Abstract
Over the years, it has become increasingly clear that males and females respond differently towards environmental stressors, highlighting the importance of including both sexes when studying the effects of stress. This study aims to provide further insight into the detailed consequences of exposing female mice to 21 days of chronic social defeat stress (CSDS). We used a protocol that relies on the ability of odorants and pheromones in male urine to trigger male mouse aggressive behavior. Collected male C57Bl/6n urine was applied to female C57Bl/6n mice who were then attacked by a novel male CD1 mouse each day according to the CDSD protocol. Control females were pair-housed and handled daily. Physiological, neuroendocrine and behavioral changes were evaluated during the experiment. CSDS exposure resulted in number of physiological changes, such as body weight gain, enlarged adrenals and reduced thymus weight, exaggerated HPA-axis negative feedback and increased anxiety-like behavior. However, no generalized social avoidance behavior was observed. This study provides important insights in the physiological, neuroendocrine and behavioral responses of female mice to CSDS, which are partially dependent on estrous cycle stage. This protocol will allow direct comparison of male and female responses to CSDS and enable sex-specific study of mechanisms underlying individual stress resilience.
In this study we found that there are differences in the way that female and male mice respond towards chronic social stress conditions when it comes to behavior and hormonal changes.
Lay summary
Introduction
Over the past few decades, mental health disorders have become a wide-spread health concern, and a leading cause of disability world-wide (World Health Organisation, Citation2020). The risk for developing a mental health disorder is dependent on the interaction between underlying genetic predispositions and environmental factors (Ron de Kloet et al., Citation2005). One of the main environmental factors modulating disease vulnerability is chronic exposure to stressors over the life time.
It is well known that stress-related disorders, such as anxiety disorders and major depressive disorder, are more common in women than men (Gutiérrez-Lobos et al., Citation2002; Kessler, Citation1994; WHO International Consortium in Psychiatric Epidemiology Citation2000) In addition, male and female responses to stress exposure differ critically on several dimensions, which has become particularly evident from a large number of rodent studies (Dalla et al., Citation2005; Galea et al., Citation1997; Hodes et al., Citation2015; Sachs et al., Citation2014; Westenbroek et al., Citation2004). Findings of these differential stress responses argue the importance of including both sexes when it comes to studying the effects of stress.
Nonetheless, for a long-time, comparison of male and female mice has been difficult in experimental chronic stress conditions, especially with regard to social stressors. Application of one of the most widely used chronic stress paradigms in mice, chronic social defeat stress (CSDS), strongly relies on aggressive behavior (Kudryavtseva et al., Citation1991; Russo & Nestler, Citation2013). In CSDS, male mice attack male intruders with a high likelihood, however, such aggressive behavior towards female mice is usually absent. Furthermore, female-to-female aggression is less likely to occur under standard laboratory conditions making it challenging to apply such CSDS protocols in females. Efforts to replicate effects of CSDS in female rodents were for a long time hampered by unavailable or particularly complex methods (Bourke & Neigh, Citation2012; Haller et al., Citation1999; Takahashi et al., Citation2017). In the past few years there have been attempts to design a hands-on protocol in which social defeat of females is achieved either using male-to-female or female-to-female aggression (Harris et al., Citation2018; Logan, Citation2019; Newman et al., Citation2019). One of these attainable CSDS models was recently described by Harris et al. This CSDS model relies on the odorants and pheromones in male urine to increase male mouse aggressive behavior towards female mice and has proved to be successful in establishing a stress phenotype (Harris et al., Citation2018).
The aim of the current study was to reproduce and extend the findings of the model described by Harris and colleagues, but with an adjustment to the length of the chronic defeat to 21 days, as a longer defeat period is also commonly used in many male CSDS studies (Haenisch et al., Citation2009; Wagner et al., Citation2011). This has previously been demonstrated to result in robust depression-like phenotypes in male mice (Kudryavtseva, Citation2000; Kudryavtseva et al., Citation1991) and offers better possibilities for pharmacological interventions (Gassen et al., Citation2014). This study provides additional insights on the different physiological, neuroendocrine and behavioral responses towards CSDS stress in females. The establishment of a CSDS protocol in female mice will allow researchers to test gene × environment interactions in both sexes. Ultimately, this will lead to increased knowledge on the differential sex effects in stress resilience mechanisms.
Methods
Animals and housing conditions
C57Bl/6n mice were bred in an in-house colony of the Max Planck Institute of Psychiatry (Munich, Germany) and used as experimental animals (females, 12 weeks old) or for the training of resident mice and urine sampling (males). Experimental animals were pair-housed in standard individually ventilated caging (IVC) system cages at least 2 weeks prior to the start of the experiment. In addition, CD1 mice (purchased from Janvier Labs, Germany) were used as residents (males, 12 weeks old, single-housed before the experiment) or social interaction partner (females, 6 weeks old, group-housed). CD1 males were allowed to habituate to the social defeat cage for two weeks before the start of the experiment.
Housing and experiments were performed under a 12 h light, 12 h dark cycle (lights on at 07.00 a.m.), constant temperature (23 ± 2 °C, humidity 55%) conditions and mice had access to food (Altromin 1324, Altromin GmbH, Germany) and water ad libitum.
Experiments were performed in accordance with the European Communities Council Directive 2010/63/EU. At all times efforts were made to minimize animal suffering during the course of the experiment. The protocols were approved by the committee for the Care and Use of Laboratory Animals of the Government of Upper Bavaria, Germany.
Experimental design
The 24 female C57Bl/6n mice were randomly divided into two groups (n = 12 CSDS; n = 12 control). The CSDS group was exposed to 21 consecutive days of the CSDS paradigm; control animals were handled daily. From day 18 until day 21 of the experimental period, behavior of CSDS and control animals was evaluated using a number of behavioral tasks assessing locomotor activity, anxiety-like behavior, social avoidance (SA) behavior and coping strategy selection (). One day after the last defeat all animals were sacrificed under basal conditions. A second cohort of female mice (C57Bl/6n, n = 11) was subjected to the same CSDS paradigm for a better quantification of the aggressive encounters during the CSDS exposure.
Figure 1. Female C57Bl/n mice are socially defeated by a CD1 male mouse over a period of 21 days when covered in male C57Bl/6n urine. (A) Over the course of 21 days female C57Bl/6n mice were exposed to a social chronic defeat stress (CSDS) paradigm. In this CSDS paradigm female mice were covered in male C57Bl/6n urine after which they were immediately exposed to a social defeat of 5 min by a CD1 male mice, subsequently separated by a transparent divider and housed together with the CD1 male for 24 h. This procedure was repeated with a novel CD1 each day. (B) In a separate cohort of animals, this 21-day CSDS paradigm led to a substantial number of attacks and chasing by the CD1 male as quantitively illustrated for the 5-min defeat on day 1, day 8 and day 14 of the experiment. (C) In this cohort, the percentages in which estrus, proestrus or metestrus/diestrus cycle phases occur from day 10 to day 18 of the experiment are comparable for control and CSDS mice. D: day; CSDS: chronic social defeat stress.
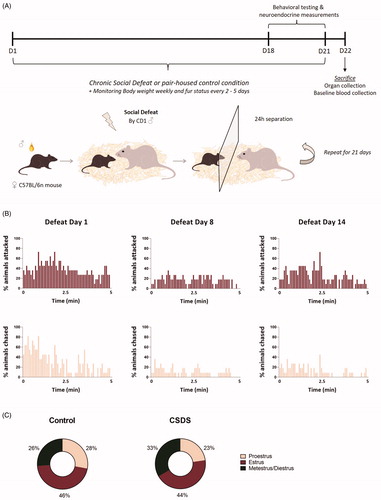
Chronic social defeat stress paradigm
Prior to the start of the CSDS experiment, resident CD1 males were tested on likelihood to attack an intruder C57Bl/6n male and the 12 most aggressive CD1 mice were selected for the CSDS experiment. Based on a protocol by Harris et al. (Citation2018) females were covered in previously collected urine (at room temperature) from C57Bl/6n male mice, in order to trigger an attack by the CD1 residents. Using a brush, urine was applied to the head, back, tail and at the vaginal orifice. The CSDS paradigm was performed as previously described (Wagner et al., Citation2011). In short, following application of male urine, female mice were immediately placed into the home-cage of a CD1 male resident. Animals were separated as soon as the aggressive confrontation was achieved, or after a maximum of 5 min. Subsequently, animals spent 24 h in the same cage (45 × 25 cm) as the resident CD1 male, separated by a transparent divider, to prevent any physical contact, but allow continuation of the social threat via visual and olfactory cues. Each day, experimental animals were introduced (after fresh urine application) into a new cage with a novel resident (). The defeat occurred between 11 a.m. and 4 p.m. each day. Starting times of the defeat varied each day in order to reduce predictability of the stressor and thereby decreasing the chances of a potential habituation effect. Control animals remained pair-housed in their home-cage throughout the experimental period and were exposed to male odor via bedding material once a week. All animals were handled on a daily basis.
In order to allow qualitative evaluation and to further describe the intensity, frequency and duration of the aggressive behavior of the CD1 males towards the female mice, videos were recorded within a second cohort of animals (n = 11 CSDS female C57Bl/6n) on day 1, day 8 and day 14 of the CSDS experiment. The number of attacks and chasing by the CD1 male during the 5-min defeat period was quantified.
Urine collection
Urine from C57Bl/6n male mice was either collected in a tube while manually restraining the mouse, or by placing mice in an empty cage on top of a metal grid. After 1 h, urine was then collected from the bottom of the cage. Fresh urine of 15–20 mice was collected every 3–4 days, mixed together and stored at 4 °C.
Fur status and body weight
Fur status was determined every 2–5 days prior to the social defeat and body weight was measured weekly for both control and CSDS mice. The condition of the fur was assessed by an experienced investigator as described previously (Mineur et al., Citation2003). In short, scores were classified according to a 4-point scale, where 1 stands for a perfect, clean fur, while 4 represents a disheveled, scruffy fur, often including traces of wounds and scurf. Ratings of 2 and 3 demonstrate intermediate fur states, respectively.
Behavioral assessment
Tests were performed between 08.00 a.m. and 12.00 p.m. and took place in a specially equipped behavioral room, adjacent to the housing room. After performance of the test, control mice were put back in their homecage and CSDS females returned to the same cage with the same CD1 as prior to test performance, separated by the transparent divider. On the days of behavioral testing chronic social defeat took place in the afternoon. All tests were recorded and tracked using the automated video-tracking system Anymaze 4.99z (Stoelting, Dublin, IE). In case manual scoring was necessary, this was performed by an experienced observer that was blinded to the experimental condition.
Open field
On day 18 of the experiment, the open field (OF) was conducted as previously performed (Schmidt et al., Citation2007; Sterlemann et al., Citation2008) to assess locomotor activity and anxiety-like behavior. The test was executed in an empty open field arena (50 cm × 50 cm × 50 cm), made out of gray polyvinyl chloride (PVC), under constant lighting conditions of approximately 20 lux. Total test duration was 10 min, in which total distance travelled and the number of entries, time spent and distance travelled within the inner zone of the OF were analyzed.
Elevated plus maze
The elevated plus maze (EPM) was performed on day 19 of the experiment to measure anxiety-like behavior as previously described (Schmidt et al., Citation2007; Sterlemann et al., Citation2008). The apparatus was comprised of a plus shaped platform, with two opposing open arms (30 cm × 5 cm × 0.5 cm) and two opposing enclosed arms (30 cm × 5 cm × 15 cm), made of gray PVC, which were connected by a central area (5 cm × 5 cm × 0.5 cm). The whole apparatus was elevated 50 cm above the floor. Lighting conditions were set at 20 lux in the open arms and less than 10 lux in the closed arms. At the start of the test, animals were placed in the central zone, facing an enclosed arm. The test lasted for 5 min, in which the following parameters were measured: number of entries, time spent and distance travelled in the open and closed arms of the EPM.
Social avoidance task
To test whether CSDS led to avoidance of a social encounter with an adolescent female CD1 mouse, the SA task was conducted on day 20 of the experimental period (according to Tsankova et al., Citation2006). The SA task consisted of two phases: the acquisition phase and the retrieval phase, which all animals underwent subsequently. In the acquisition phase animals were placed in the OF arena with a small empty cage placed in a fixed position of the arena. The acquisition phase lasted for 2.5 min. While animals remained in the OF arena, the empty cage was replaced with a cage containing a female adolescent CD1 mouse, allowing experimental animals to socially interact or avoid interaction with the newly introduced mouse. This retrieval phase lasted for another 2.5 min. A “social zone” was defined, which included an area closely surrounding the inserted cage. Time spent in this social zone was manually scored when the experimental mouse was also directing its head towards the cage.
Forced swim task
The forced swim task (FST) was performed on day 21 of the experiment in order to assess choice of coping strategy upon exposure to a stressful and unescapable environment (as described by Hartmann et al., Citation2012). For this, animals were put in a 2 liter glass beaker (diameter: 13 cm; height: 24 cm) filled with tap water (21 ± 1 °C) up to 15 cm height, so that the mouse could not touch the bottom of the beaker with its hind paws or tail, nor climb out of the beaker. The test lasted for 6 min and after completion of the test, mice were dried with a towel to prevent hypothermia. The following parameters were manually scored: time spent swimming, struggling and floating.
Vaginal smear sampling
Wet vaginal smear samples were taken on each day of behavioral testing. Smears were taken in between 11 a.m. and 1 p.m., right after completion of the behavioral task. As previously described (Caligioni, Citation2009), 40 µl of sterile PBS (room temperature) was carefully pipetted up and down the vaginal canal multiple times, without penetrating the vaginal canal. The collected fluid was then placed on a glass slide and air-dried for at least 1 h. Subsequently, slides were stained with a Giemsa-Wright staining (Sigma-Aldrich) for 30 s and washed with distilled water for 3–7 min. Afterwards, slides were analyzed using a light microscope at 10x magnification. It was decided to divide the cycle in a biphasic manner with proestrus/estrus (P/E) as stages where estradiol levels are usually high and with metestrus/diestrus (M/D) as stages where estradiol levels are usually lower (McLean et al., Citation2012). When samples were ambiguous, they were excluded from analysis.
Stress neuroendocrine assessment
After completion of the FST, all animals were placed in a novel cage to recover from the acute stressor. Neuroendocrine response profiles were assessed by collecting blood samples in 1,5 mL EDTA-coated microcentrifuge tubes (Kabe Labortechnik, Germany) via a tailcut (Fluttert et al., Citation2000) 30 min after onset of the stressor (stress response) and 90 min following onset of the FST (stress recovery). After completion of blood sampling, animals returned to their home-cage (for controls) or their CSDS cage. Blood samples were kept on ice and subsequently centrifuged at 8000 rpm, for 15 min at 4 °C. At least 5 µl of plasma was collected and stored at −20 °C. Later, corticosterone levels (ng/ml) were quantified by radioimmunoassay following the manufacturer’s instructions (MP Biomedicals Inc.; sensitivity 12.5 ng/ml).
Sampling procedure
One day after the last defeat, animals were weighed and subsequently sacrificed by decapitation following quick anesthesia by isofluorane. Baseline trunk blood was collected in 1.5 mL EDTA-coated microcentrifuge tubes (Kabe Labortechnik, Germany). Blood samples were saved on ice and subsequently centrifuged at 8000 rpm, for 15 min at 4 °C. Blood plasma was collected and stored at −20 °C. In addition, the adrenal glands and the thymus were removed, dissected from fat and weighed.
Statistical analyses
Data were analyzed using the IBM SPSS statistics v25 package and graphs were prepared using Graphpad Prism v8.3.
Physiological and neuroendocrine measures were analyzed using independent t-tests. In case data were not normally distributed the non-parametric Mann–Whitney U test was performed. In time-course analyses, a repeated measures analysis of variance (ANOVA) with time as a within-subject factor and condition (CSDS vs. control) as a between-subject factor was applied. For fur status results the non-parametric Friedman test, followed by Chi Square post-hoc testing, was performed. All behavioral measures were analyzed using a two-way ANOVA with condition (CSDS vs. control) and estrous cycle (P/E vs. M/D) as fixed factor. If no effect of estrous cycle and no interaction effect were found, the data were analyzed using a one-way ANOVA with condition as fixed factor and estrous cycle stage as co-variate. In case behavioral data were not normally distributed, a log transformation was performed to normalize data before analyses. If significant main or interaction effects were found, post-hoc independent sample t-tests were performed. Correlation analyses were performed using the Pearson’s correlation coefficient. ANOVA significance levels for main effects were set to p < 0.05 and for interaction effects to p < 0.1. For all post-hoc tests, the significance level was set to p < 0.05. Values outside a margin of two times the standard deviation were considered outliers and excluded from analyses. Data are visualized as the mean ± the standard error of the mean (SEM), including the individual data points. If main effects for estrous cycle or an interaction effect between estrous cycle and condition were found, data were additionally illustrated as separated by cycle stage.
Results
CSDS procedure
The encounter of the resident CD1 male with the urine-covered experimental C57Bl/6n female led to a robust physical attack by the CD1 resident in 60% of the cases (in 150 of the confrontations out of a total 252 confrontations female mice were attacked by the CD1 male). Even though animals were not attacked by a CD1 male on every day of the experiment, overall there was no separation in the cohort with regard to the frequency of aggressive encounters throughout the 21 days. If animals were not attacked, we still observed physical contact between the CD1 resident and experimental mouse in the form of chasing. In most cases, experimental mice displayed a defensive posture upon approach by the CD1 resident, even if this encounter did not lead to an attack. For a more qualitative evaluation of the aggressive behavior of the CD1 males, defeats were recorded on day 1, day 8 and day 14 in a separate CSDS cohort (Supplementary Videos 1–3). The number of attacks and chasing by the CD1 male during the 5-min defeat period on these three days of the experiment were quantified (), illustrating a robust exposure of females to aggressive behavior by the CD1 males in this paradigm. In this cohort, CSDS did not affect cycling ().
Physiological parameters
At the start of the experiment, animals did not significantly differ in body weight. A repeated measures ANOVA revealed a significant within-subjects effect for time (F(2.2,44.3) = 148.732, p < 0.000), as well as time × condition interaction (F(2.2,44.3) = 6.168, p < 0.01) and an in-between-subjects effect for condition (F(1,20) = 5.017, p < 0.05; ). Post-hoc tests indicated that from day 15 of the CSDS paradigm onwards, CSDS mice had an increased body weight compared to control animals (t(20) = −3.597, p < 0.01) and this effect remained until sacrifice at day 22 of the experiment (t(20) = −3.345, p < 0.01).
Figure 2. Chronic social defeat stress leads to changes in body weight, organ weight and fur condition in female C57Bl/6n mice. Exposure to a 21-day social defeat led to (A) a significant increase in body weight from day 15 of the defeat onwards. In addition, (B) fur condition was worsened in socially defeated mice compared to control animals from day 7 of the defeat onwards. Chronic social defeat stress exposure led to (C) a significant increase in relative adrenal gland weight and (D) a tendency for reduced relative thymus weight. CSDS: chronic social defeat stress; data represent mean ± SEM; *p < 0.05; **p < 0.01, ***p < 0.001.
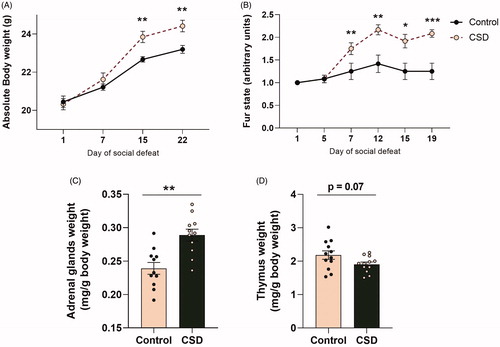
Fur condition scores also did not differ at the start of the experiment, but the non-parametric Friedman test detected differences in fur condition (χ2(5) = 54.459, p < 0.001; ). Post-hoc tests revealed that already at day 7 of the defeat paradigm, CSDS mice had a higher fur state score (χ2(2) = 11.169, p < 0.01) and this difference continued to be present at day 12 (χ2(2) = 12.103, p < 0.01), day 15 (χ2(2) = 9.214, p < 0.05) and day 19 (χ2(2) = 18.333, p < 0.001) of the experiment.
One day after the last defeat, animals were sacrificed and organs were collected and weighed. Following 21 days of CSDS the weight of the adrenal glands corrected for body weight was significantly increased when compared to control animals (t(21) = −3.761, p < 0.01) and although not statistically significant, relative thymus weight tended to be reduced (t(22) = 1.917, p = 0.07) vs. control mice ().
Neuroendocrine measures
Basal and stress neuroendocrine profiles of CSDS and control mice were further investigated by quantifying basal morning levels of corticosterone (at day 22 of the experiment) and corticosterone response and recovery levels upon exposure to an acute stressor (on day 21 of the experiment; respectively 30 min or 90 min following the onset of the FST). We did not observe differences in basal morning corticosterone levels, nor any differences in the corticosterone response 30 min following an acute stressor (). However, the Mann–Whitney U test revealed significantly reduced corticosterone concentrations in the plasma of CSDS mice vs. control mice, during the recovery phase following an acute stressor (U = 29, p < 0.05; ).
Figure 3. Chronic social defeat stress affects corticosterone recovery levels following a stressor in female C57Bl/6n mice. (A) Basal morning corticosterone levels are unaffected following 21 days of social defeat. Following the stressor (forced swim test; FST) the corticosterone response (B) 30 min after FST onset was affected by chronic social defeat (CSDS) exposure, whereas the corticosterone recovery (C) 90 min after FST onset was reduced in CSDS mice compared to controls. CSDS: chronic social defeat stress; data represent mean ± SEM; *p < 0.05.
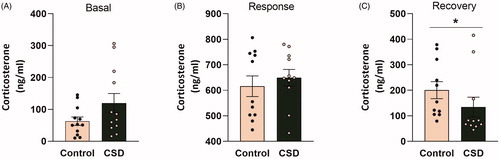
Behavioral assessment
Open field
The OF was used to assess whether CSDS exposure affects locomotor activity and anxiety-like behavior. Mice did not present any changes in overall locomotor activity, as total distance travelled did not differ between CSDS and control mice (F(1,20) = 0.077, p = 0.785; ). A cumulative representation of the distance travelled in the total area of the OF arena is illustrated in , for which a repeated measures ANOVA revealed no differences between CSDS and control animals (F(1,20) = 0.085, p = 0.774).
Figure 4. Chronic social defeat stress exposure increases anxiety-like behavior in female C57Bl/6n mice and this effect is most prominent in the metestrus/diestrus phase of the cycle. Chronic social defeat stress (CSDS) exposure does not affect (A) total distance travelled after 10 min in the Open Field (OF), nor could (B) any changes be observed during the course of the test between CSDS mice and controls. However, exposure to CSDS increases anxiety-like behavior in female C57Bl/6n mice as demonstrated by (C) a decreased number of entries into the inner zone of the OF and (D) a decreased amount of time spent in the inner zone of the OF. The effects on time spent in the inner zone of the OF (E) were most prominent in mice that were in the metestrus/diestrus cycle phase. CSD: chronic social defeat stress; P/E: proestrus/diestrus; M/D: metestrus/diestrus; data represent mean ± SEM. *p < 0.05.
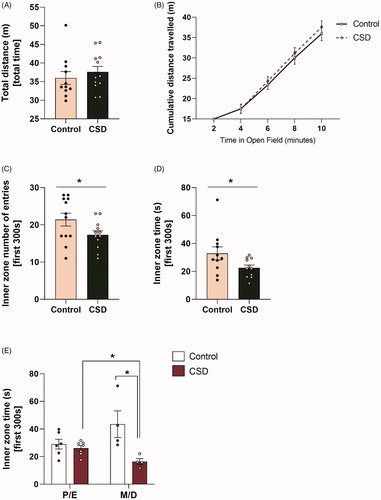
To assess anxiety-like behaviour in the OF, the first 5 min of OF exposure were analyzed. Here, the total number of entries into the inner zone of the OF (F(1,20) = 6.717, p < 0.05) and the time spent in the inner zone of the OF (F(1,18) = 5.718, p < 0.05) was reduced in CSDS mice vs. control mice (). In addition, a two-way ANOVA showed an condition × estrous cycle interaction effect for the parameter time spent in seconds in the inner zone of the OF during the first 300 s (F(1,17) = 7.110, p < 0.05; ). Differences between CSDS and control mice for time in the inner OF zone were only present within mice that were in the M/D phase (t(6) = 2.719, p < 0.05). Furthermore, within the CSDS group, animals within the M/D phase spent even less time in the inner zone of the OF than CSDS animals in the P/E phase (t(9) = 3.196, p < 0.05). No effect for estrous cycle was found for number of entries into the inner zone of the OF (F(1,19) = 0.797, p = 0.383).
Elevated plus maze
In contrast to data from the OF, no differences were found between CSDS and control mice for time spent (F(1,22) = 1.938, p = 0.178), nor for distance travelled (F(1,22) = 0.818, p = 0.376) on the open arms of the EPM. In addition, no estrous cycle effects were found for time spent or distance travelled on the open arms of the EPM (respectively F(1,19) = 0.536, p = 0.473 and F(1,19) = 0.514, p = 0.482).
Social avoidance
Data from the SA task revealed that socially defeated females spent less time in close proximity (time in social zone) of an inanimate object (empty cage) than control mice (F(1,20) = 4.575, p < 0.05; ). A two-way ANOVA showed a condition × estrous cycle interaction effect (F(1,19) = 3.030, p < 0.1) and from post hoc tests it became clear that avoidance of an inanimate object is stronger in CSDS mice within the P/E phase and therefore the effect of CSD is mostly driven by mice in the P/E phase (). However, even though CSDS mice avoided an inanimate object, no differences were found for time spent in the social zone when an adolescent CD1 female mouse was placed in the cage (F(1,19) = 0.217, p = 0.647).
Figure 5. Chronic social defeat stress exposure leads to avoidance of an inanimate object, but not to social avoidance in female C57Bl/6n mice and this process is influenced by the cycle phase. Chronic social defeat stress (CSDS) exposure (A) leads to a decreased approach of an inanimate object, but did not affect the approach of a CD1 female adolescent mouse. The avoidance of an inanimate object (B) was stronger in CSDS mice within the proestrus/estrus (P/E) phase and therefore the effect of CSDS is mostly driven by mice in this P/E phase. CSD: chronic social defeat stress; P/E: proestrus/diestrus; M/D: metestrus/diestrus; data represent mean ± SEM. *p < 0.05; **p < 0.01.
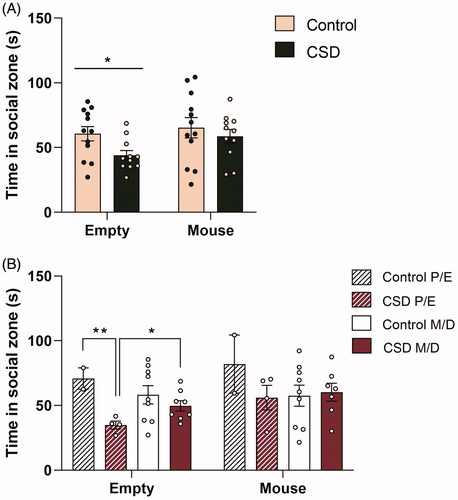
Forced swim task
Finally, the FST was performed to investigate whether CSDS exposure leads to different coping strategy choices upon exposure to a stressful and unescapable environment. As these data were not normally distributed, a log transformation was first applied to the parameters time spent struggling, time spent swimming and time spent floating. An ANOVA with estrous cycle as a covariate subsequently revealed no differences between CSDS and control mice in either time spent struggling (F(1,19) = 0.777, p = 0.389; ), time spent swimming (F(1,19) = 0.230, p = 0.637; ) or time spent floating (F(1,19) = 2.097, p = 0.164; ) in seconds in the FST.
Figure 6. Chronic social defeat does not affect coping strategy selection in female C57Bl/6n mice. Chronic social defeat exposure does not significantly affect (A) time swimming, (B) time struggling or (C) floating behavior in the forced swim task (FST). CSD: chronic social defeat; data represent mean ± SEM.
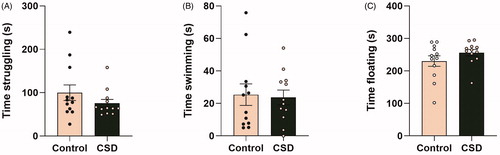
Correlations between physiological and neuroendocrine parameters
A Pearson’s correlation revealed interesting correlations between a number of physiological and neuroendocrine parameters (). Most interestingly, basal morning corticosterone levels at sacrifice and corticosterone recovery levels, 90 min after onset of the stressful FST, were positively correlated with body weight gain after 15 days of CSDS (r = 0.593, n = 24, p < 0.01; ) and negatively correlated with actual body weight following 15 days of CSDS (r = −0.512, n = 20, p < 0.05; ), respectively. Relative adrenal weight was positively correlated with body weight after 15 days of CSDS (r = 0.535, n = 21, p < 0.05; ) and negatively correlated with corticosterone recovery levels, 90 min following onset of the FST (r = −0.460, n = 21, p > 0.05; ).
Figure 7. Physiological parameters of stress are correlated with each other. (A) Basal corticosterone levels at sacrifice and (B) corticosterone recovery levels, 90 min after onset of the stressor (forced swim task; FST) were respectively positively correlated with body weight gain after 15 days of chronic social defeat stress (CSDS) and negatively correlated with actual body weight following 15 days of CSDS. Relative adrenal weight was (C) positively correlated with body weight after 15 days of CSDS and (D) negatively correlated with corticosterone recovery levels, 90 min following onset of the FST. CSDS: chronic social defeat stress. Data represent mean ± SEM. r = Pearson’s correlation, p = significance level.
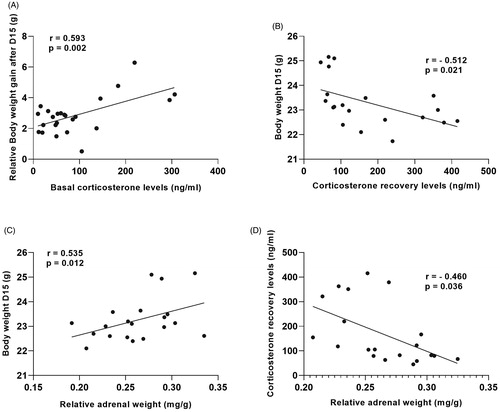
Discussion
Over the past decades it has become more and more evident that male and female responses towards environmental stressors critically differ (Dalla et al., Citation2005; Hodes et al., Citation2015; Kessler, Citation1994; Westenbroek et al., Citation2004) and this has emphasized the importance of including both sexes within stress research. Here, we extend our knowledge on the detailed consequences of CSDS in females, showing that 21 days of CSDS in female C57Bl/6n mice induced many of the physiological, neuroendocrine and behavioral changes often described in males. At the same time, important differences in the response of females to CSDS in comparison to males were uncovered.
In male mice, CSDS commonly causes various physiological changes. Previous studies frequently reported body weight gain, deterioration of fur condition, adrenal glands enlargement and shrinkage of the thymus (Chuang et al., Citation2010; Hartmann et al., Citation2012; Wagner et al., Citation2011). Apart from an increase in adrenal weight in one study (Haller et al., Citation1999), most studies using an alternative method for CSDS in females remarkably do not indicate any of these classical physiological changes (Bourke & Neigh, Citation2012; Newman et al., Citation2019; Takahashi et al., Citation2017; Yohn et al., Citation2019; Citation2019). Harris et al. (Citation2018) neither reported any alterations in physiological parameters as a result of their CSDS protocol. Unlike these earlier studies in females, we show that 21 consecutive days of exposure to CSDS does indeed lead to the physiological changes that are generally found upon CSDS exposure in males, underlining that the stress procedure is effective and that the general physiological adaptations to CSDS are very similar in males and females.
In addition to physiological modifications, alterations in basal and stress-induced neuroendocrine profiles are commonly observed in males that underwent CSDS. As a result of chronic stress exposure, male mice often display elevated basal corticosterone levels as well as dysregulation of hypothalamic-pituitary-adrenal (HPA) axis negative feedback (Hartmann et al., Citation2012; Ron de Kloet et al., Citation2005; Schmidt et al., Citation2010; Sterlemann et al., Citation2008; Wagner et al., Citation2011). In contrast to studies in male mice, no changes in basal neuroendocrine profiles were found in previous chronic stress studies with females and also in our study we did not observe such changes. An explanation for this differential pattern could be that females have higher baseline corticosterone levels than male mice under non-stress conditions (Viau, Citation2002) and this may mask a further slight increase following chronic stress exposure. In the current study we did not observe the in males commonly detected overshoot of the corticosterone response to a novel stressor, which may in part be due to the large variability in the two groups. Interestingly, during the recovery phase, CSDS females displayed significantly reduced corticosterone levels, which may point towards an improved negative feedback regulation. However, other systems like the sympathetic nervous system or the immune system also affect corticosterone feedback regulation and further studies will need to address this phenotype mechanistically.
Adding to these findings, a number of physiological and neuroendocrine parameters of stress were correlated with each other. As previously mentioned, body weight gain can be an indicator of chronic stress exposure and increased basal corticosterone levels or HPA axis activity changes are often associated with vulnerability to stress (Schmidt et al., Citation2010). We found that animals with greater body weight gain during the experiment also had higher basal corticosterone levels. Conversely, body weight during the experiment was inversely correlated with HPA axis activity during the recovery phase of an acute stressor. Furthermore, higher adrenal weight – one of the classical indicators of a hyperactive HPA axis – was associated with increased body weight during the experiment and animals with larger adrenals also had lower HPA axis recovery activity. Altogether, these correlations further underline that the physiological and neuroendocrine responses to CSDS in females are reflective of a stressful state and that we observe individual variability in stress vulnerability and resilience.
Chronic stress also consistently results in a marked behavioral phenotype. Anxiety-like behaviors are often observed in both males and females following chronic stress exposure (Harris et al., Citation2018; Hartmann et al., Citation2012; Schmidt et al., Citation2007; Sterlemann et al., Citation2008; Takahashi et al., Citation2017; Wagner et al., Citation2011; Yohn et al., Citation2019; Citation2019). Here we found that CSDS mice display more anxious-like behavior in the OF test. Moreover, when tested during the SA task, female mice avoided an inanimate object in the open field, suggesting an aversion for novel environments or environmental changes. Interestingly, the estrous cycle phase also modulates anxiety-related behavior in females and therefore plays a significant role in the degree of stress-induced anxiety.
Another behavior that is typically affected following CSDS exposure is social behavior. A large number of studies has found a decrease in social interaction or increase in SA behavior following CSDS exposure and reduced social interaction is often presented as one of the key phenotypic features of vulnerability to chronic stress (Golden et al., Citation2011; Harris et al., Citation2018; Krishnan et al., Citation2007; Newman et al., Citation2019; Russo & Nestler, Citation2013; Takahashi et al., Citation2017; Yohn et al., Citation2019). Remarkably, we did not find such social behavioral changes in our study. One explanation for this could be the choice of the social target. Often a male CD1 mouse, with an identical appearance and age as the initial attacker, is used. However, we selected a female adolescent CD1 mouse as the social target to investigate the generalized nature of a potential SA behavior. We can therefore conclude from our data that in female mice, SA following CSDS is at least not generalized to mice from another sex or age as the initial attacker. It will therefore be important for future studies to differentiate SA behavior based on the sex and age of the tested conspecific.
Our study design was based on the CSDS protocol as described by Harris and colleagues (Harris et al., Citation2018), with only applying an adjustment to the length of the chronic defeat to 21 days. Even though we have reported findings that add to the stress phenotype as described by Harris et al. we have also found some discrepancies between the studies. First of all, Harris and colleagues report increased corticosterone levels, 30 min following the first defeat and 30 min following the defeat on day 10 of the experiment compared to the control condition. In our study, we did not find any changes in corticosterone levels at baseline, nor at 30 min following exposure to the forced swim test. Even though these results may look contrary at first sight, the environmental exposure and/or type of stressor were different in both studies and a direct comparison of the neuroendocrine results can therefore not be made. Moreover, in our study the stressor was applied to both the control and CSDS group, explicitly investigating differential stress reactivity, whereas Harris et al. studied the acute effect of a social defeat (with and without prior CSDS exposure) versus no stress exposure at all. On the behavioral level, one finding that we could not replicate was the presence of SA behavior following CSDS stress, as discussed above. Interestingly, Harris and colleagues further investigated the resiliency or vulnerability towards CSDS in their sample and subsequently stratified for this, which was not possible in our study due to insufficient statistical power. On a further note, the behavioral assessment in the current study was limited to a few tests and we would like to avoid an over-interpretation based on the current results. In fact, a lack of observed phenotype in a specific assay (e.g. the social avoidance task) does not mean that socially stressed females do not show alterations in social behavior. The current available data on the effects of social stress in female mice is – compared to the males – unfortunately still limited, again arguing for the inclusion of females in future studies and a deeper and more thorough phenotyping.
Even though this study and the former report by Harris et al. show that increased male-to-female aggressive behavior, by introducing male odorants and pheromones, leads to a substantial stress phenotype in female mice, there are a few limitations to the study design. Firstly, the number of attacks towards female mice is less than the days of aggression reported in male studies (Golden et al., Citation2011). While all females were attacked regularly during the 21-day defeat period there were variations in attack duration and frequency, including individual days were no aggressive encounter occurred. Even though attack variations over the 21 days was similar for all experimental females, we still cannot rule out that variations in attack frequency across the CSDS procedure may have contributed to the variability in the behavioral and physiological outcomes. However, the presence of an overall robust stress phenotype may reduce this concern. Another concern may be that application of male odors and pheromones alone may affect behavior in female mice. In this study, the sole effect of urine application was not tested. However, control mice were also exposed to male odors on a weekly basis by introducing CD1 male bedding into their homecage. Furthermore, Harris et al. (Citation2018) did include an additional control sample in their study and showed that urine application alone does not affect behavioral outcomes. A last important point of debate is whether a model utilizing male-to-female aggression can be considered etiologically relevant, as compared to a female-to-female aggression models (Logan, Citation2019; Newman et al., Citation2019). It is clear that rendering females to be perceived as males comes along with questions on how etiologically relevant the model is. However, the same is true for CSDS models that rely on female-to-female aggression, where a behavior is elicited that is not observed under (semi)naturalistic conditions and requires substantial experimental manipulation. In fact, also the male CSDS model is very artificial and adapted to a laboratory setting, as in natural mouse populations subordinate males will quickly leave the territory of an alpha male. We believe that having the availability of a simple paradigm that is reproducible across laboratories and simulates a chronic social stress situation in female mice under standard laboratory conditions is of high scientific value.
An important aim of this study was to further describe the differential responses of females to CSDS as compared to male mice. Even though the absence of data from a contemporary, non-historic male sample makes it harder to draw definite conclusions on potential sex differences, the establishment of the CSDS phenotype in males has been repeatedly reported (Golden et al., Citation2011; Hartmann et al., Citation2012; E. J. Nestler & Hyman, Citation2010; Sterlemann et al., Citation2008; Wagner et al., Citation2011), which allows for a more general comparison. When studying female cohorts, the estrous cycle and their corresponding estradiol levels may largely contribute to the variability in neuroendocrine and behavioral outcomes (Green et al., Citation2018; Shansky & Woolley, Citation2016) and in-depth analyses revealed that in our sample the estrous cycle indeed modulated the CSDS induced anxiety-like behavior. However, even though the biphasic estrous cycle assessment was based on predictive estradiol levels (usually high estradiol levels in proestrus/estrus cycle phase vs. the usually lower estradiol levels in the metestrus/diestrus phase; McLean et al., Citation2012), we did not quantify blood estradiol levels. Behavioral effects may therefore just as well be the result of alternative alternating hormone levels, such as progesterone. Moreover, chronic stress may influence the regularity of the female cycling. Even though, based on the observations in our sample there is no indication that in this particular protocol CSDS females stop cycling, the sample size of collected smears was insufficient to exclude more moderate effects of the stress exposure on the female cycle. Nevertheless, it should be clear that the study of the estrus cycle is not a prerequisite when including female cohorts and data of females without the information on cycle stage are equally valid. Many of the effects of CSDS are expected to occur in females independent of the estrous cycle, and this is supported by the data in this study. Therefore, the current model does not require the monitoring of the estrous cycle and the behavioral consequences of CSDS in females can also be studied without taking the cycle of the females in to account.
Taken together, this study provides further insights in the typical physiological, neuroendocrine and behavioral responses of female mice to CSDS. The results pave the way for a direct comparison of the responses of both males and females to chronic social stress and will ultimately allow the sex-specific study of mechanisms underlying individual stress resilience.
Author contributions
LvD and MVS conceived the study. LvD, HY, JB, LB, CE and FT performed the study. LvD analyzed the data and wrote the first draft of the manuscript. All authors contributed to the revision of the manuscript.
Acknowledgements
The authors thank Bianca Schmid and Rainer Stoffel for the outstanding technical assistance and Jessica Keverne for language editing the manuscript.
Disclosure statement
The authors declare no conflict of interest.
Additional information
Funding
References
- Bourke, C. H., & Neigh, G. N. 2012. Exposure to repeated maternal aggression induces depressive-like behavior and increases startle in adult female rats. Behavioural Brain Research, 227(1), 270–275. https://doi.org/10.1016/j.bbr.2011.11.001
- Chuang, J. -C., Krishnan, V., Yu, H. G., Mason, B., Cui, H., Wilkinson, M. B., Zigman, J. M., Elmquist, J. K., Nestler, E. J., & Lutter, M. 2010. A Β3-adrenergic-leptin-melanocortin circuit regulates behavioral and metabolic changes induced by chronic stress. Biological Psychiatry, 67(11), 1075–1082. https://doi.org/10.1016/j.biopsych.2009.12.003
- Caligioni, C. S. 2009. Assessing reproductive status/stages in mice. Current Protocols in Neuroscience, 48(1), A.4I.1–A.4I.8. https://doi.org/10.1002/0471142301.nsa04is48
- Dalla, C., Antoniou, K., Drossopoulou, G., Xagoraris, M., Kokras, N., Sfikakis, A., & Papadopoulou-Daifoti, Z. 2005. Chronic mild stress impact: Are females more vulnerable? Neuroscience, 135(3), 703–714. https://doi.org/10.1016/j.neuroscience.2005.06.068
- Fluttert, M., S. Dalm, & M. S. Oitzl. 2000. A refined method for sequential blood sampling by tail incision in rats. Laboratory Animals, 34(4), 372–378. https://doi.org/10.1258/002367700780387714
- Galea, L. A. M., McEwen, B. S., Tanapat, P., Deak, T., Spencer, R. L., & Dhabhar, F. S. 1997. Sex differences in dendritic atrophy of CA3 pyramidal neurons in response to chronic restraint stress. Neuroscience, 81(3), 689–697. https://doi.org/10.1016/S0306-4522(97)00233-9
- Gassen, N. C., Hartmann, J., Zschocke, J., Stepan, J., Hafner, K., Zellner, A., Kirmeier, T., Kollmannsberger, L., Wagner, K. V., Dedic, N., Balsevich, G., Deussing, J. M., Kloiber, S., Lucae, S., Holsboer, F., Eder, M., Uhr, M., Ising, M., Schmidt, M. V., Rein, T. 2014. Association of FKBP51 with priming of autophagy pathways and mediation of antidepressant treatment response: Evidence in cells, mice, and humans. PLoS Medicine, 11(11), e1001755. https://doi.org/10.1371/journal.pmed.1001755
- Golden, S. A., Covington, H. E., Berton, O., & Russo, S. J. 2011. A standardized protocol for repeated social defeat stress in mice. Nature Protocols, 6(8), 1183–1191. https://doi.org/10.1038/nprot.2011.361
- Green, M. R., Marcolin, M. L., & McCormick, C. M. 2018. The effects of ovarian hormones on stressor-induced hormonal responses, glucocorticoid receptor expression and translocation, and genes related to receptor signaling in adult female rats. Stress, 21(2), 90–100. https://doi.org/10.1080/10253890.2017.1409719
- Gutiérrez-Lobos, K., Scherer, M., Anderer, P., & Katschnig, H. 2002. The influence of age on the female/male ratio of treated incidence rates in depression. BMC Psychiatry, 2(1), 3. https://doi.org/10.1186/1471-244x-2-3
- Haenisch, B., Bilkei-Gorzo, A., Caron, M. G., & Bönisch H. 2009. Knockout of the norepinephrine transporter and pharmacologically diverse antidepressants prevent behavioral and brain neurotrophin alterations in two chronic stress models of depression. Journal of Neurochemistry, 111(2), 403–416. https://doi.org/10.1111/j.1471-4159.2009.06345.x
- Haller, J., Fuchs, E., Halász, J., & Makara, G. B. 1999. Defeat is a major stressor in males while social instability is stressful mainly in females: Towards the development of a social stress model in female rats. Brain Research Bulletin, 50(1), 33–39. https://doi.org/10.1016/S0361-9230(99)00087-8
- Harris, A. Z, Atsak, P., Bretton, Z. H., Holt, E. S., Alam, R., Morton, M. P., Abbas, A. I., Leonardo, E. D., Bolkan, S. S., Hen, R., Gordon, J. A. 2018. A novel method for chronic social defeat stress in female mice. Neuropsychopharmacology, 43(6), 1276–1283. https://doi.org/10.1038/npp.2017.259
- Hartmann, J., Wagner, K. V., Liebl, C., Scharf, S. H., Wang, X. -D., Wolf, M., Hausch, F., Rein, T., Schmidt, U., Touma, C., Cheung-Flynn, J., Cox, M. B., Smith, D. F., Holsboer, F., Müller, M. B., Schmidt, M. V. 2012. The involvement of FK506-binding protein 51 (FKBP5) in the behavioral and neuroendocrine effects of chronic social defeat stress. Neuropharmacology, 62(1), 332–339. https://doi.org/10.1016/j.neuropharm.2011.07.041
- Hodes, G. E., Pfau, M. L., Purushothaman, I., Ahn, H. F., Golden, S. A., Christoffel, D. J., Magida, J., Brancato, A., Takahashi, A., Flanigan, M. E., Ménard, C., Aleyasin, H., Koo, J. W., Lorsch, Z. S, Feng, J., Heshmati, M., Wang, M., Turecki, G., Neve, R., … Russo, S. J. 2015. Sex differences in nucleus accumbens transcriptome profiles associated with susceptibility versus resilience to subchronic variable stress. Journal of Neuroscience, 35(50), 16362–16376. https://doi.org/10.1523/JNEUROSCI.1392-15.2015
- Kessler, R. C. 1994. Lifetime and 12-month prevalence of DSM-III-R psychiatric disorders in the United States: Results from the national comorbidity survey. Archives of General Psychiatry, 51(1), 8. https://doi.org/10.1001/archpsyc.1994.03950010008002
- Ron de Kloet, E., Joëls, M., & Holsboer, F. 2005. Stress and the brain: From adaptation to disease. Nature Reviews Neuroscience, 6(6), 463–475. https://doi.org/10.1038/nrn1683
- Krishnan, V., Han, M. -H., Graham, D. L., Berton, O., Renthal, W., Russo, S. J., LaPlant, Q., Graham, A., Lutter, M., Lagace, D. C., Ghose, S., Reister, R., Tannous, P., Green, T. A., Neve, R. L., Chakravarty, S., Kumar, A., Eisch, A. J., Self, D. W., … Nestler EJ. 2007. Molecular adaptations underlying susceptibility and resistance to social defeat in brain reward regions. Cell, 131(2), 391–404. https://doi.org/10.1016/j.cell.2007.09.018
- Kudryavtseva, N. N. 2000. Agonistic behavior: A model, experimental studies, and perspectives. Neuroscience and Behavioral Physiology, 30(3), 293–305. https://doi.org/10.1007/BF02471782
- Kudryavtseva, N.N., Bakshtanovskaya, I.V., & Koryakina, L. A. 1991. Social model of depression in mice of C57BL/6J strain. Pharmacology Biochemistry and Behavior, 38(2), 315–320. https://doi.org/10.1016/0091-3057(91)90284-9
- Logan, R. W. 2019. Adapting social defeat stress for female mice using species-typical interfemale aggression. Biological Psychiatry, 86(9), e31–e32. https://doi.org/10.1016/j.biopsych.2019.08.007
- McLean, A. C., Valenzuela, N., Fai, S., & Bennett, S. A. L. 2012. Performing vaginal lavage, crystal violet staining, and vaginal cytological evaluation for mouse estrous cycle staging identification. Journal of Visualized Experiments, (67), e4389. https://doi.org/10.3791/4389
- Mineur, Y. S., Prasol, D. J., Belzung, C., & Crusio, W. E. 2003. Agonistic behavior and upredictable chronic mild stress in mice. Behavior Genetics, 33(5), 513–519. https://doi.org/10.1023/A:1025770616068
- Nestler, E. J, & Hyman, S. E. 2010. Animal models of neuropsychiatric disorders. Nature Neuroscience, 13(10), 1161–1169. https://doi.org/10.1038/nn.2647
- Newman, E. L., Covington, H. E., Suh, J., Bicakci, M. B., Ressler, K. J., DeBold, J. F., & Miczek, K. A. 2019. Fighting females: Neural and behavioral consequences of social defeat stress in female mice. Biological Psychiatry, 86(9), 657–668. https://doi.org/10.1016/j.biopsych.2019.05.005
- Russo, S. J., & Nestler, E. J. 2013. The brain reward circuitry in mood disorders. Nature Reviews Neuroscience, 14(9), 609–625. https://doi.org/10.1038/nrn3381
- Sachs, B. D., Ni, J. R., & Caron, M. G. 2014. Sex differences in response to chronic mild stress and congenital serotonin deficiency. Psychoneuroendocrinology, 40, 123–129. https://doi.org/10.1016/j.psyneuen.2013.11.008
- Schmidt, M.V., Scharf, S. H., Sterlemann, V., Ganea, K., Liebl, C., Holsboer, F., & Müller, M. B. 2010. High susceptibility to chronic social stress is associated with a depression-like phenotype. Psychoneuroendocrinology, 35(5), 635–643. https://doi.org/10.1016/j.psyneuen.2009.10.002
- Schmidt, M.V., Sterlemann, V., Ganea, K., Liebl, C., Alam, S., Harbich, D., Greetfeld, M., Uhr, M., Holsboer, F., & Müller, M. B. 2007. Persistent neuroendocrine and behavioral effects of a novel, etiologically relevant mouse paradigm for chronic social stress during adolescence. Psychoneuroendocrinology, 32(5), 417–429. https://doi.org/10.1016/j.psyneuen.2007.02.011
- Shansky, R. M., & Woolley, C. S. 2016. Considering sex as a biological variable will be valuable for neuroscience research. The Journal of Neuroscience, 36(47), 11817–11822. https://doi.org/10.1523/JNEUROSCI.1390-16.2016
- Sterlemann, V., Ganea, K., Liebl, C., Harbich, D., Alam, S., Holsboer, F., Müller, M. B., & Schmidt, M. V. 2008. Long-term behavioral and neuroendocrine alterations following chronic social stress in mice: Implications for stress-related disorders. Hormones and Behavior, 53(2), 386–394. https://doi.org/10.1016/j.yhbeh.2007.11.001
- Takahashi, A., Chung, J. -R., Zhang, S., Zhang, H., Grossman, Y., Aleyasin, H., Flanigan, M. E., Pfau, M. L., Menard, C., Dumitriu, D., Hodes, G. E., McEwen, B. S., Nestler, E. J., Han, M. -H., & Russo, S. J. 2017. Establishment of a repeated social defeat stress model in female mice. Scientific Reports, 7(1), 12838. https://doi.org/10.1038/s41598-017-12811-8
- Tsankova, N. M., Berton, O., Renthal, W., Kumar, A., Neve, R. L., & Nestler, E. J. 2006. Sustained hippocampal chromatin regulation in a mouse model of depression and antidepressant action. Nature Neuroscience, 9(4), 519–525. https://doi.org/10.1038/nn1659
- Viau, V. 2002. Functional cross-talk between the hypothalamic-pituitary-gonadal and -adrenal axes: Testosterone and corticosterone interact on HPA function. Journal of Neuroendocrinology, 14(6), 506–513. https://doi.org/10.1046/j.1365-2826.2002.00798.x
- Wagner, K. V., Wang, X.-D., Liebl, C., Scharf, S. H., Müller, M. B., & Schmidt, M. V. 2011. Pituitary glucocorticoid receptor deletion reduces vulnerability to chronic stress. Psychoneuroendocrinology, 36(4), 579–587. https://doi.org/10.1016/j.psyneuen.2010.09.007
- Westenbroek, C., Den Boer, J.A., Veenhuis, M., Ter Horst, G.J. 2004. Chronic stress and social housing differentially affect neurogenesis in male and female rats. Brain Research Bulletin, 64(4), 303–308. https://doi.org/10.1016/j.brainresbull.2004.08.006
- WHO International Consortium in Psychiatric Epidemiology. 2000. Cross-national comparisons of the prevelances and correlates of mental disorders. Bulletin of the World Health Organization, 78(4): 413–426.
- World Health Organisation. 2020. Mental disorders affect one in four people. WHO. Retrieved January 31, 2020, from https://www.who.int/whr/2001/media_centre/press_release/en/
- Yohn, C. N., Ashamalla, S. A., Bokka, L., Gergues, M. M., Garino, A., & Samuels, B. A. 2019. Social instability is an effective chronic stress paradigm for both male and female mice. Neuropharmacology, 160(December), 107780. https://doi.org/10.1016/j.neuropharm.2019.107780
- Yohn, C. N., Dieterich, A., Bazer, A. S., Maita, I., Giedraitis, M., & Samuels, B. A. 2019. Chronic non-discriminatory social defeat is an effective chronic stress paradigm for both male and female mice. Neuropsychopharmacology, 44(13), 2220–2229. https://doi.org/10.1038/s41386-019-0520-7