Abstract
This study has aimed to evaluate the current tectonic structure of the Suşehri Basin located on the eastern part of the North Anatolian Fault Zone (NAFZ), one of the most important active faults in Turkey. The work extends earlier investigations of offset and seismicity on the NAFZ and tests a range of evolutionary models. In this study, buried faults have been determined from Ground penetrating radar and magnetic anomalies and possible discontinuities identified by interpolating these data in a region between Gölova and Suşehri. The discontinuities are shown to be linked to negative flower structures formed within the strike-slip fault zone. Quickbird satellite images have been used to map faults and produce kinematic analyses which show that the active stress regime is dominantly strike-slip. However, normal faults and oblique-slip faults are also observed in the basin together with strike-slip faults and the stress regime creating the strike-slip faults is shown to have formed under NW-SE directed transtension. In addition, oblique faults formed under an extensional regime with NNE-SSW direction also occur in the Suşehri Basin as subsets formed under the constraining strike-slip regime. We conclude that the Suşehri Basin started to grow as a fault wedge basin following which it transformed into a pull-apart basin by a south splay on the NAFZ so it is now dominantly a transtensional pull-apart feature.
1. Introduction
The North Anatolian Fault is a 1200-km-long dextral strike-slip fault zone that formed by progressive strain localization in a generally westerly widening right-lateral keirogen in northern Turkey mostly along an interface juxtaposing subduction-accretion material to its south and older and stiffer continental basements to its north (Şengör et al., Citation2005). Along much of its length, this fault zone consists of a few shorter subparallel fault strands that sometimes display an anastomosing pattern. This transform fault zone forms the part of the boundary between the Eurasian Plate to the north and Anatolian Plate to the south, being subparallel to the Black Sea coast (Bozkurt, Citation2001).
Tectonic basins with a range of characteristics have formed on the North Anatolian Fault Zone (NAFZ), provide valuable information on the age and evolution of this intracontinental transform. One of these basins is the Suşehri Basin described as a fault wedge by Koçyiğit (Citation1989). The study area comprises the Suşehri Basin located 130 km northeast of Sivas city and covers the area between Suşehri and Gölova counties (Figure ). The basin is bounded by the Kelkit River to the north and the Kılıçkaya and Çamlıgöze Dams are sited on this river. NAFZ enters to study area from south of Gölova county and extends to Akçaağıl village. It passes through the middle of the basin.
In this study, we investigate the geological and neotectonic features of this basin along a strike length of about 40 km using techniques not previously applied in here, with the aim of producing a detailed neotectonic map of the area between the west of Suşehri and the east of Gölova (Figure ). This region includes the 1939 Erzincan earthquake surface rupture, which has been clearly defined together with morphotectonic structures and fault displacements; in total 350 fault planes have been measured at 37 locations in the Suşehri basin and appropriate kinematic analysis has been applied. In addition, geophysical studies have been applied to define the shallow geological structures.
2. Methods
2.1. Geophysical surveys
2.1.1. Ground penetrating radar (GPR) study
In this study, the GPR application aims to determine invisible surface ruptures screened by sedimentary cover in the inner basin and define fault geometries. Before starting the GPR measurements, specified profile locations are located on satellite images by taking into consideration faults observed in the field and formed in the alluvium. A secondary consideration is access suitable for carrying equipment. For the purposes of this investigation, the measurements were carried out on seven profiles from east to west shown on the neotectonic (Figure ).
The GPR profiles were chosen to be as long as possible in order to cut all possible discontinuities in the basin. A 250 MHz intermediate frequency antenna (~8–9 m deep) was used to take into account the high sedimentation rate and the layer determination in this study is dimensionally accurate to ~20 cm and above in vertical displacement of the 250 MHz antenna. This was the maximum vertical depth reached by 250 MHz antenna in the basin. Possible discontinuities were determined by processing and interpreting the data obtained on each radar section. As an example, we show the evaluation for Profile-1. Here a discontinuity is observed in three different places. To show discontinuities clearly within a realistic total profile length the vertical scale is exaggerated 10 times. The observed layers and faults have been shown on these images (Figure ).
Figure 2. (a) Processed GPR Profile ‘Profile-1(A)’, (b) interpreted GPR Profile ‘Profile-1(A)’. Dashed colored lines shows five different layers, thin red lines show the possible fault zone.
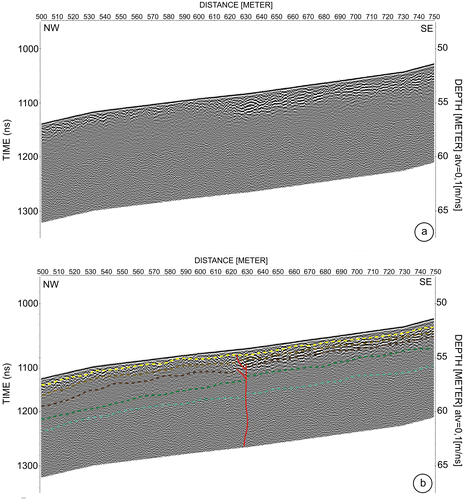
Results obtained for the GPR sections are interpreted by projected satellite images to show possible discontinuities on vertical sections (Figure ). When all GPR section and fault results are shown on the same figure, faults obtained with field studies and discontinuities obtained with GPR studies are largely compatible. Average SW-NE directed topographic sections include GPR sections 3.5 km in length were drawn and faults identified on the GPR data and compared with faults recognized from surface studies. This yields a block diagram of the basin (Figure (a)). Geological cross-sections of the basin extending NW to SE are typically observed in alluvial deposits although Late Miocene–Pliocene age clastic units of the Kadıköy Formation were cut north of Profile-7. The same units are observed both north and south of Profile-6 and south of Profile-5. Pre-Miocene age basement rocks are observed south of Profile-1 and Profile-2.
Figure 3. Discontinuities obtained from the Profile-2 on vertical cross-section and satellite image.

Figure 4. (a) The block diagram showing the discontinuities obtained from GPR data and the Master Fault Zone, (b) Releasing bend and negative flower structure.
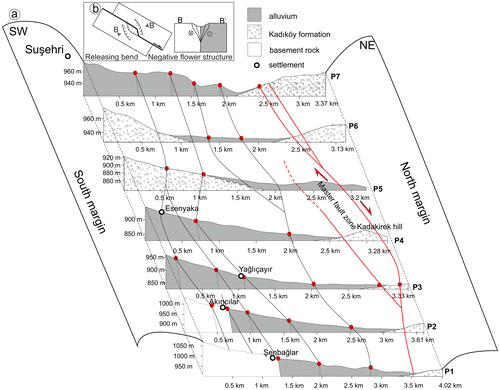
Discontinuities detected by the GPR data in the block diagram are shown as red dots on the topographic surface. The dip of faults obtained by interpretation of the sections are southward in the north and northward in the south, but nearly vertical in the middle of the sections. This relationship is comparable to the form of negative flower structures developed along releasing bends on dextral strike-slip fault zones (Figure (b)).
2.1.2. Study of magnetic field
Measurements of the magnetic field can be used to determine possible buried faults in the basin when rocks of contrasting magnetic susceptibility are offset relative to one another. Magnetic surveys have been performed here at specific locations determined from satellite images and chosen as much as possible to be near to the GPR profiles (Figure (a)); a Proton Magnetometer was used for the measurements. Magnetic anomalies due to faults depend on their displacement, dip and contrast in susceptibility and in the case of near-vertical examples tend to be similar in form to gravity anomalies. Walked profiles were measured perpendicular to the basin at 50 m intervals; it was not possible to complete profiles due to agricultural activity, topography or swampy ground unsuitable for walking.
Figure 5. (a) Chosed locations for Magnetic measurements, (b) the graphic of Magnetic measurement and faults on the satellite image of Profile-15 measured at Location-5.
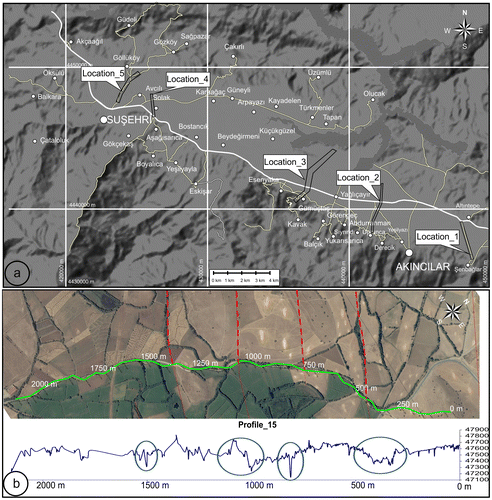
Sections obtained from the profiles are interpreted with the aid of satellite images and Profile-15 is explained as an example. This profile starts northeast of Suşehri and terminates approximately 1 km southeast of Göllüköy; it is approximately 2150 m in length (Figure (b)). Four discontinuities are observed on this profile between 0–500, 500–1000 and 1000–1500 m and correlate with faults mapped by field observations. All of the remaining profiles were analyzed in a similar way to Profile-15 and discontinuities identified by collective comparison of the GPR measurements, magnetic measurements and field observations.
2.2. Field study
2.2.1. Mapping of neotectonic structures
The Suşehri Basin has been shaped by the NAFZ located in this region and fault sets and the 1939 Erzincan Earthquake surface rupture have been mapped in detail between Suşehri and Gölova towns. The location of the NAFZ Master Displacement Zone (NAFMDZ) (Koçyiğit, Citation1989) enters the north side of the Suşehri Basin about 1 km west of Akçaağıl village and cuts the basin at an angle of 112° (Arpat & Şaroğlu, Citation1975; Koçyigit, Citation1988, Citation1989). As noted by previous studies, it enters the study area west of Akçaağıl and continues through the village of Göllüköy to the east. The northern strand of the NAFMDZ enters the basin as two strands and passes south of Çelbaba hill; it then enters Akçaağıl village by passing north of Mihrap hill and the southern strand joins the northern strand by passing south of Akçaağıl village.
Other two strands continue south of Göllüköy and bifurcate into three strands that join further east. The fault then moves up Halilbaba hill as two strands which join here and then enter Kılıçkaya Dam by passing the pasture of Karaağaç as one strand. The fault trace is very clearly observed morphologically in Halilbaba hill and the Karaağaç pasture and is specifically recognized by the surface rupture of the 1939 Erzincan earthquake in the Karaağaç region. Fault planes advance in an oblique direction to the NAFMDZ in Halilbaba hill and are measured as K10–15B, 70–82 GB. The strike-slip right lateral fault has a 25–35° angle of rake and the general course of the NAFZMDZ in this region is K61°B.
Strike-slip fault with normal components having negative flower structure in Kadakirek hill are observed. Right at the edge of Kılıçkaya dam 33 fault planes approximately 100 m long recognized in the defile created by the dam water were measured in the (Pliocene) Kadıköy formation in rock facies including sandstone, conglomerate and claystone. Clearly developed fault lines were not observed in these rocks due to poor degrees of lithification. However, slickensides observed in some claystones were measured at 80o–85o and, by making use of the location of the hanging walls, these faults were identified as dip-slip normal faults. Solutions derived from the use of software Faultkin yield σ1, σ2 and σ3 principal stress axes directions and indicate a σ3 direction of opening for this region of K44°D (Figure ).
Figure 6. (a) 1939 Erzincan earthquake rupture (40°08′39.60′′N–38°15′7.14′′E), (b) elongated ridge in Master displacement Zone and (c) kinematic solution of faults at the Kadakirek hill.
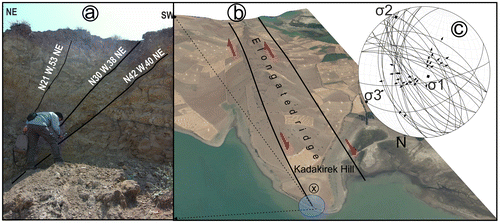
The NAFZMDZ north and south of the elongated ridge corresponds to the surface rupture of the 1939 Erzincan earthquake (Figure ) and the rupture is reported to have caused approximately 4–5 m offsets in field borders although these borders were later readjusted by the villagers. The amount of slip specified by the villagers is compatible with slip data detected at Suşehri as a result of the 1939 Erzincan earthquake (Koçyiğit, Citation1989). Two strands extend further east again to enter the lake region at Kılıçkaya dam. Strands join to the west of Akıncılar and then extend to Ortaköy by continuing through the fields south of Altıntepe village. Fault morphology is very distinct around SE of Ortaköy where surface ruptures also occurred during the 1939 Erzincan earthquake.
The rupture moves up to Süt Lake as a single strand. Alluvial deposits in many places form the boundary of the (Pliocene) Kadıköy Formation. Beyond Eymür Farm it passes through Taşboynu Hill north of Çicekli village continuing through the lake location north of Eskibağ village. It then continues NE of Asağıyeniköy and extends up to Süt Lake. After moving through Süt Lake it continues eastward and outside of the study area from Aşağıtepecik Lake and Çobanlı River. The direction of the fault is N70°W here and Süt Lake and Aşağıtepecik Lake evidently developed within a buckling sag pond structure.
The surface rupture of the 1939 earthquake is observed as three strands. The middle strand starts from the Çobanlı stream in the east and continues up to Aşağıtepecik, Ortaköy, Altıntepe, Yağlıçayır, Küçükgüzel, Yeniköy, Göllüköy and Akçaağıl villages. The southern strand continues to the west by passing south of Geyikpınar, Akıncılar, Esenyaka, Beydeğirmeni, Bostancık, Aşagısarıca and Öksülü villages. These strands are parallel in most places and move in a NW-SE direction. While the south strand ends around Koyulhisar at the west of the study region, the middle strand continues further west and forms the main segment of the 1939 earthquake surface rupture. In addition to these two strands, a third surface rupture located further north around Türkmenler, Kayadelen and Arpayazı villages is approximately 3 km long but does not continue further west. In the north, in addition to these faults, a surface rupture has occurred in Göllüköy and Güdeli villages. The Akçaağıl, Kelkit and Beydeğirmeni fault sets have played the most effective roles in forming the basin. These faults start NW of Akçaağıl Village and continue to the northwest of Altıntepe village to form the Akçaağıl Fault Set. At the NW side of the basin, two faults parallel to each other are observed on the southern slope of Çelbaba Hill as it slopes to the SE. These faults have significant lateral slip (7.5 m at the southeast of Çelbaba hill) and oblique fault properties with a normal component (Figures and (a)).
Figure 7. Right lateral offset of the road at the southeast of Çelbaba hill (40°12′59.8013′′N, 38°02′36.3942′′E).
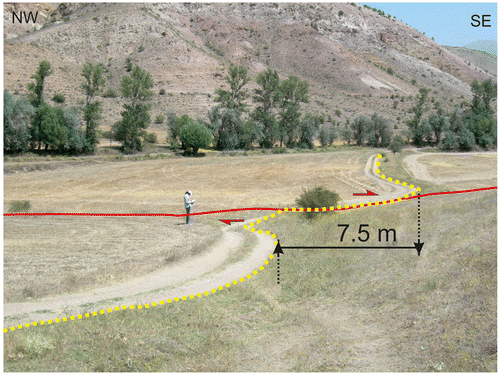
Figure 8. (a) Fault plane observed south of Celbaba hill (40°13′.05′′N–38°2′49.03′′E), (b) left lateral strike-slip fault observed south of Akcaaagil village (40°12′11.33′′N–38°5′18.17′′E), (c) fault planes observed southwest of Yelkesen village (40°13′51.28′′N–38°9′11.39′′E) and (d) deep slip normal fault observed southwest of the Bostancık village and kinematic solution (40°8′55.02′′N–38°9′58.99′′E).
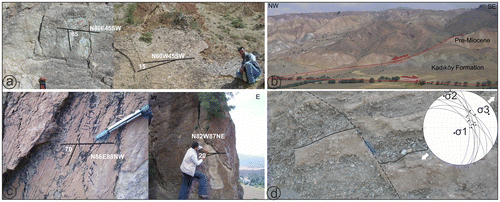
Another fault passing to the north of Akçaağıl village moves approximately 7 km in a N60°W direction and goes up to Güneyli village. Another strand parallel to this is divided into two strands to the north of Karaağaç village. While one strand reaches to Karaağaç village, the other reaches to Güneyli village. One of the faults passing through Güneyli village extends up to Tapan village by passing north of Arpayazı and Kayadelen villages. Other faults parallel to these faults are also observed north of Türkmenler village. Since the fault plane was not clearly detected in the field, these faults trends can only be fixed approximately although morphological data from satellite images point to their continuity. Another strand in the north can be observed along the boundary between the Kadıköy Formation and the basement rocks.
Fault planes are observed on the edge of Kılıçkaya dam south of Tapan village. Small-scale folds are also observed in units of Pliocene age in this region. The axial trends of these folds are N65–70°E and are compatible with the direction of compression in this region. The fault extending into the dam lake field southwest of Tapan village continues approximately 2 km in a direction N60°W and then turns south to end north of Altıntepe village. Faults with strike-slip, left-lateral and oblique-slip normal motions have also been observed east and west of Akçaağıl village with their direction changing between N30–50°E. They end by expanding toward the NAFZ which is morphologically very distinct south of Akçaağıl (Figure (b)). In units belonging to the Kadıköy Formation planes belonging to this fault branch are observed in rock units belong to the basement further north.
The faults which enter the study area at the NW edge of the basin continue along the valley of Kelkit and are named the ‘Kelkit Fault Set’. They can be observed as a single fissure from the north of Çelbaba Hill in the northeast of Akçaağıl to the north of Güdeli village. This fault passes to the north by separating into two strands at the location of Suçıkan and continues up to Yumurcaktaş village. Another fault starting from Çelbaba Hill passes south of Güdeli village. The strand in the south of Güdeli moved again during the 1939 Erzincan earthquake and continues up to Akkaya Hill in a N75°W direction after which it bends to the south and, after continuing approximately 3 km in an E–W direction, turns to a N10°W direction to move up to Dikkaya Ridge.
The slope of each strand of the Kelkit Fault Set is 70–80° north and these faults collectively show en echelon morphology. Some surface ruptures formed by the 1939 Erzincan earthquake follow the Kelkit Fault Set where the northern block fell between 30 cm and 8 m (Koçyiğit, Citation1990). In the field studies made around Güdeli and Yelkesen villages, fault planes on basement rocks were observed and measured. Side bending angles of up to 20–70° in fault lines on these faults identify normal fault components and collectively these data show cumulative oblique-slip characteristics (Figure (c)). Hot water seepages come from the basement rocks northwest of Akçaağıl village and further confirm that this fault is still active.
The faults trending toward Çicekli village start south of Avcılı village and are observed in the south edge of the basin to form the Beydeğirmeni Fault Set. Many of these faults moved to create surface ruptures in the 1939 Erzincan earthquake. In the NW they move to the east to parallel each other south of Avcılı village. One strand starting from Kösedağ stream in the south goes through the Kadıköy Formation and continues to the east of Eskişar village. The other strand goes up to Eskibağ village starting from the fan north of Suşehri. A large part of this fault moved to create a surface break in the 1939 Erzincan earthquake and fault planes are observed along the fault trace. There are advanced and very obvious fault planes in the Kadıköy Formation north of Aşağısarıca village. Most of these fault planes are directed N60–70°W and inclined to the north. Fault planes observed southwest of Bostancık village were observed and kinematic solutions have been made. Since no slickenside on fault plane were observed, the motions were determined to be normal according to the location of the hanging wall and an extension in the N64°E direction was obtained (Figure (d)).
The faults passing north and south of Esenyaka village go to Akıncılar in a direction approximately N60°W. Fault planes in the basement rocks NW of Gümüştaş village and south of Esenyaka village were observed. In the fault planes observed in the basement rocks south of Esenyaka village there are slickensides showing normal fault motions with dip-slip, oblique-slip and strike-slip motions (Figure ). These results show that the region is under stress regimes that change from time to time. The fault direction bends to the north in Akıncılar town where the faults are observed approximately 3 km in an E–W direction before returning to their former direction. These faults have a step structure and are observed in the main units between Akıncılar town and Şenbağlar village; they were activated by the 1939 Erzincan earthquake. These faults continue in the north, south and inside of Şenbağlar village as three strands continuing up to Eskibağ village and the other two strands go to Çicekli village. Thrust faults are observed north of Suşehri, around Güneyli and Üzümlü villages, and between Karacaköy and Çobanlı villages in the east in the study area.
2.2.2. Kinematic analysis of faults
Three hundred and fifty fault planes were measured at 37 locations in the Suşehri basin along a strike length of approximately 40 km (Figure ). The majority of the faults yield strike-slip and dip-slip normal motions in the study area located within the wider strike-slip system. Slickensides are not clear on many fault planes although slips are observable especially in sandstone and conglomerate lithologies in the young units at the margins of basin. The faults with observable slickensides were used for analysis and data obtained from fault measurements were considered by the Carey method. These data were collected from the 11 sites, are shown in Figure , and sites-4a and -4b will be explained as examples. Site-4 includes fault plane measurements in the stream east of Öksülü village and at this site there are clearly observed fault planes with strike-slip measurable (Figure (a) and (b)) on planes cutting horizontal units of the Kadıköy Formation and in serpentinites.
Figure 10. Fault measurement locations, (1)Northwest section of the basin, (2) Southeast section of the basin. (a–b) Fault planes of Site-4 (Northeast of Öksülü village), (a) normal fault, (b) strike-slip fault (40°11′20.98′′N, 38°3′26.27′′E) and (c) kinematic solution for Site-4a, (d) kinematic solution for Site 4-b. (e) Stress ellipsoid obtained from results of kinematic analysis and extension and compression directions.
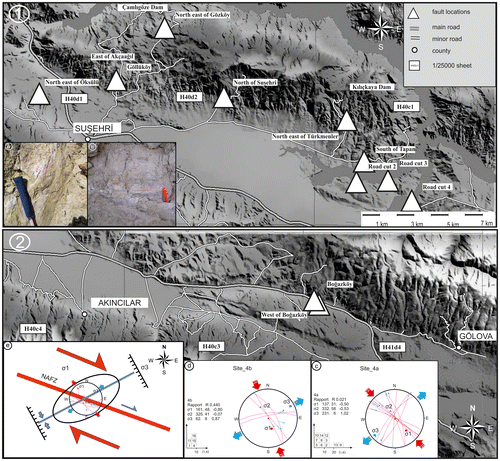
Kinematic solutions have been made by considering strike-slip faults and normal faults respectively at Site-4a and Site-4b. According to these solutions the R value was determined as .021 with N43°W–S43°E trending compression; N51°E–S51°W trending extension was obtained for Site-4a (Figure (c)). For Site-4b on measured oblique normal faults, the R value was determined as .440 with N19°W–S19°E trending compression and N62°E–S62°W trending extension (Figure (d)).
The kinematic analysis was applied using faults evaluated at a total of 10 locations around Suşehri. As a result of this analysis it is seen that the effective regime in this region is determined as strike-slip faulting developed under NW-SE trending compression. In addition to the strike-slip faults, normal- and oblique-slip faults are observed in the basin and we assume that these are collectively part of the strike-slip NAFZ and mechanically compatible with the fault (Özden, Över, & Ünlügenç, Citation2002). We conclude that the fault systems have been formed in a NNE-SSW and NE-SW trending extension regime which has been effective in developing the Suşehri Basin.
Table summarizes the results of the kinematic analysis. When the results of the analysis are evaluated collectively and mapped on a stress ellipsoid, the correspondence between NAFZ and motion mechanism is clearly recognized (Figure (e)).
Table 1. Results of kinematic measurements (N: Number of fault plane, R: stress ratio R = (σ2 – σ1)/(σ3 – σ1), Locations are on the Figure ).
3. Post-miocene stratigraphy
Since neotectonic structures form the main subject of this study, only units formed after the Miocene in the Suşehri Basin and the Upper Kelkit river region have been mapped in detail. The basement comprises serpentinized ultrabasic rocks and flysch-facies sediments overlain by intermediate composition volcanics of Lower-Middle Eocene and Oligocene sediments. The Kösedağ batholiths which are dated as Upper Eocene (Priabonian, see Kalkancı, Citation1974), are emplaced into the Lower-Middle Eocene units. Sedimentation onto this deformed basement started during the Mesozoic and had largely terminated by Pliocene time although the plains forming the present Suşehri basin are largely covered with Quaternary alluvium. Units of Miocene age lie unconformably on units of Oligocene age represented by conglomerates, sandstones, marls and gypsum; in the areas without this transgressive Miocene cover, units of Pliocene age lie unconformably on rocks of Mesozoic age (Ulakoğlu, Citation1986).
The Kadıköy Formation records an entirely terrestrial environment of Upper Miocene-Pliocene-Quaternary age and commences with a thick conglomerate-sandstone alternation passing upward into lacustrine formations with the products of the latter represented by a claystone-limestone sequence. The palaeoenvironment was evidently calm with occasional landslides producing sudden arrivals of poorly sorted material into the streams and lake basins (Özbayrak & Özgür, Citation1992). This sequence covers all units, including those of Oligo-Miocene age, with angular unconformity (Yılmaz & Yılmaz, Citation2010). The Kadıköy Formation covers a large part of the study region (Figure (a)) with the internal parts of the basin are covered by alluvium. A wider spread is observed in the northern part of NAFZ, while at the southern margin it extends to the east and to the west of Suşehri; in the west side it continues up to the west of Beydeğirmeni village, while units of Upper Cretaceous-Paleocene age are observed further west.
Figure 11. (a)1/25000 scaled geological map showing distribution of Pliocene age Kadıköy formation in the study area, (b) alluvial fans developed on Kösedağ and Gemin streams and (c) şenbağlar alluvial fan observed north of Şenbağlar village (40°5′7.39′′N–38°23′52.84′′E).
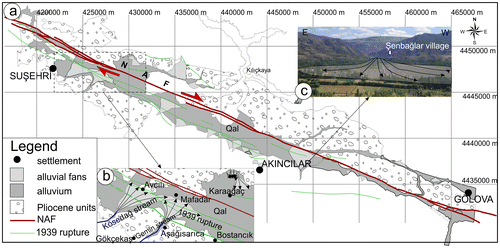
In some areas the Kadıköy Formation includes conglomerate, sandstone (partially grade and cross-bedded), claystone and tuffite levels and basalt blocks, which are decomposed, are observed from place to place inside this unit in sediments formed in lake and river environments. The conglomerates are composed of material derived largely from serpentinites, although they may include volcanics and limestone pebbles derived from underlying Miocene; chert pebbles are observed in the upper levels. The lower levels are polygenetic with coarse to block size particles (10–15 cm thick) at the base and more fine-grained conglomerate in the size range 5–10 cm above; gray and yellow colors dominate with weak intergranular cements. Lower levels are bluish in color with gray-yellow and white colors dominating toward the top. These sediments are 100–150 m thick in the study area but expand to 500 m thickness further east. Some of the levels contain coal mostly in the form of small lenses and pockets (Özbayrak & Özgür, Citation1992).
The Kadıköy Formation is overlain by alluvial fans and sediments which attain their most important development in the interior of the basin as part of a wide drainage network producing an extensive alluvial cover in the vicinity of Suşehri and to the northwest (Figure (a)). This cover comprises main river bed alluvial clastics, terrace pads, talus, alluvial fans and landslide material and has been shifted from place to place by deformation on the NAFZ and its tributaries. Alluvial fan formations are observed on both sides of the basin and are higher on the south side than the north side. A fan of approximately 2 km2 area forms the Kösedağ stream north of Suşehri and fan sediments extending to Avcılı village are limited by the fault. Another large fan formed with sediments brought in by the Gemin stream is also observed further east; the surface rupture of the 1939 Erzincan earthquake passed through and deformed this fan (Figure (b)).
A fan development of approximately 3.5 km2 in an area at Şenbağlar village and on the north side within the Kadıköy formation has also been observed (Figure (c)). It starts in the village and ends in swamps located at the south end of Altıntepe village. Fans in the basin terminate with the Şenbağlar fan further east. Alluvial fans are further observed at Eskibağ and Çiçekli further to the east and at Boğazköy and Dikköy in the north. In addition, many fans are developed on a small-scale depending on control by the active faults seen in the Suşehri region.
4. Two-phased (multi-phased) evolution of the Suşehri Basin
Many researchers agree that the Ladik, Erbaa, Niksar and Suşehri basins formed along the NAFZ are pull-apart basins (Aktimur et al., Citation1989; Barka, Citation1984; Barka, Akyüz, Cohen, & Watchorn, Citation2000; Durukal, Keçer, Tüfekçi, Durukal, & ve Soylu, Citation1984; Keçer & Tüfekçi, Citation1986; Ketin, Citation1976; Şaroğlu, Emre, & Boray, Citation1987; Sav & Sungur, Citation1984; Şengör, Citation1979; Şengör et al., Citation2005; Seymen, Citation1975; Tatar, Piper, Park, & Gürsoy, Citation1995. However, Koçyiğit (Citation1989, Citation1990) has also argued that the Suşehri and Gölova basins are active fault wedge basins.
According to Koçyiğit (Citation1989), the Suşehri Basin may have begun to develop as a pull-apart basin during early Pliocene times and later the NAFZMDS cut the basin (Figure ). This divided the basin into secondary faults and transformed it into a fault wedge basin due to the double divergent character of the Master Displacement Zone (Christie-Blick & Biddle, Citation1985; Crowell, Citation1974). In addition, according to Şengör et al. (Citation2005), the Suşehri Basin has a narrow pull-apart basin structure cutting the master strand of the NAFZ and actually consists of two sub basins comprising the Suşehri and Gölova (Hempton & Dunne, Citation1983; Kazancı, Citation1993; Koçyiğit, Citation1989, Citation1990; Toprak, Citation1988).
Figure 12. Block diagram showing Suşehri fault wedge basin (Koçyiğit, Citation1989).
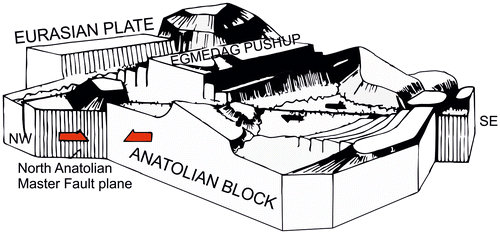
The right step over of the NAFZ in the Suşehri region is considered to be a simple pull-apart basin by Hempton and Dunne (Citation1983). However, the alternative views noted above conclude that the basin is not a pull-apart basin as defined by these authors. Since two main faults in different directions enter the Suşehri basin, the southern fault bends toward to the south and constitutes the southern edge of the basin, while the NW main fault is flat and constitutes the northern edge of the basin. From the fault pattern analysis we propose that the Suşehri Basin may have characteristics of both a fault wedge and pull-apart basins. When the basement units and the faults bounding these units are mapped, it is seen that the Kelkit Fault Set and the Akçaağıl Fault Set have important roles to play in the basin opening. We therefore propose a model for the basin opening that includes three different geometries developed in two phases from the Pliocene to the present day (Figure (a)–(c)).
Figure 13. Geological evolution model phases for Suşehri basin ((a) phase 1, (b) phase 2 and (c) phase 3).
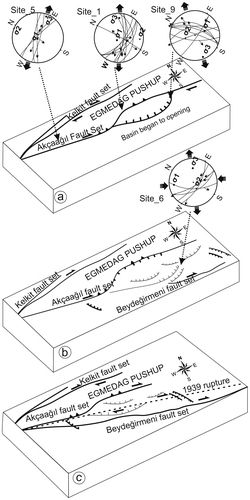
The basin started opening beginning from the south during Pliocene times (Figure (a)). The southern strand (Akçaağıl fault set) makes a restraining bend as to form the Eğmedağ Uplift, bounded by the Kelkit Fault Set in the north, to form as a positive flower structure. In the southern strand, thrust faults developed depending on the configuration of this structure. This fault working with a normal component of motion on small-scale normal faults in the south started to open the basin; the opening directions obtained from kinematic analysis favor this model. NE-SW trending extension obtained from kinematic analysis of Site-5 at the east of Akçaağıl village corresponds to the northwest end of the basin. Site-1 and Site-9 are located in the middle of basin and near to the southeast end of the basin, respectively. Considering the extension directions obtained from these sites, a clockwise rotation is evident from NW to SE.
Phase II: Pull-apart basin, the fault stepped over to the south and the basin has continued to open. Following that stage, the basin progressed as a pull-apart structure (Figure (b)). The result of kinematic analysis of faults measurements near Beydeğirmeni village shows a N37°W–S37°E trending compression and tension regime perpendicular to this. At the present time, the master strand of the NAF continues through the basin with a strike N60–65°W and the surface rupture of the 1939 Erzincan earthquake mostly follows this line (Figure (c)).
Thus, in the light of the present data, the Suşehri Basin is considered to have started as a fault wedge basin and progressed later into a pull-apart basin. This latter regime continues on today. Due to the accumulation of the 1939 surface rupture of the Erzincan earthquake at the NAFMDZ and the southern faults, it is considered that there is more extension toward the south. Thus, the observation of surface rupture only in Türkmenler and Güdeli villages at the north supports this view. In addition, at the east and west ends of the basin, normal faults are observed in the Pliocene and younger units and cross-sections obtained by GPR and magnetic methods identify the current structure of basin as a pull-apart feature.
5. Discussions and conclusions
This study develops a new basin model for the NAFZ between the Suşehri and Gölova regions by combined geological and geophysical study. Satellite images (60 cm resolution) used for observation of morphological structure permit clear mapping of the geometry of the NAFZ and fractures in the middle of the basin have been mapped in detail using field observation, satellite images and geophysical methods. To determine buried faults in the middle part of the basin and understand better the basin geometry, GPR and magnetic methods have been used with profiles having different orientations. Extensional and lithological features of the fill and probable discontinuities not observable on the surface have been detected and fractures correlated with the 1939 Erzincan earthquake surface rupture. In the GPR measurement results, we recognize south edge faults and north edge faults dipping to the north and south, respectively. On a large-scale, this represents a negative flower structure observable in the pull-apart basin and supports the view that the basin is continuing to develop as a pull-apart.
In addition to this study, magnetic measurements were taken along 15 different profiles almost parallel to GPR profiles with the longest being 2153 m. In 13 of these profiles, faults were cut. Although many electricity lines and iron pipe irrigation channels in the basin impaired some of the geophysical measurements, collectively the GPR and magnetic measurements yield compatible data.
The Suşehri Basin is divided by active faults planes which in most of the region are very well developed with resolvable kinematic parameters although slickensides could not be seen in the middle part of the basin in fault planes developed in pebblestone and sandstone lithologies. Results of the kinematic analysis show that the extensional regime forming strike-slip faults in this region developed under the control of a NW-SE directed transpressional regime. In addition to strike-slip faulting, normal faults and oblique-slip faults are observed and considered to be integrated with the right-lateral strike-slip NAFZ as inferred by Özden et al. (Citation2002). These faults are interpreted to have formed in NNE-SSW and NE-SW directed transtensional regimes. Collectively this faulting was effective in forming the Suşehri basin under the regional control of the strike-slip faulting.
Prior to the present study, two models have been proposed for the development of the basin incorporating pull-apart and fault wedge concepts. Koçyiğit (Citation1989) proposed that the pull-apart basin model suggested by Hempton and Dunne (Citation1983) is incorrect and modeled the basin as a fault wedge. From the present work we propose a two phase history and conclude that the basin first opened as a fault wedge basin to the south of the Kelkit and Akçaağıl fault sets and then subsequently converted into a pull-apart basin by right stepover of the NAFZ, a regime which continues to the present day.
Acknowledgments
This work was supported by DPT Project No. 2006–120220K and CUBAP Project No. M356. The authors thank to Süha ÖZDEN (Çanakkale Onsekiz Mart University), Haluk TEMİZ (Cumhuriyet University), Zafer AKPINAR (Cumhuriyet University) and Sinan KOŞAROĞLU (Cumhuriyet University) for their contributions on kinematic analysis, modeling and magnetic studies.
References
- Aktimur, K. T., Tekirli, M. E., Yurdakul, M. E., Ateş, Ş., Ürgün, B. M., Teoman, M. Ş., Keçer, M. Turşucu, A. Genç, S. 1989. Geological Features Around Niksar, Erbaa and Destek. General Directorate of Mineral Research and Exploration of Turkey Report no: 8894. Ankara.
- Arpat, E., and Şaroğlu, F. (1975). Some Important young tectonic events In Turkey. Bull. Geol. Soc. Turkey 18/1, 91–101.
- Barka, A. A. (1984). Geology and Tectonic Evolution of Some Neogen and Quaternary Basins on North Anatolian Fault Zone,Ketin symposium (pp. 209–227). Geological Society of Turkey, Ankara.
- Barka, A. A., Akyüz, S. H., Cohen, H. A., & Watchorn, F. (2000). Tectonic evolution of the Niksar and Taşova-Erbaa pull-apart basins, North Anatolian Fault Zone; their significance for the motion of the Anatolian block. Tectonophysics, 322, 513–530.
- Bozkurt, E. (2001). Neotectonics of Turkey – A synthesis. Geodinamica Acta, 14, 3–30.
- Christie-Blick, N., & Biddle, K. T. (1985). Deformation and basin formation along strike-slip faults. In K. T. Biddle & N. ve Christieblick (Eds.), Strike-slip faulting and basin formation. Society of Economic Paleontologists and Mineralogists. Special Publications 37, 1–34, Tulsa, OK, USA..
- Crowell, J. C. (1974). Origin of late Cenozoic basins in Southern California. Society of Economic Paleontologists and Mineralogists, Special Publications, 22, 190–204.
- Durukal, S., Keçer, M., Tüfekçi, K., Durukal, A., Soylu, C. C. 1984. Geomorphology of Şebinkarahisar, Aluçra (Giresun) and Suşehri (Sivas): General Directorate of Mineral Research and Exploration of Turkey Report no: 7664, Ankara.
- Hempton, M. R., & Dunne, L. A. (1983). Sedimentation in pull-apart basins: Active examples in eastern Turkey. Journal of Geology, 92, 513–530.
- Kalkancı, Ş. 1974. Etude ge’ologique et pe’trochimique de sud de la region de Suşehri: ge’ochronologie du massif syenitique de Kösedağ (NE de Sivas-Turquie) [Geological and petrochemical study southern region of Suşehri: geochronology of syenite massif Kösedağ (NE of Sivas-Turkey)] 3rd cycle Doctorate thesis, Univ. Grenoble, 135 pp., unpublished.
- Kazancı, N. (1993). Kuzey Anadolu Fayı üzerinde tektonik kontrollu depolanma örneği; Suşehri Havzası (Pliyosen-Holosen) KD Türkiye. Doğa-Türk Yerbilimleri Derg., 2, 89–102. Ankara.
- Keçer, M., Tüfekçi, K. (1986). Kuzey Anadolu Fayına bağlı olarak Neotektonik dönemde oluşan Suşehri Havzasının Evrimi [Evolution of Suşehri Basin Formed Neotectonic Period related with North Anatolian Fault]. Jeomorfoloji Der. Sa., 14. Ankara.
- Ketin, İ. 1976. San Andreas ve Kuzey Anadolu Fayları arasında bir kar¸sılastırma [A comparison between the San Andreas and the North Anatolian faults]. Türk. Jeol. Kurumu Bül., 19, 149–154. Ankara.
- Koçyigit, A. (1988). Basic geological characteristics and total offset of the North Anatolian Fault Zone in Suşehri area, NE Turkey Middle East Technical University, Pure and Application Science, 23, 43–48.
- Koçyigit, A. (1988). Basic geological characteristics and total offset of the North Anatolian Fault Zone in Suşehri area NE Turkey Middle East Technical University. Pure and Appl. Sci., 22(3), 43–48.
- Koçyiğit, A. (1989). Suşehri Basin: An active fault-wedge Basin on the North Anatolian Fault Zone, Turkey. Tectonophysics, 167, 11–29.
- Koçyiğit, A. (1990). Tectonic setting of the Gölova Basin: Total offset of the North Anatolian Fault Zone, E. Pontide, Turkey. Annales Tectonicae, 4, 155–170.
- Özbayrak, İ. H., Özgür. (1992). Suşehri-Ağacaağıl (Sivas) Alanının Jeolojisi ve Jeotermal Enerji Olanakları (MTA raporu Rapor No. 10504) [Geology and geothermal energy facilities of Suşehri-Ağacaağıl (sivas). General Directorate of Mineral Research and Exploration of Turkey Report no:10504].
- Özden, S., Över, S., & Ünlügenç, U. C. (2002). Quaternary stress regime change along the eastern North Anatolian Fault Zone, Turkey. International Geology Review, 44, 1037–1052.
- Şaroğlu, F., Emre, Ö., Boray, A. 1987. Active faults and seismicity of Turkey, General Directorate of Mineral Research and Exploration of Turkey Report no:. 8174. pp. 394Ankara.
- Sav, H., Sungur, G. 1984. Earth Science Data Sivas-Suşehri Plain and Its Surroundings: General Directorate of Mineral Research and Exploration of Turkey Report no: 7557, Ankara.
- Şengör, A. M. C. (1979). The North Anatolian transform fault: Its age, offset and tectonic significance. Journal of the Geological Society, 136, 269–282. London.
- Şengör, A. M. C., Tüysüz, O., İmren, C., Sakınç, M., Eyidoğan, H., Görür, N., … Rangin, C. (2005). The North Anatolian Fault: A new look. Annual Review of Earth and Planetary Sciences, 33, 37–112.
- Seymen, İ. 1975. Kelkit Vadisi Kesiminde Kuzey Anadolu Fay Zonunun Tektonik Özelliği: Dr. Eng [Characteristics of Kelkit Valley part of the North Anatolian Fault Zone; PhD Thesis]. İ.T.U. Publications, pp. 192İstanbul.
- Tatar, O., Piper, J. D. A., Park, R. G., & Gürsoy, H. (1995). Palaeomagnetic study of block rotations in the Niksar overlap region of the North Anatolian Fault Zone, central Turkey. Tectonophysics, 244, 251–266.
- Toprak, V. (1988). Neotectonic characteristics of the North Anatolian Fault Zone between Koyulhisar and Suşehri (NE Turkey). METUJ Pure and Application Science, 21, 155–166.
- Ulakoğlu, S. (1986). Suşehri (Sivas) dolayının jeolojisi. İstanbul Üniversitesi Mühendislik Fakultesi Yerbilimleri Dergisi, 5, 1–19.
- Yılmaz, A., & Yılmaz, H. (2010). Kuzey Anadolu Fayı’nın Suşehri ile Gölova (Agvanis) arasındaki bölgede atımı. Cumhuriyet Yerbilimleri Dergisi, 27, 89–96.