ABSTRACT
Objectives: Chronic immune thrombocytopenia (cITP) is common in children. However, the pathogenesis has not been fully elucidated. This study aimed to determine whether thrombopoietin (TPO) and its receptor c-mannosylation of the TPO receptor (c-Mpl) have an impact on childhood cITP.
Methods: Sixty-four patients with newly diagnosed ITP (nITP), 64 patients with persistent ITP, 80 patients with cITP, and 64 healthy children (control) were enrolled in this study. Plasma TPO was measured with an ELISA, and c-Mpl was determined by flow cytometry.
Results: Plasma TPO levels showed differences among the four groups (p = 0.001). TPO levels in the cITP group were significantly decreased compared to those in the nITP group (p < 0.05). The mean fluorescence intensity (MFI) of c-Mpl was significantly different among the four groups (p = 0.0275). c-Mpl MFI was lower in the cITP group than in the nITP group(p < 0.05). Quantitative real-time PCR analysis showed that TPO mRNA expression was higher in the control group than in the ITP groups (p < 0.0001). The c-Mpl mRNA levels also showed significant differences among the four groups (p = 0.023). The control group, compared with the other groups, had lower levels of c-Mpl mRNA.
Conclusions: The expression of TPO and c-Mpl was significantly decreased in the cITP group compared to the nITP group, suggesting that TPO and its receptor may play important roles in childhood cITP pathogenesis.
Introduction
Primary immune thrombocytopenia (ITP) is the most common hemorrhagic disease reported in children. ITP is most common in children between the ages of 2 and 10 years, and the incidence of childhood ITP has been reported to vary between 3 and 8 per 100,000 [Citation1]. Differing from adult ITP, childhood ITP is self-limiting, and approximately 70% of patients with childhood ITP recover within 6 months, while the remaining 20–30% of patients develop chronic or refractory ITP [Citation2–4]. ITP is defined as newly diagnosed ITP (nITP), that is, within 3 months from the diagnosis; persistent ITP (pITP), that is, between 3 and 12 months from diagnosis, including patients not reaching spontaneous remission or not maintaining a complete response to therapy, and chronic ITP (cITP), that is, lasting for more than 12 months [Citation5].
At present, two main mechanisms of ITP pathogenesis are proposed. One is autoimmune destruction of platelets, and the other is reduced platelet production [Citation6]. Immune activation reduces platelet production and has been implicated in nITP; however, the mechanisms responsible for cITP may be more complicated [Citation7–9]. In our previous study, we found that the levels of platelet-specific auto-antibodies and platelet-associated antibodies declined during prolonged ITP. The expression of platelet antibodies was different between cITP and acute immune thrombocytopenia (aITP) patients, but no significant difference between cITP patients and the healthy control group was reported [Citation10], suggesting that antibody-mediated destruction of platelets may not be the primary cause of childhood cITP.
Thrombopoietin (TPO), also known as megakaryocyte growth development factor, is the most important regulator of proliferation, differentiation, and maturation of megakaryocytes and of platelet production. As a kind of early hematopoietic promoting factor, TPO exerts a biological effect by combining with its receptor, c-Mpl [Citation11,Citation12]. We aimed to explore the changes in TPO and its receptor in ITP, especially in cITP patients; three different types of ITP patients and healthy control children were included in our study.
Patients and methods
Patients
In total, 208 patients were enrolled in this study in our hospital from August 2013 to February 2017. The patients consisted of 108 males and 100 females with a median age of 4.87 years (3 months – 14 years). All patients were diagnosed with primary ITP according to the international diagnosis (International Working Group) and were divided into the nITP group, pITP group, and cITP group according to the duration of disease when diagnosed at our hospital [Citation5], after exclusion of the duplicate cases, and those in which thrombocytopenia was caused by hereditary thrombocytopenia, aplastic anemia, leukemia, other immunological disease, and infection such as hepatitis C virus and drug. Additionally, 64 cases of healthy children undergoing routine physical examination at our hospital were enrolled in the healthy control group ().
Table 1. Basic information on patients.
Follow-up was performed until 30 June 2017, resulting in a median follow-up time of 32 months (4–46 months). Written informed consent was obtained from all patients and the study was approved by the ethics committee of our hospital.
Determination of TPO concentration with an ELISA
After 8 hours of fasting, peripheral blood samples from subjects were collected using ethylene diamine tetraacetie acid dipotassium salt (EDTA-K2) as an anticoagulant. The TPO concentration of the platelet-free plasma was assessed using a Human Thrombopoietin Quantikine® ELISA kit (DTP00B, R&D Systems, MInneapolis, MN, USA) according to the manufacturer’s instructions.
PMBC surface c-Mpl mean fluorescence intensity determined by flow cytometry
Peripheral blood mononuclear cells (PBMCs) were isolated from peripheral blood using Ficoll-Hypaque (GE Healthcare, Uppsala, Sweden) gradient separation techniques. c-Mpl levels on the surface of PBMCs were assessed using a Fluorokine® Biotinylated Human Tpo kit (catalogue number: NFTP0; R&D Systems, Minneapolis, MN, USA) according to the guidelines provided by the manufacturer. The MFI of c-Mpl staining was assessed by flow cytometry (FCM) (Becton Dickinson Biosciences, USA) using CellQuest Pro software (version 3.3).
mRNA detection by quantitative real-time PCR
Cells were lysed using TRIzol (Life Technologies, USA). An RNA purity test was conducted using OD260/OD280. cDNA synthesis was performed using the PrimeScriptTM RT Master (Real-Time Perfect). The following gene-specific primers were used:
TPO forward primer: 5'- CCCCATCCCCTTTACTATCAT -3';
TPO reverse primer: 5' –GCGCTCCCATTTATTCCTTA- 3';
c-Mpl forward primer: 5'- CTGAGGTACGAACTCCGCTATG3';
c-Mpl reverse primer: 5' GCAGGTTTCTGTGGCAATCA- 3';
β-actin forward primer: 5'-TAGTTGCGTTACACCCTTTCTTG-3'; and
β-actinreverse primer: 5'-TCACCTTCACCGTTCCAGTTT -3';
β-actin PCR products were used as an internal control. 2-ΔΔCt was calculated to represent the relative mRNA expression of the target genes.
Statistical analysis
All statistical analyses were performed using GraphPad Prism 5 (GraphPadSoftware, Inc., La Jolla, CA, USA) and SPSS version 17.0 (SPSS Inc., Chicago, IL, USA). Data were compared using the Kruskal–Wallis H test for multi-groups, and using the Mann–Whitney U test for two groups. p < 0.05 was considered to indicate a statistically significant difference.
Results
Plasma TPO levels
Plasma TPO levels showed differences among the control group (mean 57.50 pg/ml; 95% CI: 48.40–66.60 pg/ml), nITP group (mean 77.88 pg/ml; 95% CI: 68.26–87.49 pg/ml), pITP group (mean72.59 pg/ml; 95% CI: 49.35–95.83 pg/ml), and cITP group (mean 56.93 pg/ml; 95% CI: 50.98–62.88 pg/ml) (p = 0.001). The TPO level in the cITP group was significantly decreased compared to that of the nITP group (p < 0.05). The TPO levels in the nITP group were significantly increased compared to those of the control group (p < 0.05). No statistically significant difference was found in the comparison between the cITP and control groups or between the cITP and pITP groups (p > 0.05) ((A)).
PBMC surface c-Mpl expression
The MFI of c-Mpl was significantly different among the four groups: control group (mean 888.3; 95% CI: 665.5–1111), nITP group (mean 1216; 95% CI: 1043–1390), pITP group (mean 1089; 95% CI: 901.8–1276), and cITP group (mean 914.2; 95% CI: 779.2–1050) (p = 0.0275). c-Mpl MFI was lower in the cITP group than in the nITP group (p < 0.05), and pITP group. There was no difference between the cITP group and the control group (p > 0.05) ((B)).
TPO and c-Mpl mRNA expressions
Quantitative real-time PCR (qRT-PCR) analysis showed that TPO mRNA expression was significantly increased in the control group (mean 3.176; 95% CI: 2.167–4.186) compared to that in the nITP group (mean 0.588, 95% CI: 0.292–0.884), pITP group (mean 0.097, 95% CI: 0.023–0.172), and cITP group (mean 0.755; 95% CI: 0.205–1.304) (p < 0.0001). The expression of TPO mRNA was also different in the three ITP groups, and the expression in the cITP group was the highest, followed by the nITP group and pITP group (p < 0.05) ((A)).
Figure 2. TPO and c-Mpl mRNA in ITP patients and the control group (A) TPO mRNA level in four groups; (B) C-Mpl mRNA level in four groups) (*mean p < 0.05).
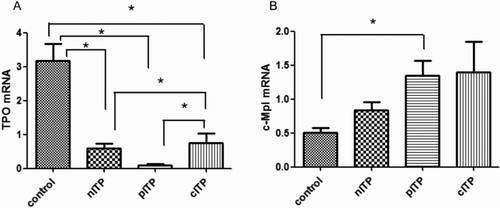
The c-Mpl mRNA levels also showed significant differences among the control group (mean 0.502; 95% CI: 0.362–0.643), nITP group (mean 0.834; 95% CI: 0.588–1.080), pITP group (mean 1.348; 95% CI: 0.912–1.784), and cITP group (mean 1.395; 95% CI: 0.486–2.303) (p = 0.023). The control group had a lower level than the other groups and was significantly decreased compared to that of the pITP group (p < 0.05) ((B)).
Discussion
It has been reported that increased destruction and reduced production of platelets mediated by an immune mechanism are involved in the pathogenesis of ITP [Citation13]. TPO plays an important role in stimulated or increased platelet production and promotes recovery from thrombocytopenia [Citation14]. TPO is produced mainly in liver, kidney, spleen, and bone marrow stromal cells [Citation15–17]. After TPO binds to c-Mpl, the TPO/c-Mpl complex is internalized, degrades in the proteasome, and is partially recycled to the cell surface. This process regulates serum TPO levels. In thrombocytopenia, as circulating levels of platelets are low, less TPO is cleared from the circulation, resulting in a high level of serum TPO [Citation17–22].
It was recently reported that different types of ITP patients have similar TPO levels. Prognostic factor analysis found TPO levels and a newly diagnosed state may have relevance. TPO levels in acute immune thrombocytopenia (disease course less than 6 months) were elevated compared with healthy controls [Citation23]. In our study, we found that the plasma TPO levels were higher in nITP patients than in healthy controls and the cITP group, similar to previous reports. Different TPO levels were found between the cITP group and nITP group, suggesting that disease development may influence TPO levels. ITP patients and the healthy control group had significantly different TPO levels, also suggesting that TPO may play an important role in ITP pathogenesis. Furthermore, it was reported that abnormal hepatic function in partial chronic patients undergoing long-term treatment may cause low TPO levels [Citation15–17], but we did not observe a similar result.
It was reported that TPO levels in patients with thrombocytopenia are related to the efficacy of TPO receptor agonists (TPO-RAs). An increased TPO level was associated with failure to respond to TPO-RA treatment. Ninety-three percent (14/15) of patients treated for ITP who had a TPO level that was lower than 95 ng/ml responded to TPO-RA treatment. However, only 17% (1/6) of patients with a TPO level that was higher than 95 ng/ml responded to TPO-RA treatment (p ≤ 0.002) [Citation24]. Therefore, TPO concentration is not only related to the pathogenesis of ITP but may also be related to its therapeutic effects.
Unlike megakaryocytes, PBMCs are not the main cells that produce TPO or c-Mpl. Very few studies reported the expression of TPO or c-Mpl in PBMCs. It has been reported that hematopoietic stem cells account for approximately 0.1% of PBMCs. In our study, washed PBMCs were incubated with biotinylated TPO that, in turn, binds to the cells via TPO receptors. The cells are then directly incubated with avidin–fluorescein, which attaches to the receptor-bound biotinylated TPO. Unbound biotinylated TPO results in an enhanced signal without compromising specificity. Cells expressing TPO receptors are fluorescently stained, with the intensity of staining proportional to the density of the receptors. The relative receptor density is then determined by FCM analysis. Therefore, we aimed to detect c-Mpl expression. Interestingly, we found that the MFIs of c-Mpl on the surface of PBMCs in different ITP groups were higher than that in the control group as well as in the nITP group compared to that in the cITP group, with statistical significance. The difference between the cITP group and control group was not significant. In patients with nITP, c-Mpl may show compensatory high expression in PBMCs, but this decompensation disappears when the disease persists to chronic conditions. This conclusion needs further study. The correlation between the expression of the TPO receptor and the therapeutic effect has not been reported in the literature. Further study will be helpful for understanding the pathogenesis and prognosis of ITP.
Some ITP patients develop cITP and/or do not respond to immunosuppressive therapy with corticosteroids or intravenous immunoglobulin. ITP has been effectively treated with recombinant human TPO and TPO-RAsin recent reports [Citation25,Citation26]. Romiplostim generated sustained remission in cITP and pITP patients. In 2015, eltrombopag was also approved by the FDA for use in children >6 years of age with ITP who were not responsive to first line therapies [Citation27–32]. The success of these therapies confirmed the role of TPO and its receptors in the pathogenesis of ITP.
To explore TPO and its receptor c-Mpl in bone marrow from patients with ITP, Zhang et al. [Citation33] used non-radioactive in situ hybridization to detect TPO and c-Mpl mRNA levels. They also used immune cytochemistry to detect c-Mpl on megakaryocytes. They found that the expression of TPO and c-Mpl mRNA in bone marrow and c-Mpl on megakaryocytes in patients with ITP was increased. Our study included different types of ITP groups, and TPO mRNA in PBMCs was detected by qRT-PCR. We found that TPO mRNA expression levels in the cITP group, pITP group, and nITP group were significantly lower than that of the control group (p < 0.05). It is an interesting phenomenon that TPO mRNA synthesis could be detected in PBMCs and was inhibited in ITP patients. There is a feedback mechanism between TPO and platelet count. TPO levels are regulated by the platelet count. A decrease in the platelet count leads to a decrease in circulating TPO in vivo and the elevated cellular TPO levels. The high level of TPO binding with c-Mpl on the surface of megakaryocytes promotes the production of platelets [Citation17–21]. In a future study, we would like to confirm whether TPO mRNA levels in PBMCs are consistent with levels in liver, kidney, spleen, and bone marrow stromal cells to understand the discrepancy between increased plasma TPO and decreased TPO mRNA levels in ITP children, compared with healthy children.
As a brief summary, we found that the expression levels of TPO and c-Mpl were significantly decreased in the cITP group compared to the nITP group. This is a new finding, suggesting that TPO and its receptor may play an important role in childhood cITP pathogenesis.
Acknowledgements
We would like to thank the clinicians at the Department of Hematology and Oncology, Shanghai Children’s Hospital, Shanghai, China, for providing the patient samples. Finally, we would like to thank the Clinical Laboratory of the Shanghai Children’s Hospital, Shanghai, China, and the Key Laboratory of Embryo Molecular Biology, Ministry of Health of China and the Shanghai Key Laboratory of Embryo and Reproduction Engineering, Shanghai, China, for their technical help and support.
Disclosure statement
No potential conflict of interest was reported by the authors.
Additional information
Funding
References
- Moulis G, Palmaro A, Montastruc JL, et al. Epidemiology of incident immune thrombocytopenia: a nationwide population-based study in France. Blood. 2014;124(22):3308–3315. doi: 10.1182/blood-2014-05-578336
- Terrell DR, Beebe LA, Neas BR, et al. Prevalence of primary immune thrombocytopenia in oklahoma. Am J Hematol. 2012;87(9):848–852. doi: 10.1002/ajh.23262
- Kuhne T, Imbach P, Bolton-Maggs PH, et al. Newly diagnosed idiopathic thrombocytopenic purpura in childhood: an observational study. Lancet. 2001;358(9299):2122–2125. doi: 10.1016/S0140-6736(01)07219-1
- Grace RF, Long M, Kalish LA, et al. Applicability of 2009 international consensus terminology and criteria for immune thrombocytopenia to a clinical pediatric population. Pediatr Blood Cancer. 2012;58(2):216–220. doi: 10.1002/pbc.23112
- Fogarty PF, Segal JB. The epidemiology of immune thrombocytopenic purpura. Curr Opin Hematol. 2007;14(5):515–519. doi: 10.1097/MOH.0b013e3282ab98c7
- Chang M, Nakagawa PA, Williams SA, et al. Immune thrombocytopenic purpura (ITP) plasma and purified ITP monoclonal autoantibodies inhibit megakaryocytopoiesis in vitro. Blood. 2003;102(3):887–895. doi: 10.1182/blood-2002-05-1475
- Blanchette V, Bolton-Maggs P. Childhood immune thrombocytopenic purpura: diagnosis and management. Pediatr Clin North Am. 2008;55(2):393–420. doi: 10.1016/j.pcl.2008.01.009
- Heitink-Polle KM, Nijsten J, Boonacker CW, et al. Clinical and laboratory predictors of chronic immune thrombocytopenia in children: a systematic review and meta-analysis. Blood. 2014;124(22):3295–3307. doi: 10.1182/blood-2014-04-570127
- Marshall GJ, Powars D, Ewing N, et al. Neutrophilic phagocytosis in autoimmune thrombocytopenia purpura. Am J Pediatr Hematol Oncol. 1982;4(4):375–383. doi: 10.1097/00043426-198224000-00004
- Aref S, Selim T, Ibrahim L, et al. Flowcytometry detection of platelets autoantibodies in children with idiopathic thrombocytopenic purpura. Indian J Hematol Blood Transfus. 2009;25(3):96–103. doi: 10.1007/s12288-009-0028-0
- Kaushansky K, Lok S, Holly RD, et al. Promotion of megakaryocyte progenitor expansion and differentiation by the c-Mpl ligand thrombopoietin. Nature. 1994;369(6481):568–571. doi: 10.1038/369568a0
- Sasazawa Y, Sato N, Suzuki T, et al. C-Mannosylation of thrombopoietin receptor (c-Mpl) regulates thrombopoietin-dependent JAK-STAT signaling. Biochem. Biophys. Res. Commun. 2015;468(1–2):262–268. doi: 10.1016/j.bbrc.2015.10.116
- Ghanima W, Holme PA, Tjønnfjord GE. Immune thrombocytopenia- pathophysiology and treatment. Tidsskr Nor Laegeforen. 2010;130(21):2120–2123. doi: 10.4045/tidsskr.09.1119
- Kuter DJ. Milestones in understanding platelet production: a historical overview. Br. J. Haematol. 2014;165(2):248–258. doi: 10.1111/bjh.12781
- Qian S, Fu F, Li W, et al. Primary role of the liver in thrombopoietin production shown by tissue-specific knockout. Blood. 1998;92(6):2189–2191.
- Sungaran R, Chisholm OT, Markovic B, et al. The role of platelet alpha-granular proteins in the regulation of thrombopoietin messenger RNA expression in human bone marrow stromal cells. Blood. 2000;95(10):3094–3101.
- Lok S, Kaushansky K, Holly RD, et al. Cloning and expression of murine thrombopoietin cDNA and stimulation of platelet production in vivo. Nature. 1994;369(6481):565–568. doi: 10.1038/369565a0
- Debili N, Wendling F, Cosman D, et al. The Mpl receptor is expressed in the megakaryocytic lineage from late progenitors to platelets. Blood. 1995;85(2):391–401.
- Forsberg EC, Prohaska SS, Katzman S, et al. Differential expression of novel potential regulators in hematopoietic stem cells. PLoS Genet. 2005;1(3):e28. doi: 10.1371/journal.pgen.0010028
- Methia N, Louache F, Vainchenker W, et al. Oligodeoxynucleotides antisense to the proto-oncogene c-mpl specifically inhibit in vitro megakaryocytopoiesis. Blood. 1993;82(5):1395–1401.
- Hattori H, Kuwayama M, Takamori H, et al. Development of chronic myelogenous leukemia during treatment with TPO receptor agonist for ITP. Rinsho Ketsueki. 2014;55(12):2429–2432.
- Hitchcock IS, Chen MM, King JR, et al. YRRL motifs in the cytoplasmic domain of the thrombopoietin receptor regulate receptor internalization and degradation. Blood. 2008;112(6):2222–2231. doi: 10.1182/blood-2008-01-134049
- Gu J, Lu L, Xu R, et al. Plasma thrombopoietin levels in patients with aplastic anemia and idiopathic thrombocytopenic purpura. Chin Med J (Engl). 2002;115(7):983–986.
- Makar RS, Zhukov OS, Sahud MA, et al. Thrombopoietin levels in patients with disorders of platelet production: diagnostic potential and utility in predicting response to TPO receptor agonists. Am J Hematol. 2013;88(12):1041–1044. doi: 10.1002/ajh.23562
- Cines DB, Gernsheimer T, Wasser J, et al. Integrated analysis of long-term safety in patients with chronic immune thrombocytopaenia (ITP) treated with the thrombopoietin (TPO) receptor agonist romiplostim. Int J Hematol. 2015;102(3):259–270. doi: 10.1007/s12185-015-1837-6
- Kuter DJ, Macahilig C, Grotzinger KM, et al. Treatment patterns and clinical outcomes in patients with chronic immune thrombocytopenia (ITP) switched to eltrombopag or romiplostim. Int J Hematol. 2015;101(3):255–263. doi: 10.1007/s12185-014-1731-7
- Vilaplana V E, Aragones JH, Fernandez-Llamazares CM, et al. Use of romiplostim for primary immune thrombocytopenia in children. Pediatr Hematol Oncol. 2012;29(2):197–205. doi: 10.3109/08880018.2011.629401
- Pasquet M, Aladjidi N, Guiton C, et al. Romiplostim in children with chronic immune thrombocytopenia (ITP): the French experience. Br J Haematol. 2014;164(2):266–271. doi: 10.1111/bjh.12609
- Seidel MG, Urban C, Sipurzynski J, et al. High response rate but short-term effect of romiplostim in paediatric refractory chronic immune thrombocytopenia. Br J Haematol. 2014;165(3):419–421. doi: 10.1111/bjh.12766
- Ramaswamy K, Hsieh L, Leven E, et al. Thrombopoietic agents for the treatment of persistent and chronic immune thrombocytopenia in children. J Pediatr. 2014;165(3):600–605.e4. doi: 10.1016/j.jpeds.2014.03.060
- Eser A, Toptas T, Kara O, et al. Efficacy and safety of eltrombopag in treatment-refractory primary immune thrombocytopenia: a retrospective study. Blood Coagul Fibrinolysis. 2016;27(1):47–52. doi: 10.1097/MBC.0000000000000380
- Rodeghiero F, Stasi R, Gernsheimer T, et al. Standardization of terminology, definitions and outcome criteria in immune thrombocytopenic purpura of adults and children: report from an international working group. Blood. 2009;113(11):2386–2393. doi: 10.1182/blood-2008-07-162503
- Zhang X, Yang Y, Shi H. Expression of thrombopoietin related genes in patients with idiopathic thrombocytopenic purpura. Zhonghua Xue Ye Xue Za Zhi. 1998;19(11):587–589.