ratio of 20 mg cm−2 of DM of the bag surface area (Nocek Citation1988). Samples were deposited in the ventral sac region of the rumen for 0, 3, 6, 12, 24, 48, 72, 96, 120, and 144 h. Subsequently, all samples were removed, washed in icy water, dried in a forced air oven at 55 °C for 120 h, and analyzed for DM and NDF content as previously mentioned. Degradation percentage was calculated as the proportion of food remaining in the bags after rumen incubation.
Data were fitted to a non-linear regression using the Gauss–Newton method and the SAS 9.0 software (SAS Institute Inc., Cary, NC), following the equation proposed by Detmann et al. (Citation2021): Dt = A + B x (1-e-c x t); where: Y = cumulative degradation of the analyzed nutrient component after time t; a = degradation curve intercept when t = 0, corresponding to the water-soluble fraction of the analyzed nutrient component; b = potential degradation of the water-insoluble fraction of the analyzed nutrient component; a + b = potential degradation of the analyzed nutrient component when time is not limiting; c = fractional degradation rate (h−1); t = incubation time. Coefficients a, b, and c were then applied to the equation proposed by Detmann et al. (Citation2021): ED = a + (b x c/c + k); where: ED = effective rumen degradation of the analyzed nutrient component; k = particle passage rate in the rumen estimated at 5% h−1 (AFRC Citation1993).
Principal component analysis
PCA was employed to gain a deeper understanding of the relationships between the studied variables and independent variables. To achieve this, 29 characteristics were considered. Based on the correlation matrix between these characteristics, data underwent PCA, wherein variables were standardized to possess a mean of zero and a variance of one. A correlation matrix was utilized instead of a covariance matrix (Johnson and Wichern Citation2008). Kaiser's method (Citation1960) was used to select the principal components that best simplified the dataset's variability, with eigenvalues equal to or greater than one (1) retained, given that the original variables also had a variance equal to one after standardization.
Statistical analysis
Data were subjected to statistical analysis using a model that incorporated fixed effects of silages (treatments). Means were compared using the Scott-Knott test at a 5% significance level. The UNIVARIATE procedure was employed to detect outliers or influential values and assess the normality of residuals.
For variables related to the fermentative profile and chemical composition, the following model was used:
Where:
Yij = observed value for the variable i regarding the treatment in the replication j; m = mean of all experimental units for the variable under study;
ti = effect of the treatment i on the observation value Yij;
eij = error associated with the independent observation Yij, which, by hypothesis, has a normal distribution with mean zero and variance δ2.
DM and NDF ruminal degradability were analyzed using a randomized block design in split plots, featuring five treatments (plots) and ten incubation times (subplots). Variation in animal body weight served as the blocking factor, and the model had the fixed effects of silages (treatments). Means were compared using contrasts at a 5% significance level. The following statistical model was applied:
Where:
Yijk = observation referring to the time P in subplot k of treatment Ti in block j;
μ = constant associated with all observations;
Ti = effect of treatment ‘i,’ with i ranging from 1 to 5;
Bj = effect of block j, with j equal to 1 and 2;
eij = experimental error associated with plots, which, by hypothesis, follows a normal distribution with mean zero and variance δ2;
P = effect of incubation time k, with k ranging from 1 to 10;
TPik = effect of the interaction between treatment level (i) and incubation time level (k);
Eijk = experimental error associated with all observations, which, by hypothesis, follows a normal distribution with mean zero and variance δ2.
For exploratory data analysis by principal component analysis (PCA) PAST® 4.03 software was used (Hammer et al. Citation2001).
Results
Aerobic stability
Upon silo opening, a significant interaction (p < 0.01) was observed between silages and time for temperature. At the opening time, the temperatures of biomass sorghum and BRS Capiaçu grass silages were not significantly different from each other (averaging 26.09 °C) but were 0.83 °C higher than the mean temperature of the other silages (). Over the next 72 h, no significant differences were recorded among silages, with mean temperatures of 25.29, 25.72, and 26.27 °C, respectively. However, at later times of aerobic exposure, the highest temperatures (P < 0.01) were observed in the ‘Volumax’ sorghum silage and biomass sorghum. All silages became aerobically unstable 108 h after the silos were opened ().
Figure 1. Mean temperatures during the aerobic stability of ‘Volumax’ sorghum, biomass sorghum (cv. BRS 716), and BRS Capiaçu grass silages, with or without cactus pear, at various times post-opening (interaction between treatments and times post-opening: P < 0.01).
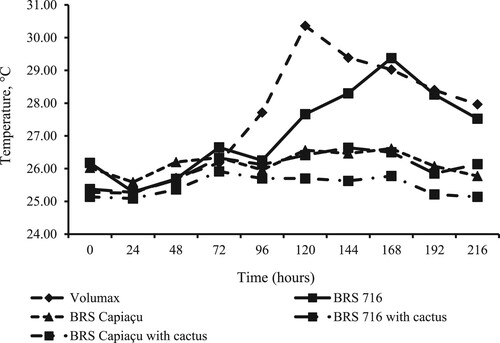
Figure 2. Temperature profile of Volumax sorghum, biomass sorghum (BRS 716), and BRS Capiaçu grass silages, with or without cactus pear, throughout the 216-hour aerobic stability test.
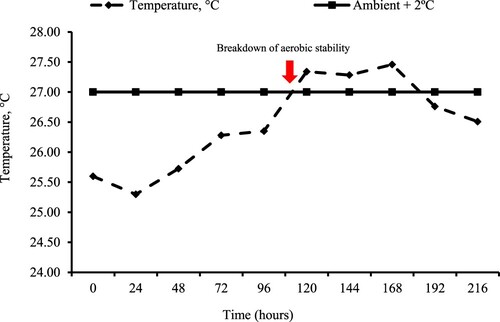
Regarding pH values, no interaction (P = 0.17) was observed between silages and time after opening during aerobic stability. Therefore, means of treatments and post-opening times were presented separately. Among the treatments, silages of BRS Capiaçu grass and BRS Capiaçu grass with cactus pear exhibited pH values (averaging 5.73) that were 22.52% higher than the other silages (). As the aerobic exposure of silages increased, a daily increase of 0.162 units in pH values was observed (). The mean pH value observed at the opening of the silo was 4.43.
Dry matter losses and fermentative profile
Silages of BRS Capiaçu grass and BRS Capiaçu grass with cactus pear showed higher gas losses (P < 0.01) and pH values (P < 0.01); lower DMR (P < 0.01); and lower concentrations of succinic, acetic, and propionic acids (P < 0.01) compared to the other silages (). Gas losses in these silages were 57.59% higher than in silages with biomass sorghum and mixture of biomass sorghum with cactus pear (averaging 52.45 g kg−1 DM). The BRS Capiaçu grass silage had higher effluent losses compared to the other treatments. Silages containing cactus pear showed similar effluent losses (averaging 52.44 kg t−1 of GM). The biomass sorghum with cactus pear silage exhibited higher concentrations of lactic and acetic acids (P < 0.01) compared to other silages. The concentration of lactic acid in biomass sorghum with cactus pear silage was 49.96% higher than in Volumax sorghum silage (41.52 g kg−1 DM on average). The highest concentration of butyric acid (P < 0.01) was observed in Volumax sorghum silage. The ethanol concentrations in biomass sorghum, BRS Capiaçu grass, and BRS Capiaçu grass silages with cactus pear did not differ significantly from each other (P > 0.05), averaging 1.37 g kg−1 DM, and were higher than in the other silages (Volumax and mixture of biomass sorghum with cactus pear).
Table 2. Fermentation profile of Volumax sorghum, biomass sorghum (BRS 716), and BRS Capiaçu grass silages, with or without cactus pear.
Chemical composition
Volumax sorghum and biomass sorghum silages had higher DM contents (P < 0.01; averaging 33.44%) compared to the other silages (). The concentration of N-NH3 was higher in BRS Capiaçu grass silage (P < 0.01) and BRS Capiaçu grass with cactus pear (P < 0.01) compared to the other silages. The DM content of the BRS Capiaçu grass with cactus pear silage was 37.65% lower than the mean of the other silages (averaging 28.20%). BRS Capiaçu grass with cactus pear silage exhibited higher ash content (P < 0.01). Volumax sorghum silage had a CP content 34.01% higher than the mean of the other silages (5.49%). No significant difference in ether extract content was observed among Volumax sorghum silages, biomass sorghum with spineless cactus, and BRS Capiaçu grass with cactus pear (averaging 3.22%). BRS Capiaçu grass and biomass sorghum silage had higher contents of NDF corrected for ash and protein (P < 0.01), and NDFpd (P < 0.01) compared to the rest. Non-fibrous carbohydrate contents in the Volumax sorghum, biomass sorghum, and biomass sorghum with cactus pear silages were 46.64% higher compared to BRS Capiaçu grass and BRS Capiaçu grass with cactus pear silages (averaging 148.7 g kg−1 DM). The lowest TDN contents (P < 0.01) were found in silages of BRS Capiaçu grass and BRS Capiaçu grass with cactus pear (averaging 458.4 g kg−1 DM). No significant difference was observed between silages on indigestible DM (P = 0.45) and iNDF (P = 0.90), averaging 371.05 g kg−1 of DM and 267.94 g kg−1 of DM, respectively. The indigestible ADF content of BRS Capiaçu grass silage was 19.48% higher (P = 0.03) than in other silages (averaging 192.11 g kg−1 DM).
Table 3. Chemical composition of Volumax sorghum, biomass sorghum (cv. ‘BRS 716’), and BRS Capiaçu grass silages, with or without cactus pear.
Ruminal kinetics
Significant differences in ruminal kinetics of DM and fibre fractions were observed among the silages (). Specifically, silages made from Volumax sorghum and biomass sorghum with cactus pear exhibited a 23.59% higher water-soluble fraction (fraction a) of DM (P < 0.01) compared to the other treatments, with an average of 19.14%. However, there were no significant differences (P = 0.24) among the silages in terms of the potentially degradable insoluble fraction (fraction b) of DM, which had a mean value of 51.75%. Notably, the silages containing cactus pear displayed the highest degradation rate for fraction b ‘c.’
Table 4. Ruminal kinetics of dry matter and fibrous fraction in Volumax sorghum silages, biomass sorghum (cv. ‘BRS 716’), and BRS Capiaçu grass silages, with or without cactus pear.
When considering potential DM degradability, silages of Volumax sorghum and biomass sorghum with cactus pear showed a 5.66 percentage unit increase, averaging 75.84%, compared to the other silages. Additionally, the silage of biomass sorghum with cactus pear exhibited higher effective DM degradability than the other silages. Moving on to the potentially degradable insoluble fraction of standardized NDF (fraction Bp; P = 0.14) and the degradation rate of fraction Bp ‘c’ (P = 0.16), no significant differences were detected among the silages, with mean values of 49.76% and 2.56% h−1, respectively.
Effective NDF degradability was notably higher in the silages made from biomass sorghum, biomass sorghum with spineless cactus, and BRS Capiaçu grass with cactus pear, with an average value of 22.79%. The principal component analysis (PCA) revealed that the first six principal components (PCs) had eigenvectors exceeding one, collectively accounting for 98.65% of the total variance in the results (). In the PCA of silages with different roughages (), PC1 (58.25%) and PC2 (16.37%) had eigenvalues that explained 74.62% of the data variance. PC1 was primarily characterized by higher weighting coefficients for indigestible DM, iNDF, and indigestible ADF, accounting for 58.28% of the observed variation. In contrast, PC2 was characterized by higher weighting coefficients for effluent losses, along with the variables from PC1. This analysis () highlighted that BRS Capiaçu grass and Volumax silages had elevated levels of indigestible DM, iNDF, and iADF, making them prominent in the exploratory data analysis. Additionally, the BRS Capiaçu grass silage exhibited greater effluent losses in PC2.
Discussion
Volumax forage sorghum has been extensively used for silage production in various regions of Brazil, particularly in semiarid areas. However, other more productive and adapted tropical forages, such as biomass sorghum and BRS Capiaçu grass, have also found applications in silage production in regions with water deficits. While cactus pear is commonly included in ruminant diets alongside grass silages due to its low fibre content, its use in silage production has been limited due to its high moisture content (above 850 g kg−1 DM), which can adversely affect fermentative quality (Monção et al. Citation2020b).
In our study, we observed that BRS Capiaçu grass with cactus pear silage had a lower DM content, averaging 175.8 g kg−1 DM. This lower DM content was due to the initial low DM content of BRS Capiaçu grass at the time of ensiling (mean of 226.38 g kg−1 DM; ). However, processing cactus pear with a forage slicer for silage production helped preserve mucilage (non-free water in cells), reducing DM losses, especially effluent. In this sense, Dubeux et al. (Citation2021) stated that cactus pear mucilage contains polymers with D-galacturonic acid chains linked by β-bonds (1-4) to L-rhamnose residues R (1-2) (Trachtenberg and Mayer Citation1981; Lee et al. Citation2003). Such biomaterial envelops fluid compounds and prevent effluent loss. Moreover, some studies have highlighted the antimicrobial properties of cactus pear mucilage, particularly against pathogenic and spoilage microorganisms (Ortiz-Rodríguez et al. Citation2016), which could contribute to silage preservation. Osuna-Martínez et al. (Citation2014) suggested that the physiological role of plant mucilage is to regulate cellular water content during prolonged drought and control plant calcium fluxes.
In contrast, BRS Capiaçu grass silage displayed a higher amount of free water, resulting in increased effluent losses, ammonia nitrogen levels, and lower DMR. Monção et al. (Citation2020b) found that crushed cactus pear should not be used for silage in combination with BRS Capiaçu grass at levels above 5% of the fresh matter. This is because the crushing process elevates the amount of free water, leading to increased effluent losses and N-NH3 in the silage due to cactus pear maceration. In our principal component analysis (PCA), effluent losses were identified as a negative factor in BRS Capiaçu grass silage production compared to other treatments.
N-NH3, expressed as a percentage of TN, is an indicator of protein degradation during the fermentation phase and is crucial for assessing the quality of ensiled mass fermentation. According to McDonald et al. (Citation1991), poorly preserved silages typically have ammonia contents exceeding 10%, with ammonia originating from amino acid breakdown. Consequently, we observed a trend towards higher nitrogen losses in the silage of BRS Capiaçu grass with cactus pear due to the higher moisture content in this combination.
Cactus pear is known for its richness in non-fibrous and water-soluble carbohydrates (Dubeux et al. Citation2021), which are essential substrates for the growth of homofermentative bacteria and lactic acid synthesis. Lactic acid, with its low pKa (3.86), plays a crucial role in reducing pH rapidly in ensiled masses, inhibiting the growth of undesirable bacteria (e.g. Enterobacteriaceae and Clostridia; Kung et al. Citation2018). This inhibition is vital for preserving nutrients in the ensiled mass. Biomass sorghum with cactus pear silages exhibited higher concentrations of lactic acid, likely due to the lower water activity in the medium. In silage of BRS Capiaçu grass with cactus pear, water activity may have influenced lactic acid synthesis.
Another critical acid in silage preservation, particularly after silo opening, is acetic acid. Acetic acid inhibits filamentous fungi, molds, and yeasts in the silage, contributing to greater aerobic stability. McDonald et al. (Citation1991) suggested that silages with acetic acid concentrations above 20 g kg−1 of DM may reduce consumption by animals. In our study, the silages of biomass sorghum and biomass sorghum with cactus pear exhibited higher acetic acid concentrations (mean of 2.38 g kg−1 DM). In biomass sorghum with cactus pear silage, this acid contributed to extended silage aerobic exposure time.
However, we observed temperature increases in biomass sorghum silage 96 h after opening the silo, potentially due to the growth of enterobacteria and clostridia, which ferment silage nutrients for growth and reproduction. This temperature rise occurred earlier in Volumax sorghum silage (72 h after silo opening). Silage aerobic stability was compromised 108 h after silo opening when temperatures exceeded the ambient temperature by 2 °C. This indicated the onset of potential DM losses due to the growth of undesirable bacteria in the presence of oxygen.
Volumax sorghum silage had a higher concentration of butyric acid (2.2 g kg−1 DM), which is indicative of clostridial activity and correlates with elevated final pH values. Both butyric acid and ethanol concentrations above 3 g kg−1 DM are undesirable in silage, as they lead to increased DM and energy losses (Borreani et al. Citation2018). Elevated ethanol concentrations were also detected in biomass sorghum, BRS Capiaçu grass, and BRS Capiaçu grass with cactus pear silages. Meanwhile, biomass sorghum silage contained higher levels of propionic acid, averaging 4.54 g kg−1 DM. However, this concentration is not a concern, as it does not indicate degradation of lactic acid by butyric bacteria, which would be a more significant issue (Roth and Undersander Citation1995).
In terms of chemical composition, BRS Capiaçu grass with cactus pear silage exhibited a higher ash content. This is attributed to the high mineral content (243 g kg−1 of DM) in cactus pear, particularly calcium (Dubeux et al. Citation2021). During periods of water scarcity, cactus pear increases its calcium content through mucilage production and the generation of lipid-like substances like waxes, which help minimize water loss to the environment. This phenomenon also explains the rise in crude fat content, contributing to elevated values in silages containing cactus pear. In contrast, Volumax sorghum silage contained panicles with grains, which are rich in non-fibre carbohydrates (NFC), protein nitrogen, and lipids, leading to higher levels of crude protein, crude fat, and TDNs.
BRS Capiaçu grass silage exhibited higher fibre content, averaging 669.1 g kg−1 DM. This result aligns with the large size of this forage and is consistent with findings from previous studies (Monção et al. Citation2020b; Jesus et al. Citation2021; Ribas et al. Citation2021). These studies evaluated BRS Capiaçu grass silages and reported mean fibre content values ranging from 686.1–711.1 g kg−1 DM. In contrast, the mixture of biomass sorghum and cactus pear exhibited lower levels of NDF corrected for ash and protein but higher levels of TDN. Both BRS Capiaçu grass silages, with and without cactus pear, had lower TDN content due to higher levels of ADF (mean of 457.55 g kg−1 DM) and indigestible ADF. Notably, our multivariate analysis highlighted that indigestible ADF content had the highest weighting coefficient, emphasizing that BRS Capiaçu grass silage, with its higher indigestible ADF content, had lower nutritional quality compared to the other treatments. This reduced nutritional quality of BRS Capiaçu grass silage was also observed by Cordeiro et al. (Citation2023), who reported lower body performance in dairy heifers fed BRS Capiaçu grass silage compared to those fed Volumax sorghum, biomass sorghum (cv. ‘BRS 716’), biomass sorghum with cactus pear, and BRS Capiaçu with cactus pear silages.
The higher potential degradability of DM observed in Volumax sorghum and biomass sorghum with cactus pear silages can be attributed to their abundant non-fibre carbohydrates, particularly water-soluble ones. These carbohydrates increase the soluble fraction of DM, thereby influencing the potential degradability of DM. Fraction b, representing potentially degradable insoluble DM, remained consistent across silages (mean of 51.75%). Furthermore, the inclusion of cactus pear in biomass sorghum silage enhanced the degradation rate of fraction b, as well as the effective degradability of DM and NDF.
Conclusion
The incorporation of cactus pear, especially in a silage mixture with biomass sorghum ‘BRS 716,’ lead to reduced losses, increased dry matter recovery (DMR), improved concentrations of desirable volatile organic acids, and enhanced nutritional value.
Compliance with ethical standards
The care and handling of animals in this experiment were conducted in accordance with the guidelines provided by the National Council for the Control of Animal Experimentation (CONCEA – Conselho Nacional de Controle de Experimentação Animal) and received approval from the Ethics Committee on Animal Use of the State University of Montes Claros (protocol CEUA # 222/2020).
Acknowledgments
The authors would like to extend their gratitude to the Research Support Foundation of the Minas Gerais State (FAPEMIG), the State University of Montes Claros (UNIMONTES), the National Council for Scientific and Technological Development (CNPq), and the National Institute of Science and Technology (INCT–Animal Science) for their invaluable support, scholarships, and research assistance.
Data availability statement
The authors confirm that the data supporting the findings of this study are available within the article [and/or] its supplementary materials.
Disclosure statement
No potential conflict of interest was reported by the author(s).
Additional information
Funding
References
- Agricultural and Food Research Council – AFRC. 1993. Energy and protein requirements of ruminants. Walligford: CAB International.
- Almeida LGF, Parrella RAC, Simeone MLF, Ribeiro PCO, Santos AS, Costa ASV, Guimarães AG, Schaffert RE. 2019. Composition and growth of sorghum biomass genotypes for ethanol production. Biomass Bioenergy. 122:343–348. doi:10.1016/j.biombioe.2019.01.030.
- Amaral RC, Carvalho BF, Costa DM, Morenz MJF, Schwan RF, Ávila CLS. 2020. Novel lactic acid bacteria strains enhance the conservation of elephant grass silage cv. BRS Capiaçu. Ani Feed Scie Tech. 264:1–15. doi:10.1016/j.anifeedsci.2020.114472.
- Borreani G, Tabacco E, Schmidt RJ, Holmes BJ, Muck RE. 2018. Silage review: factors affecting dry matter and quality losses in silages. J Dairy Sci. 101(5):3952–3979. doi:10.3168/jds.2017-13837.
- Casali AO, Detmann E, Valadares Filho SC, Pereira JC, Cunha M, Detmann KSC, Paulino MF. 2009. Estimation of fibrous compounds contents in ruminant feeds with bags made from different textiles. Rev Bras Zootec. 38(1):130-138. doi:10.1590/S1516-35982009000100017.
- Cordeiro MWS, Rocha Júnior VR, Monção FP, Palma MNN, Rigueira JPS, Carvalho CCS, Costa M, D D, Angelo MFSV, Costa NM, Oliveira LIS. 2023. Tropical grass silages with spineless cactus in diets of Holstein × Zebu heifers in the semiarid region of Brazil. Trop Anim Health Prod. 55(2):89–104. doi:10.1007/s11250-023-03506-6.
- Daniel JLP, Bernardes TF, Jobim CC, Schmidt P, Nussio LG. 2019. Production and utilization of silages in tropical areas with focus on Brazil. Grass and Forage Sci. 74(2):188–200. doi:10.1111/gfs.12417.
- Detmann E, Silva LFC, Rocha GC, Palma MNN, Rodrigues JPP. 2021. Métodos para análise de alimentos. 2ª Edição, Visconde do Rio Branco, MG: Suprema, 350 p.
- Dubeux Jr JCB, Santos MVF, Cunha MV, Santos DC, Souza RTA, Mello ACL, Souza TC. 2021. Cactus (Opuntia and Nopalea) nutritive value: a review. Ani Feed Scie Tech. 275:1–11. doi.10.1016/j.anifeedsci.2021.114890.
- Hammer O, Harper DAT, Ryan PD. 2001. PAST: Paleontological Statistics software package for education and data analysis. Palaeontol Electr. 4:1–9.
- Hora FF, Júnior R, Monção VR, Rigueira FP, Cordeiro JPS, Caldeira MWS, Cruz LA, Alencar FNF, Albuquerque AMS, Leal CJB, et al. 2023. Nutritional parameters and performance of crossbred heifers fed with millet and BRS 716 biomass sorghum silages. J Appl Anim Res. 51(1):74–83. doi:10.1080/09712119.2022.2155172.
- Jesus MA, Monção FP, Rigueira JPS, Rocha Júnior VR, Gomes VM, Delvaux Junior NA, Pires DAA, Sales ECJ, Carvalho CCS, Santos AS. 2021. Effects of microbial inoculant and fibrolytic enzymes on fermentation quality and nutritional value of BRS capiaçu grass silage. Semina: Ciênc Agrár. 42(3):1837–1852.
- Jobim CC, Nussio LG, Reis RA, Schmidt P. 2007. Avanços metodológicos na avaliação da qualidade da forragem conservada. Rev Bras Zootec. 36:101–119. doi:10.1590/S1516-35982007001000013.
- Johnson RA, Wichern DW. 2008. Multivariate analysis. New Jersey: Springer Briefs Public Heal. 71–81. doi:10.1007/978-3-319-77203-5_8.
- Kaiser HF. 1960. The application of electronic computers to factor analysis. Educ Psychol Meas. 20(1):141–151. doi:10.1177/001316446002000116.
- Köppen, W. 1948. Climatologia: Con un estudio de los climas de la tierra. Fondo de Cultura Econômica. México.
- Kung Jr L, Shaver RD, Grant RJ, Schmidt RJ. 2018. Silage review: Interpretation of chemical, microbial, and organoleptic components of silages. J Dairy Sci, 101(5):4020–4033. doi:10.3168/jds.2017-13909.
- Lee EH, Kim HJ, Song YS, Jin C, Lee KT, Jungsook C, Lee YS. 2003. Constituents of the stems and fruits ofOpuntia ficus-indica var.saboten. Arch Pharm Res. 26:1018–1023. doi:10.1007/BF02994752.
- Martínez UO, Esparza JR, Fragoso LR. 2014. Cactus (Opuntia ficus-indica): a review on its antioxidants properties and potential pharmacological use in chronic diseases. Nat Prod Chemistry Res. 2:1–8. doi. 10.4172/2329-6836.1000153.
- McCary CL, Vyas D, Faciola AP, Ferraretto LF. 2020. Graduate student literature review: current perspectives on whole-plant sorghum silage production and utilization by lactating dairy cows. J Dairy Sci. 103(6):5783–5790. doi:10.3168/jds.2019-18122.
- McDonald P, Henderson AR, Heron S. 1991. The biochemistry of silage, 2nd ed. Marlow: Chalcombe, 340 p.
- Monção FP, Costa MAMS, Rigueira JPS, Moura MMA, Rocha Júnior VR, Gomes VM, Leal DB, Maranhão CMA, Albuquerque CJB, Chamone JMA. 2019. Yield and nutritional value of BRS Capiaçu grass at different regrowth ages. Semina. Agrár. 40(5):2045-2055. doi:10.5433/1679-0359.2019v40n5p2045.
- Monção FP, Costa MAMS, Rigueira JPS, Sales ECJ, Leal DB, Silva MFP, Gomes VM, Chamone JMA, Alves DD, Carvalho CCS, et al. 2020a. Productivity and nutritional value of BRS Capiaçu grass (Pennisetum purpureum) managed at four regrowth ages in a semiarid region. Trop Ani Health Prod. 52:235–241. doi:10.1007/s11250-019-02012-y.
- Monção FP, Rocha Júnior VR, Silva JT, Jesus NG, Marques OFC, Rigueira JPS, Sales ECJ, Silva Júnior AAG, Alves DD, Carvalho CCC, et al. 2020b. Nutritional value of BRS Capiaçu grass (Pennisetum purpureum) silage associated with cactus pear. Iranian J Applied Ani Sci, 10(1):25–29
- Moran JP, Weinberg ZG, Ashbell G, Hen Y, Owen TR. 1996. A comparison of two methods for the evaluation of the aerobic stability of whole crop wheat silage. In: International silage conference, 11., 1996, Aberystwyth. Proceedings … Aberystwyth: University of Wales Aberystwyth, p.162-163.
- National Research Council – International [NRC]. 2001. Nutrient requirements of dairy cattle, 7th rev. ed. Washington, DC, USA: National Academies Press.
- Nocek JE. 1988. In situ and other methods to estimate ruminal protein and energy digestibility: a review. J Dairy Sci. 71(8):2051–2069. doi:10.3168/jds.S0022-0302(88)79781-7.
- Noel RJ, Hambleton LG. 1976. Collaborative study of a semiautomated method for the determination of crude protein in animal feeds. J AOAC Inter. 59(1):134–140. doi:10.1093/jaoac/59.1.134.
- Ortiz-Rodríguez R, Aguilar-Barrera JL, Valdez-Alarcón JJ, Val-Arreola D, Esquivel-Córdoba J, Martínez-Flores HE, Pérez-Sánchez RE. 2016. Effect of adding mucilage from Opuntia ficus indica and Opuntia atropes to raw milk on mesophilic aerobic bacteria and total coliforms. Nova Scientia. 8(16):1–17. doi:10.21640/ns.v8i16.279.
- Pastorelli G, Serra V, Vannuccini C, Attard E. 2022. Opuntia spp. as alternative fodder for sustainable livestock production. Animals (Basel). 12(13):1597–1515. doi:10.3390/ani12131597.
- Pryce JD. 1969. A modification of the Barker-Summerson method for the determination of lactic acid. Analyst. 94:1151–1152. doi:10.1039/an9699401151.
- Qu H, Liu HB, Dong CF, Lu XY, Shen YX. 2014. Field performance and nutritive value of sweet sorghum in eastern China. Field Crops Res. 157:1–7. doi:10.1016/j.fcr.2013.11.015.
- Queiroz FE, Júnior R, Monção VR, Rigueira FP, Silva JPS, Alencar FV, Cordeiro AMS, Gomes MWS, Carvalho VM, Costa CCS, D M. 2021. Effect of replacement of forage sorghum silage with biomass sorghum silage in diets for dairy heifers. Semina: Ciênc Agrár. 43(1):121–140. doi:10.5433/1679-0359.2022v43n1p121.
- Ramos JCP, Rocha Júnior VRR, Monção FP, Parrela RAC, Caxito AM, Cordeiro MWS, Hora FP, Pires DAA. 2021. Effect of replacing forage sorghum silage with biomass sorghum silage in diets for F1 Holstein × Zebu lactating cows. Trop. Anim. Health Prod. 53(1):1–12. doi:10.1007/s11250-020-02503-3
- Ribas WFG, Monção FP, Rocha Júnior VR, Maranhão CMA, Ferreira HC, Santos AS, Gomes VM, Rigueira JPS. 2021. Effect of wilting time and enzymatic-bacterial inoculant on the fermentative profile, aerobic stability, and nutritional value of BRS Capiaçu grass silage. Rev Bras Zootec, 50:1-13. doi. 10.37496/rbz5020200207
- Roth G, Undersander D. 1995. Silage additives. In: Corn silage production management and feeding. Madison American Society of Agronomy, 27-29.
- Souza IDAS, Rocha Júnior VR, Monção FP, Parrela RAC, Cordeiro MWS, Carvalho CCS, Rigueira JPS, Silva FV, Delvaux Junior NA. 2021. Yield and nutritional value of biomass sorghum (BRS 716) managed in different row spacing and maturity at harvest in the semiarid region of Brazil. Semina: Ciênc Agrár. 42(6):3463–3484. doi:10.5433/1679-0359.2021v42n6p3463.
- Souza JF, Rigueira JPS, Albuquerque CJB, Júnior R, Santos VR, Carvalho AS, Leal CCS, Mendes DB, Parrella BM, Silva RAC, et al. 2023. Yield and nutritional value of silage of different sorghum hybrids inoculated with Azospirillum brasilense. J Applied Ani Res. 51(1):424–433. doi:10.1080/09712119.2023.2216760.
- Sucu E, Kalkan H, Canbolat O, Filya I. 2016. Effects of ensiling density on nutritive value of maize and sorghum silages. Rev Bras Zootec. 45(10):596–603. doi:10.1590/S1806-92902016001000003.
- Trachtenberg S, Mayer AM. 1981. Composition and properties of Opuntia ficus-indica mucilage. Phytochemistry. 20(12):2665–2668. doi:10.1016/0031-9422(81)85263-6.