ABSTRACT
Objectives: To explore the immunological abnormalities in patients with primary immune thrombocytopenia (ITP), and analyze its relationship with treatment.
Methods: Proportion of different immune cell subsets were detected in the peripheral blood of 124 ITP patients at different time points and 45 normal controls by flow cytometry. The treatments included glucocorticoids, intravenous IgG as first-line treatment and second-line drugs.
Results: Elevated CD4/CD8 ratio and decreased the proportion of NK and CD4 + CD25 + CD127low regulatory T cells (Tregs) were found in pre-treated ITP patients than healthy controls. The newly diagnosed group had a significantly higher CD4/CD8 ratio than the relapsed group, but no differences in the proportion of B cells, NK cells and Tregs. No relationships were found between the curative effect and the pre-treated cell subsets within both the effective and ineffective groups. Furthermore, compared with the ineffective group, the effective group had higher Tregs and lower CD4/CD8 ratio post-treatment, but no significant differences in NK and B cells.
Conclusion: ITP patients presented with a high CD4/CD8 ratio and low levels of Tregs and NK cells, suggesting that immune deregulation was involved in the pathogenesis of ITP. The pre-treated immune status of ITP patients may not be related to the curative effect. Tregs significantly increased in the effective group post-treatment, highlighting that the mechanism of restoring Tregs may be involved in the treatment of ITP. However, whether or not the targeted regulation of Tregs is an effective treatment for ITP still requires further studies.
Introduction
Immune thrombocytopenia (ITP) is an acquired immune-mediated disorder characterized by increased platelet destruction and reduced platelet production, eventually lead to decreased platelet count and hemorrhage symptoms [Citation1]. The incidence of ITP is approximately 2 and 12 per 100,000 adults and children, respectively, per year, and the mortality rate is about 1–3% per year in severely effective cases [Citation2,Citation3]. ITP may occur in isolation (primary ITP), or in association with other diseases (secondary ITP). Secondary ITP often relates to autoimmune diseases (such as systemic lupus erythematosus), viral infections (such as hepatitis C [HCV]) or drugs [Citation4]. However, the pathogenesis of primary ITP still remains unclear and is heterogeneous and complex. The presence of autoreactive antibodies against platelet glycoproteins (such as GPIIb/IIIa and GPIb/IX) was considered to play a central role in the cause of ITP [Citation5], while recently dysfunctional cellular immunity was found to be important in pathogenesis of ITP.
Abnormal cellular immune responses have been reported in ITP patients, which may link to a breakdown of the normal balance between immunity and self-tolerance in ITP [Citation6]. CD4+ T helper cells include Th1 cells (producing IFN-γ, IL-2 and TNF-β) and Th2 cells (producing IL-4, IL-5, IL-6, IL-10 and IL-13). Th1 cytokines may promote a pro-inflammatory response by facilitating macrophage activation and proliferation of cytotoxic T cells, and Th2 responses facilitate B-cell activation, proliferation and promote antibody production [Citation7,Citation8]. CD8+T cytotoxic cell-induced lysis of autologous platelets have also been found in patients with active ITP [Citation9]. However, is the immune state similarly in newly diagnosed and relapsed ITP patients? Is the immune state of patients with active ITP (newly diagnosed and relapsed ITP) related to the outcome of treatment? Are there any immunologic differences between effective and ineffective ITP patients after treatment? These questions still need to be investigated.
In this study, we detected changes in peripheral blood (PB) mononuclear cells within a cohort of ITP patients before and after treatment and analyzed the relationship between different groups according to the curative effect.
Materials and methods
Patients and controls
Institutional review board approval was obtained from The Second Affiliated Hospital of Anhui Medical University for the study, and informed consent was obtained from patients and the healthy donors to allow their information to be used in this study in accordance with the Declaration of Helsinki.
The patients with thrombocyte counts <100 × 109/l have no other causes or disorders that were associated with thrombocytopenia. These patients who have normal or increased megakaryocyte in bone marrow biopsy with no splenomegaly were diagnosed as ITP and enrolled in this study. One hundred twenty-four patients diagnosed as primary ITP were involved in this study (see ). Forty-five healthy volunteers were examined as normal controls. The ‘Newly diagnosed’ group (total n=64) who did not receive any initial treatment consisted of 23 males and 41 females with mean age 44.69 ± 18.57 years who were newly diagnosed. The ‘Relapsed’ group (total n=60) consisted of 18 males and 42 females with mean age 40.65 ± 18.65 years who lose complete response (CR) or response (R) (platelet count below 100 × 109/l or bleeding [from CR] or below 30 × 109/l or less than twofold increase of baseline platelet count or bleeding [from R], baseline platelet count refers to platelet count at the time of starting of the investigated treatment). In addition, 83 patients who were treated and evaluated for efficacy were selected and divided into two groups. The effective group included 19 males and 50 females with an age range of 14–78 (mean age 39.65 ± 19.80). The ineffective group included 4 males and 10 females with an age range of 18–68 (mean age 38.85 ± 21.68).
Table 1. Baseline characteristics of patients with ITP and controls.
Treatment
The patients in this retrospective study were all treated with IVIG (400 mg/kg per day for 5 days) plus corticosteroids (dexamethasone 40 mg per day for 4 days or prednisone at 1.0 mg/kg per day for 1–3 weeks, then tapered) according to first-line treatment standardization. Some patients with severe bleeding symptom were also given platelet transfusion to increase platelet counts. In addition to standard therapy, cyclosporine A, rituximab, vincristine, azathioprine, danazol, interleukin-11 and TPO were used as salvage therapy on non-respond patients.
Treatment responses
Treatment responses were evaluated according to the consensus definition of the International Working Group [Citation4], including CR, R, NR (no response) and loss of CR or R. In our study, the ‘Effective’ group is defined as whose response is CR or R. The ‘Ineffective’ group is defined as whose response is NR. Criteria to assessing these responses are CR: platelet count ≥100 × 109/l and the absence of bleeding. R: platelet count ≥30 × 109/l and at least twofold increase the baseline count and the absence of bleeding. NR: platelet count < 30 × 109/l or less than twofold increase of baseline platelet count or bleeding. Loss of CR or R: platelet count below 100 × 109/l or bleeding (from CR) or below 30 × 109/l or less than twofold increase of baseline platelet count or bleeding (from R). The blood samples were collected from the patients before they started treatment with thrombopoietic agents in ‘newly diagnosed’ and ‘relapsed’ group. In patients after treatment, the blood samples were all collected at the timing of maximal response. The study observation period to assess the response was at least 3 months at the time of the visit or until death (due to any cause) if it occurred before this date.
Flow cytometry
The following monoclonal antibodies (mAbs) specific for human surface antigens were all purchased from Beckman Coulter – Immunotech (Marseille, France).
Assessment of T-cell subsets, B cells and NK cells
The relative distributions of the T-cell subsets (CD3+ T cells, CD3 + CD4+ helper T cells, CD3 + CD8+ cytotoxic T cells), CD19 + CD20+ B cells and CD16 + CD56+ NK cells in the PB were stained with two- and three-color mAb, using FITC-conjugated CD4-specific mAb (13B8.2 clone), CD10-specific mAb (ALB1 clone) and CD16-specific mAb (3G8 clone), PE-conjugated CD8-specific mAb (B9.11 clone), CD19-specific mAb (J3.119 clone) and CD56-specific mAb (N901 clone), Percp-Cy5.5-conjugated anti-CD3 mAb (UCHT1 clone), APC-conjugated CD45-specific mAb (J.33 clone) and then analyzed with multiparameter FCM.
Assessment of regulatory T cells
2–5 ml of PB was collected from the patient. The sample should be anti-coagulated with heparin and examined within 4 h. About 100 μl of PB mononuclear cell (5×105) from PB was incubated at 25°C for 15 min with the FITC-conjugated CD25-specific mAb (B1.49.9 clone), PE-conjugated CD127-specific mAb (R34.34 clone) and Percp-Cy5.5-conjugated anti-CD4 mAb (13B8.2 clone), and appropriate isotype controls. After incubation, red blood cells were lysed and washed twice in PBS. Stained cells were assessed by flow cytometer FC-500 (Beckman Coulter, Miami, FL, U.S.A.) and analyzed using the Expo 32 multicomp Software (Beckman Coulter, Miami, FL, U.S.A.). Within the CD4+ T cell gate on lymphocytes, CD4+ regulatory T cells (Tregs) are identified as CD25 + CD127low.
Statistical analysis
Statistical analysis was performed using the SPSS 17.0 software (SPSS Inc, Chicago, IL, U.S.A.). Differences in the cell subsets among the groups were analyzed by the nonparametric unpaired Mann–Whitney U-test and the parametric Student's t-test for paired or unpaired samples when appropriate. Frequency differences of sex, splenomegaly, hepatomegaly or hemorrhagic symptoms according to the baseline characteristics of patients with ITP and controls were assessed using a chi-square test. A P-value of less than 0.05 was considered to be statistically significant.
Results
Immune state in active ITP
All the patients involved in this study were diagnosed as primary ITP and divided into two groups: newly diagnosed and relapsed. Hemorrhagic symptoms were present in 111 patients (89.5%) in the form of petechial hemorrhage and some bruising. None of the patients had enlarged liver, spleen or lymphadenopathy. There were no significant differences in age and sex between ITP and relevant control groups. Platelet count was significantly decreased within ITP patients as compared with controls (see ). No significant differences were detected in the proportions of CD3+T, CD8+T and B lymphocytes between ITP patients and controls. The proportion of CD8+T lymphocytes was found to be significantly different between the ‘newly diagnosed’ and control groups. The percentage of CD4+T lymphocytes was significantly increased in the ‘newly diagnosed’ group than the controls, but not in the ‘relapsed’ group. The CD4/CD8 ratio and NK and CD4 + CD25 + CD127lowTreg cell counts were significantly decreased in all ITP patients in contrast to controls (see ).
Table 2. Proportion of cell subsets in new diagnosed ITP, relapsed ITP and healthy controls.
The newly diagnosed patients have a significantly higher ratio of CD4/CD8 than in relapsed patients. This difference would be due to higher levels of CD8+ T cells in group of relapsed ITP. The reasons for the difference in the CD4/CD8 ratio between the newly diagnosed and relapsed ITP patients may be related to the treatment within the relapsed patients. However, there are no significant differences in the percentage of NK cell and Tregs between those two groups (see ).
Pre-treatment immune status in ‘effective’ and ‘ineffective’ groups with active ITP
There were no significant differences in age, sex and platelet count between the two groups. We also analyzed the proportion of cell subsets prior to treatment in all patients involved. The results showed that there were no significant differences, and also indicated that there was no relationship between the therapeutic effect and the cell subsets before treatment (see ).
Table 3. Cell subsets in ‘effective’ and ‘ineffective’ group before treatment.
Post-treatment immune status in ‘effective’ and ‘ineffective’ groups with active ITP
We examined the T cell subsets and Tregs after treatment in 29 patients and analyzed the correlation between the CD4/CD8 ratio and the levels of Tregs during the patients’ response to ITP therapy. Eighteen patients designated as the ‘effective’ group and 11 patients as the ‘ineffective’ group were examined, and significant differences were found in the CD4/CD8 ratio and the proportion of CD4+Treg cells between the two groups. But no significant differences were detected in the proportions of NK and B cells (see ).
Figure 1. CD4/CD8 ratio, CD4+Treg cells, NK cells and B cells in ‘effective’ and ‘ineffective’ group after treatment. (A) CD4/CD8 ratio in ‘ineffective’ group, ‘effective’ group, healthy controls were 2.23 ± 1.10, 1.39 ± 0.80 and 1.25 ± 0.29, respectively. (B) The proportion of CD4+Treg cells (within CD4+ T cell gate) in ‘ineffective’ group, ‘effective’ group, healthy controls were 5.16 ± 1.92, 7.26 ± 2.23 and 5.21 ± 1.17, respectively. (C) The proportion of NK cells (within lymphocyte gate) in ‘ineffective’ group, ‘effective’ group, healthy controls were 6.36 ± 4.54, 7.05 ± 5.42 and 14.15 ± 5.42, respectively. (D) The proportion of B cells (within lymphocyte gate) in ‘ineffective’ group, ‘effective’ group, healthy controls were 3.77 ± 2.75, 5.08 ± 3.16 and 4.40 ± 1.94, respectively.
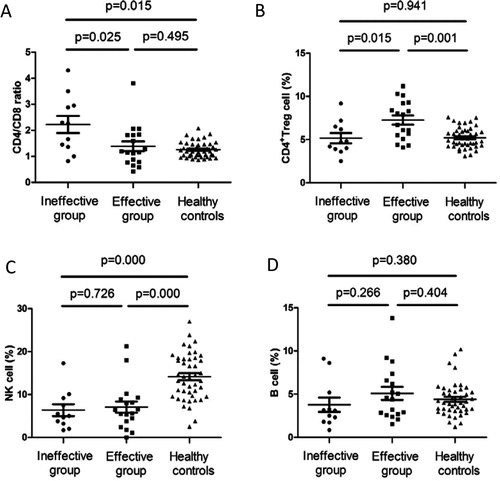
Compared with the ‘ineffective’ group, the ‘effective’ group had higher levels of Tregs and a lower CD4/CD8 ratio after treatment, while NK and B cell counts remained relatively unchanged. As compared with healthy controls, the proportion of NK cells were significantly decreased in both the ‘effective’ and ‘ineffective’ groups, however, no significant differences were found in the percentage of B cells.
In terms of the patient's examinations which were all determined by clinicians, only 15 patients were examined pre- and post-therapy, including 10 and 5 patients from the ‘effective’ and ‘ineffective’ groups, respectively. The result showed that the proportion of CD4+ Treg cells was only significantly increased in the ‘effective’ group (see ).
Figure 2. Pre- and post-treatment immune state in ‘effective’ and ‘ineffective’ group. (A) CD4/CD8 ratio, proportion of CD4+Treg cell, NK cell and B cell in ‘effective’ group (n=10). (B) CD4/CD8 ratio, proportion of CD4+Treg cell, NK cell and B cell in ‘ineffective’ group (n=5).
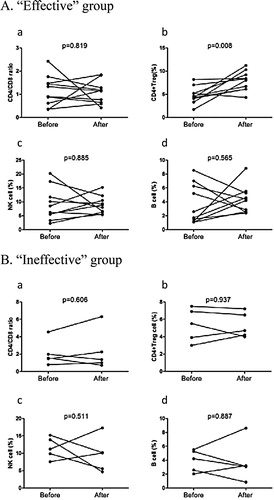
In addition, for patients that have either received or not received platelet transfusion, we divided the ‘effective’ group into two sub groups: transfused vs. non-transfused. We then analyzed for differences between the groups. The results show that there were no significant differences in the immune status after treatment (see supplemental Table S1).
Discussion
ITP is one of the common causes of thrombocytopenia in both children and adults. Despite studies showing that increased levels of autoantibodies against self-antigens plays a crucial role in platelet destruction, the pathophysiology of ITP still remains complicated and requires further studies. As ITP is an autoimmune disease, it is essential to further understand the influence of the immune-related mechanisms in primary ITP at the time of diagnosis and during treatment. In this retrospective study, we analyzed T lymphocyte subsets, B lymphocytes and NK cells within the PB of 124 patients diagnosed with primary ITP before and after treatment in our department. We also examined these parameters in patients who received effective and ineffective treatments and tried to analyze the relationship between these immune parameters and therapeutic outcomes. This might be helpful for clinicians in choosing alternative treatments for ITP patients.
Abnormal T-lymphocyte subpopulations in patients with ITP have been previously reported. Some studies have reported increased CD8+ T lymphocytes and decreased CD4/CD8 ratios [Citation10]. Other reports, however, have indicated normal CD4/CD8 ratios [Citation11–13], increased numbers of both CD4+ T and CD8+ T lymphocytes [Citation12,Citation14], or decreased CD8+ T lymphocytes alone in patients with ITP [Citation15]. In our study, we found that CD4/CD8 ratios in active ITP patients were increased. The differences of CD4/CD8 ratios may be related to the complex pathogenesis of ITP. According to treatment responses, the CD4/CD8 ratios almost returned to normal in the ‘effective’ group than the ‘ineffective’ and control groups. This result further confirmed that cellular immunity participated in the pathogenesis of ITP.
NK cells are a type of cytotoxic lymphocytes critical to the innate immune system. They can regulate B-cell immunoglobulin production and may have an influence on autoantibody production within autoimmune diseases. However, whether it participates in the pathogenesis of ITP and the associated mechanisms still need more studies. In our study, we found that NK cells were significantly decreased in active ITP patients than in controls. This was in agreement with Talaat et al. [Citation16] and El-Rashedi et al. [Citation10]. For ITP patients who had undergone therapy, Garcia-Suarez, et al. found that the activity of NK cells was correlated with therapy treatment, meaning that there was an increase in the number of NK cells in ITP patients who were effectively treated [Citation17]. However, no significant difference was found in our study between ITP patients who were effective treated or not. This may due to the small sample size, we will do more studies in the future.
B cells can produce antiplatelet autoantibodies and cause platelet destruction in ITP patients. Several studies have shown that B cells may be higher in ITP patients than normal individuals [Citation7,Citation18]. As we know, the mechanism behind the production of antigen-specific antibodies by B lymphocytes is controlled by various regulatory agents [Citation19]. The antigen should be recognized by B cells, APCs and CD4 + Th cells first, from which it will undergo proteolytic processes and be subsequently presented to Th cells. Afterwards, the Th cells become activated and secrete lymphokines, which stimulate antigen-specific B cells to produce and secrete antibodies. In our study, there were no significant differences in the number of B cells between our groups. This may due to the complex mechanism behind B cell activation, and the different functions in the subgroups of the B cell line. We will conduct more research into the B cell line subgroups within ITP in the future.
Tregs also play an important role in the maintenance of peripheral tolerance. A number of studies have reported on abnormal Treg activity in ITP patients. Among these studies, the majority of them demonstrated a decreased frequency of Tregs in the PB of ITP patients in contrast with healthy controls [Citation20], while others have found no such significant differences [Citation21–24]. The lowest frequency of Tregs was found in patients with acute phase of the disease, which consequently increased during remission but remained unchanged during non-remission [Citation25,Citation26]. In our study, we also found that Tregs were reduced in newly diagnosed and relapsed patients, which were recovered following treatment in our ‘effective’ group. This result showed that Tregs may have had some effect during the course of the treatment, and therefore the approach to increase Tregs could be considered in the treatment of ITP. We have previously used low-dose IL-2 to treat several ITP cases, and the result also showed that the elevation in platelet count was correlated with the increase in Treg numbers [Citation27,Citation28].
In conclusion, a high ratio of CD4/CD8 and low levels of Tregs and NK cells present in ITP patients, suggesting the immune deregulation has involved in the pathogenesis of ITP. The pre-treatment immune status of ITP patients may not be related to the curative effect. The number of Tregs significantly increased in ITP patients who received effective therapy, demonstrating that the restoration of Tregs may be a mechanism involved in the treatment of ITP. Whether or not the targeted regulation of Tregs is an effective treatment for ITP still requires further studies.
Acknowledgment
The authors thank the patients and their families and all the investigators, including the physicians and laboratory technicians in this study.
Disclosure statement
No potential conflict of interest was reported by the authors.
Additional information
Funding
References
- Cines DB, Blanchette VS. Immune thrombocytopenic purpura. N Engl J Med. 2002;346(13):995–1008. doi: 10.1056/NEJMra010501
- Cohen YC, Djulbegovic B, Shamai-Lubovitz O, et al. The bleeding risk and natural history of idiopathic thrombocytopenic purpura in patients with persistent low platelet counts. Arch Intern Med. 2000;160(11):1630–1638. doi: 10.1001/archinte.160.11.1630
- Michel M. Immune thrombocytopenic purpura: epidemiology and implications for patients. Eur J Haematol Suppl. 2009;82:3–7. doi: 10.1111/j.1600-0609.2008.01206.x
- Rodeghiero F, Stasi R, Gernsheimer T, et al. Standardization of terminology, definitions and outcome criteria in immune thrombocytopenic purpura of adults and children: report from an international working group. Blood. 2009;113(11):2386–2393. doi: 10.1182/blood-2008-07-162503
- Gernsheimer T. Chronic idiopathic thrombocytopenic purpura: mechanisms of pathogenesis. Oncologist. 2009;14(1):12–21. doi: 10.1634/theoncologist.2008-0132
- Ji X, Zhang L, Peng J, et al. T cell immune abnormalities in immune thrombocytopenia. J Hematol Oncol. 2014;7:139.
- Culić S, Labar B, Marusić A, et al. Correlations among age, cytokines, lymphocyte subtypes, and platelet counts in autoimmune thrombocytopenic purpura. Pediatr Blood Cancer. 2006;47(5 Suppl):671–674. doi: 10.1002/pbc.20999
- Zhang G, Zhang P, Liu H, et al. Assessment of Th17/Treg cells and Th cytokines in an improved immune thrombocytopeniamouse model. Hematology. 2017;22(8):493–500.
- Olsson B, Andersson PO, Jernås M, et al. T-cell-mediated cytotoxicity toward platelets in chronic idiopathic thrombocytopenic purpura. Nat Med. 2003;9(9):1123–1124. doi: 10.1038/nm921
- El-Rashedi FH, El-Hawy MA, Helwa MA, et al. Study of CD4+, CD8+, and natural killer cells (CD16+, CD56+) in children with immune thrombocytopenic purpura. Hematol Oncol Stem Cell Ther. 2017;10(1):8–14. doi: 10.1016/j.hemonc.2017.01.001
- Lauria F, Raspadori D, Gobbi M, et al. T-cell subsets defined by monoclonal antibodies in autoimmune thrombocytopenic purpura (ATP). Haematologica. 1983;68(5):600–606.
- Scott CS, Wheeler R, Ford P, et al. T lymphocyte subpopulations in idiopathic thrombocytopenic purpura (ITP). Scand J Haematol. 1983;30(5):401–406. doi: 10.1111/j.1600-0609.1983.tb02525.x
- Koyanagi H, Kishida K, Shimomura K, et al. Immunological study of childhood acute ITP at onset. Pediatr Hematol Oncol. 1992;9(1):11–19. doi: 10.3109/08880019209006391
- Mizutani H, Katagiri S, Uejima K, et al. T-cell abnormalities in patients with idiopathic thrombocytopenic purpura: the presence of OKT4+8+ cells. Scand J Haematol. 1985;35(2):233–239. doi: 10.1111/j.1600-0609.1985.tb01580.x
- Ware RE, Howard TA. Phenotypic and clonal analysis of T lymphocytes in childhood immune thrombocytopenic purpura. Blood. 1993;82(7):2137–2142.
- Talaat RM, Elmaghraby AM, Barakat SS, et al. Alterations in immune cell subsets and their cytokine secretion profile in childhood idiopathic thrombocytopenic purpura (ITP). Clin Exp Immunol. 2014;176(2):291–300. doi: 10.1111/cei.12279
- Garcia-Suarez J, Prieto A, Reyes E, et al. Severe chronic autoimmune thrombocytopenic purpura is associated with an expansion of CD56+ CD3- natural killer cells subset. Blood. 1993;82(5):1538–1545.
- Rong W, Yan-xiang Z, Shan-shan X, et al. Lymphocyte subsets in primary immune thrombocytopenia. Blood Coagul Fibrinolysis. 2014;25(8):816–819. doi: 10.1097/MBC.0000000000000155
- Semple JW, Freedman J. Increased antiplatelet T helper lymphocyte reactivity in patients withautoimmune thrombocytopenia. Blood. 1991;78(10):2619–2625.
- Nishimoto T, Kuwana M. CD4+CD25+Foxp3+ regulatory T cells in the pathophysiology of immune thrombocytopenia. Semin Hematol. 2013;50(Suppl 1):S43–S49. doi: 10.1053/j.seminhematol.2013.03.018
- Yu J, Heck S, Patel V, et al. Defective circulating CD25 regulatory T cells in patients with chronic immune thrombocytopenic purpura. Blood. 2008;112:1325–1328. doi: 10.1182/blood-2008-01-135335
- Olsson B, Ridell B, Carlsson L, et al. Recruitment of T cells into bone marrow of ITP patients possibly due to elevated expression of VLA-4 and CX3CR1. Blood. 2008;112:1078–1084. doi: 10.1182/blood-2008-02-139402
- Bao W, Bussel JB, Heck S, et al. Improved regulatory T-cell activity in patients with chronic immune thrombocytopenia treated with thrombopoietic agents. Blood. 2010;116:4639–4645. doi: 10.1182/blood-2010-04-281717
- Audia S, Samson M, Guy J, et al. Immunologic effects of rituximab on the human spleen in immune thrombocytopenia. Blood. 2011;118:4394–4400. doi: 10.1182/blood-2011-03-344051
- Teke HÜ, Gündüz E, Akay OM, et al. Abnormality of regulatory T-cells in remission and non-remission idiopathic thrombocytopaenic purpura patients. Platelets. 2013;24(8):625–631. doi: 10.3109/09537104.2012.748188
- Stasi R, Cooper N, Del Poeta G, et al. Analysis of regulatory T-cell changes in patients with idiopathic thrombocytopenic purpura receiving B cell-depleting therapy with rituximab. Blood. 2008;112(4):1147–1150. doi: 10.1182/blood-2007-12-129262
- Zhang J, Ruan Y, Shen Y, et al. Low dose IL-2 increase regulatory T cells and elevate platelets in a patient with immune thrombocytopenia. Cytometry B Clin Cytom. 2016;Nov. doi: 10.1002/cyto.b.21494
- Zhang J, Ruan Y, Xu X, et al. Therapeutic potential of low-dose IL-2 in immune thrombocytopenia: An analysis of 3 cases. Cytometry B Clin Cytom. 2017;Nov. doi: 10.1002/cyto.b.21601