ABSTRACT
Thiamine (vitamin B1) deficiency of salmonines, caused by an abundant lipid-rich fish diet and consequently, the abundance of polyunsaturated fatty acids, is called the M74 syndrome in the Baltic Sea. Because of its deleterious effects on wild Atlantic salmon (Salmo salar) stocks and progeny production in fish cultivation, a model was developed to derive the annual female-specific mortality percentages of yolk-sac fry (YSFM) from the free thiamine concentrations of unfertilized eggs. In years with a high M74 incidence, thiamine-deficient females were larger, with a larger condition factor (CF) than non-M74 females. Otherwise, M74 females were generally smaller. The mean CF of M74 females was in most years higher than that of non-M74 females. The model compiled enables the cost-effective estimation of YSFM of individual female salmon, without the incubation of eggs and hatched yolk-sac fry for several months, thus benefitting the management of salmon stocks and their efficient utilization.
Graphical Abstract
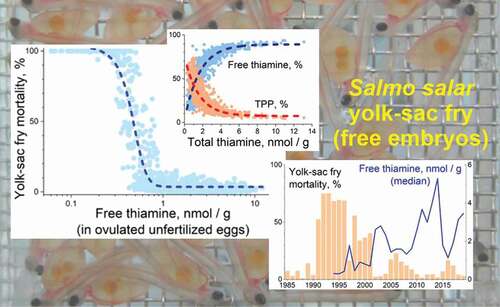
1. INTRODUCTION
1.1. What is thiamine deficiency M74?
As fatty fish species, salmonines suffer from thiamine (vitamin B1) deficiency caused by feeding abundantly on lipid-rich fish and therefore the abundance of n-3 polyunsaturated fatty acids (n-3 PUFAs) (Keinänen et al. Citation2012; Futia et al. Citation2019). First, the requirement of thiamine increases with an increase in the dietary energy content (Woodward Citation1994; Lonsdale and Marrs Citation2019). Second, PUFAs, and especially long-chain PUFAs (LC-PUFAs) among them, are extremely sensitive to lipid peroxidation (Tacon Citation1996; Spector Citation2000). Thiamine, which as the thiamine pyrophosphate (TPP) derivative is needed as a coenzyme in all enzymatic reactions of oxidative metabolism yielding ATP (Lonsdale and Marrs Citation2019) and in fatty acid metabolism (Casteels et al. Citation2007), is depleted in the peroxidation reactions of PUFAs (Gibson and Zhang Citation2002; Depeint et al. Citation2006). High dietary fish-based lipid content increases both the lipid and n-3 LC-PUFA content of fatty predatory fish species, and therefore the susceptibility of their tissues to peroxidation reactions (Alvarez et al. Citation1998; Kjær et al. Citation2008).
In the Baltic Sea region (), lipid-related thiamine deficiency is called the M74 syndrome (Bylund and Lerche Citation1995; Koski et al. Citation1999; Keinänen et al. Citation2012, Citation2018). Thiamine deficiency manifests itself as typical symptoms (Fisher et al. Citation1995; Lundström et al. Citation1999a; Keinänen et al. Citation2000b, Citation2008, Citation2012; Vuorinen et al. Citation2014a) (description of M74 symptoms in Supplemental material) and mortality of different degrees among yolk-sac fry [i.e. free embryos or eleutheroembryos (Balon Citation1975)] of Atlantic salmon (Salmo salar) that had been feeding in the Baltic Sea (hereafter, Baltic salmon or salmon). The equivalent syndrome in many landlocked salmonine species in North American lakes is called Thiamine Deficiency Complex (TDC), previously known as ‘Early Mortality Syndrome’ (EMS) and ‘Cayuga Syndrome’ (Fisher et al. Citation1995; Czesny et al. Citation2009; Futia et al. Citation2019). How soon, calculated as day-degrees (sum of daily temperature degrees), the first thiamine deficiency symptoms and mortalities are noticeable in yolk-sac fry after hatching is related to the thiamine concentration of the eggs (Amcoff et al. Citation1999; Czesny et al. Citation2009; Keinänen et al. Citation2014). Due to oxidative stress caused by lipid peroxidation and thiamine deficiency (Gibson and Zhang Citation2002; Vuorinen et al. Citation2020), the concentration of carotenoids, efficient antioxidants (Miki Citation1991; Shimidzu et al. Citation1996), is also smaller in the eggs of females whose offspring suffer from M74 during the yolk-sac phase (M74 female) than in non-M74 females (Pickova et al. Citation1998, Citation2003; Lundström et al. Citation1999b). However, carotenoid deficiency does not cause M74 mortality (Koski et al. Citation1999).
Figure 1. Locations of the rivers around the Baltic Sea into which salmon entered for spawning and at the mouth of which they were caught for M74 monitoring. The River Tenojoki, which flows into the Arctic Ocean and where the control salmon were caught, is also shown. The map was originally from the International Council for the Exploration of the Sea (ICES)
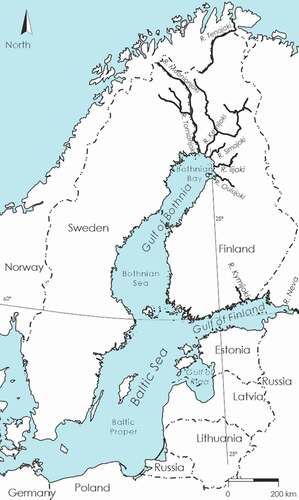
When the thiamine deficiency of salmon is slight, yolk-sac fry can survive until the end of the yolk-sac phase for up to four–six weeks, depending on the water temperature, and only a small proportion, or sometimes almost none, may die, but they may be inactive at that time (Keinänen et al. Citation2014). In the wild, such fry are unlikely to survive. At worst, both female and male brood fish can suffer from severe thiamine deficiency during the pre-spawning period, which appears as unbalanced swimming, and before or after spawning, mortality ensues (Keinänen et al. Citation2014). If such females survive up to egg stripping, M74 symptoms appear in yolk-sac fry shortly after hatching, and they all die soon (Amcoff et al. Citation1999; Keinänen et al. Citation2000b, Citation2008, Citation2014). Males do not contribute to M74 symptoms or the mortality of yolk-sac fry (Koski Citation2002).
The salmon stocks of all the rivers of the Gulf of Bothnia have suffered from M74, as well as the salmon of the River Kymijoki flowing into the Gulf of Finland () (Karlström Citation1999; Keinänen et al. Citation2008, Citation2014; ICES Citation2020a). Most salmon from the stocks of the Gulf of Bothnia migrate for feeding to the southern Baltic Sea, the Baltic Proper, although the migration routes and its timing on average vary somewhat between stocks and between individuals within a stock (Aro Citation1989; Kallio-Nyberg et al. Citation2015; Jacobson et al. Citation2020). Salmon from the River Kymijoki largely feed in the Gulf of Finland (Aro Citation1989; Keinänen et al. Citation2000b).
The main prey fish of the Baltic salmon are sprat (Sprattus sprattus) and smallish herring (Clupea harengus), but the prevalence of these species differs between the different Baltic Sea basins (Karlsson et al. Citation1999; Hansson et al. Citation2001; ICES Citation2020b). As the lipid content varies by species, being on average higher in sprat than in herring, but also by age and the Baltic Sea areas, dietary lipid content and energy density also vary (Keinänen et al. Citation2012). Docosahexaenoic acid (DHA, 22:6 n-3) is also the prevalent n-3 LC-PUFA in Baltic salmon as in sprat and herring (Keinänen et al. Citation2017, Citation2018; Vuorinen et al. Citation2020). However, the fatty acid composition of sprat and herring differs significantly, despite some intra-species differences in them between areas and seasons (Røjbek et al. Citation2014; Keinänen et al. Citation2017). In addition, one fatty acid change in the north–south direction of the Baltic Sea prevails, regardless of species (Keinänen et al. Citation2017; Lind et al. Citation2018). These enabled the estimation of feeding history regarding dietary species, and the feeding area of feeding and spawning salmon using fatty acid signature analysis (Keinänen et al. Citation2018) similarly to other salmonines (Happel et al. Citation2017, Citation2019; Futia et al. Citation2019).
Brown trout (Salmo trutta) feeding in the Baltic Sea have also suffered from thiamine deficiency M74 (Amcoff et al. Citation1999; Landergren et al. Citation1999). Due to their more diverse diet (Landergren et al. Citation1999), more benthic feeding habits, and smaller lipid content, the incidence of M74 among brown trout has been smaller than in salmon. Unlike most Baltic salmon, the salmon of the rivers flowing into the Gulf of Riga and feeding there on a more diverse diet containing no sprat are not known to suffer from M74 (Karlsson et al. Citation1999). Nor have Atlantic salmon feeding in the Atlantic Ocean or other ocean-feeding salmonines displayed thiamine deficiency (Pickova et al. Citation1998, Citation2003; Welch et al. Citation2018).
1.2. Why is monitoring M74 necessary?
Most of the salmon and trout stocks in the rivers flowing into the Baltic Sea have been destroyed as a result of damming or water pollution (Karlsson and Karlström Citation1994). Of the 50 rivers around the Gulf of Bothnia, only eleven rivers on the Swedish and two on the Finnish side have maintained their original, naturally spawning salmon stock (Rappe and Soler Citation1999). The majority of salmon in the Baltic Sea basins originate from the rivers of the northern part of the Gulf of Bothnia, the Bothnian Bay, and most of them nowadays come from natural spawning in the River Tornionjoki and its large tributary the River Muonionjoki (ICES Citation2020a) (). However, salmon that prey in the Gulf of Finland are principally from more regional rivers like the River Kymijoki () (Aro Citation1989; Keinänen et al. Citation2000b).
The largest part of the yolk-sac fry of salmon that had been feeding in the Baltic Sea died of M74 in fish farms, and in Finnish and Swedish salmon rivers in the 1990s for several years (Karlström Citation1999; Romakkaniemi et al. Citation2003; Keinänen et al. Citation2008, Citation2018; ICES Citation2020a). M74 endangered the wild salmon stocks of the Baltic Sea, which had already weakened due to heavy open sea fishing in the 1980s. Baltic salmon stocks approached extinction in the 1990s but recovered because of strict fishing restrictions and large-scale and expensive recovery measures. As a result of compensatory introductions, the proportion of females originating from natural reproduction decreased, being at its lowest of <5% among female salmon ascending the River Simojoki in 1998–2000 (Backman Citation2004; Keinänen et al. Citation2008). M74 has continued, with its incidence annually varying (Keinänen et al. Citation2018).
Although the salmon stock of the River Tornionjoki, for example, has recovered from the collapse of the 1990s and 2000s, many stocks still need to be artificially maintained and strengthened. In any case, the existence of all remaining stocks needs to be safeguarded (see Supplemental material) (ICES Citation2020a), which is a responsibility of the Natural Resources Institute Finland, Luke (until 31 December 2014 the Finnish Game and Fisheries Research Institute, FGFRI).
The Baltic Salmon and Trout Assessment Working Group of the International Council for the Exploration of the Sea (ICES WGBAST) annually requires a prognosis and data on the incidence of M74 and M74 mortality to assess the status of salmon stocks, and based on this among other data, provides recommendations for salmon fishing quotas. In the event that M74 worsens, salmon fishing restrictions may need to be tightened, and the quotas set at lower levels. The worsening of the M74 syndrome would inevitably impair the recruitment success of salmon, which would only become evident in the numbers of ascending salmon in the Bothnian Bay rivers, in most cases after 4–5 years (Karlsson and Karlström Citation1994). To minimize the impact of M74 mortality on smolt production, it must be ensured that sufficiently large numbers of returning salmon have the possibility to spawn. As M74 mortality has varied by almost 60 percentage points among successive year classes of salmon (Keinänen et al. Citation2008, Citation2018; Vuorinen et al. Citation2014a), and the incidence of M74 can be predicted only within one salmon spawning year class at a time, thiamine deficiency must be monitored annually.
In addition to thiamine deficiency, mortalities in the hatcheries also occur for other reasons such as temperatures that are too high, the consequences of technical problems, and Saprolegnia infections (ICES Citation2020a). To provide reliable results, it has been necessary to verify whether yolk-sac fry mortality (YSFM) was caused by thiamine deficiency or another reason. On the basis of free thiamine (THIAM) concentrations in eggs, Finnish M74 monitoring, conducted by Luke, has provided the annual predictions for the proportions of females whose offspring suffer from thiamine deficiency, with variable proportions of them dying, and the proportions of females all of whose offspring die of thiamine deficiency (Keinänen et al. Citation2014, Citation2018). Thus, far it has not been possible to predict the percentage of female-specific M74 mortality on the basis of the THIAM concentrations.
The aims of the present investigation were 1) to develop a model to predict the percentage of female-specific YSFM of salmon from the thiamine concentration of ovulated unfertilized eggs for the cost-effective monitoring of lipid-related thiamine deficiency syndromes, 2) to validate the grounds for how we came to this guideline, 3) to examine the variation in the incidence of the thiamine deficiency syndrome M74, and 4) to evaluate whether the other indicators used or their combinations would be of value in the prediction of the incidence of M74 instead of thiamine alone.
2. MATERIAL AND METHODS
2.1. Salmon stocks and M74 monitoring
Monitoring of M74 in Atlantic salmon (Salmo salar L.) ascendants has been performed for the Rivers Simojoki, Tornionjoki, and Kemijoki, all of which drain into the Bothnian Bay of the Gulf of Bothnia, and for the River Kymijoki, which flows into the Gulf of Finland (). In the Rivers Simojoki and Tornionjoki, salmon reproduces naturally. Salmon in the River Kemijoki are maintained by annual releases of hatchery-reared smolts of the stocks of the Rivers Iijoki and Tornionjoki. In the River Kymijoki, there is now some natural reproduction of salmon that originate in the stock of the River Neva.
For River Simojoki salmon, female-specific egg and yolk-sac fry incubations were originally started to study the possible effects of environmental toxicants on salmon reproduction (Vuorinen et al. Citation1997), so data on their mortality are continuous from the 1985/1986 reproductive period (spawning year/hatching year) onwards. However, the proportions of M74 females are reported only during M74 monitoring proper, from 1991/1992 onwards. River Simojoki salmon could not be caught in 2018 because the river mouth was in a quarantine safety area (Infectious Haematopoietic Necrosis, IHN) at a rainbow trout (Oncorhynchus mykiss) net pen culture. Nor were they caught in 2019. M74 monitoring for River Tornionjoki salmon started from 1993/1994, but data are missing for 1995/1996, 1996/1997, 2004/2005, 2010/2011, and 2013/2014–2016/2017. Salmon of the River Kemijoki were included in monitoring in 2005/2006–2010/2011 and 2018/2019. M74 for salmon from the River Kymijoki was monitored in 1994/1995–1999/2000 and 2001/2002–2006/2007. In addition, in the falls of 2013 and 2019, a few River Kymijoki salmon females were caught for the renewal of the brood stock. In addition, naturally reproducing salmon from the Arctic River Tenojoki, which drains into the Arctic Ocean (), were sampled as a non-Baltic control during 1997/1998. More detailed information on the rivers and salmon stocks is provided in the Supplemental material.
2.2. Sampling and collection of fish data
For monitoring, salmon of the stocks of the Rivers Simojoki and Kemijoki were caught by trap net at the mouth of the river between June and early July, and salmon from the stock of the River Tornionjoki were caught during July and August in different places and using different methods (cf. Supplemental material; Keinänen et al. Citation2000b, Citation2008; Vuorinen et al. Citation2014a).
After capture, the salmon were transported to the hatchery, where they were anesthetized singly in a water bath with MS-222 (tricaine methane sulfonate, 130 mg l−1), which was neutralized with NaHCO3, and aerated to remove CO2 and to oxygenate the water (Keinänen et al. Citation2000a; Vuorinen et al. Citation2003). The total length and weight were measured, a few scales taken for age determination, and fish were marked with Carlin-tags for later individual identification ( and in Vuorinen et al. Citation2014a). The salmon were kept until the eggs were stripped at the Lautiosaari hatchery and later at the Luke’s Keminmaa hatchery in glass fiber or concrete basins with water flowing from the River Kemijoki. River Kymijoki salmon were caught between late October and early November and kept in wooden keepnets in the River Kymijoki next to the hatchery of the local fishery association until the eggs were stripped. River Tenojoki salmon were caught in the river in August 1997 by local fishers. Further details on catching salmon are given in the Supplemental material.
Figure 2. Yolk-sac fry mortality (YSFM), proportions of females with partial M74 mortality among their offspring (M74 females) and those females whose offspring all died of M74 [M74 (100%) females], and the median free thiamine (THIAM) concentration in unfertilized eggs of salmon from the Bothnian Bay rivers (Rivers Simojoki, Tornionjoki, and Kemijoki) during the 1985/1986–2019/2020 reproductive periods. The number of females is given in parentheses. The rectangle defines the reproductive periods, when M74 symptoms and YSFM were observed in experimental incubations in the laboratory
![Figure 2. Yolk-sac fry mortality (YSFM), proportions of females with partial M74 mortality among their offspring (M74 females) and those females whose offspring all died of M74 [M74 (100%) females], and the median free thiamine (THIAM) concentration in unfertilized eggs of salmon from the Bothnian Bay rivers (Rivers Simojoki, Tornionjoki, and Kemijoki) during the 1985/1986–2019/2020 reproductive periods. The number of females is given in parentheses. The rectangle defines the reproductive periods, when M74 symptoms and YSFM were observed in experimental incubations in the laboratory](/cms/asset/db516605-b07b-493d-a174-889964815202/gmfw_a_1941942_f0002_oc.jpg)
Figure 3. The annual mean a) concentration of total thiamine as a sum of free thiamine (THIAM), thiamine monophosphate (TMP), and thiamine pyrophosphate (TPP) in unfertilized eggs of salmon ascending the Rivers Simojoki, Tornionjoki, and Kemijoki and caught for monitoring of M74 during the 1994/1995–2019/2020 reproductive periods, and b) yolk-sac fry mortality (YSFM) and the proportions of THIAM, TMP, and TPP of total thiamine. The last column in both figures depicts the respective thiamine values for salmon ascending the River Tenojoki from the Arctic Ocean in 1997. The number of observations is given in parentheses
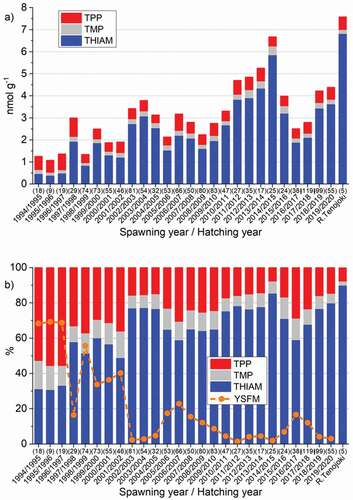
Between late September and early November, depending on the stock, salmon were stripped of ovulated eggs, for which they were anesthetized as described above. The total length was measured, and with some exceptions, the salmon were weighed before and after stripping. Fulton’s condition factor (CF) was calculated as CF = 100 * (total weight before stripping, g)/(total length, cm)3. The intensity of the orange color of the unfertilized eggs was visually recorded by scoring from light yellow to dark orange on a scale of 1 to 5. A sample for the biochemical analyses of eggs was taken by collecting ovulated eggs on a sieve to filter off the ovarian fluid, and approximately 1 dl of unfertilized eggs were sealed in a ziplock plastic bag as a single layer and immediately frozen at −20°C ( in Vuorinen et al. Citation2014a). As soon as possible, the egg samples were transported frozen to the laboratory, where they were preserved at −80°C until biochemical analysis (for 4–8 weeks). After the stripping of the eggs, the salmon were killed, and ca. 200 g of the left epaxial white muscle was cut into a ziplock plastic bag for other determinations and the biobank, and frozen. Veterinarians in the laboratory of the Finnish Food Authority (until 31 December 2018 the Finnish Food Safety Authority Evira) in Oulu, and in the case of the River Kymijoki salmon, in Helsinki, investigated the M74-monitored salmon for the occurrence of possible diseases. These were never detected.
Figure 4. Relationships of a) the concentrations of free thiamine (THIAM), thiamine monophosphate (TMP), and thiamine pyrophosphate (TPP) and b) proportions of THIAM and TPP with the total thiamine (TotTH), and c) the relationship between the proportions of TPP and THIAM in the unfertilized eggs of salmon of the Rivers Simojoki, Tornionjoki, Kemijoki, and Kymijoki during the 1994/1995–2009/2010 reproductive periods. The respective models are presented and for all the models applies: p <0.0001 and N = 995
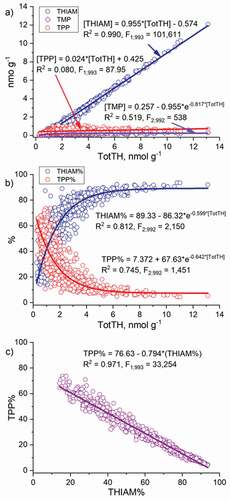
The eggs were fertilized according to the aquaculture protocol ( in Vuorinen et al. Citation2014a), after which the eggs were allowed to swell for ca. three hours before distribution onto incubation trays on a female basis. After embryos reached the ‘eyed stage’ at the hatchery in February, the incubation trays were cleaned, and the dead eggs were removed. At this stage, 200 eggs with a live embryo from each egg group were counted and moved onto separate trays to monitor mortality at the hatchery. Approximately 2–3 weeks before the expected hatching in the spring (on the basis of the cumulative day-degrees) an additional 1 dl of eggs per female were packed dry into specific polystyrene boxes, each holding 16 egg batches, with ice on the topmost compartment, and transported as flight cargo to Helsinki for laboratory incubation between 1995 and 2010.
Figure 5. Box plots of free thiamine (THIAM) concentrations in unfertilized eggs of salmon ascending the River Simojoki and caught for M74 monitoring in 1994–2017. Whiskers depict the minimum and maximum observations, upper and lower parts of the boxes 25 and 75% of observations, horizontal lines the median value, and diamonds the mean concentration. The number of females is given in parentheses
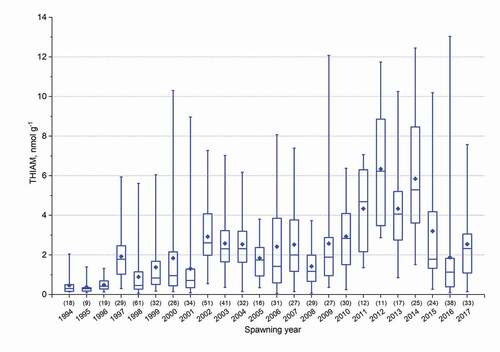
Eggs and yolk-sac fry (eleutheroembryos) can be used in experiments because the use of these developmental phases is not included in animal experimentation legislation within the European Union. Moreover, M74 monitoring, including the handling of adult salmon, is performed as part of fish cultivation of Luke.
2.3. Experimental incubations of eggs and yolk-sac fry
In the FGFRI laboratory in Helsinki, eggs during the rest of their embryonic phase and yolk-sac fry after hatching were incubated in a thermostatted room. The room temperature was first adjusted to 4°C, and according to the progress of the development, the temperature was increased to 6–8°C. The incubations were performed on experimental trays (Keinänen et al. Citation2000b, Citation2008; Figure 11 in Vuorinen et al. Citation2014a) constructed of acryl and acid-resistant stainless steel mesh. Water flow, using silicone and polyethylene tubing and polyethylene basins, was arranged through the bottom mesh upward through the layer of eggs. Helsinki city tap water was used after filtration through activated carbon, and approximately one-third of the water was renewed with freshwater per hour, and the water was recirculated through the incubation system with UV sterilization. Each tray could hold five egg batches (Figure 11 in Vuorinen et al. Citation2014a) so that at the start, 115–150 eggs (varied annually) were counted in one compartment, one was left empty to await hatched fry, and one held extra eggs that were sampled for observation. To handle each offspring group and its incubation trays, separate equipment such as brushes and pipettes were used. The water temperature in the trays was recorded daily to count the day-degrees from mean hatching onwards.
Within 1–3 days of hatching, the color of the large oil droplet in the yolk of the yolk-sac fry was estimated by sight against a 6-degree color plate, compiled of previously photographed yolk -sacs ( in Keinänen et al. Citation2014). Every day (including weekends), the trays were inspected, dead eggs were removed and recorded, and hatched yolk-sac fry were picked up with a special polyethylene pipette, transferred onto their own compartment of the tray, and recorded. Yolk-sac fry were inspected daily for possible symptoms, and dead fries removed until ca. 450 day-degrees from hatching, which was in their alevin phase (Balon Citation1975). In this phase, fry still had some yolk left but would have started external feeding.
The THIAM concentrations of unfertilized eggs were compared with the observations from the experimental incubation. It was thus verified that YSFM was caused by thiamine deficiency. The annual incidence of M74 was reported as three numbers (Supplemental material, Figure S1): 1) the mean (± SE) YSFM of all females; 2) the proportion of M74 females, that is, those whose offspring had M74 symptoms and thiamine deficiency-related mortality of different degrees; and 3) the proportion of M74 females all of whose offspring died of M74 [M74 (100%) females].
2.4. Data for the calculations
Only female salmon at spawning (spawners) were included in the data. For the calculations of YSFM and connections of YSFM with thiamine content and other parameters, only the data for the Rivers Simojoki, Tornionjoki, Kemijoki, and Kymijoki for the 1994/1995–2009/2010 reproductive periods were included although the entire data from 1985/1986 to 2019/2020 for these rivers were used in the calculations on some occasions ().
During 1994/1995–2009/2010, yolk-sac fry incubations of different salmon stocks were performed annually at the same time in the same conditions in the laboratory. As monitoring of yolk-sac fry and inspection of M74 symptoms were performed daily, the procedure was more detailed and careful than could be carried out in hatchery conditions, where the mortality of yolk-sac fry was recorded twice during the entire incubation period. The frequency of the different determinations for salmon females and offspring for 1994/1995–2009/2010 is presented in Supplemental material Table S1: the determination of age; the analysis of the concentrations of thiamine components; total carotenoids and astaxanthin in the eggs; and the estimation of egg color and/or the color of the large oil droplet within the yolk.
For 1994/1995–2009/2010, female salmon from each of the rivers were treated separately, combined for the Rivers Tornionjoki and Simojoki with natural reproduction, or combined for the Rivers Simojoki, Tornionjoki, and Kemijoki as ‘Bothnian Bay salmon’; hereafter, ‘BB salmon or BB females’ and ‘BB rivers.’ Salmon from the River Kymijoki were treated separately due to their different feeding migration (Aro Citation1989; Keinänen et al. Citation2000b). In addition, the body form of these salmon differs slightly from that of BB salmon, meaning CF comparisons were not appropriate.
2.5. Analysis of thiamine components
Thiamine components were analyzed in samples of ovulated unfertilized eggs by high-performance liquid chromatography (HPLC) according to Vuorinen et al. (Citation2002). The components comprised of two phosphorylated thiamine derivatives, thiamine monophosphate (TMP), and TPP, as well as unphosphorylated THIAM, which were summed up as total thiamine (TotTH). The method was only slightly modified over the years, and the updating is as follows: Approximately 0.5 g of eggs was weighed and homogenized (IKA Euro-ST P DV) with 2.0 ml of 2% tricarboxylic acid (TCA), and the tube was incubated at 100°C for 10 min. Homogenization was repeated with 1.5 ml of 10% TCA. The sample was centrifuged (Beckman Avanti J-30 I) at 14,000 × g for 15 mins at a temperature of +4°C. The supernatant was washed four times with an equal volume of ethyl acetate-hexane (3:2). To convert thiamine components into their corresponding thiochromes, an aliquot of 425 µl of supernatant was taken, and 75 µl of 0.1% potassium hexacyanoferrate in 1.2 M (1.5 M for standards) sodium hydroxide was added. The sample was filtered (Spartan 13/0.45 RC, S&S) prior to the HPLC run. The standards were subjected to the same procedure as the tissue samples with the mentioned exception. The injection volume was 20 µl, and the analysis time was 27 minutes, using a linear gradient flow of eluents: Eluent A was a 0.5% methanol and 99.5% 35 mM potassium phosphate buffer, pH 8.4; eluent B consisted of a 55% methanol and 45% 35 mM potassium phosphate buffer, pH 8.4. All reagents were at least of analytical grade. The HPLC apparatus consisted of two Waters 510 pumps, a Waters 717 Plus Autosampler equipped with a cooling unit (sample tray at 4°C), a thermostatic (40°C) column oven, a Waters 474 fluorescence detector, and an Empower 1 Chromatography Manager.
Along with the samples, a subsample of the laboratory control sample (salmon egg homogenate) was processed and analyzed along with the samples for quality assurance. The comparability of the analyses over time was ensured by analyzing the batches of successive laboratory control samples in parallel when the control batch was changed. The analysis results were not corrected for recoveries. No certified reference material for thiamine is available.
2.6. Analysis of carotenoids and lipid
The total carotenoid concentration of ovulated unfertilized eggs was measured spectrophotometrically according to Pettersson and Lignell (Citation1999), with slight modifications as described in Vuorinen et al. (Citation2020).
Astaxanthin concentration was determined according to Pettersson and Lignell (Citation1999) in the cyclohexane extract above, using the same HPLC apparatus as for thiamine analysis, but equipped with a vis/UV detector.
The total lipid content in the muscle samples was determined according to Tarhanen et al. (Citation1989) for the samples from 1988 to 1993, and for the 2001 samples, as in Vuorinen et al. (Citation2012). In the 2004–2016 samples, the total lipids of muscle were determined on the basis of the Schmid-Bondzynski-Ratzlaff procedure (ISO Citation2004) as in Keinänen et al. (Citation2017). The good congruence of the method used in Vuorinen et al. (Citation2012) and ISO (Citation2004) was ascertained by running 24 sprat and herring homogenates through both methods (y = 1.01 x – 0.53, R2 = 0.982, p <0.001).
2.7. Statistical analysis
Spearman rank correlations were applied to examine correlations between variables, and Bonferroni adjustment was used to control the risks of erroneous decisions of significance in multiple simultaneous comparisons. A one-way ANOVA with SNK post hoc test (p <0.05) was applied to test the differences in the mean values of various parameters between the salmon from the river stocks. The same testing was used in comparing significant differences in YSFM and THIAM between the classes of egg and yolk oil droplet color. A general linear model was applied to test the relationship between the concentrations of THIAM or TPP and TotTH, the proportions of TPP and THIAM, and the total carotenoid and astaxanthin concentrations in the eggs. Exponential models were fitted for the relationships of the concentrations of TMP with those of TotTH, and the proportions of THIAM and TPP with the concentration of TotTH. A power function was fitted for the relationship between the THIAM and total carotenoid concentrations. A dose response model for the relationships between the concentrations of thiamine components and YSFM was compiled, which enabled the calculation of female-specific yolk-sac fry mortality percentages from thiamine concentrations, and the threshold concentrations for incipient M74 mortality and 100% mortality of offspring, and in addition, different LC values. In all calculations with percentage values, they were used untransformed (Warton and Hui Citation2011).
For the models YSFM vs. concentrations of thiamine components, and for the relationship between THIAM and carotenoid concentrations, OriginPro 2020 software was used. Other statistical calculations were performed using the Statistical Analysis System software (SAS ver 9.4).
3. RESULTS
3.1. Variation in the incidence of M74
The M74 syndrome suddenly broke out in the spawners of 1991 in the River Simojoki stock, but increased mortality was already observed among the offspring of the River Tornionjoki 1990 spawners (Supplemental material, Figure S1). M74 incidence remained generally very high through the mid-1990s and remained high until the early 2000s. The variation of M74 is presented in as YSFM, proportions of M74 females, and those females whose offspring all died of M74, and as the median THIAM concentration in eggs, for BB salmon during 1985/1986–2019/2020. The comparison between the mean and median THIAM concentrations in relation to YSFM for River Simojoki salmon in the 1994–2009 spawning years is presented in Supplemental material Figure S2. In some years, such as 2006, the median was somewhat better related to YSFM than the mean, probably because of a large intra-annual variation in THIAM concentrations. However, these two parameters changed very similarly between years.
During 1992/1993–1993/1994, all the offspring of even 75% of BB females died due to M74. In 1994/1995–1996/1997, the percentages of M74 females among BB salmon were 76–92% (mean YSFM 66–76%), with annual mean egg THIAM concentrations of <0.5 nmol g−1. In 1997/1998, when the proportion of M74 females among BB salmon decreased to 31%, the mean THIAM concentration multiplied to nearly 2 nmol g−1, and the mean YSFM decreased to below 25%. However, in the next reproductive period (1998/1999), when the proportion of M74 females again increased and was 57%, THIAM concentration decreased to 0.8 nmol g−1, and YSFM more than doubled to 50%. During three successive years (1999/2000–2001/2002), the proportion of M74 females remained at 34–45%, the annual mean concentrations of THIAM ranged between 1 and 2 nmol g−1, and mean YSFM between 32% and 39%. Thereafter, M74 eased off for three reproductive periods (2002/2003–2004/2005), so that only sporadic M74 females were detected, individual THIAM concentrations were 0.157–8.985 nmol g−1, and M74 mortality was insignificant. After worsening for several years, so that in 2005/2006–2009/2010, annual mean THIAM concentrations were 1.6–2.2 nmol g−1, and YSFM between 9% and 23%, M74 was finally absent in 2011/2012–2014/2015. However, thiamine deficiency worsened again for 2015/2016–2017/2018 ().
During the laboratory incubation periods (1994/1995–2009/2010) for the two naturally spawning stocks, those from the Rivers Simojoki and Tornionjoki, the difference in YSFM was a maximum of 25 and an average of 2 percentage points; in River Simojoki salmon, YSFM was in 6 cases larger and in 8 cases smaller than in River Tornionjoki salmon. However, the differences were not significant (Supplemental material, Table S2), apparently due to a large annual variation in YSFM (from 100% to close to zero). Considering the 1994–2017 period, when salmon from both stocks were included in monitoring, the mean egg THIAM concentration for River Simojoki salmon was in 10 cases larger and in 4 cases smaller than for River Tornionjoki salmon (). However, the difference was significant in only 3 years: in 2000 and 2012, the larger THIAM concentration was for River Simojoki salmon, and in 2003, for River Tornionjoki salmon.
Table 1. Mean (± SE) concentrations (nmol g−1) of unphosphorylated thiamine (THIAM) in ovulated unfertilized eggs of salmon ascending three rivers of the Bothnian Bay and the River Kymijoki during the M74 monitoring in the 1994/1995–2019/2020 reproductive periods
In general, YSFM of River Kemijoki salmon was an average of 10 percentage points larger than YSFM of salmon from the Rivers Simojoki and Tornionjoki during 2005/2006–2009/2010, when salmon from the River Kemijoki were included in monitoring (Supplemental material, Table S2). However, the difference in YSFM was significant only in 2006/2007, when it was larger in River Kemijoki salmon than in River Tornionjoki salmon (Supplemental material, Table S2). The egg THIAM concentration of River Kemijoki salmon was annually an average of 0.77 nmol g−1 smaller than that of River Simojoki and River Tornionjoki salmon, but the differences were not significant. Only in 2005/2006, when the egg THIAM concentration of River Kemijoki salmon among the rivers was smallest, was it significantly smaller than that of River Kymijoki salmon ().
In 1994/1995, YSFM of River Kymijoki salmon was significantly smaller than YSFM of River Simojoki salmon, and in 1998/1999, it was significantly smaller than River Tornionjoki salmon (Supplemental material, Table S2). In all, YSFM in River Kymijoki salmon was an average of 12 percentage points lower, and egg THIAM concentration was an average of 0.19 nmol g−1 larger than for BB salmon. However, in 1997/1998, when M74 temporarily eased among BB salmon, YSFM of River Kymijoki salmon was largest among the rivers, and egg THIAM concentration was significantly lower for River Kymijoki salmon than for BB salmon ( and Supplemental material, Table S2).
In 1996/1997, the mean YSFM of River Kymijoki salmon was 56%. Although the proportion of M74 females was then as high as 79%, only 29% were females, all of whose offspring died due to thiamine deficiency. The difference between the proportion of M74 females and YSFM was therefore more than 20 percentage points.
3.2. Content of thiamine components in the eggs
During the 1990s, the mean TotTH concentration in the eggs of BB females was mostly <1.5 nmol g−1, apart from 1997, when it was more than 2 nmol g−1 (). Since 1999, and particularly since the early 2000s, the TotTH concentrations have been significantly higher, although they have varied greatly between years. In BB females, the highest mean TotTH concentrations of eggs were detected for the spawners of 2011–2014, when no M74 mortality was recorded in Finnish M74 monitoring. However, the TotTH concentrations were even then significantly (p <0.05) lower than in the eggs of the Arctic River Tenojoki salmon.
Among the thiamine components, the concentration of THIAM varied most between and within years. In the data on BB salmon in 1994–2019, the variation between annual mean values in concentrations of THIAM was 15-fold (0.385–5.847 nmol g−1), whereas that of TMP and TPP were 3.3-fold (0.125–0.410 nmol g−1) and 2.3-fold (0.358–0.840 nmol g−1), respectively, and for the concentration of TotTH, the variation was 6.2-fold (1.079–6.676 nmol g−1). THIAM was the largest thiamine component in most years. However, during the 1994/1995–1996/1997 period, i.e. the years of the highest M74 incidence, the TPP component was largest ( and b). The mean THIAM concentration in the eggs of the control salmon from the Arctic River Tenojoki was 6.812 ± 0.371 nmol g−1, and that of TPP was 0.591 ± 0.077 nmol g−1.
In the eggs of BB salmon from 1994–1996, the proportion of THIAM was <35%, whereas that of TPP was >50% (). After the spawning year 1997, the proportion of THIAM was >50%, and the proportion of TPP was correspondingly 10–35%. The proportion of TMP was quite constant and small throughout (). In the eggs of individual River Tenojoki salmon, the proportion of THIAM was 87–91%.
The THIAM concentration had a strong linear relationship with the TotTH concentration (R2 = 0.990) among BB and River Kymijoki salmon in 1994/1995–2009/2010 (). The concentration of TMP was less strongly, and the TPP concentration very weakly, related to the TotTH concentration, R2 = 0.519 and R2 = 0.080, respectively.
The proportions of THIAM and TPP were oppositely related to the TotTH concentration among BB and River Kymijoki salmon during 1994/1995–2009/2010, R2 = 0.812 and R2 = 0.745, respectively (), and the proportions of THIAM and TPP had a strong negative linear (R2 = 0.971) relationship (). Although the proportions of TPP and THIAM of the TotTH concentration changed oppositely (), the change in the TotTH concentration mainly resulted from the large variation in the THIAM concentration ().
Typically, THIAM and TotTH concentrations varied widely among egg batches of females in each of the rivers in the spawning years. The widest annual variation in the concentration of THIAM, 0.098–13.040 nmol g−1, i.e. a 133-fold difference, was detected in the eggs of the River Simojoki salmon in 2016 (). However, during the most severe M74 years, in the eggs of 1994–1996 River Simojoki spawners, the variation in the THIAM concentration was small.
3.3. YSFM in relation to egg THIAM concentration
THIAM concentration had a stronger negative relationship with YSFM than the concentrations of TotTH or phosphorylated derivatives TPP and TMP, and the concentration of TPP had the weakest relationship (). Among the thiamine components, the concentration of THIAM varied most and widely in relation to YSFM ( and b). Although the concentrations of TPP and TMP also decreased with the increase in YSFM, they were more or less constant over the range of YSFM as seen from the annual mean values of the entire monitoring period () or from the individual egg batches of 1994/1995–2009/2010 (, d).
Table 2. Spearman correlations of various parameters (correlation coefficient, significance, and number of observations) in female salmon returning to the Bothnian Bay Rivers Simojoki, Tornionjoki, and Kemijoki, and monitored for M74 with experimental laboratory incubations in 1994/1995–2009/2010
Figure 6. Relationship of the annual mean yolk-sac fry mortality (YSFM), a) with the annual mean concentrations of free thiamine (TIAM), thiamine monophosphate (TMP) and thiamine pyrophosphate (TPP), and relationship of YSFM with the concentrations of b) THIAM, c) TPP, and d) TMP in ovulated unfertilized eggs of individual females. Note the difference of scales in the x-axis of the panels
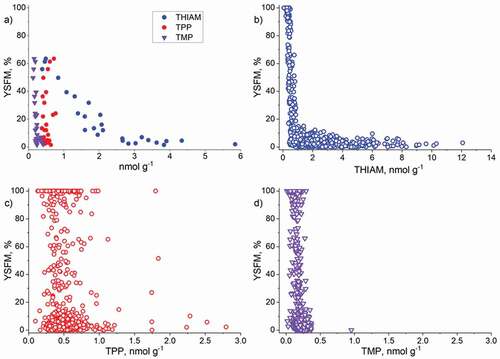
No increased mortality due to thiamine deficiency during the embryonic phase prior to hatching was detected. The offspring of all M74 females hatched, but for some females, classified as the M74 (100%) females, all offspring died within 1–2 weeks. The concentration of THIAM in the eggs of such females ranged between 0.060 and 0.448 nmol g−1, so that for 10 females it was <0.1 nmol g−1, while the mean was 0.218 ± 0.008 nmol g−1 (N = 127), and the median was 0.204. For the TotTH, the respective parameters were: range 0.253–1.579 nmol g−1, mean 0.755 ± 0.022 nmol g−1 and median 0.752 nmol g−1.
Dose-response models between YSFM and the thiamine concentrations of BB salmon and River Kymijoki salmon of 1994/1995–2009/2010 were constructed. As the models for BB and River Kymijoki salmon separately gave results with non-significant differences, the data concerning them were combined ().
Figure 7. Relationship of yolk-sac fry mortality (YSFM) among offspring of individual females with the concentration of free thiamine (THIAM; a logarithmic scale) in their unfertilized eggs from the stocks of the Bothnian Bay rivers (Rivers Simojoki, Tornionjoki, and Kemijoki) and River Kymijoki for the 1994/1995–2009/2010 reproductive periods. A dose response model for estimation of YSFM from the concentration of THIAM with the 95% confidence band is presented, and the threshold THIAM concentrations for incipient and 100% M74 mortality (vertical dashed lines) and for 50% mortality (dotted horizontal and vertical lines) are given. The inset figure presents the distribution of females with 100% offspring mortality according to the THIAM concentration of unfertilized eggs
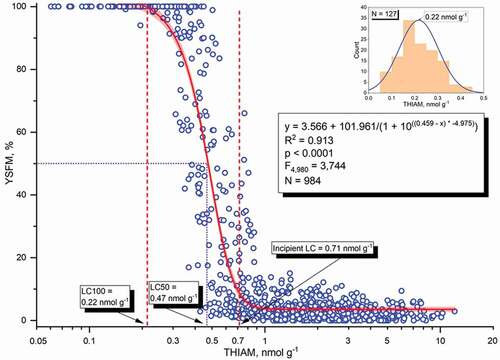
The dose-response-based model between YSFM and the THIAM concentration is:
(YSFM) = 3.566 + 101.961/(1 + 10((0.459 – [THIAM]) * −4.975)),
R2 = 0.913, F4,980 = 3,744, p <0.0001, N = 984.
The similar model for YSFM and TotTH concentration,
(YSFM) = 3.011 + 104.726/(1 + 10((1.077 – [TotTH]) * −2.450)),
R2 = 0.797, F4,980 = 1,462, p <0.0001, N = 984,
had much weaker power than the YSFM vs. THIAM concentration model. From the models, based on the recordings of female-specific YSFM in the experimental incubations and the respective THIAM concentrations in the unfertilized eggs, the threshold THIAM concentrations for incipient M74 mortality and 100% offspring mortality (LC100) can therefore be set at the THIAM concentrations 0.71 (0.66–0.77, 95% confidence limits) and 0.22 (0.19–0.22) nmol g−1 in ovulated unfertilized eggs, respectively; for the threshold THIAM concentration of incipient M74 mortality, 2.5 times the background YSFM was applied; and the LC100 value was set from the distribution of M74 (100%) females according to the THIAM concentration of unfertilized eggs (). The corresponding concentrations for TotTH are 1.60 (1.49–1.68) and 0.63 (0.59–0.64) nmol g−1 respectively. The LC50 value for the THIAM concentration was 0.47 (0.45–0.49) nmol g−1 (), and that for the TotTH concentration was 1.11 (1.07–1.14) nmol g−1. For those females that die of thiamine deficiency before the stripping of eggs, the egg THIAM concentration of 0.2 nmol g−1 may be applied in estimation calculations.
The models for the ratio [THIAM]/[TPP] or the sum [TMP]+[THIAM] and YSFM did not produce a good fit and are not presented.
3.4. Carotenoids
The total carotenoid concentration in unfertilized eggs was weakly related to YSFM ( and ). In the range of approximately 2–5 µg g−1 of total carotenoids, some 100% YSFM was detected, but in the range 2.5–7.0 µg g−1, mostly very low YSFM occurred. The concentrations of total carotenoids and THIAM were related according to an exponential model, but with a poor coefficient of determination (Supplemental material, Figure S3a). The relationship between the concentrations of total carotenoids and astaxanthin was linear (Supplemental material, Figure S3b). However, the concentration of astaxanthin was not related to YSFM or the THIAM concentration ( and ).
Figure 8. Relationship of yolk-sac fry mortality (YSFM, %) with a) the concentration of total carotenoids, b) the concentration of astaxanthin, c) the color of unfertilized eggs, and d) the color of the oil droplet of yolk (yolk-sac fry of age 1–3 days). The number of females is given as N (a and b) or on the top line (c and d)
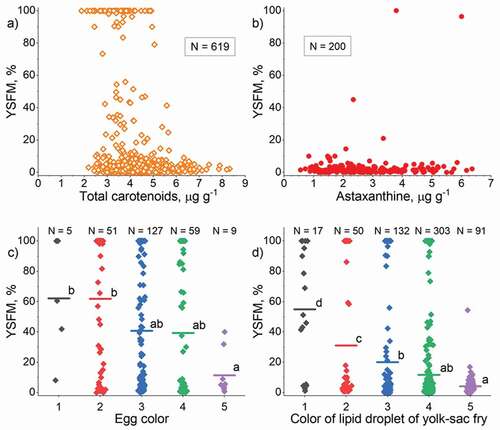
The color of unfertilized eggs or the large oil droplet in the yolk of sac fry were or tended to be paler the higher the mean YSFM and smaller the mean THIAM concentration ( c and d; Supplemental material, Figure S3c and d). However, the variation within the color classes was large meaning that single YSFM or THIAM values of different color classes overlapped.
3.5. Female salmon body characteristics
Most of the returned BB females in 1994–2009, 77.5%, were 2nd sea-year females, and 18.4% were 3rd sea-year females (Supplemental material, Figure S4a). The proportions were nearly the same for M74 and non-M74 females separately (not shown). The annual percentage distribution of the sea-age of BB females in monitoring during 1985–2019 is presented in Supplemental material, Figure S4b.
The CF of M74 females (0.96 ± 0.003, N = 1,117) was significantly larger (F1,1482 = 23.59, p <0.0001) than that of non-M74 females (0.93 ± 0.006, N = 366) for the spawning years 1990–2019. Within years, the CF was significantly larger in M74 females than in non-M74 females in 1992 and 1999, and in most years, the CF of M74 females tended to be larger (Supplemental material, Figure S5a). In all, the CF was highest during the early 1990s, i.e. the years of the highest incidence of M74. In 1994, for the 2nd sea-year females of the Rivers Simojoki and Tornionjoki, there was a significant negative correlation of the CF with egg THIAM concentration, and positive with YSFM ().
Table 3. Spearman correlations of the total body mass, total length, and condition factor (CF) with free thiamine (THIAM) concentration of eggs and YSFM of the Rivers Simojoki and Tornionjoki, second sea-year salmon ascending for spawning in 1994–2019
In 1993, both the total mass and total length were larger (p <0.05) in M74 females than in non-M74 females. In contrast, in 1996 and 2000, the total length, and in 2001, 2006, and 2018, both the total mass and total length of M74 females, were significantly (p <0.05) smaller than those of non-M74 females (length data in Supplemental material, Figure S5b, data for weight not shown).
When the period (1994–2019) for the 2nd sea-year River Simojoki and River Tornionjoki salmon is considered, THIAM concentration was lower in eggs of larger salmon than smaller salmon when M74 incidence was relatively high, as indicated in 1998 and 1999 by Spearman correlations (). In the same years, YSFM correlated positively with the size indices of salmon. In contrast, during years of lower M74 incidence, for example, in 1997, egg THIAM concentration was smaller in smaller salmon, as indicated by the positive correlation ().
The mean total lipid content in the muscle of BB salmon at spawning was 7.2–18.5% during the 1990s, whereas a lower mean lipid content, 3.3–5.9%, was detected in the 2000s and 2010s (Supplemental material, Figure S6). The highest mean lipid content was recorded in 1990 and the lowest in 2004, but lipid content was not measured every year.
4. DISCUSSION
4.1. Thiamine content and thiamine deficiency-related mortality
4.1.1. Free thiamine as the indicator of offspring mortality
THIAM was confirmed to be the best indicator of thiamine deficiency mortality because it correlated best with YSFM, and its concentration of thiamine components varied most. In addition to Atlantic salmon, the relationship of thiamine deficiency mortality to low egg THIAM concentration has also been reported for some other salmonine species (Brown et al. Citation2005; Czesny et al. Citation2009, Citation2012; Futia and Rinchard Citation2019).
The YSFM vs. THIAM model also enables the calculation of the egg THIAM concentrations for different mortality percentages. The LC50 value of THIAM in eggs (0.47 nmol g−1) was between those derived for two fatty Pacific salmon species, chinook (O. tschawytscha) and coho salmon (O. kisutch), 0.6 and 0.4 nmol g−1, respectively (Brown et al. Citation2005). Our results are comparable with these results obtained by the thiamine analysis method launched by Brown et al. (Citation1998). For four salmonine species of Lake Ontario in North America, the LC50 values of THIAM in eggs varied from 0.26 to 1.32 nmol g−1 (Futia and Rinchard Citation2019), but the models were not very clear.
In addition, it has been suggested that sublethal thiamine concentrations decrease survival and reduce the growth of lake trout (Salvelinus namaycush) offspring by decreasing their foraging success and increasing vulnerability to predation (Fitzsimons et al. Citation2009; Ivan et al. Citation2018). However, the sublethal TotTH concentrations presented in these studies, 5.1 and 7.3 nmol g−1, based on reduced growth, seem unconvincing and inappropriate for Atlantic salmon. If they were appropriate, 92–98% of offspring would not have been viable in our experimental M74 data (1994–2009), whereas more than 60% [including even those 127 (13%) females with 100% mortality] actually survived, and they were lively at the alevin phase in the laboratory. Yet a higher than threshold THIAM concentration for incipient M74 mortality may also have the potential to decrease salmon recruitment: At least in a few offspring groups of BB females with an egg THIAM concentration of up to 0.97nmolg−1, the majority of alevins were alive but passive at the end of the yolk-sac phase, and may not have been able to survive under natural conditions. Yet background YSFM of 3.6% is included in our model, because it appears that not all the offspring of non-M74 females survive, for example, due to some congenital malformations.
Although the THIAM/TPP ratio has also been suggested as an indicator of thiamine deficiency mortality (Futia and Rinchard Citation2019), it was less sensitive in our dataset than THIAM. The TPP concentration of the eggs did not differ significantly between M74 and non-M74 females or between the years of moderate (1998) and insignificant (2004) M74 incidence (Keinänen et al. Citation2018). In all, thiamine is principally located in the muscle tissue, the largest tissue in fish. In the muscle of salmon on their spawning run and at spawning, TPP accounted for 80–88% of TotTH (Vuorinen et al. Citation2020). The co-enzyme form TPP in general accounts for the major part of thiamine in vertebrate soft tissues such as the muscle, brain, and liver (Combs and McClung Citation2017). Muscle TPP probably does not easily supply additional thiamine for transportation to developing oocytes because >90% of TPP in the muscle is bound to the enzymes in the mitochondria (Depeint et al. Citation2006). Consequently, the concentration and proportion of THIAM in the muscle of pre-spawning salmon were very low, 0.03–0.13 nmol g−1 and 0.5–1.9%, respectively (Vuorinen et al. Citation2020). During the feeding migration, the smallest THIAM proportions and concentrations of muscle were detected in the fattiest salmon specimens (Keinänen et al. unpubl.). In several landlocked salmonine species, the THIAM concentration and proportion of muscle were similarly low (mean values 0.07–0.20 nmol g−1 and 1.28–3.36%) (Futia and Rinchard Citation2019). In sockeye salmon (O. gorbuscha) that had been feeding in the Pacific Ocean, the reported concentration and proportion of THIAM in the muscle (3.7 nmol g−1 and 30%, respectively,) were much higher than in Baltic salmon, as was the TotTH concentration (12.2 nmol g−1) (Welch et al. Citation2018).
4.1.2. Variation in content of egg thiamine components
Among all BB females, through M74 monitoring, the lowest THIAM concentration of the eggs was 0.060 nmol g−1, and for TotTH, 0.253 nmol g−1, and the highest concentration for THIAM was 13.040 nmol g−1, and for TotTH 14.029 nmol g−1. In lake trout eggs, THIAM concentration also varied by about two orders of magnitude (Czesny et al. Citation2012). As thiamine analyses started in the fall of 1994, the lowest concentrations were probably undetected because the salmon were the fattiest, having the highest CF in the falls of 1990 and 1991 (Mikkonen et al. Citation2011).
In cases where the TotTH concentration in eggs was high, and no M74 mortality was expected among yolk-sac fry, THIAM was the largest component of egg thiamine, whereas when the TotTH concentration of the eggs was low, TPP was the largest. In the mid-1990s, during the high M74 incidence, the annual mean proportion of THIAM was 20–40%, whereas that of TPP was more than half of TotTH. Among five salmonine species from Lake Ontario, in the eggs of lake trout, coho salmon, and steelhead trout (O. mykiss) with high TDC mortality, the proportion of THIAM was similarly lower than that of TPP (Czesny et al. Citation2009; Futia and Rinchard Citation2019). The large BB salmon dataset demonstrated that the proportions of THIAM and TPP had a highly significant inverse linear relationship. The proportions were equal at a TotTH concentration of 1.19 nmol g−1, while the threshold of TotTH concentration for incipient M74 mortality was 1.60 nmol g−1 from the YSFM vs. TotTH model.
The model for the proportions of thiamine components showed that at TotTH concentrations >3.75 nmol g−1, THIAM constituted 80–90% of thiamine. In the eggs of other landlocked salmonines that apparently did not suffer from thiamine deficiency, THIAM was similarly the major thiamine component (Brown et al. Citation1998; Czesny et al. Citation2012; Futia and Rinchard Citation2019). A slightly larger proportion, approximately 90%, of TotTH (6.2–8.9 nmol g−1) consisted of THIAM in the eggs of salmon from the River Tenojoki that had been feeding in the Arctic Ocean than the eggs of non-M74 BB salmon. Compared to River Tenojoki salmon, several Oncorhynchus species that had been feeding in the Pacific Ocean contained thiamine in eggs in even higher concentrations when they returned for spawning (TotTH 10.9–19.5 nmol g−1). In them, THIAM (8.0–18.4 nmol g−1) was by far the largest component (73–94%) of TotTH in the eggs (Welch et al. Citation2018).
The proportion and concentration of monophosphorylated thiamine derivative TMP were lowest among the thiamine components in the eggs, regardless of THIAM or TotTH concentration. A similar observation has been reported for other salmonines (Czesny et al. Citation2009; Welch et al. Citation2018; Futia and Rinchard Citation2019). However, the TMP concentration had a stronger negative relationship with YSFM than the concentration of TPP. Consistently, the mean TMP concentration was higher in River Simojoki salmon eggs in a year (2004) with insignificant incidence of M74 than in a year (1998) with moderate M74 incidence, and higher in the eggs of non-M74 females compared to M74 females in 1998 (Keinänen et al. Citation2018). TMP concentration is linked with the THIAM concentration, probably because TMP is an essential intermediate in thiamine transport between extra- and intracellular compartments (Manzetti et al. Citation2014; Combs and McClung Citation2017).
4.2. Variation in the incidence of M74
4.2.1. Inter-annual variation depends on changes in fish stocks
The annual incidence of M74 varied very similarly in the salmon stocks of the monitored BB rivers and in all the other Gulf of Bothnia rivers (ICES Citation2020a) because the main feeding grounds of all these stocks are located in the Baltic Proper (Aro Citation1989; Kallio-Nyberg et al. Citation2015; Jacobson et al. Citation2020). The substantial variation in the incidence of M74 between years was principally related to the changes in sprat recruitment and thus the abundance of young fatty sprat in the sea, and the size of the sprat stock (Karlsson et al. Citation1999; Mikkonen et al. Citation2011; Keinänen et al. Citation2012, Citation2018). The respective thiamine deficiency TDC in many landlocked salmonine species of the Great Lakes in North America and in the Atlantic salmon of New York’s Finger Lakes is correspondingly linked to increased preying on a fatty fish species, alewife (Alosa pseudoharengus) and its increasing abundance (Fisher et al. Citation1995; Czesny et al. Citation2009; Riley et al. Citation2011; Happel et al. Citation2017; Futia et al. Citation2019). To a small extent, M74 syndrome could also develop from eating herring in the Bothnian Sea after recruitment of a strong herring year class, when there was a large number of young herring, and prey fish were fatty (Keinänen et al. Citation2018; Vuorinen et al. Citation2020).
Sprat has in general been the dominant prey fish of salmon in the Baltic Proper (Karlsson et al. Citation1999; Hansson et al. Citation2001; Vuorinen et al. Citation2014b), where the main spawning grounds of sprat and cod (Gadus morhua), the principal predator of sprat, are located (Aro Citation1989). Herring is the dominant prey fish species of salmon in the Bothnian Sea of the Gulf of Bothnia (Karlsson et al. Citation1999; Hansson et al. Citation2001; Salminen et al. Citation2001; Mikkonen et al. Citation2011). However, some sprat migrate from the Baltic Proper according to their abundance to the southern parts of the Bothnian Sea, where their proportion in the food biomass of salmon has been <10% (Salminen et al. Citation2001; Mikkonen et al. Citation2011).
The high incidence of M74 is not related to a low thiamine concentration in the prey; surprisingly, the greater the supply of thiamine and lipids from sprat in the southern Baltic Proper, the lower the concentration of thiamine in salmon eggs (Keinänen et al. Citation2012). The amount of lipids and PUFAs provided by the diet therefore determines the extent to which thiamine is decreased in an individual salmon (Vuorinen et al. Citation2020). As M74 is related to high dietary energy density and lipid content, it is also linked to a low total thiamine concentration per unit of energy, lipid, and PUFAs in the total prey biomass of salmon (Keinänen et al. Citation2012, Citation2017).
The average lipid content of sprat was double that of herring (Vuorinen et al. Citation2002; Keinänen et al. Citation2012, Citation2017). Moreover, the youngest specimens of sprat were the fattiest among the age groups, but this relationship was not clear for herring (Vuorinen et al. Citation2002; Keinänen et al. Citation2012, Citation2017). However, the concentration of n-3 PUFAs, and even more clearly the concentration of DHA, was largest in the youngest specimens, decreasing with age in both species (Keinänen et al. Citation2017).
Although the concentration of thiamine did not in general differ between sprat and herring (Keinänen et al. Citation2012), in the fall, thiamine concentration was lower in fattier sprat than in herring (Vuorinen et al. Citation2002). Probably due to lipid peroxidation reactions, against which thiamine acts as a site-specific antioxidant (Lukienko et al. Citation2000; Gibson and Zhang Citation2002; Depeint et al. Citation2006), the concentration of thiamine already decreased in relation to DHA and PUFA content in prey fish (Keinänen et al. Citation2017) and in the liver of salmon during the feeding migration in the sea (Keinänen et al., unpubl.). However, thiamine declined in salmon liver and ovaries especially during pre-spawning fasting, and this depletion was connected with the lipid peroxidation of PUFAs accumulated in the body’s lipid stores during the feeding migration (Vuorinen et al. Citation2020). Lipid peroxidation manifested itself in salmon at spawning as an increase in the hepatic concentration of malondialdehyde (MDA) (Vuorinen et al. Citation2020), which is the major lipid peroxidation product (Moore and Roberts Citation1998; Del Rio et al. Citation2005).
When acting as an antioxidant in lipid peroxidation, thiamine is converted to thiochrome and other metabolites that can no longer function as a co-enzyme and are excreted (Gibson and Zhang Citation2002; Combs and McClung Citation2017). As the decline in thiamine enhances oxidative stress (Gibson and Zhang Citation2002; Chauhan et al. Citation2018), all this at worst leads to a vicious spiral of peroxidation-oxidative stress-depletion of antioxidants, which also became apparent as reduced carotenoid concentrations. Diminished thiamine body stores of M74 females did not allow adequate deposition of thiamine into developing oocytes, which meant the development of offspring could not be sustained until fry started external feeding.
The M74 peak during the first years of the 1990s coincided with an exponential increase in the size of the sprat stock after the collapse of the cod stock (Mikkonen et al. Citation2011). After the mid-1990s, when cod had become more abundant, the spawning stock biomass of sprat decreased (Mikkonen et al. Citation2011; Keinänen et al. Citation2014; ICES Citation2020b). This became evident as a temporal decrease in the incidence of M74 in BB salmon in 1997/1998. The next and even more favorable period in relation to M74 occurred in 2002/2003–2004/2005, after the size of the sprat stock had decreased to its lowest since the early 1990s (Mikkonen et al. Citation2011; Keinänen et al. Citation2014). The cod stock further recovered to some degree toward the 2010s (ICES Citation2020b), which was reflected in the incidence of M74: No M74 mortalities were observed during 2011/2012 – 2014/2015. However, for 2015/2016–2018/2019, the M74 syndrome deteriorated again as a consequence of the exceptionally strong sprat 2014 year class, when the eastern cod and its stock were in a poor state (Neuenfeldt et al. Citation2019; ICES Citation2020b).
4.2.2. Variation in relation to salmon condition factor and size
The effect of salmon size on M74 incidence is determined by size-dependent prey availability (Jacobson et al. Citation2018; Jacobson Citation2020). Even large salmon prefer smallish prey fish (Hansson et al. Citation2001), yet they can eat larger prey than small salmon (Jacobson et al. Citation2018). In the southern Baltic Sea, herring grow so fast that only the very youngest age groups are of a suitable size to be preyed on by salmon (Mikkonen et al. Citation2011). In contrast, all age groups of sprat are suitable because sprat do not grow to more than about 15 cm in length (Vuorinen et al. Citation2002). In the Baltic Proper, where the proportion of sprat in the prey biomass of salmon has in general been larger than that of herring (Mikkonen et al. Citation2011; Vuorinen et al. Citation2014b; Jacobson et al. Citation2018), smallish salmon apparently prey principally on sprat even if the proportion of herring in the salmon prey biomass is prominent. Because the smallest salmon, with a small mouth gap, can only prey on the smallest prey specimens, they inevitably prey more on sprat than herring, and more on smaller than larger sprat (Jacobson et al. Citation2018; Jacobson Citation2020).
As a consequence of the heavy recruitment of sprat (e.g. 1992–1995, 2014), young sprat have been so abundant in the Baltic Proper that both small and large salmon have preyed mainly on them, large salmon even more than small salmon, to meet their energy needs. An abundant fatty diet manifested itself as a high CF – and a reduced thiamine status. Accordingly, the mean CF (range 0.84–1.03) of 2nd sea-year BB females in the monitoring data analyzed up to 2006 was highest in 1990 and 1991 (Mikkonen et al. Citation2011), i.e. when the lipid content in the muscle was also highest, and the incidence of M74 erupted. Although the biomass of herring in the Baltic Proper influenced the growth rate of salmon along with the impact of sprat biomass, the increase in the CF of salmon was only related to the increase in sprat biomass (Mikkonen et al. Citation2011).
As the lipid and PUFA content of the youngest sprat is largest among the prey specimens of salmon (Vuorinen et al. Citation2002; Keinänen et al. Citation2017), large salmon are more susceptible to lipid peroxidation after such years. This was extremely augmented in the early years of the 1990s, when the sprat biomass exponentially increased because of the high recruitment rate of sprat in several years when the size of the cod stock was small (Mikkonen et al. Citation2011). In the salmon diet in the mid-1990s, the smallest sprat (age groups 0 and 1) were more numerous than in the early 1960s (Hansson et al. Citation2001). Therefore, after such years (e.g., in 1998/1999 and 1999/2000), larger salmon have suffered more from M74 than small salmon. Similarly, a low egg THIAM concentration resulting in high TDC mortality was related to a large body mass of Atlantic salmon feeding in Lake Michigan in North America (Werner et al. Citation2006).
In contrast, in years of low M74 incidence (e.g. in 1997/1998 and 2004/2005), small salmon had lower average egg THIAM concentrations because they relied more than large salmon on small sprat in their diet. This also explains the observation of Backman (Citation2004) that among wild ascending salmon, in those specimens that were larger and ascended first, there were fewer M74 females than among those that ascended later and were smaller.
Yet, over the years, females with a low egg THIAM concentration and high YSFM had higher average CF, which indicated that they had consumed food with a high calorie content, i.e. fatty fish, which were young sprat in the Baltic Proper. This is in line with the finding that size-specific prey availability rather than a high total prey biomass explains the high CF of salmon (Jacobson et al. Citation2018).
4.2.3. Intra-annual differences between and within rivers are related to migration routes and diet
In the most severe M74 years at the beginning of the 1990s, intra-annual variation in THIAM concentrations between rivers and salmon individuals within a river was low. In years with an intermediate or low M74 incidence, a large variation, with annual differences of even more than 100-fold in the egg THIAM concentration between females of the same stock, is partly explained by the differences in feeding migration routes and feeding areas, and – due to size-dependent prey availability – salmon size, all of which affect the diet (Jacobson et al. Citation2018, p. 2020).
Egg THIAM concentrations were also analyzed for salmon of some other Gulf of Bothnia rivers in addition to BB salmon in 2015, 2016, and 2018 (unpubl. data). In 2016 and 2018, there were no significant differences in the THIAM concentration between salmon from the Rivers Dal, Iijoki, Kemijoki, Simojoki, and Tornionjoki, or the River Ume, which is located in a north–south direction between the River Dal and the BB rivers. Only in 2015, when the THIAM concentrations monitored for River Simojoki salmon suddenly decreased after four good M74 years, the mean egg THIAM concentration was still significantly larger for River Simojoki salmon (3.150 nmol g−1, N = 28) than for the salmon of the Rivers Ume (1.529 nmol g−1, N = 38) and Dal (1.361 nmol g−1, N = 33). These differences in THIAM concentrations between salmon stocks indicate some differences in their feeding migration. For example, salmon from the River Dal, unlike salmon from the more northern rivers, on average migrate first northwards in the Gulf of Bothnia before heading to the southern Baltic Sea (Jacobson et al. Citation2020).
Apart from varying between years, amounts and proportions of sprat and herring vary between the different parts of the Baltic Proper (Mikkonen et al. Citation2011; Keinänen et al. Citation2012; Jacobson et al. Citation2018), which results in differences in lipid content and energy density in the salmon prey biomass between the subareas (Keinänen et al. Citation2012). In general, salmon individuals from the stocks of the more northern rivers appeared on average to migrate sooner and more frequently to the Baltic Proper than salmon of the more southern rivers (Jacobson et al. Citation2020), which may also explain why very high M74 mortality was observed a year earlier in River Tornionjoki than in River Simojoki salmon.
4.2.4. Some M74 originate in the Bothnian Sea
In 2004/2005, the only M74 female with 100% offspring mortality in M74 monitoring had mainly fed on herring in the Bothnian Sea (Keinänen et al. Citation2018; Vuorinen et al. Citation2020). This was confirmed by fatty acid signature analysis (Keinänen et al. Citation2018). During the feeding migration of salmon in 2002–2004, young herring had been abundant in the Bothnian Sea (Mikkonen et al. Citation2011; Keinänen et al. Citation2014; ICES Citation2020b), because the 2002 herring year class was very strong there (ICES Citation2020b). During the late summer, herring aged 0 are already appropriate prey for salmon (Salminen Citation2002). In 2003–2004, the average lipid content of salmon prey fish was higher in the Bothnian Sea than in the Baltic Proper, and lowest in the Gulf of Finland (Vuorinen et al. Citation2012; Keinänen et al. Citation2017). Although sprat is on average fattier than herring, the proportion of PUFAs was or tended to be higher on average in herring than in sprat (Røjbek et al. Citation2014; Keinänen et al. Citation2017), apparently as a species-specific feature. The concentration of DHA and total PUFAs in the youngest herring, as in sprat, were therefore highest among the different age groups (Keinänen et al. Citation2017). Consequently, the proportion of DHA and total PUFAs were also higher in Bothnian Sea salmon than in salmon caught in the Baltic Proper in 2004 (Keinänen et al. Citation2018). Feeding abundantly on young fatty herring containing DHA and PUFAs in high concentrations in the Bothnian Sea therefore also resulted in M74.
As only a minority of wild salmon from BB rivers feed in the Bothnian Sea (Kallio-Nyberg et al. Citation2011), the proportion of M74 cases originating there is not essential on a large scale. In the Bothnian Sea, the growth rate of salmon has generally been slower, and they have been smaller than in the southern Baltic Sea (Salminen et al. Citation1994; Keinänen et al. Citation2018). In addition to 2004/2005, in 1997/1998, 2006/2007 and 2015/2016, smaller 2nd sea-year salmon had lower egg THIAM concentrations than larger specimens in the combined data of the Simojoki and Tornionjoki rivers. Without fatty acid signature analysis, it is impossible to know where the M74 females of those years had been feeding.
The somewhat higher incidence of M74 in salmon that ascended the River Kemijoki compared to salmon from the Rivers Simojoki and Tornionjoki may have partly resulted from feeding in the Bothnian Sea. Kemijoki salmon that were included in monitoring during 2005/2006–2009/2010 originate solely from introductions, and a somewhat larger proportion of reared smolts remains to feed in the Bothnian Sea than of wild smolts (Kallio-Nyberg et al. Citation2015). In years with high numbers of young herring in the Bothnian Sea, the M74 incidence in River Kemijoki salmon and other salmon stocks maintained by introductions may therefore be somewhat stronger than in other BB salmon.
4.2.5. M74 weaker in Gulf of Finland salmon
M74 appeared to be somewhat milder in the River Kymijoki salmon than in BB salmon. The majority of River Kymijoki salmon of River Neva origin feed in the Gulf of Finland, and only a small number of them in the Baltic Proper (Aro Citation1989; Keinänen et al. Citation2000b). Although herring was previously the dominant prey fish of salmon in the Gulf of Finland, after exponential recruitment of sprat at the beginning of the 1990s, it also became abundant in the Gulf of Finland (Keinänen et al. Citation2000b). As a result of increased competition for food between sprat specimens, and between sprat and small herring (Casini et al. Citation2006), salmon prey fish were leaner in areas where sprat was abundant (Mikkonen et al. Citation2011; Vuorinen et al. Citation2012). In the Gulf of Finland, the CF and lipid content, and consequently the PUFA concentration, of salmon prey fish was smaller than in the Baltic Proper and the Bothnian Sea (Keinänen et al. Citation2017). The leanness of prey fish became apparent in Gulf of Finland salmon as a low lipid content compared to salmon from the other Baltic Sea basins in 2004 (Vuorinen et al. Citation2012). Thus, milder M74 in some years in River Kymijoki salmon than in BB salmon was probably related to their lower dietary and body lipid and PUFA content. The River Kymijoki salmon also has a short spawning run, and specimens for M74 monitoring were caught in the late fall. River Kymijoki salmon may therefore have been feeding closer to spawning than BB salmon, while the body’s thiamine stores reduce most during the pre-spawning fast (Vuorinen et al. Citation2020).
However, the proportion of M74 females and YSFM could also be larger among the River Kymijoki salmon than among salmon from the BB rivers. This occurred in 1997/1998, when the M74 incidence in BB salmon temporarily decreased as a consequence of a decrease in sprat recruitment and in the size of the sprat stock in the Baltic Proper (Mikkonen et al. Citation2011; Keinänen et al. Citation2014).
4.2.6. Diet in the Arctic Ocean results in good thiamine status
The THIAM concentration in the eggs of salmon from the Arctic River Tenojoki was higher compared to BB salmon, which was expected. Nor did salmon that had been feeding in the Atlantic and had ascended a river on the western coast of southern Sweden display M74 mortality in the heavy M74 years of the 1990s (Pickova et al. Citation1998, Citation2003). The diet of salmon in the Atlantic is much more diverse, consisting of many fish species and abundant crustaceans (Jacobsen and Hansen Citation2001), whose lipid and PUFA content is smaller than in sprat and herring (Hyvönen and Koivistoinen Citation1994). Even Baltic salmon that have fed in the Gulf of Riga do not suffer from M74 (Karlsson et al. Citation1999). Sprat did not occur there in the salmon diet, whereas the alternative less fatty prey fish species constituted approximately 40% of their diet in addition to herring and three-spined stickleback (Gasterosteus aculeatus) (Karlsson et al. Citation1999; Hansson et al. Citation2001; Keinänen et al. Citation2012).
4.3. Decrease in carotenoids indicate oxidative stress
Although the average carotenoid concentration was lower in the eggs of M74 females than in the eggs of non-M74 females, the carotenoid concentration, unlike the THIAM and TotTH concentrations, had no clear relationship with YSFM. Nor did the carotenoid concentration decrease significantly in the tissues of salmon during the spawning migration (Vuorinen et al. Citation2020). Large-scale peroxidation of PUFAs in spawning salmon was revealed by the doubling of the hepatic MDA concentration (Vuorinen et al. Citation2020), and the accumulation of MDA in salmon yolk-sac fry has been linked to M74 mortality (Lundström et al. Citation1999b). As thiamine deficiency as such also increases oxidative stress (Gibson and Zhang Citation2002; Chauhan et al. Citation2018), carotenoids, which are powerful general antioxidants and much more efficient singlet oxygen quenchers than α-tocopherol (Shimidzu et al. Citation1996), reduced (Lundström et al. Citation1999b; Vuorinen et al. Citation2020). Apparently therefore, whereas thiamine functions as a site-specific antioxidant preventing microsomal lipid peroxidation, as well as oleic acid oxidation (Gibson and Zhang Citation2002; Depeint et al. Citation2006), the carotenoid concentration of the eggs had a poorer relationship with YSFM than the concentrations of THIAM and TotTH.
Astaxanthin was the major carotenoid in salmon eggs, as found in other studies (Lundström et al. Citation1999b; Pickova et al. Citation2003), but its concentration was unrelated to YSFM or THIAM concentration in eggs. Consistently, the astaxanthin concentration in the unfertilized eggs of Lake Ontario lake trout did not differ between thiamine deficient and non-thiamine deficient females, although the concentration of total carotenoids did (Palace et al. Citation1998). Although the orange color of unfertilized eggs, and especially of the large oil droplet of the yolk, had on average a relationship with the THIAM concentration and YSFM (Keinänen et al. Citation2014), these cannot be predicted individually from color, because the values of the groups overlap.
5. CONCLUSIONS AND RECOMMENDATIONS
The percentage of thiamine deficiency-related female-specific offspring mortality of Atlantic salmon can be estimated cost-effectively, without laborious egg and yolk-sac fry incubation, by means of the THIAM vs. YSFM model compiled in the present investigation. The model enables the estimation of female-specific YSFM of salmon from the THIAM concentration of unfertilized eggs. The model is based on the large and thorough Finnish M74 monitoring dataset on ascended salmon females, biochemical analyses of indicators in unfertilized eggs, and exact observations of symptoms and mortality among their offspring in experimental incubations in the laboratory over 16 years. The threshold concentration of THIAM for the occurrence of offspring deaths due to thiamine deficiency is 0.71 nmol g−1, and that of TotTH is 1.60 nmol g−1. The respective threshold concentrations for 100% offspring mortality are 0.22 and 0.63 nmol g−1. This mortality includes the basic natural mortality. Analyzing egg carotenoids provides no additional benefit to the estimation.
In the Baltic Sea, M74 should ideally be monitored for all rivers sustaining natural salmon reproduction because of slight differences between the rivers. However, as the incidence of M74 on a large scale varies in a similar way, at least in the different Gulf of Bothnia rivers, to decrease expenses, thiamine deficiency could be monitored for a river sustaining natural reproduction. It is recommended that the number of salmon monitored annually be at least 40 females per river, and preferably 60, due to the large intra-annual variation in egg THIAM concentrations. On a case-by-case basis, M74 would be monitored for those additional rivers, along with the requirements of aquaculture to catch salmon for the purposes of stock maintenance. In this case, monitoring results from the different Gulf of Bothnian rivers could be pooled to strengthen reliability. Because reared salmon smolts remain more often than wild salmon to feed in the Bothnian Sea, M74 monitoring should not be based only on salmon from such rivers as the River Kemijoki. It is recommended that salmon for monitoring be caught by a trap net in the river mouth because those caught by rod, especially from the river, do not necessarily survive. Catching always causes a degree of stress even if the salmon are not injured.
Supplemental Material
Download PDF (904.6 KB)Acknowledgments
We wish to thank everyone who has in some way participated in M74 monitoring and helped obtain the results of this study. The English was edited by Rupert Moreton.
Disclosure Statement
No potential conflict of interest was reported by the author(s).
Supplementary material
Supplemental data for this article can be accessed here.
References
- Alvarez MJ, Lopez-Bote CJ, Diez A, Corraze G, Arzel J, Dias J, Kaushik SJ, Bautista JM. 1998. Dietary fish oil and digestible protein modify susceptibility to lipid peroxidation in the muscle of rainbow trout (Oncorhynchus mykiss) and sea bass (Dicentrarchus labrax). Br J Nutr. 80(3):281–289. doi:https://doi.org/10.1017/S0007114598001330.
- Amcoff P, Börjeson H, Landergren P, Vallin L, Norrgren L. 1999. Thiamine (vitamin B1) concentrations in salmon (Salmo salar), brown trout (Salmo trutta) and cod (Gadus morhua) from the Baltic sea. Ambio. 28:48–54.
- Aro E. 1989. A review of fish migration patterns in the Baltic. Rapp P -v Réun Cons Int Explor Mer. 190:72–96.
- Backman J 2004. Itämeren hydrologisten vaihteluiden sekä biologisten tekijöiden yhteys lohen M74-oireyhtymään Helsingin yliopisto, Bio- ja ympäristötieteiden laitos, Akvaattiset tieteet/hydrobiologia, Toukokuu 2004. (Master thesis in Finnish).
- Balon EK. 1975. Terminology of intervals in fish development. J Fish Res Board Can. 32(9):1663–1670. doi:https://doi.org/10.1139/f75-196.
- Brown SB, Honeyfield DC, Vandenbyllaardt L 1998. Thiamine analysis in fish tissues. In: McDonald G, Fitzsimons JD, Honeyfield DC, editors. Early life stage mortality syndrome in fishes of the Great Lakes and the Baltic Sea. American Fisheries Society, Symposium 21, Bethesda, Maryland. p. 73–81.
- Brown SB, Arts MT, Brown LR, Brown M, Moore K, Villella M, Fitzsimons JD, Honeyfield DC, Tillitt DE, Zajicek JL. 2005. Can diet-dependent factors help explain fish-to-fish variation in thiamine-dependent early mortality syndrome?. J Aquat Anim Health. 17(1):36–47. doi:https://doi.org/10.1577/H03-072.1.
- Bylund G, Lerche O. 1995. Thiamine therapy of M74 affected fry of Atlantic salmon Salmo salar. Bull Eur Ass Fish Pathol. 15:93–97.
- Casini M, Cardinale M, Hjelm J. 2006. Inter-annual variation in herring, Clupea harengus, and sprat, Sprattus sprattus, condition in the central Baltic Sea: what gives the tune?. Oikos. 112(3):638–650. doi:https://doi.org/10.1111/j.0030-1299.2006.13860.x.
- Casteels M, Sniekers M, Fraccascia P, Mannaerts GP, VanVeldhoven PP. 2007. The role of 2-hydroxyacyl-CoA lyase, a thiamin pyrophosphate-dependent enzyme, in the peroxisomal metabolism of 3-methyl-branched fatty acids and 2-hydroxy straight-chain fatty acids. Biochem Soc Trans. 35(5):876–880. doi:https://doi.org/10.1042/BST0350876.
- Chauhan A, Srivastva N, Bubber P. 2018. Thiamine deficiency induced dietary disparity promotes oxidative stress and neurodegeneration. Indian J Clin Biochem. 33(4):422–428. doi:https://doi.org/10.1007/s12291-017-0690-1.
- Combs GF Jr., McClung JP. 2017. Thiamin. In: Combs GF Jr., McClung JP, editors. The vitamins, Fundamental aspects in nutrition and health. 5th ed. London (San Diego,Cambridge, Oxford): Academic Press; p. 297–314.
- Czesny S, Dettmers JM, Rinchard J, Dabrowski K. 2009. Linking egg thiamine and fatty acid concentrations of Lake Michigan lake trout with early life stage mortality. J Aquat Anim Health. 21(4):262–271. doi:https://doi.org/10.1577/H07-056.1.
- Czesny SJ, Rinchard J, Lee BJ, Dabrowski K, Dettmers JM, Cao Y. 2012. Does spatial variation in egg thiamine and fatty-acid concentration of Lake Michigan lake trout Salvelinus namaycush lead to differential early mortality syndrome and yolk oedema mortality in offspring?. J Fish Biol. 80(7):2475–2493. doi:https://doi.org/10.1111/j.1095-8649.2012.03304.x.
- Del Rio D, Stewart AJ, Pellegrini N. 2005. A review of recent studies on malondialdehyde as toxic molecule and biological marker of oxidative stress. Nutr Metab Cardiovasc Dis. 15(4):316–328. doi:https://doi.org/10.1016/j.numecd.2005.05.003.
- Depeint F, Bruce WR, Shangari N, Mehta R, O’Brien PJ. 2006. Mitochondrial function and toxicity: role of the B vitamin family on mitochondrial energy metabolism. Chem Biol Interact. 163(1–2):94–112. doi:https://doi.org/10.1016/j.cbi.2006.04.014.
- Fisher JP, Spitsbergen JM, Iamonte T, Little EE, DeLonay A. 1995. Pathological and behavioral manifestations of the “Cayuga syndrome”, a thiamine deficiency in larval landlocked Atlantic salmon. J Aquat Anim Health. 7(4):269–283. doi:https://doi.org/10.1577/1548-8667(1995)007<0269:PABMOT>2.3.CO;2.
- Fitzsimons JD, Brown SB, Williston B, Williston G, Brown LR, Moore K, Honeyfield DC, Tillitt DE. 2009. Influence of thiamine deficiency on lake trout larval growth, foraging, and predator avoidance. J Aquat Anim Health. 21(4):302–314. doi:https://doi.org/10.1577/H08-019.1.
- Futia MH, Connerton MJ, Weidel BC, Rinchard J. 2019. Diet predictions of Lake Ontario salmonines based on fatty acids and correlations between their fat content and thiamine concentrations. J Great Lakes Res. 45(5):934–948. doi:https://doi.org/10.1016/j.jglr.2019.08.005.
- Futia MH, Rinchard J. 2019. Evaluation of adult and offspring thiamine deficiency in salmonine species from Lake Ontario. J Great Lakes Res. 45(4):811–820. doi:https://doi.org/10.1016/j.jglr.2019.05.010.
- Gibson GE, Zhang H. 2002. Interactions of oxidative stress with thiamine homeostasis promote neurodegeneration. Neurochem Int. 40(6):493–504. doi:https://doi.org/10.1016/S0197-0186(01)00120-6.
- Hansson S, Karlsson L, Ikonen E, Christensen O, Mitans A, Uzars D, Petersson E, Ragnarsson B. 2001. Stomach analyses of Baltic salmon from 1959–1962 and 1994–1997: possible relations between diet and yolk-sac-fry mortality (M74). J Fish Biol. 58:1730–1745.
- Happel A, Maier C, Farese N, Czesny S, Rinchard J. 2019. Fatty acids differentiate consumers despite variation within prey fatty acid profiles. Freshw Biol. 64(8):1416–1426. doi:https://doi.org/10.1111/fwb.13315.
- Happel A, Pattridge R, Walsh M, Rinchard J. 2017. Assessing diet compositions of Lake Ontario predators using fatty acid profiles of prey fishes. J Great Lakes Res. 43(5):838–845. doi:https://doi.org/10.1016/j.jglr.2016.12.008.
- Hyvönen L, Koivistoinen P. 1994. Fatty acid analysis, TAG equivalents as net fat value, and nutritional attributes of fish and fish products. J Food Compos Anal. 7(1–2):44–58. doi:https://doi.org/10.1006/jfca.1994.1005.
- ICES. 2020a. Baltic salmon and trout assessment working group (WGBAST). ICES Sci Rep. 2(22):261.
- ICES. 2020b. Baltic fisheries assessment working group (WGBFAS). ICES Sci Rep. 2(45):643.
- ISO. 2004. Cheese and processed cheese products - determination of fat content - gravimetric method (Reference method). ISO 1735:2004/IDF 5:2004.
- Ivan LN, Schmitt BR, Rose KA, Riley SC, Rose JB, Murphy CA. 2018. Evaluation of the thiamine dose-response relationship for lake trout (Salvelinus namaycush) fry using an individual based model. J Great Lakes Res. 44(6):1393–1404. doi:https://doi.org/10.1016/j.jglr.2018.08.013.
- Jacobsen JA, Hansen LP. 2001. Feeding habits of wild and escaped farmed Atlantic salmon, Salmo salar L., in the Northeast Atlantic. ICES J Mar Sci. 58(4):916–933. doi:https://doi.org/10.1006/jmsc.2001.1084.
- Jacobson P 2020. Size-dependent predator-prey interactions, distribution and mortality in salmon: effects on individuals and populations. Öregrund: Swedish University of Agricultural Sciences, SLU. (Doctoral thesis).
- Jacobson P, Gådmark A, Huss M. 2020. Population and size-specific distribution of Atlantic salmon Salmo salar in the Baltic Sea over five decades. J Fish Biol. 96(2):408–417. doi:https://doi.org/10.1111/jfb.14213.
- Jacobson P, Gårdmark A, Östergren J, Casini M, Huss M. 2018. Size-dependent prey availability affects diet and performance of predatory fish at sea: a case study of Atlantic salmon. Ecosphere. 9(1):1–13. doi:https://doi.org/10.1002/ecs2.2081.
- Kallio-Nyberg I, Romakkaniemi A, Jokikokko E, Saloniemi I, Jutila E. 2015. Differences between wild and reared Salmo salar stocks of two northern Baltic Sea rivers. Fish Res. 165:85–95. doi:https://doi.org/10.1016/j.fishres.2014.12.022.
- Kallio-Nyberg I, Saloniemi I, Jutila E, Jokikokko E. 2011. Effect of hatchery rearing and environmental factors on the survival, growth and migration of Atlantic salmon in the Baltic Sea. Fish Res. 109(2–3):285–294. doi:https://doi.org/10.1016/j.fishres.2011.02.015.
- Karlsson L, Ikonen E, Mitans A, Hansson S. 1999. The diet of salmon (Salmo salar) in the Baltic sea and connections with the M74 syndrome. Ambio. 28:37–42.
- Karlsson L, Karlström Ö. 1994. The Baltic salmon (Salmo salar L.): its history, present situation and future. Dana. 10:61–85.
- Karlström Ö. 1999. Development of the M74 syndrome in wild populations of Baltic salmon (Salmo salar) in Swedish rivers. Ambio. 28:82–86.
- Keinänen M, Iivari J, Juntunen E-P, Kannel R, Heinimaa P, Nikonen S, Pakarinen T, Romakkaniemi A, Vuorinen PJ 2014. Thiamine deficiency M74 of salmon can be prevented. Riista- ja kalatalous–Selvityksiä. 14/2014. (In Finnish with abstract in English).
- Keinänen M, Käkelä R, Ritvanen T, Myllylä T, Pönni J, Vuorinen PJ. 2017. Fatty acid composition of sprat (Sprattus sprattus) and herring (Clupea harengus) in the Baltic Sea as potential prey for salmon (Salmo salar). Helgol Mar Res. 71(1):1–16. doi:https://doi.org/10.1186/s10152-017-0484-0.
- Keinänen M, Käkelä R, Ritvanen T, Pönni J, Harjunpää H, Myllylä T, Vuorinen PJ. 2018. Fatty acid signatures connect thiamine deficiency with the diet of the Atlantic salmon (Salmo salar) feeding in the Baltic Sea. Mar Biol. 165(10):161. doi:https://doi.org/10.1007/s00227-018-3418-8.
- Keinänen M, Peuranen S, Nikinmaa M, Tigerstedt C, Vuorinen PJ. 2000a. Comparison of the responses of the yolk-sac fry of pike (Esox lucius) and roach (Rutilus rutilus) to low pH and aluminium: sodium influx, development and activity. Aquat Toxicol. 47(3–4):161–179. doi:https://doi.org/10.1016/S0166-445X(99)00021-1.
- Keinänen M, Tolonen T, Ikonen E, Parmanne R, Tigerstedt C, Rytilahti J, Soivio A, Vuorinen PJ 2000b. Reproduction disorder of Baltic salmon M74. Riista- ja kalatalouden tutkimuslaitos, Kalatutkimuksia–Fiskundersökningar. 165. (In Finnish with abstract in English).
- Keinänen M, Uddström A, Mikkonen J, Casini M, Pönni J, Myllylä T, Aro E, Vuorinen PJ. 2012. The thiamine deficiency syndrome M74, a reproductive disorder of Atlantic salmon (Salmo salar) feeding in the Baltic Sea, is related to the fat and thiamine content of prey fish. ICES J Mar Sci. 69(4):516–528. doi:https://doi.org/10.1093/icesjms/fss041.
- Keinänen M, Uddström A, Mikkonen J, Rytilahti J, Juntunen E-P, Nikonen S, Vuorinen PJ 2008. The M74 syndrome of Baltic salmon: the monitoring results from Finnish rivers up until 2007. Riista- ja kalatalous–Selvityksiä. 4/2008. (In Finnish with abstract in English).
- Kjær M, Todorcevic M, Torstensen B, Vegusdal A, Ruyter B. 2008. Dietary n-3 HUFA affects mitochondrial fatty acid β-oxidation capacity and susceptibility to oxidative stress in Atlantic salmon. Lipids. 43(9):813–827. doi:https://doi.org/10.1007/s11745-008-3208-z.
- Koski P. 2002. Parental background predisposes Baltic salmon fry to M74 syndrome. Acta Vet Scand. 43(2):127–130. doi:https://doi.org/10.1186/1751-0147-43-127.
- Koski P, Pakarinen M, Nakari T, Soivio A, Hartikainen K. 1999. Treatment with thiamine hydrochloride and astaxanthine for the prevention of yolk-sac mortality in Baltic salmon fry (M74 syndrome). Dis Aquat Org. 37:209–220. doi:https://doi.org/10.3354/dao037209.
- Landergren P, Vallin L, Westin L, Amcoff P, Börjeson H, Ragnarsson B. 1999. Reproductive failure in Baltic sea trout (Salmo trutta) compared with the M74 syndrome in Baltic salmon (Salmo salar). Ambio. 28:87–91.
- Lind Y, Huovila T, Käkelä R. 2018. A retrospective study of fatty acid composition in Baltic herring (Clupea harengus membras) caught at three locations in the Baltic Sea (1973–2009). ICES J Mar Sci. 75(1):330–339. doi:https://doi.org/10.1093/icesjms/fsx127.
- Lonsdale D, Marrs C. 2019. Thiamine deficiency disease, dysautonomia, and high calorie malnutrition London. San Diego: Academic Press.
- Lukienko PI, Mel’nichenko NG, Zverinskii IV, Zabrodskaya SV. 2000. Antioxidant properties of thiamine. B Exp Biol Med+. 130(3):874–876. doi:https://doi.org/10.1007/BF02682257.
- Lundström J, Börjeson H, Norrgren L. 1999a. Histopathological studies of yolk-sac fry of Baltic salmon (Salmo salar) with the M74 syndrome. Ambio. 28:16–23.
- Lundström J, Carney B, Amcoff P, Pettersson A, Börjeson H, Förlin L, Norrgren L. 1999b. Antioxidative systems, detoxifying enzymes and thiamine levels in Baltic salmon (Salmo salar) that develop M74. Ambio. 28:24–29.
- Manzetti S, Zhang J, van der Spoel D. 2014. Thiamin function, metabolism, uptake, and transport. Biochemistry. 53(5):821–835. doi:https://doi.org/10.1021/bi401618y.
- Miki W. 1991. Biological functions and activities of animal carotenoids. Pure Appl Chem. 63(1):141–146. doi:https://doi.org/10.1351/pac199163010141.
- Mikkonen J, Keinänen M, Casini M, Pönni J, Vuorinen PJ. 2011. Relationships between fish stock changes in the Baltic Sea and the M74 syndrome, a reproductive disorder of Atlantic salmon (Salmo salar). ICES J Mar Sci. 68(10):2134–2144. doi:https://doi.org/10.1093/icesjms/fsr156.
- Moore K, Roberts LJ. 1998. Measurement of lipid peroxidation. Free Radic Res. 28(6):659–671. doi:https://doi.org/10.3109/10715769809065821.
- Neuenfeldt S, Bartolino V, Orio A, Andersen KH, Andersen NG, Niiranen S, Bergström U, Ustups D, Kulatska N, Casini M. 2019. Feeding and growth of Atlantic cod (Gadus morhua L.) in the eastern Baltic Sea under environmental change. ICES J Mar Sci. 77(2):624–632. doi:https://doi.org/10.1093/icesjms/fsz224.
- Palace VP, Brown SB, Baron CL, Fitzsimons J, Woodin B, Stegeman JJ, Klaverkamp JF. 1998. An evaluation of the relationships among oxidative stress, antioxidant vitamins and early mortality syndrome (EMS) of lake trout (Salvelinus namaycush) from Lake Ontario. Aquat Toxicol. 43(2–3):195–208. doi:https://doi.org/10.1016/S0166-445X(97)00107-0.
- Pettersson A, Lignell Å. 1999. Astaxanthin deficiency in eggs and fry of Baltic salmon (Salmo salar) with the M74 syndrome. Ambio. 28:43–47.
- Pickova J, Dutta PC, Pettersson A, Froyland L, Kiessling A. 2003. Eggs of Baltic salmon displaying M74, yolk sac mortality syndrome have elevated levels of cholesterol oxides and the fatty acid 22:6 n-3. Aquaculture. 227(1–4):63–75. doi:https://doi.org/10.1016/S0044-8486(03)00495-2.
- Pickova J, Kiessling A, Pettersson A, Dutta PC. 1998. Comparison of fatty acid composition and astaxanthin content in healthy and by M74 affected salmon eggs from three Swedish river stocks. Comp Biochem Physiol B. 120(2):265–271. doi:https://doi.org/10.1016/S0305-0491(98)10016-0.
- Rappe C, Soler T 1999. Baltic salmon rivers - status in the late 1990s as reported by the countries in the Baltic region. Ed. by C Rappe and T Soler, International Baltic Sea Fishery Commission (IBSFC) and HELCOM.
- Riley SC, Rinchard J, Honeyfield DC, Evans AN, Begnoche L. 2011. Increasing thiamine concentrations in lake trout eggs from Lakes Huron and Michigan coincide with low alewife abundance. N Am J Fish Manage. 31(6):1052–1064. doi:https://doi.org/10.1080/02755947.2011.641066.
- Røjbek MC, Tomkiewicz J, Jacobsen C, Støttrup JG. 2014. Forage fish quality: seasonal lipid dynamics of herring (Clupea harengus L.) and sprat (Sprattus sprattus L.) in the Baltic Sea. ICES J Mar Sci. 71(1):56–71. doi:https://doi.org/10.1093/icesjms/fst106.
- Romakkaniemi A, Pera I, Karlsson L, Jutila E, Carlsson U, Pakarinen T. 2003. Development of wild Atlantic salmon stocks in the rivers of the northern Baltic Sea in response to management measures. ICES J Mar Sci. 60(2):329–342. doi:https://doi.org/10.1016/S1054-3139(03)00020-1.
- Salminen M 2002. Marine survival of Atlantic salmon in the Baltic Sea. North Pacific Anadromous Fish Commission, Techn Rep No 4, pp. 27-29.
- Salminen M, Erkamo E, Salmi J. 2001. Diet of post-smolt and one-sea-winter Atlantic salmon in the Bothnian Sea, northern Baltic. J Fish Biol. 58(1):16–35. doi:https://doi.org/10.1111/j.1095-8649.2001.tb00496.x.
- Salminen M, Kuikka S, Erkamo E. 1994. Divergence in feeding migration of Baltic salmon (Salmo salar L.); the significance of smolt size. Nordic J Freshw Res. 69:32–42.
- Shimidzu N, Goto M, Miki W. 1996. Carotenoids as singlet oxygen quenchers in marine organisms. Fish Sci. 62(1):134–137. doi:https://doi.org/10.2331/fishsci.62.134.
- Spector AA. 2000. Lipid metabolism: essential fatty acids. In: Stipanuk MH, editor. Biochemical and physiological aspects of human nutrition. Philadelphia: Saunders/Elsevier; p. 365–383.
- Tacon AGJ. 1996. Lipid nutritional pathology in farmed fish. Arch Anim Nutr. 49:33–39.
- Tarhanen J, Koistinen J, Paasivirta J, Vuorinen PJ, Koivusaari J, Nuuja I, Kannan N, Tatsukawa R. 1989. Toxic significance of planar aromatic compounds in Baltic ecosystem - new studies on extremely toxic coplanar PCBs. Chemosphere. 18(1–6):1067–1077. doi:https://doi.org/10.1016/0045-6535(89)90238-5.
- Vuorinen PJ, Keinänen M, Heinimaa P, Iivari J, Juntunen E-P, Kannel R, Pakarinen T, Romakkaniemi A. 2014a. M74-oireyhtymän seuranta Itämeren lohikannoissa. RKTL:n työraportteja. 41/2014. (In Finnish).
- Vuorinen PJ, Keinänen M, Kiviranta H, Koistinen J, Kiljunen M, Myllylä T, Pönni J, Peltonen H, Verta M, Karjalainen J. 2012. Biomagnification of organohalogens in Atlantic salmon (Salmo salar) from its main prey species in three areas of the Baltic Sea. Sci Total Environ. 421-422:129–143. doi:https://doi.org/10.1016/j.scitotenv.2012.02.002.
- Vuorinen PJ, Keinänen M, Peuranen S, Tigerstedt C. 2003. Reproduction, blood and plasma parameters and gill histology of vendace (Coregonus albula L.) in long-term exposure to acidity and aluminum. Ecotoxicol Environ Safety. 54(3):255–276. doi:https://doi.org/10.1016/S0147-6513(02)00078-7.
- Vuorinen PJ, Kiviranta H, Koistinen J, Pöyhönen O, Ikonen E, Keinänen M. 2014b. Organohalogen concentrations and feeding status in Atlantic salmon (Salmo salar L.) of the Baltic Sea during the spawning run. Sci Total Environ. 468-469:449–456. doi:https://doi.org/10.1016/j.scitotenv.2013.08.075.
- Vuorinen PJ, Paasivirta J, Keinänen M, Koistinen J, Rantio T, Hyötyläinen T, Welling L. 1997. The M74 syndrome of Baltic salmon (Salmo salar) and organochlorine concentrations in the muscle of female salmon. Chemosphere. 34(5–7):1151–1166. doi:https://doi.org/10.1016/S0045-6535(97)00415-3.
- Vuorinen PJ, Parmanne R, Vartiainen T, Keinänen M, Kiviranta H, Kotovuori O, Halling F. 2002. PCDD, PCDF, PCB and thiamine in Baltic herring (Clupea harengus L.) and sprat [Sprattus sprattus (L.)] as a background to the M74 syndrome of Baltic salmon (Salmo salar L.). ICES J Mar Sci. 59(3):480–496. doi:https://doi.org/10.1006/jmsc.2002.1200.
- Vuorinen PJ, Rokka M, Ritvanen T, Käkelä R, Nikonen S, Pakarinen T, Keinänen M. 2020. Changes in thiamine concentrations, fatty acid composition, and some other lipid-related biochemical indices in Baltic Sea Atlantic salmon (Salmo salar) during the spawning run and pre-spawning fasting. Helgol Mar Res. 74(1):1–24. doi:https://doi.org/10.1186/s10152-020-00542-9.
- Warton DI, Hui FKC. 2011. The arcsine is asinine: the analysis of proportions in ecology. Ecology. 92(1):3–10. doi:https://doi.org/10.1890/10-0340.1.
- Welch DW, Futia MH, Rinchard J, Teffer AK, Miller KM, Hinch SG, Honeyfield DC. 2018. Thiamine levels in muscle and eggs of adult Pacific salmon from the Fraser River, British Columbia. J Aquat Anim Health. 30(3):191–200. doi:https://doi.org/10.1002/aah.10024.
- Werner RM, Rook B, Greil R. 2006. Egg-thiamine status and occurrence of early mortality syndrome (EMS) in Atlantic salmon from the St. Marys River, Michigan. J Great Lakes Res. 32(2):293–305. doi:https://doi.org/10.3394/0380-1330(2006)32[293:ESAOOE]2.0.CO;2.
- Woodward B. 1994. Dietary vitamin requirements of cultured young fish, with emphasis on quantitative estimates for salmonids. Aquaculture. 124(1–4):133–168. doi:https://doi.org/10.1016/0044-8486(94)90375-1.