Abstract
Early life stress (ELS) is a risk factor in the development of psychiatric disorders. The underlying biological mechanisms governing this phenomenon are not fully understood, but dysregulation of stress responses is likely to play a key role. Males and females differ in their propensity to develop psychiatric disorders, with far higher rates of anxiety, major depressive disorder, affective disorders and post-traumatic stress disorder found in women. We hypothesized that sex differences in response to ELS may play a crucial role in differential vulnerability between the sexes. To test this, we evaluated the consequences of pre-pubertal stress (PPS) on the HPA axis in adult female and male Lister Hooded rats. PPS animals were exposed to swim, restraint and elevated platform stress on postnatal days 25–27, controls remained in their home cage. Once adult, animals were either a) sacrificed directly and brains collected or b) sacrificed 20 minutes or 1 week after a social test and trunk blood collected. In the female hippocampal formation, PPS increased expression of FKBP5 and AVPR1a. In the female prefrontal cortex, PPS resulted in increased glucocorticoid receptor expression, increased glucocorticoid:mineralocorticoid (GR:MR) receptor expression ratio and decreased AVPR1a expression. Females exposed to PPS did not show the normal rise in blood corticosterone levels following a social interaction test. In contrast, PPS did not alter the expression of oxytocin or oxytocin receptors, and no effects of PPS were seen in males. However, striking sex differences were found. Females had higher oxytocin receptor expression in the prefrontal cortex and AVPR1a and oxytocin expression in the hypothalamus, whereas males demonstrated higher expression of GR, MR, GR:MR, FKBP5 and oxytocin receptor in the hypothalamus. These results demonstrate heightened reactivity of the female HPA axis to PPS and may help explain why in humans females display an increased susceptibility to certain stress-related psychopathologies.
Women are at greater risk of developing several psychiatric illnesses. Using a rodent model, we show that the female stress system is more reactive to the lasting effects of early life stress. This heightened reactivity of the female stress response may help explain why women are at a greater risk of developing psychiatric disorders.
LAY SUMMARY
Introduction
Adverse experiences early in life are linked with an increased risk of developing psychiatric disorders later in life (Heim & Nemeroff, Citation2001; Juruena, Baes, Menezes, & Graeff, Citation2015; Teicher & Samson, Citation2016; Teicher, Samson, Anderson, & Ohashi, Citation2016). Dysregulation of the stress response is a potential mechanism through which early life stress (ELS) increases vulnerability to illness. Prolonged or excessive stress may lead to a maladaptive stress response, and when experienced early in life could also alter brain development, increasing vulnerability to psychiatric disorders. Stress results in several adaptive physiological and behavioral responses, a major mediator of this is the hypothalamic-pituitary-adrenal (HPA) axis.
Both psychological and physical stressors result in the release of corticotrophin releasing hormone (CRH) and arginine vasopressin (AVP) from the paraventricular nucleus (PVN) of the hypothalamus. These neuropeptides act on the pituitary, stimulating the release of adrenocorticotrophic hormone (ACTH) which in turn causes the release of glucocorticoid stress hormones (corticosterone in rodents, cortisol in humans (CORT)) from the adrenal cortex (de Kloet, Joels, & Holsboer, Citation2005). Glucocorticoids cross the blood brain barrier and bind to corticosteroid receptors (CR: glucocorticoid (GR) and mineralocorticoid (MR) receptors) distributed throughout the brain. Feedback mechanisms then ensure the response is terminated in a healthy system. In contrast to AVP, the closely related neuropeptide oxytocin (OXT) inhibits the activity of the HPA axis (Neumann & Landgraf, Citation2019). HPA axis dysfunction is prevalent in psychiatric illness, for example HPA axis hyperactivity is often found in major depression and bipolar disorder, and increased or decreased HPA axis activity may be a direct consequence of ELS (Juruena, Cleare, & Young Citation2018; Murri et al., Citation2016; Zorn et al., Citation2017).
Long-term effects of ELS on the HPA axis differ between the sexes. Early trauma is associated with a more severely blunted cortisol response to social stress in women, and fewer stressful events early in life are required to trigger liability to PTSD in women. Conversely, lower levels of recent stress are capable of provoking major depression in men than women (Bunea, Szentágotai-Tătar, & Miu, Citation2017; McLaughlin, Conron, Koenen, & Gilman, Citation2010). Furthermore, women display 2–3 times higher rates of anxiety, affective disorders, major depressive disorder and post-traumatic stress disorder (PTSD) (Christiansen & Hansen, Citation2015; Kessler et al., Citation2003; Kessler, Chiu, Demler, Merikangas, & Walters, Citation2005; Kessler, McGonagle, Swartz, Blazer, & Nelson, Citation1993; Remes, Brayne, van der Linde, & Lafortune, Citation2016). These differences are not purely attributable to sex-specific life experiences; studies controlling for stressful life events and sex-specific risk factors still find higher prevalence in women (Tiwari & Gonzalez, Citation2018). Sex differences in HPA axis function may underlie this. Basal secretion of CORT from the adrenal gland is higher in females than males, and this is attributed to sex-differences in gonadal hormones, with estrogen sensitizing and testosterone dampening the HPA-axis (Heck & Handa, Citation2019; Seale et al., Citation2004).
Animal studies demonstrate that ELS has profound implications for later HPA axis function. Prenatal and early post-natal stressors alter basal and stress-induced corticosterone release from the adrenal glands, brain corticosteroid receptor (GR and MR) expression, as well as expression of AVP and OXT in a timing and sometimes sex-specific manner (Llorente et al., Citation2011; Lupien, McEwen, Gunnar, & Heim, Citation2009; Neumann & Landgraf, Citation2019; Schroeder, Notaras, Du, & Hill, Citation2018; Tobon, Jeffrey, & Nemeroff, Citation2018). However, despite well-established sex differences in the HPA axis, comparatively few preclinical studies include male and female animals. Compared to the prenatal and post-natal periods, less is known about the effects of stress experienced in the post-weaning, pre-pubertal phase (PPS), a time-point suggested as more akin to human childhood (Brydges, Citation2016). The limbic system and prefrontal cortex are undergoing maturation during this period, areas which are crucial for cognition and emotion and are extremely stress reactive due to high densities of CR, particularly in the hippocampal formation (Herman, Citation1993).
The present study investigated the effects of PPS on long-term neurochemical and molecular alterations in the adult HPA axis in male and female animals by measuring the brain regional expression of CR (GR and MR), AVP, OXT and their receptors (AVP receptor 1a (AVPR1a) and oxytocin receptor (OXTR)) and FKBP5. FKBP5 encodes the FK506 binding protein 51 co-chaperone protein of the GR complex, and is extremely responsive to stress (Wochnik et al., Citation2005). When FKBP5 is bound to the GR complex, CORT binds with lower affinity and nuclear translocation of the receptor is less efficient, decreasing negative feedback regulation of the HPA axis (Wochnik et al., Citation2005). There is evidence that genetic modifications in FKBP5 interact with childhood, but not adulthood stress to increase risk for several psychiatric disorders (Matosin, Halldorsdottir, & Binder, Citation2018). We also measured plasma corticosterone following a social test in adult rats as a behavioral measure of altered HPA axis function. Altered social function is a core component of several adult psychiatric illnesses and ELS has been shown to impact on social behavior and functioning in both animal and human studies (Nicol, Pope, Romaniuk, & Hall, Citation2015; Palmier-Claus et al., Citation2016; Sandi & Haller, Citation2015). Furthermore, early life trauma is associated with blunted cortisol responses to social stress in humans, particularly in women (Bunea et al., Citation2017). For this reason, we elected to focus on corticosterone rather than other components of the HPA axis, such as ACTH.
We hypothesized that PPS would alter the expression of GR, MR, GR:MR ratio, AVPR1a, AVP and OXT in the rodent brain, and the direction of change would be region and receptor/neuropeptide specific. Given their higher vulnerability to stress-related psychiatric illnesses, we hypothesized dysregulation resulting from PPS would be more pronounced in females. We also hypothesized that corticosterone responses to social stress would be blunted in both males and females, but more exaggerated in females, as early life trauma is often associated with a more pronounced blunting of the corticosterone response in females (Bunea et al., Citation2017; McLaughlin et al., Citation2010).
Methods
Animals
Male and female Lister Hooded rats were bred at Cardiff University from 16 adult pairs (Charles River). Females were primiparous. Litters ranged between 11 and 18 animals, with an average of 14.5, and an average sex-ratio of 6.2 males to 8.3 females. All litters were used and weaning from the birth dams took place on postnatal day (PND) 21, and offspring were housed in groups of 2–4 in same litter, same sex cages (32 cm × 50 cm × 21 cm) lined with wood shavings. Light was maintained on 12:12 hour light/dark cycle, a wooden stick, nesting material and cardboard tube were provided for enrichment and food and water provided ad libitum. All experiments were approved by Cardiff University’s Animal Welfare and Ethical Review Body and adhered to the UK Home Office Animals (Scientific Procedures) Act 1986 and European regulations on animal experimentation.
Pre-pubertal stress (PPS)
Half of the offspring (8 litters) were pseudo-randomly allocated to a PPS protocol (Jacobson-Pick & Richter-Levin, Citation2010) on PND 25-27 such that litters and sexes were equally distributed between treatment groups (PPS/control, male/female). PPS took place in a designated room separate to the holding room, with regular room lighting. On PND 25, animals were placed into an opaque swim tank (25 cm high, 34 cm diameter) filled with 6 L of 25 ± 1 °C water for a 10 minute swim stress. On PND26 the rats were restrained in plastic restraint tubes (15 cm length 5 cm diameter) for 3 × 30 minute sessions (separated by 30 minute breaks in the home cage) and lastly on PND27 they were exposed to elevated platforms (15 × 15 cm, 115 cm high) for 3 × 30 minute sessions (separated by 60 minute breaks in the home cage). Animals were observed by the experimenter during all stress procedures, and males and females reacted in a similar manner to each stressor. Following PPS, animals were left undisturbed until adulthood aside from weekly cage cleaning. Control animals were left undisturbed from weaning until adulthood, aside from weekly cage cleaning.
RT-qPCR
Forty rats (male: 12 control, 10 PPS; female: 8 control, 10 PPS) were sacrificed at PND 60-70 using a rising concentration of CO2. Brains were removed, dissected and stored at -80 °C until analysis. Total cell RNA was extracted from hippocampal formation, prefrontal cortex and hypothalamus using the Qiagen RNeasy Kit (Qiagen, Manchester, UK) and DNAse treated in accordance with the supplied protocols. RNA was used to create cDNA for analysis using RNA to cDNA Easy Premix (Clontech Laboratories, France), heated at 42 °C for 75 minutes, followed by 80 °C for 15 minutes. Sample was then diluted 1:15 in nuclease-free water. 96-well plates were loaded, each well containing a total of 15 μl reaction mixture (1.9 μl sterile RNAase free water, 0.3 μl 10 μM forward primer, 0.3 μl 10 μM reverse primer, 7.5 μl SensiMix (Bioline) and 5 μl cDNA). Gapdh and Hprt1 primers (Sigma) were used as housekeeping controls and all results were normalized from these values. After loading, plates were spun down at 3,000 rpm for approximately 10–20 seconds before being transferred to Real-Time PCR instrument (Applied Biosystems®) and run for 45 cycles (95 °C for 20 s, 60 °C for 20 s, 72 °C for 20 s). The expression of GR, MR, AVP, OXT, AVPR1a, OXTR and FKBP5 was measured (see for primers).
Table 1. PCR primers.
Social test
Sixty-two animals (females: 22 control, 18 PPS; male: 12 control, 10 PPS) were given a social test in same-sex pairs in adulthood (PND 60-67). Three hours before testing animals were single housed in the holding room, and one hour before testing transferred to the testing room. All animals were given an intraperitoneal injection of a vehicle (15%DMSO, 2% Tween 80 in 0.9% saline) 30 minutes before testing as part of a design to measure the effect of PPS on social behavior directly, an experiment which included a drug treated group. PPS had a significant effect on social interaction, and this behavioral data is reported elsewhere (Brydges et al. Citationunder review). Animals were weighed on the day of testing and placed in weight-matched pairs (weight difference did not exceed 20 g) into a clear acrylic arena (65 cm × 65 cm × 40 cm high) on the floor in the middle of a dimly lit room (45 lux) for 15 minutes. Animal pairs were from the same group, control or PPS, but different litters so were strangers to each other.
Corticosterone ELISA
One animal from each pair was sacrificed 20 minutes after the social test to investigate corticosterone responses to social interaction, the other sacrificed one week later for baseline analysis. Animals were decapitated and trunk blood was collected using EDTA microvette collection tubes (Sarstedt, Germany). Blood was spun at 1,500 × g for 10 minutes, plasma was removed and stored at −20 °C until analysis. Cortiscosterone was analyzed by ELISA, according to the manufacturer’s instructions (Abcam, UK, ab108821). The sensitivity of this ELISA is 0.28 ng/ml and the intra-assay coefficient of variation is 5.3%. Samples were run in triplicate on several plates, counterbalancing between groups.
Data analysis
JMP statistical software (SAS Institute, Cary, NC, USA) was used to run generalized linear models. For mRNA analysis, group (control/PPS), sex and group*sex were fitted as factors and mRNA expression (normalized to Gapdh & Hprt1) as response. For corticosterone, group (control/PPS), time of sacrifice (baseline vs 20 mins post social testing), sex and all two and three way interactions were fitted as factors, corticosterone level as response. Post-hoc analysis was performed only for within group comparisons. For all models, litter was nested within group and fitted as a random factor to account for the use of multiple animals per litter. Data were checked for normality and homogeneity of variance. Post-hoc t-tests were used when significant interactions were found. The most relevant statistics are reported below, please see for a full statistical summary.
Table 2. Full statistical report.
Results
mRNA – Hippocampal formation. FKBP5 (group*sex: F1,29.85 = 4.33, p = .04, ) and AVPR1a (group*sex: F1,26.64 = 4.47, p = .04, ) expression was significantly higher in the female hippocampal formation following PPS, whereas GR (group: F1,7.63 = 0.01, p = .92), MR (group: F1,9.44 = 0.86, p = .38), GR:MR (group: F1,8.06 = 0.88, p = .38), AVP (group: F1,10.93 = 0.62, p = .45), OXT (group: F1,9.56 = 0.26, p = .62) and OXTR (group: F1,9.84 = 0.02, p = .9) were unchanged in males and females.
Figure 1. Hippocampal formation. PPS increased expression of (a) FKBP5 and (b) AVPR1a in the female hippocampal formation. Con: control; PPS: pre-pubertal stress; F: female; M: male. Male: 12 control; 10 PPS; female: 8 control; 10 PPS. *p < .05. Error bars represent 1 S.E. and bars joined by a line and asterisk are significantly different to one another.
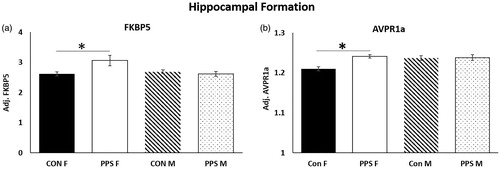
PFC. In the female PFC, PPS resulted in significantly higher GR expression (group*sex: F1,24.94 = 7.17, p = .01, ), a higher GR:MR ratio (group*sex: F1,25.37 = 4.97, p = .03, ) and reduced AVPR1a expression (group*sex: F1,23.17 = 6.94, p = .01, ) when compared to control females. MR (group: F1,8.81 = 0.14, p = .72), AVP (group: F1,9.77 = 0.87, p = .37), OXT (group: F1,10.34 = 0.17, p = .68), OXTR (group: F1,10.39 = 0.16, p = .7) and FKPB5 (group: F1,9.23 = 1.43, p = .26) were unchanged in male and female PFC following PPS, but OXTR expression was higher in females than males (sex: F1,27.9 = 28.65, p < .0001, .
Figure 2. PFC. PPS increased (a) GR and (b) GR:MR ratio and decreased (c) AVPR1a in the female PFC. OXTR expression was higher in female than male PFC. Con: control; PPS: pre-pubertal stress; F: female; M: male. Male: 12 control; 10 PPS; female: 8 control; 10 PPS. *p < .05, **p < .01, ***p < .0001. Error bars represent 1 S.E. and bars joined by a line and asterisk are significantly different to one another.
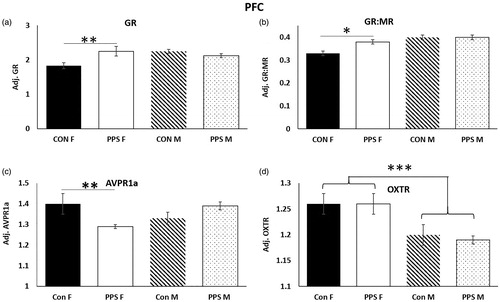
Hypothalamus. In the hypothalamus, GR (sex: F1,30.7 = 13.68, p < .001), MR (sex: F1,25.52 = 63.73, p < .0001), GR:MR (sex: F1,28.33 = 8.93, p < .01), FKBP5 (sex: F1,26.23 = 43.7, p < .0001) and OXTR (sex: F1,29.24 = 118.81, p < .0001) were lower in females than males regardless of treatment (), whereas AVPR1a (sex: F1,25.55 = 27.06, p < .0001) and OXT (sex: F1,26.76 = 42.06, p < .0001, ) were higher in females. There was no effect of PPS on GR (group: F1,10.79 = 0.08, p = .78), MR (group: F1,9 = 0.53, p = .49), GR:MR (group: F1,11.03 = 0.15, p = .71), AVP (group: F1,7.69 = 0.86, p = .38), AVPR1a (group: F1,10.42 = 0.04, p = .84), OXT (group: F1,10.33 = 0.4, p = .54), OXTR (group: F1,10.84 = 0.43, p = .53) or FKBP5 (group: F1,10.64 = 0.63, p = .44) expression. See for summary of regional gene expression changes.
Figure 3. Hypothalamus. (a) GR, (b) MR, (c) GR:MR, (d) FKBP5 and (e) OXTR were higher in male than female hypothalamus, whereas (f) AVPR1a and (g) OXT were higher in female hypothalamus. Con: control; PPS: pre-pubertal stress; F:, female; M: male. Male: 12 control; 10 PPS; female: 8 control; 10 PPS. **p < .01, ***p < .0001. Error bars represent 1 S.E. and bars joined by a line and asterisk are significantly different to one another.
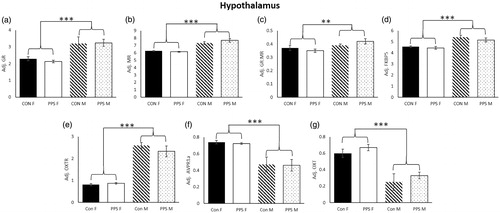
Table 3. Summary of gene expression and CORT changes as a result of PPS and sex differences.
Corticosterone. There was no effect of PPS on baseline expression of plasma corticosterone, but 20 minutes after a social interaction test PPS blunted the normal corticosterone rise in females (group*sex*time of sacrifice: F7,37.77 = 3.53, p = .0052, , ).
Figure 4. Corticosterone. Social interaction significantly elevated corticosterone above baseline in control animals and PPS males. This response was blunted in PPS females. Con: control; PPS: pre-pubertal stress; F: female; M: male. Females: 22 control; 18 PPS; male: 12 control; 10 PPS *p < .05. Error bars represent 1 S.E. and bars joined by a line and asterisk are significantly different to one another.
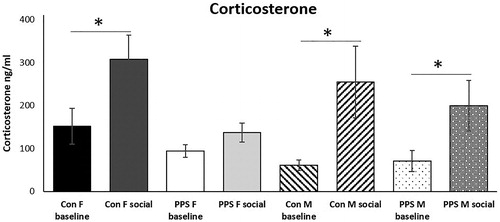
Discussion
PPS altered the expression of receptors and neuropeptides involved in HPA axis function in the hippocampal formation and PFC, but not hypothalamus of adult females, whereas in males the expression of major HPA axis components were unaffected in all brain regions studied. In the female prefrontal cortex, PPS increased GR and GR:MR receptor ratio and reduced AVPR1a expression. In the female hippocampal formation, PPS increased expression of FKBP5 and AVPR1a. In the periphery, females exposed to PPS did not show the normal rise in blood corticosterone levels following a social interaction test. We also found sex differences in baseline gene expression, particularly in the hypothalamus.
GR and MR are nuclear receptors/transcription factors which mediate the actions of glucocorticoid stress hormones, playing a key role in the stress response and also regulation of brain development and neuronal plasticity (Liston & Gan, Citation2011). These corticosteroid receptors (CR) act through delayed, long-lasting transcription-dependent mechanisms, but also exert more rapid effects which dampen the activated HPA axis in a negative feedback, transcription-independent manner (Gjerstad, Lightman, & Spiga, Citation2018; Tasker & Herman, Citation2011). Distributed throughout the brain, CR expression is highest in the limbic system (Herman, Citation1993). We found that PPS had no effect on GR or MR expression in male or female hippocampal formation. In contrast, stressors applied at earlier time points (e.g. prenatal stress and maternal separation) generally decrease hippocampal CR expression in males and females (although precise effects can vary depending on nature of the stress) (Aisa, Tordera, Lasheras, Del Rio, & Ramirez, Citation2008; Brunton & Russell, Citation2010; Kapoor, Dunn, Kostaki, Andrews, & Matthews, Citation2006; Levitt, Lindsay, Holmes, & Seckl, Citation1996; Maccari et al., Citation1995; Plotsky & Meaney, Citation1993; van Bodegom, Homberg, & Henckens, Citation2017; Welberg, Seckl, & Holmes, Citation2001). Stressors at later time points produce different effects, with chronic variable stress in adolescence decreasing GR in the male hippocampus, and increasing/decreasing MR in male/female hippocampus respectively (Isgor, Kabbaj, Akil, & Watson, Citation2004; Llorente et al., Citation2011). One study using mice found that PPS increased MR and decreased GR:MR ratio in the hippocampus of adult male and female animals (Brydges et al., Citation2014). Although PPS did not alter hippocampal CR expression directly in the present study, we did find evidence of altered CR activity following PPS in females through increased expression of FKBP5. FKBP5 is a co-chaperone of heat shock protein 90 (hsp90) which regulates GR sensitivity. Activation of GR leads to increased expression of FKBP5, creating an ultrashort negative feedback loop which inhibits GR signaling (Wochnik et al., Citation2005; Zannas, Wiechmann, Gassen, & Binder, Citation2016). Therefore, increased FKBP5 likely indicates increased GR activity. Indeed, increased expression of FKBP5 in the limbic system (hippocampus and amygdala) is associated with increased stress responsiveness (anxiety) and decreased stress coping behaviors, whereas experimental reduction of FKBP5 has opposite effects (Touma et al., Citation2011; Zannas et al., Citation2016).
The brain undergoes significant development postnatally, and the PFC is one of the last brain regions to mature, undergoing synaptic remodeling in childhood and adolescence (Barfield & Gourley, Citation2018). Therefore pre-pubertal and adolescent stress may be particularly detrimental for the PFC, yet little is known of the effects of early life stress (ELS) on CR expression in this region (Patel, Katz, Karssen, & Lyons, Citation2008). In agreement with a previous study, we found that PPS did not impact GR or MR expression in the male PFC (Fuentes, Carrasco, Armario, & Nadal, Citation2014). However, PPS did increase GR and GR:MR ratio in the female PFC. Stress at an earlier timepoint, between PND 7-14, increased GR expression in the PFC of female and male rats, again highlighting the importance of timing and sex (Alteba, Korem, & Akirav, Citation2016). We found no evidence of altered FKBP5 in the PFC following PPS, but prenatal stress and maternal separation in rats decreases FKBP5 expression in the male PFC with no effects in the hippocampus, whereas chronic unpredictable stress in adolescence increases FKBP5 in the male hippocampus, PFC and amygdala (Szymanska et al., Citation2009; van der Doelen et al., Citation2014; Xu et al., Citation2017, Citation2019). Overall our results suggest that PPS alters CR function in the adult hippocampus and PFC, and this effect is specific to females.
PPS did not impact baseline corticosterone in males or females in agreement with the majority of previous rodent research (Fuentes et al., Citation2014; Grigoryan, Ardi, Albrecht, Richter-Levin, & Segal, Citation2015; Jacobson-Pick & Richter-Levin, Citation2010). In humans, studies have found increased, decreased and no change in basal cortisol following ELS (Agorastos, Pervanidou, Chrousos, & Baker, Citation2019; Lupien et al., Citation2009). Differences are likely attributable to variation in the nature and timing of stress as well as genetics, factors which are rarely considered in human studies. In the present study, exposure to social interaction with a stranger resulted in elevated corticosterone in the plasma of control females and all males, but this response was blunted in females with experience of PPS. Note that the increases in corticosterone are not an acute response to systemic vehicle administration but are a result of the social interaction, since all animals, both baseline and socially experienced rats, received vehicle injections. Furthermore, the injection occurred sixty-five minutes before sacrifice, so any acute corticosterone rise resulting from this would no longer be detectable. In contrast to the results presented here, mild prenatal stress results in heightened corticosterone response to restraint stress in adult females (Aisa et al., Citation2008). Interestingly, more prolonged prenatal stress is necessary to induce the same effects in males (Gobinath, Mahmoud, & Galea, Citation2015). Maternal separation elevates or blunts male and female corticosterone responses to restraint stress, depending on the study (Desbonnet, Garrett, Daly, McDermott, & Dinan, Citation2008; Lehmann, Russig, Feldon, & Pryce, Citation2002; Roman, Gustafsson, Berg, & Nylander, Citation2006), and chronic variable adolescent stress between PND 45-58/37-49 blunted corticosterone responses to a stressor in adult females but not males (Bourke & Neigh, Citation2011; Wulsin, Wick-Carlson, Packard, Morano, & Herman, Citation2016). This again suggests that the female HPA axis is more sensitive to ELS, although specific outcomes are mediated by exact timing of stress and adult testing paradigm (e.g. social vs restraint). An adaptive stress response is characterized by a rapid corticosterone or cortisol (CORT) increase, followed by a progressive decline. Excessive or repeated activation of the HPA axis and release of CORT can lead to blunted CORT secretion in response to acute stress (Kinlein, Wilson, & Karatsoreos, Citation2015). A healthy CORT response is necessary for appropriate behavior and survival, therefore a blunted CORT response to acute stress may be considered a maladaptive phenotype.
PPS increased expression of AVPR1a in the female hippocampal formation and decreased it in the PFC. No changes were observed in the hypothalamus. OXT and AVP are closely related neuropeptides that exert opposite effects on the HPA axis. Stress results in the release of hypothalamic AVP, which stimulates the release of adrenocorticotrophic hormone from the pituitary and eventual production of CORT (de Kloet et al., Citation2005). In contrast, OXT dampens the HPA axis (Neumann & Landgraf, Citation2019). Both AVP and OXT exert effects on behavior through OXTR and AVPR1a/AVPR1b situated in the brain (Song & Albers, Citation2018). Effects of prenatal stress on AVP/OXT systems are mixed, with some studies finding decreased OXT/AVP expression in the male hypothalamus, others no changes in males or females (Desbonnet et al., Citation2008; Lee, Brady, Shapiro, Dorsa, & Koenig, Citation2007; Schmidt et al., Citation2018). Poor maternal care in rodents decreases OXT and OXTR expression centrally (hypothalamus and amygdala) and peripherally (blood plasma) in female animals (Francis, Young, Meaney, & Insel, Citation2002; Tobon, Jeffrey, & Nemeroff, Citation2018), whereas maternal separation increases hypothalamic AVP and alters OXT expression and OXT/AVP receptor binding in an age and sex-specific manner (Lukas, Bredewold, Neumann, & Veenema, Citation2010; Murgatroyd et al., Citation2009; Veenema, Bredewold, & Neumann, Citation2007; Veenema & Neumann, Citation2009). Our previous work found PPS increased protein levels of AVP in the supraoptic (but not paraventricular) nucleus of the hypothalamus and blood plasma in male and female rats (Brydges et al. Citationin Review). In the present study, the hypothalamus was analyzed as a whole, it is possible differences may have been found if the supraoptic and paraventricular nuclei had been analyzed separately. Alternatively, PPS may alter translation rather than transcription of AVP in this region. Considering their opposing effects on behavior (AVP exerts anxiogenic and depressive-like effects, whereas OXT is an endogenous anxiolytic), the balance of AVP and OXT in the brain is thought crucial for appropriate emotional behaviors (Mak, Broussard, Vacy, & Broadbear, Citation2012; Neumann & Landgraf, Citation2012). In the present study, we find altered AVPR1a expression in the female limbic system in the absence of altered OXT/OXTR expression. This indicates a dysregulated HPA axis which may predispose towards anxiety or depressive phenotypes following stress (Lesse, Rether, Groger, Braun, & Bock, Citation2017; Neumann & Slattery, Citation2016; Nowacka-Chmielewska, Kasprowska-Liśkiewicz, Barski, Obuchowicz, & Małecki, Citation2017).
PPS increased AVPR1a expression in the female hippocampal formation, yet decreased expression in the PFC. Bi-directional projections exist between the hippocampus and hypothalamus (production site of AVP): the hippocampus is a target of AVP and is capable of decreasing AVP expression in the hypothalamus (Nettles, Pesold, & Goldman, Citation2000; Zhang & Hernandez, Citation2013). PPS leads to increased AVP (Brydges et al. Citationunder review), therefore increased AVPR1a expression in the hippocampal formation may be a compensatory mechanism, enhancing the sensitivity to and subsequent inhibitory effects of the hippocampus on hypothalamic AVP secretion. The PFC is also thought to exert inhibitory effects over the hypothalamus, but direct connections between these two structures are lacking, and it is hypothesized that the PFC may act via other structures to exert this influence (Spencer, Buller, & Day, Citation2005). Whether the decreased AVPR1a expression following PPS in this region is due to adaptation or pathology remains to be elucidated.
Striking sex differences were seen regardless of PPS. GR, MR, GR:MR, FKBP5 and OXTR expression were significantly higher in male than female hypothalamus, whereas AVPR1a and OXT showed the opposite pattern. OXTR expression was higher in female PFC. These findings are consistent with previous studies finding AVPR1a expression is higher in female vs male rodents, and GR, MR and OXTR expression higher in the male hypothalamus (although species, age and region studied can all affect direction of difference) (Albers, Citation2015; Bale & Dorsa, Citation1995; Dumais, Bredewold, Mayer, & Veenema, Citation2013; Smith et al., Citation2017; Turner, Citation1990). One study investigating binding in 35 different rodent brains regions similarly found sex differences in OXTR and AVPR1a expression (increased/decreased depending on region) (Smith et al., Citation2017), but less is known about FKBP5. These sex differences may confer a natural heightened reactivity to stress in females which may underlie the greater vulnerability of the female HPA axis to ELS.
The balance between MR and GR functioning is thought crucial for appropriate HPA axis function, and dysregulation and imbalance between CR is suggested as a candidate mechanism underlying psychiatric disorders such as major depression, a disorder which has been repeatedly associated with hyperactive HPA axis function (de Kloet et al., Citation2005; Juruena et al., Citation2015; Oitzl, Champagne, van der Veen, & de Kloet, Citation2010). Polymorphisms associated with enhanced expression of FKBP5 following GR activation are overrepresented in major depression, bipolar and PTSD (Binder, Citation2009; Matosin et al., Citation2018). FKBP5 is implicated in a number psychiatric disorders, particularly in combination with early life stress (Wang, Shelton, & Dwivedi, Citation2018). In humans, there is an interaction between FKBP5 (FK506 binding protein 5) variability and childhood trauma on psychosis, paranoia, social stress appraisal and prefrontal cortex function (Harms et al., Citation2017; Misiak et al., Citation2018; Wang et al., Citation2018). Our results suggest that PPS plays a role in altered FKBP5 functioning in females, a key regulator of the HPA axis. Also in agreement with our findings, the human literature shows early trauma is associated with blunted CORT responses to social stimuli, particularly in women.
Conclusions
We found the adult female HPA axis was sensitive to PPS, with changes seen throughout the system. This highlights the pre-pubertal phase as a particularly sensitive time for re-programming of the female HPA axis by stress. In contrast, the male HPA axis was unaffected. This sex-specific vulnerability may underlie the greater propensity for women to develop psychiatric disorders including depression, anxiety and PTSD, disorders which are frequently associated with HPA axis dysregulation. Although we found greater effects in females in the present study, males are not immune to the effects of PPS. For example, in previous studies we found that PPS significantly impaired hippocampal-dependent behavior and hippocampal neurogenesis in males but not females, and social behavior is equally affected in both sexes (Brydges et al., Citation2018, Brydges et al. Citationin Review). Furthermore, others have found several behavioral and neurobiological effects of PPS in male animals (Albrecht et al., Citation2017; Brydges, Citation2016). This suggests males and females differ in their responses to PPS, potentially resulting in sex-specific vulnerabilities to certain disorders. This strengthens the argument for including both sexes in preclinical and clinical studies.
Disclosure statement
The authors declare no competing interest.
Additional information
Funding
Notes on contributors
Nichola M. Brydges
NMB & KLT designed the experiments and wrote the manuscript, NMB & CB performed the experiments and analysed the data.
Kerrie L. Thomas
NMB & KLT designed the experiments and wrote the manuscript, NMB & CB performed the experiments and analysed the data.
References
- Agorastos, A., Pervanidou, P., Chrousos, G.P., & Baker, D.G. (2019). Developmental trajectories of early life stress and trauma: A narrative review on neurobiological aspects beyond stress system dysregulation. Frontiers in Psychiatry, 10, 1–25. doi:10.3389/fpsyt.2019.00118
- Aisa, B., Tordera, R., Lasheras, B., Del Rio, J., & Ramirez, M.J. (2008). Effects of maternal separation on hypothalamic-pituitary-adrenal responses, cognition and vulnerability to stress in adult female rats. Neuroscience, 154, 1218–1226. doi:10.1016/j.neuroscience.2008.05.011
- Albers, H.E. (2015). Species, sex and individual differences in the vasotocin/vasopressin system: Relationship to neurochemical signaling in the social behavior neural network. Frontiers in Neuroendocrinology, 36, 49–71. doi:10.1016/j.yfrne.2014.07.001
- Albrecht, A., Müller, I., Ardi, Z., Çalışkan, G., Gruber, D., Ivens, S., … Richter-Levin, G. (2017). Neurobiological consequences of juvenile stress: A GABAergic perspective on risk and resilience. Neuroscience & Biobehavioral Reviews, 74, 21–43. doi:10.1016/j.neubiorev.2017.01.005
- Alteba, S., Korem, N., & Akirav, I. (2016). Cannabinoids reverse the effects of early stress on neurocognitive performance in adulthood. Learning & Memory, 23, 349–358. doi:10.1101/lm.041608.116
- Bale, T.L., & Dorsa, D.M. (1995). Sex-differences in and effects of estrogen on oxytocin receptor messenger-ribonucleic-acid expression in the ventromedial hypothalmaus. S. Endocrinology, 136, 27–32. doi:10.1210/en.136.1.27
- Barfield, E.T., & Gourley, S.L. (2018). Prefrontal cortical trkB, glucocorticoids, and their interactions in stress and developmental contexts. Neuroscience & Biobehavioral Reviews, 95, 535–558. doi:10.1016/j.neubiorev.2018.10.015
- Binder, E.B. (2009). The role of FKBP5, a co-chaperone of the glucocorticoid receptor in the pathogenesis and therapy of affective and anxiety disorders. Psychoneuroendocrinology, 34, S186–S195. doi:10.1016/j.psyneuen.2009.05.021
- Bourke, C.H., & Neigh, G.N. (2011). Behavioral effects of chronic adolescent stress are sustained and sexually dimorphic. Hormones and Behavior, 60, 112–120. doi:10.1016/j.yhbeh.2011.03.011
- Brunton, P.J., & Russell, J.A. (2010). Prenatal social stress in the rat programmes neuroendocrine and behavioural responses to stress in the adult offspring: Sex-specific effects. Journal of Neuroendocrinology, 22, 258–271. doi:10.1111/j.1365-2826.2010.01969.x
- Brydges , et al. in review. Brydges, N.M., Hall, J., Best, C., Rule, L, Watkin, H., Drake, A.J., Lewis, C., Thomas, K.L. & Hall, J. Early life stress impairs social function through AVP-dependent mechanisms.
- Brydges, N.M. (2016). Pre-pubertal stress and brain development in rodents. Current Opinion in Behavioral Sciences, 7, 8–14. doi:10.1016/j.cobeha.2015.08.003
- Brydges, N.M., Jin, R.W., Seckl, J., Holmes, M.C., Drake, A.J., & Hall, J. (2014). Juvenile stress enhances anxiety and alters corticosteroid receptor expression in adulthood. Brain and Behavior, 4, 4–13. doi:10.1002/brb3.182
- Brydges, N.M., Moon, A., Rule, L., Watkin, H., Thomas, K.L., & Hall, J. (2018). Sex specific effects of pre-pubertal stress on hippocampal neurogenesis and behaviour. Translational Psychiatry, 8, 1–12. doi:10.1038/s41398-018-0322-4
- Bunea, I.M., Szentágotai-Tătar, A., & Miu, A.C. (2017). Early-life adversity and cortisol response to social stress: A meta-analysis. Translational Psychiatry, 7, 1–8. doi:10.1038/s41398-017-0032-3
- Christiansen, D.M., & Hansen, M. (2015). Accounting for sex differences in PTSD: A multi-variable mediation model. European Journal of Psychotraumatology, 6, 26068. doi:10.3402/ejpt.v6.26068
- de Kloet, E.R., Joels, M., & Holsboer, F. (2005). Stress and the brain: From adaptation to disease. Nature Reviews Neuroscience, 6, 463–475. doi:10.1038/nrn1683
- Desbonnet, L., Garrett, L., Daly, E., McDermott, K.W., & Dinan, T.G. (2008). Sexually dimorphic effects of maternal separation stress on corticotrophin-releasing factor and vasopressin systems in the adult rat brain. International Journal of Developmental Neuroscience, 26, 259–268. doi:10.1016/j.ijdevneu.2008.02.004
- Dumais, K.M., Bredewold, R., Mayer, T.E., & Veenema, A.H. (2013). Sex differences in oxytocin receptor binding in forebrain regions: Correlations with social interest in brain region- and sex- specific ways. Hormones and Behavior, 64, 693–701. doi:10.1016/j.yhbeh.2013.08.012
- Francis, D.D., Young, L.J., Meaney, M.J., & Insel, T.R. (2002). Naturally occurring differences in maternal care are associated with the expression of oxytocin and vasopressin (V1a) receptors: Gender differences. Journal of Neuroendocrinology, 14, 349–353. doi:10.1046/j.0007-1331.2002.00776.x
- Fuentes, S., Carrasco, J., Armario, A., & Nadal, R. (2014). Behavioral and neuroendocrine consequences of juvenile stress combined with adult immobilization in male rats. Hormones and Behavior, 66, 475–486. doi:10.1016/j.yhbeh.2014.07.003
- Gjerstad, J.K., Lightman, S.L., & Spiga, F. (2018). Role of glucocorticoid negative feedback in the regulation of HPA axis pulsatility. Stress, 21, 403–416. doi:10.1080/10253890.2018.1470238
- Gobinath, A.R., Mahmoud, R., & Galea, L.A.M. (2015). Influence of sex and stress exposure across the lifespan on endophenotypes of depression: Focus on behavior glucocorticoids, and hippocampus. Frontiers in Neuroscience, 8, 1–18. doi:10.3389/fnins.2014.00420
- Grigoryan, G., Ardi, Z., Albrecht, A., Richter-Levin, G., & Segal, M. (2015). Juvenile stress alters LTP in ventral hippocampal slices: Involvement of noradrenergic mechanisms. Behavioural Brain Research, 278, 559–562. doi:10.1016/j.bbr.2014.09.047
- Harms, M.B., Birn, R., Provencal, N., Wiechmann, T., Binder, E.B., Giakas, S.W., … Pollak, S.D. (2017). Early life stress, FK506 binding protein 5 gene (FKBP5) methylation, and inhibition-related prefrontal function: A prospective longitudinal study. Development and Psychopathology, 29, 1895–1903. doi:10.1017/S095457941700147X
- Heck, A.L., & Handa, R.J. (2019). Sex differences in the hypothalamic-pituitary-adrenal axis' response to stress: An important role for gonadal hormones. Neuropsychopharmacology, 44, 45–58. doi:10.1038/s41386-018-0167-9
- Heim, C., & Nemeroff, C.B. (2001). The role of childhood trauma in the neurobiology of mood and anxiety disorders: Preclinical and clinical studies. Biological Psychiatry, 49, 1023–1039. doi:10.1016/S0006-3223(01)01157-X
- Herman, J.P. (1993). Regulation of adrenocorticosteroid receptor messenger-RNA expression in the central nervous system. Cellular and Molecular Neurobiology, 13, 349–372. doi:10.1007/BF00711577
- Isgor, C., Kabbaj, M., Akil, H., & Watson, S.J. (2004). Delayed effects of chronic variable stress during peripubertal-juvenile period on hippocampal morphology and on cognitive and stress axis functions in rats. Hippocampus, 14, 636–648. doi:10.1002/hipo.10207
- Jacobson-Pick, S., & Richter-Levin, G. (2010). Differential impact of juvenile stress and corticosterone in juvenility and in adulthood, in male and female rats. Behavioural Brain Research, 214, 268–276. doi:10.1016/j.bbr.2010.05.036
- Juruena, M.F., Baes, C.V., Menezes, I.C., & Graeff, F.G. (2015). Early life stress in depressive patients: Role of glucocorticoid and mineralocorticoid receptors and of hypothalamic-pituitary-adrenal axis activity. Current Pharmaceutical Design, 21, 1369–1378. doi:10.2174/1381612821666150105125500
- Juruena, M.F., Cleare, A.J., & Young, A.H. (2018). The role of early life stress in HPA axis and depression. In Understanding depression (pp. 71–80). Amsterdam, Netherland: Springer.
- Kapoor, A., Dunn, E., Kostaki, A., Andrews, M.H., & Matthews, S.G. (2006). Fetal programming of hypothalamo-pituitary-adrenal function: Prenatal stress and glucocorticoids. The Journal of Physiology, 572, 31–44. doi:10.1113/jphysiol.2006.105254
- Kessler, R.C., Berglund, P., Demler, O., Jin, R., Koretz, D., Merikangas, K.R., … Wang, P.S. (2003). The epidemiology of major depressive disorder – Results from the National Comorbidity Survey Replication (NCS-R). JAMA, 289, 3095–3105. doi:10.1001/jama.289.23.3095
- Kessler, R.C., Chiu, W.T., Demler, O., Merikangas, K.R., & Walters, E.E. (2005). Prevalence, severity, and comorbidity of 12-month DSM-IV disorders in the national comorbidity survey replication (vol 62, pg 617, 2005). Archives of General Psychiatry, 62, 709–709. doi:10.1001/archpsyc.62.6.617
- Kessler, R.C., McGonagle, K.A., Swartz, M., Blazer, D.G., & Nelson, C.B. (1993). Sex and depression in the national comorbidity survey.1. lifetime prevelance, chronicity and recurrence. Journal of Affective Disorders, 29, 85–96. doi:10.1016/0165-0327(93)90026-G
- Kinlein, S.A., Wilson, C.D., & Karatsoreos, I.N. (2015). Dysregulated hypothalamic-pituitary-adrenal axis function contributes to altered endocrine and neurobehavioral responses to acute stress. Frontiers in Psychiatry, 6, 1–9. doi:10.3389/fpsyt.2015.00031
- Lee, P.R., Brady, D.L., Shapiro, R.A., Dorsa, D.M., & Koenig, J.I. (2007). Prenatal stress generates deficits in rat social behavior: Reversal by oxytocin. Brain Research, 1156, 152–167. doi:10.1016/j.brainres.2007.04.042
- Lehmann, J., Russig, H., Feldon, J., & Pryce, C.R. (2002). Effect of a single maternal separation at different pup ages on the corticosterone stress response in adult and aged rats. Pharmacology Biochemistry and Behavior, 73, 141–145. doi:10.1016/S0091-3057(02)00788-8
- Lesse, A., Rether, K., Groger, N., Braun, K., & Bock, J. (2017). Chronic postnatal stress induces depressive-like behavior in male mice and programs second-hit stress-induced gene expression patterns of OxtR and AvpR1a in adulthood. Molecular Neurobiology, 54, 4813–4819. doi:10.1007/s12035-016-0043-8
- Levitt, N.S., Lindsay, R.S., Holmes, M.C., & Seckl, J.R. (1996). Dexamethasone in the last week of pregnancy attenuates hippocampal glucocorticoid receptor gene expression and elevates blood pressure in the adult offspring in the rat. Neuroendocrinology, 64, 412–418. doi:10.1159/000127146
- Liston, C., & Gan, W.B. (2011). Glucocorticoids are critical regulators of dendritic spine development and plasticity in vivo. Proceedings of the National Academy of Sciences of Sciences, 108, 16074–16079. doi:10.1073/pnas.1110444108
- Llorente, R., Miguel-Blanco, C., Aisa, B., Lachize, S., Borcel, E., Meijer, O.C., … Viveros, M.P. (2011). Long term sex-dependent psychoneuroendocrine effects of maternal deprivation and juvenile unpredictable stress in rats. Journal of Neuroendocrinology, 23, 329–344. doi:10.1111/j.1365-2826.2011.02109.x
- Lukas, M., Bredewold, R., Neumann, I.D., & Veenema, A.H. (2010). Maternal separation interferes with developmental changes in brain vasopressin and oxytocin receptor binding in male rats. Neuropharmacology, 58, 78–87. doi:10.1016/j.neuropharm.2009.06.020
- Lupien, S.J., McEwen, B.S., Gunnar, M.R., & Heim, C. (2009). Effects of stress throughout the lifespan on the brain, behaviour and cognition. Nature Reviews Neuroscience, 10, 434–445. doi:10.1038/nrn2639
- Maccari, S., Piazza, P.V., Kabbaj, M., Barbazanges, A., Simon, H., & Le Moal, M. (1995). Adoption reverses the long-term impairment in glucocorticoid feedback induced by prenatal stress. The Journal of Neuroscience, 15, 110–116. doi:10.1523/JNEUROSCI.15-01-00110.1995
- Mak, P., Broussard, C., Vacy, K., & Broadbear, J.H. (2012). Modulation of anxiety behavior in the elevated plus maze using peptidic oxytocin and vasopressin receptor ligands in the rat. Journal of Psychopharmacology, 26, 532–542. doi:10.1177/0269881111416687
- Matosin, N., Halldorsdottir, T., & Binder, E.B. (2018). Understanding the molecular mechanisms underpinning gene by environment interactions in psychiatric disorders: The FKBP5 Model. Biological Psychiatry, 83, 821–830. doi:10.1016/j.biopsych.2018.01.021
- McLaughlin, K.A., Conron, K.J., Koenen, K.C., & Gilman, S.E. (2010). Childhood adversity, adult stressful life events, and risk of past-year psychiatric disorder: A test of the stress sensitization hypothesis in a population-based sample of adults. Psychological Medicine, 40, 1647–1658. doi:10.1017/S0033291709992121
- Misiak, B., Stramecki, F., Gawęda, Ł., Prochwicz, K., Sąsiadek, M.M., Moustafa, A.A., & Frydecka, D. (2018). Interactions between variation in candidate genes and environmental factors in the etiology of schizophrenia and bipolar disorder: A systematic review. Molecular Neurobiology, 55, 5075–5100. doi:10.1007/s12035-017-0708-y
- Murgatroyd, C., Patchev, A.V., Wu, Y., Micale, V., Bockmühl, Y., Fischer, D., … Spengler, D. (2009). Dynamic DNA methylation programs persistent adverse effects of early-life stress. Nature Neuroscience, 12, 1559–U1108. doi:10.1038/nn.2436
- Murri, M.B., Prestia, D., Mondelli, V., Pariante, C., Patti, S., Olivieri, B., … Amore, M. (2016). The HPA axis in bipolar disorder: Systematic review and meta-analysis. Psychoneuroendocrinology, 63, 327–342. doi:10.1016/j.psyneuen.2015.10.014
- Nettles, K.W., Pesold, C., & Goldman, M.B. (2000). Influence of the ventral hippocampal formation on plasma vasopressin, hypothalamic-pituitary-adrenal axis, and behavioral responses to novel acoustic stress. Brain Research, 858, 181–190. doi:10.1016/S0006-8993(99)02281-7
- Neumann, I.D., & Landgraf, R. (2012). Balance of brain oxytocin and vasopressin: Implications for anxiety, depression, and social behaviors. Trends in Neurosciences, 35, 649–659. doi:10.1016/j.tins.2012.08.004
- Neumann, I.D., & Landgraf, R. (2019). Tracking oxytocin functions in the rodent brain during the last 30 years: From push-pull perfusion to chemogenetic silencing. Journal of Neuroendocrinology, 31, e12695. doi:10.1111/jne.12695
- Neumann, I.D., & Slattery, D.A. (2016). Oxytocin in general anxiety and social fear: A translational approach. Biological Psychiatry, 79, 213–221. doi:10.1016/j.biopsych.2015.06.004
- Nicol, K., Pope, M., Romaniuk, L., & Hall, J. (2015). Childhood trauma, midbrain activation and psychotic symptoms in borderline personality disorder. Translational Psychiatry, 5, e559. doi:10.1038/tp.2015.53
- Nowacka-Chmielewska, M.M., Kasprowska-Liśkiewicz, D., Barski, J.J., Obuchowicz, E., & Małecki, A. (2017). The behavioral and molecular evaluation of effects of social instability stress as a model of stress-related disorders in adult female rats. Stress, 20, 549–561. doi:10.1080/10253890.2017.1376185
- Oitzl, M.S., Champagne, D.L., van der Veen, R., & de Kloet, E.R. (2010). Brain development under stress: Hypotheses of glucocorticoid actions revisited. Neuroscience & Biobehavioral Reviews, 34, 853–866. doi:10.1016/j.neubiorev.2009.07.006
- Palmier-Claus, J., Berry, K., Darrell-Berry, H., Emsley, R., Parker, S., Drake, R., & Bucci, S. (2016). Childhood adversity and social functioning in psychosis: Exploring clinical and cognitive mediators. Psychiatry Research, 238, 25–32. doi:10.1016/j.psychres.2016.02.004
- Patel, P.D., Katz, M., Karssen, A.M., & Lyons, D.M. (2008). Stress-induced changes in corticosteroid receptor expression in primate hippocampus and prefrontal cortex. Psychoneuroendocrinology, 33, 360–367. doi:10.1016/j.psyneuen.2007.12.003
- Plotsky, P.M., & Meaney, M.J. (1993). Early postnatal experience alters hypothalamic corticotropin-releasing factor (CRF) messenger-RNA, median eminence CRF content and stress-indiced release in adult rats. Molecular Brain Research, 18, 195–200. doi:10.1016/0169-328X(93)90189-V
- Remes, O., Brayne, C., van der Linde, R., & Lafortune, L. (2016). A systematic review of reviews on the prevalence of anxiety disorders in adult populations. Brain and Behavior, 6, e00497. doi:10.1002/brb3.497
- Roman, E., Gustafsson, L., Berg, M., & Nylander, I. (2006). Behavioral profiles and stress-induced corticosteroid secretion in male Wistar rats subjected to short and prolonged periods of maternal separation. Hormones and Behavior, 50, 736–747. doi:10.1016/j.yhbeh.2006.06.016
- Sandi, C., & Haller, J. (2015). Stress and the social brain: Behavioural effects and neurobiological mechanisms. Nature Reviews Neuroscience, 16, 290–304. doi:10.1038/nrn3918
- Schmidt, M., Braun, K., Brandwein, C., Rossetti, A.C., Guara Ciurana, S., Riva, M.A., … Gröger, N. (2018). Maternal stress during pregnancy induces depressive-like behavior only in female offspring and correlates to their hippocampal Avp and Oxt receptor expression. Behavioural Brain Research, 353, 1–10. doi:10.1016/j.bbr.2018.06.027
- Schroeder, A., Notaras, M., Du, X., & Hill, R.A. (2018). On the developmental timing of stress: Delineating sex-specific effects of stress across development on adult behavior. Brain Sciences, 8, 1–29. doi:10.3390/brainsci8070121
- Seale, J.V., Wood, S.A., Atkinson, H.C., Bate, E., Lightman, S.L., Ingram, C.D., … Harbuz, M.S. (2004). Gonadectomy reverses the sexually diergic patterns of circadian and stress-induced hypothalamic-pituitary-adrenal axis activity in male and female rats. Journal of Neuroendocrinology, 16, 516–524. doi:10.1111/j.1365-2826.2004.01195.x
- Smith, C.J.W., Poehlmann, M.L., Li, S., Ratnaseelan, A.M., Bredewold, R., & Veenema, A.H. (2017). Age and sex differences in oxytocin and vasopressin V1a receptor binding densities in the rat brain: Focus on the social decision-making network. Brain Structure and Function, 222, 981–1006. doi:10.1007/s00429-016-1260-7
- Song, Z.M., & Albers, H.E. (2018). Cross-talk among oxytocin and arginine-vasopressin receptors: Relevance for basic and clinical studies of the brain and periphery. Frontiers in Neuroendocrinology, 51, 14–24. doi:10.1016/j.yfrne.2017.10.004
- Spencer, S.J., Buller, K.M., & Day, T.A. (2005). Medial prefrontal cortex control of the Paraventricular hypothalamic nucleus response to psychological stress: Possible role of the bed nucleus of the stria terminalis. The Journal of Comparative Neurology, 481, 363–376. doi:10.1002/cne.20376
- Szymanska, M., Budziszewska, B., Jaworska-Feil, L., Basta-Kaim, A., Kubera, M., Leskiewicz, M., … Lason, W. (2009). The effect of antidepressant drugs on the HPA axis activity, glucocorticoid receptor level and FKBP51 concentration in prenatally stressed rats. Psychoneuroendocrinology, 34, 822–832. doi:10.1016/j.psyneuen.2008.12.012
- Tasker, J.G., & Herman, J.P. (2011). Mechanisms of rapid glucocorticoid feedback inhibition of the hypothalamic–pituitary–adrenal axis. Stress, 14, 398–406. doi:10.3109/10253890.2011.586446
- Teicher, M.H., & Samson, J.A. (2016). Annual research review: Enduring neurobiological effects of childhood abuse and neglect. Journal of Child Psychology and Psychiatry, 57, 241–266. doi:10.1111/jcpp.12507
- Teicher, M.H., Samson, J.A., Anderson, C.M., & Ohashi, K. (2016). The effects of childhood maltreatment on brain structure, function and connectivity. Nature Reviews Neuroscience, 17, 652. doi:10.1038/nrn.2016.111
- Tiwari, A., & Gonzalez, A. (2018). Biological alterations affecting risk of adult psychopathology following childhood trauma: A review of sex differences. Clinical Psychology Review, 66, 69–79. doi:10.1016/j.cpr.2018.01.006
- Tobon, A., Jeffrey, N., & Nemeroff, C.B. (2018). The role of oxytocin in early life adversity and later psychopathology: A review of preclinical and clinical studies. Current Treatment Options in Psychiatry, 5, 401–415. doi:10.1007/s40501-018-0158-9
- Touma, C., Gassen, N.C., Herrmann, L., Cheung-Flynn, J., Büll, D.R., Ionescu, I.A., … Rein, T. (2011). FK506 binding protein 5 shapes stress responsiveness: Modulation of neuroendocrine reactivity and coping behavior. Biological Psychiatry, 70, 928–936. doi:10.1016/j.biopsych.2011.07.023
- Turner, B.B. (1990). Sex difference in glucocorticoid binding in rat pituitary is estrogen dependent. Life Sciences, 46, 1399–1406. doi:10.1016/0024-3205(90)90340-W
- van Bodegom, M., Homberg, J.R., & Henckens, M. (2017). Modulation of the hypothalamic-pituitary-adrenal axis by early life stress exposure. Frontiers in Cellular Neuroscience, 11, 33. doi:10.3389/fncel.2017.00087
- van der Doelen, R.H.A., Calabrese, F., Guidotti, G., Geenen, B., Riva, M.A., Kozicz, T., & Homberg, J.R. (2014). Early life stress and serotonin transporter gene variation interact to affect the transcription of the glucocorticoid and mineralocorticoid receptors, and the co-chaperone FKBP5, in the adult rat brain. Frontiers in Behavioral Neuroscience, 8, 1–11. doi:10.3389/fnbeh.2014.00355
- Veenema, A.H., Bredewold, R., & Neumann, I.D. (2007). Opposite effects of maternal separation on intermale and maternal aggression in C57BL/6 mice: Link to hypothalamic vasopressin and oxytocin immunoreactivity. Psychoneuroendocrinology, 32, 437–450. doi:10.1016/j.psyneuen.2007.02.008
- Veenema, A.H., & Neumann, I.D. (2009). Maternal separation enhances offensive play-fighting, basal corticosterone and hypothalamic vasopressin mRNA expression in juvenile mate rats. Psychoneuroendocrinology, 34, 463–467. doi:10.1016/j.psyneuen.2008.10.017
- Wang, Q.Z., Shelton, R.C., & Dwivedi, Y. (2018). Interaction between early-life stress and FKBP5 gene variants in major depressive disorder and post-traumatic stress disorder: A systematic review and meta-analysis. Journal of Affective Disorders, 225, 422–428. doi:10.1016/j.jad.2017.08.066
- Welberg, L.A.M., Seckl, J.R., & Holmes, M.C. (2001). Prenatal glucocorticoid programming of brain corticosteroid receptors and corticotrophin-releasing hormone: Possible implications for behaviour. Neuroscience, 104, 71–79. doi:10.1016/S0306-4522(01)00065-3
- Wochnik, G.M., Ruegg, J., Abel, G.A., Schmidt, U., Holsboer, F., & Rein, T. (2005). FK506-binding proteins 51 and 52 differentially regulate dynein interaction and nuclear translocation of the glucocorticoid receptor in mammalian cells. Journal of Biological Chemistry, 280, 4609–4616. doi:10.1074/jbc.M407498200
- Wulsin, A.C., Wick-Carlson, D., Packard, B.A., Morano, R., & Herman, J.P. (2016). Adolescent chronic stress causes hypothalamo-pituitary-adrenocortical hypo-reponsiveness and depression-like behavior in adult female rats. Psychoneuroendocrinology, 65, 109–117. doi:10.1016/j.psyneuen.2015.12.004
- Xu, J.J., Wang, R., Liu, Y., Liu, D.X., Jiang, H., & Pan, F. (2017). FKBP5 and specific microRNAs via glucocorticoid receptor in the basolateral amygdala involved in the susceptibility to depressive disorder in early adolescent stressed rats. Journal of Psychiatric Research, 95, 102–113. doi:10.1016/j.jpsychires.2017.08.010
- Xu, J.J., Wang, R., Liu, Y., Wang, W., Liu, D.X., Jiang, H., & Pan, F. (2019). Short- and long-term alterations of FKBP5-GR and specific microRNAs in the prefrontal cortex and hippocampus of male rats induced by adolescent stress contribute to depression susceptibility. Psychoneuroendocrinology, 101, 204–215. doi:10.1016/j.psyneuen.2018.11.008
- Zannas, A.S., Wiechmann, T., Gassen, N.C., & Binder, E.B. (2016). Gene-stress-epigenetic regulation of FKBP5: Clinical and translational implications. Neuropsychopharmacology, 41, 261–274. doi:10.1038/npp.2015.235
- Zhang, L., & Hernandez, V.S. (2013). Synaptic innervation to rat hippocampus by vasopressin-immuno-positive fibres from the hypothalmaic supraoptic and paraventricular nuclei. Neuroscience, 228, 139–162. doi:10.1016/j.neuroscience.2012.10.010
- Zorn, J.V., Schur, R.R., Boks, M.P., Kahn, R.S., Joels, M., & Vinkers, C.H. (2017). Cortisol stress reactivity across psychiatric disorders: A systematic review and meta-analysis. Psychoneuroendocrinology, 77, 25–36. doi:10.1016/j.psyneuen.2016.11.036