Abstract
Sleep disruptions are hallmarks in the pathophysiology of several stress-related disorders, including Major Depressive Disorder (MDD) and Post-Traumatic Stress Disorder (PTSD), both known to disproportionately affect female populations. Although previous studies have attempted to investigate disordered sleep in women, few studies have explored and compared how repeated stress affects sleep in both sexes in either human or animal models. We have previously shown that male rats exhibit behavioral and neuroendocrine habituation to 5 days of repeated restraint, whereas females do not; additional days of stress exposure are required to observe habituation in females. This study examined sex differences in sleep measures prior to, during, and after repeated restraint stress in adult male and female rats. Our data reveal that repeated stress increased time spent awake and decreased slow-wave sleep (SWS) and REM sleep (REMS) in females, and these effects persisted over 2 days of recovery. In contrast, the effects of stress on males were transient. These insomnia-like symptoms were accompanied by a greater number of exaggerated motor responses to waking from REMS in females, a phenotype similar to trauma-related nightmares. In sum, these data demonstrate that repeated stress produces disruptions in sleep that persist days after the stress is terminated in female rats. These disruptions in sleep produced by 5 days of repeated restraint may be due to their lack of habituation.
Introduction
Stress is associated with the onset of several psychiatric disorders including Major Depressive Disorder (MDD) and Post-Traumatic Stress Disorder (PTSD) (Abdallah et al., Citation2019; Whitaker et al., Citation2014). Sleep disturbances are common in stress-related psychiatric disorders, often presenting as recurrent nightmares and hyperarousal in the form of insomnia (Ross et al., Citation1989). Incidence rates for stress-related psychiatric disorders are twice as high in women compared with men. Thus, biological sex appears to be an important factor in the etiology of these diseases (Bangasser & Valentino, Citation2014).
There is robust evidence suggesting that sex modifies the circuitry of stress response systems as females generally present increased endocrine and neurobehavioral responses to repeated stress (Bangasser & Wiersielis, Citation2018; Grafe, Cornfeld, et al., Citation2017; Grafe & Bhatnagar, Citation2018). Further, there are differences in the prevalence of certain sleep disorders in males and females. For instance, rapid eye movement sleep (REMS) behavior disorder is higher in men, whereas insomnia disorder is more common in women (Mong & Cusmano, Citation2016).
Although previous studies have investigated disordered sleep in women, few studies have methodically compared how repeated stress affects sleep macroarchitecture and microarchitecture in both sexes (Mallampalli & Carter, Citation2014). Thus, the goal of this study was to better understand sex differences in sleep measures after repeated stress using a rat model. Specifically, we examined sleep architecture before, during, and after five consecutive days of restraint stress in male and female rats. We have previously shown that male rats exhibit behavioral and neuroendocrine habituation to 5 days of restraint, whereas females require additional days of restraint in order to habituate (Grafe, Cornfeld, et al., Citation2017). In this study, we observed that repeated restraint stress caused increases in time spent awake and decreases in Slow-wave sleep (SWS) and REMS in female, but not male, rats. Moreover, female rats compared with males demonstrated a higher number of exaggerated motor responses to waking from REMS.
The neuropeptides orexins are important in promoting stress and wake (Johnson et al., Citation2012; Winsky-Sommerer et al., Citation2003; Xu et al., Citation2013), and have been shown to contribute to sex differences in the stress response (Grafe, Cornfeld, et al., Citation2017). Importantly, female rats demonstrate heightened orexin activity and expression, which is associated with exacerbated behavioral and neuroendocrine responses to stress. Thus, we predicted that orexins would contribute to increased wake after stress in female rats. To test this hypothesis, orexin system activity was reduced in females prior to each restraint stress using an inhibitory DREADDs (Designer Receptors Exclusively Activated by Designer Drugs) construct. However, inhibition of orexin neurons prior to each restraint did not alter the stress-induced sleep changes in female rats. In sum, our data suggest that repeated stress results in persistent sleep disturbances in female rats and that orexins released by daily stress are not a significant contributor to the sleep deficits observed in females.
Materials and methods
Animals
Sprague–Dawley rats 65–75 days of age were obtained from Charles River Laboratories (n = 10 males, 26 females). More specifically, Cohort 1: Sex difference Study: 10 males, 10 females; Cohort 2: DREADDs inhibition in female rats: 8 veh females, 8 CNO females. (Wilmington, MA, USA). They were singly housed under a 12-h light/dark cycle (lights on at 7am and off at 7pm), had food and water available ad libitum, and were acclimated to the housing and lighting conditions for at least 5 days prior to any experimental procedures. The Institutional Animal Care and Use Committee of The Children’s Hospital of Philadelphia Research Institute approved all experimental procedures.
Instrumentation surgery, telemetric recordings, and restraint stress procedures
Surgical procedures were performed under sterile conditions, and Isoflurane (2–3%) was used to induce and maintain anesthesia throughout the surgery. A midline incision was performed into the abdominal cavity to suture a telemetric transmitter (Physiotel HD-S02; Data Science International, St. Paul, MN, USA) to the peritoneal wall. It was equipped with sensors that measured animals’ core body temperature and motor activity and had two biopotential amplifiers.
Two screws were implanted into the skull (1 mm to the right and to the left of lambda) and connected to an amplifier to measure cortical electroencephalogram (EEG) activity. Postural muscle electromyogram (EMG) activity was recorded via two insulated stainless steel wire electrodes attached bilaterally to dorsal neck muscles. After instrumentation, the scalp and the opening into the intraperitoneal cavity were sutured and the analgesic meloxicam was administered (2 mg/kg, sc). Animals were given one week to recover from surgery.
Twenty-four-hour continuous EEG and EMG recordings were initiated by remote activation of a magnetic switch in the transmitter. In each animal, the first 2 days of the protocol were used to collect baseline activity. Then, each animal was exposed to 5 consecutive days of 30-min restraint, and recording was continued for 2 days after the last restraint day (). Restraint stress was applied within 2 hours after lights-on using Plexiglas restraint tubes, as described previously (Grafe, Cornfeld, et al., Citation2017).
Figure 1. Experimental paradigm and representative sleep traces. (A) Experimental paradigm depicting the timeline for implantation of telemetry devices, continuous wireless EEG/EMG recordings, and 5 days of repeated restraint stress. The light period (analyzed from 10am to 7pm) is represented by open white boxes, while the dark period (analyzed from 7pm to 6am) is represented by black boxes. 30-min restraint took place during the light period. (B) Representative EEG, EMG, and activity traces of wake (denoted as W), slow wave sleep (denoted as S) and REM sleep (denoted as P for paradoxical sleep).
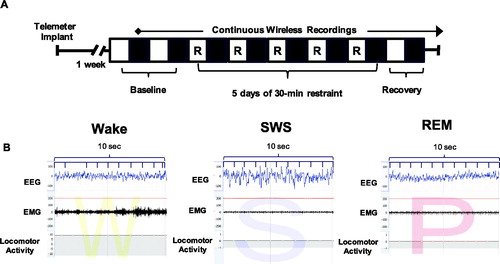
6 animals run in the study had unreadable telemeter recordings, and thus, were not included in the analysis. Thus, the final numbers are as follows: Cohort 1: Sex Difference Study: 8 males, 7 females; Cohort 2: DREADDs inhibition in female rats: 7 veh females, 8 CNO females.
Sleep scoring and analysis
Sleep-wake states were scored in 10-s epochs using Neuroscore software (Data Science International, St. Paul, MN, USA). Wake and sleep percentage data were calculated using the automated Rodent Sleep Scoring Module 2 in Neuroscore. Using a probability matrix, Neuroscore determines the most likely sleep or wake states: wake (denoted as W on traces), slow-wave sleep (denotes as S on traces), and REM sleep (denoted as P on traces for paradoxical sleep); see for examples.
The EEG data were analyzed using a fast Fourier transform (FFT), which efficiently calculates either the discrete Fourier transform (DFT) of a signal or its inverse (IDFT), a representation of the signal in the frequency domain. In EEG, the two bands of interest examined are Delta power (frequencies between 0.05 and 4 Hz) and Theta power (frequencies between 4 and 8 Hz).
The EMG signal was analyzed by amplitude in relation to baseline. Motor activity data are derived using an algorithm that compares the signal strength generated by the telemeter implant with that received by the receiver antenna. While this signal strength naturally fluctuates, the algorithm generates activity counts only when it changes by a certain amount, indicating that the subject is active. For more information, see https://support.datasci.com/hc/en-us/articles/115005030328-Understanding-the-Ponemah-Activity-Derived-Parameter.
Depending on the EMG value and presence of activity (indicated by the movement of the transmitter), the epoch will likely be scored as a wake. If EMG and activity recordings are low, then EEG measurements are examined using delta ratio (delta power divided by total power from 0.5 to 25 Hz) and the Theta Ratio (Theta power divided by Delta power). The 10-s epoch is likely scored as slow-wave sleep when there is high Delta power and REM sleep when there is high Theta power. This algorithm is very reliable as long as the signals are relatively clean. Additionally, two research assistants blind to group conditions visually cross-checked the accuracy of the recognition of sleep-wake stages.
Percentage of time in the wake and sleep states (wake, slow-wave sleep, and REM sleep), occurrences of total awakenings, exaggerated motor activation at awakening, and REM sleep continuity was analyzed. These data were averaged across the 2 baseline days, then calculated for 5 days of restraint, and finally averaged over 2 recovery days. Percentage of time in sleep and wake states were split into light and dark periods. In the light period (), data were analyzed from 10am to 7pm on the days of restraint (in order to capture the light period after the end of restraint stress until lights-off). The same time frame (10am to 7pm) was analyzed for the baseline and recovery time points. For the dark period (), data were analyzed from 7pm to 6am (starting at lights-off) at the same time periods.
Figure 2. Percent wake, SWS, and REM in the light (A–C) and dark (D–F) periods before, during, and after repeated restraint. (A) Percent time spent awake during the light period in male and female rats at baseline, restraint, and recovery. Repeated restraint stress increases wake in both sexes, but these changes persist during the recovery period in females (but not males). (B) Percent time spent in slow wave sleep (SWS) during the light period in male and female rats at baseline, restraint, and recovery. 5 days of repeated restraint stress decreases SWS in both sexes. (C) Percent time spent in REMS during the light period in male and female rats at baseline, restraint, and recovery. Repeated restraint stress does not significantly affect percent time in REMS. (D) Percent time spent awake during the dark period in male and female rats at baseline, restraint, and recovery. Females demonstrated more wake than males after restraint. (E) Percent time spent in slow wave sleep (SWS) during the light period in male and female rats at baseline, restraint, and recovery. Females exhibited less SWS than males on post-restraint stress days. (F) Percent time spent in REMS during the light period in male and female rats at baseline, restraint, and recovery. 5 days of restraint stress decreases percent REM compared to 1 day of restraint stress in both sexes. *p < 0.05.
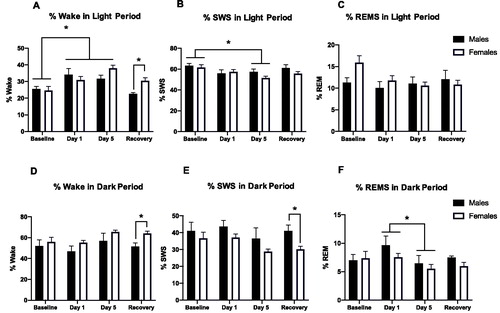
Exaggerated motor responses to waking were analyzed over a 6-h period (10am to 4pm) and averaged on baseline days, days 1 and 5 of restraint, and recovery days. They were identified by visual examination of the nuchal EMG and locomotor activity over a 10-s period following the termination of each sleep bout. Awakenings were categorized as exaggerated when the burst of nuchal EMG crossed a pre-set threshold (200 μV) and there was concurrent locomotor activity within the first 10 s after awakening. The EMG threshold used in this analysis was validated in a separate group of rats in which a systematic search was conducted for a threshold value that effectively discriminated between awakenings with and without immediate transition into a motor response as previously described (Grafe et al., Citation2020). shows examples of a typical awakening and an awakening associated with exaggerated motor activation, respectively.
Figure 3. Number of exaggerated motor responses during transitions to waking from SWS or REMS in the light period before, during, and after repeated restraint. (A) Representative typical awakening: a trace of EEG, EMG, and locomotor activity data during arousal from a REMS episode (initiated at arrow). Note that EMG spikes do not go above the 200 μV threshold in red within 10 s of the sleep transition. (B) Representative exaggerated motor response at awakening: a trace of EEG, EMG, and locomotor activity data during arousal from a REMS episode (initiated at arrow). Note the recurrent EMG spikes greater than 200 μV (denoted with black arrows) that occur within 10 s of the sleep transition with concurrent locomotor activity. (C) Total counts of exaggerated motor responses to waking during 6 h of the light period at baseline, over 5 days of restraint, and during the recovery period. There were no significant main effects of Stress or Sex on the total number of exaggerated motor responses to waking. (D, E) Exaggerated motor responses counted separately following arousals from REMS and SWS, respectively. Females had significantly more exaggerated responses to waking from REMS after restraint compared with males. However, stress and sex did not affect the number of exaggerated motor responses to waking from SWS. *p < 0.05.
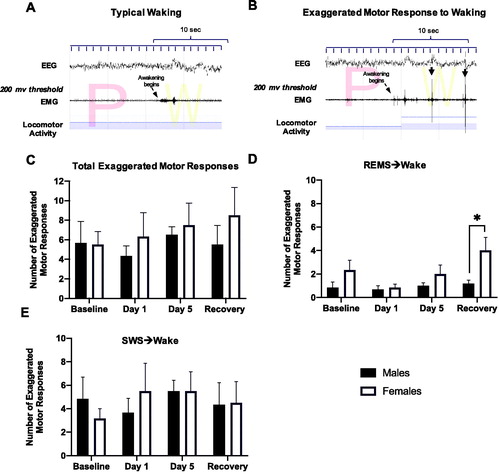
Fragmentation of REMS was assessed by visually scoring the relative times of occurrence of individual REM sleep episodes. According to the classification introduced by (Amici et al., Citation1994), two types of REMS episodes were distinguished: those that were followed by another REMS episode after an interval longer than 3 min (“single” episodes, sin-REMS), and those followed by another REMS episode after 3 min or less (“sequential” episodes, seq-REMS). The total numbers of episodes of each type were counted over 6 h of recording (10am to 4pm) at baseline, days 1 and 5 of restraint, and over 2 recovery days. Seq-REMS episodes are, on average, of shorter duration than sin-REMS episodes and tend to occur in clusters (Amici et al., Citation1994). Importantly, a shift toward more seq-REMS was previously shown to be indicative of increased fragmentation of REMS (Amici et al., Citation1994; DaSilva et al., Citation2011).
DREADDs inhibition of orexin neurons in female rats
To elicit inhibition of orexin neurons for up to 4 hrs (Alexander et al., Citation2009; Farrell & Roth, Citation2013), an inhibitory DREADDs construct with an orexin promoter expressed in a recombinant adenovirus (rAAV2/1-Ple112-hM4Di-mCitrine) was used as previously described (Grafe, Cornfeld, et al., Citation2017; Grafe, Eacret, et al., Citation2017; Grafe et al., Citation2018) in a separate cohort of female rats (Cohort 2; See for the timeline of the experimental paradigm). In short, an hM4D DREADDs virus (109 titer, 1 µl bilaterally) was stereotactically injected into the lateral hypothalamus (2.5 mm caudal to bregma, 1.8 mm from mid-line and 8 mm ventral) of female rats anesthetized using a cocktail of ketamine, xylazine, and acepromazine. The viral injection surgery occurred 3 weeks prior to telemetric instrumentation surgery.
Female rats received injections of vehicle (saline and 8% DMSO) or CNO (Sigma-Aldrich; St Louis, MO 2 mg/kg ip) 60 min prior to the start of the 30-min restraint for 5 consecutive days as previously described (Grafe, Cornfeld, et al., Citation2017). Previous studies have shown that CNO treatment in non-DREADDs-expressing rats does not show off-target behavioral effects (Eacret et al., Citation2019). Sleep scoring and analysis were conducted as described in the previous section. After behavioral experiments concluded, expression of the DREADDs construct was verified by immunofluorescence as previously described (Grafe, Cornfeld, et al., Citation2017; Grafe, Eacret, et al., Citation2017; Grafe et al., Citation2018) (see ).
Statistical analysis
Data are presented as the mean ± standard error of the mean (SE). Two-way repeated-measures ANOVAs were run to examine sleep-wake percentages (Sex × Stress), exaggerated motor responses (Sex × Stress), REM fragmentation (Sex × Stress), and sleep-wake percentages after DREADDs manipulation (CNO × Stress). Graphs were generated using GraphPad Prism (GraphPad Software, La Jolla, CA, USA). Multiple comparison analyses were run to further investigate the interactions between sex and stress. In all analyses, α = 0.05 was regarded statistically significant.
Results
Percentages of time in the wake and different sleep stages during the light period were calculated before, during, and after repeated restraint stress in male and female rats (). A two-way ANOVA revealed a significant main effect of Stress and an interaction between Stress and Sex for percent time in wake (wake time/total recording period time; ; Stress, F(3, 22) = 10.3, p < 0.001; Stress × Sex F(3, 30) = 3.7, p < 0.05). Post hoc tests revealed that both day 1 and day 5 of restraint stress increased percent wake in both sexes, and this persisted for females (but not males) during the recovery period. We further examined locomotor activity on days 1–5 of restraint and found that anticipatory activity increased 30 min prior to each restraint in both sexes compared to baseline and recovery measures. Though females had a higher anticipatory activity to restraint than males overall, it was most apparent on days 2 and 4 of restraint (data not shown). For SWS, a two-way ANOVA revealed a main effect of Stress (; Stress, F(3, 25) = 5.2, p < 0.05). Specifically, post hoc tests indicated decreased SWS in both sexes on day 5 of restraint. There were no significant main effects of stress or sex on percent time in REMS during the light period. We next compared the latency to the first REMS episode in males and females after 30-min restraint on Day 1 versus Day 5. However, there was no effect of restraint day or sex on latency to REMS (data not shown). In sum, repeated restraint stress increases wake and decreases SWS in both sexes, but these changes persist during the recovery period in females (but not males). That is, repeated restraint stress causes persistent disruptions in sleep in female rats during the light period (when sleep should be prominent) compared with male rats.
Percentages of time in wake and different sleep stages during the dark period were also calculated before, during, and after repeated restraint stress in males and females (). Both percent wake and percent SWS showed main effects of Sex in two-way ANOVAs (Wake Sex effect: F(1, 11) = 5.8, p < 0.05; SWS Sex effect: F(1, 11) = 5.7, p < 0.05). Specifically, during the dark period, females demonstrated more wake and less SWS than males after restraint. There was an effect of stress on percent REM during the dark period (F(3, 28) = p < 0.05). Post hoc tests revealed that day 5 of restraint resulted in significantly less percent REM than Day 1 of restraint in both sexes.
In order to analyze sleep to wake transitions, we calculated the number of exaggerated motor responses to waking, as defined in the Methods. There were no significant main effects of Stress or Sex on the total number of exaggerated motor responses to waking (). However, when awakenings were further categorized into those terminating SWS or REMS, a two-way ANOVA revealed a main effect of Stress and Sex on exaggerated motor responses to waking from REM sleep (, Stress, F(3, 22) = 3.7, p < 0.05; Sex, F(1, 10) = 5.6, p < 0.05). Post hoc tests revealed that females had significantly more exaggerated responses to waking from REMS after restraint compared with males. However, Stress and Sex did not affect the number of exaggerated motor responses to waking from SWS ().
As restraint stress-induced sex-specific effects on arousal from REMS, we also investigated REMS continuity by examining REMS microarchitecture (). The number of single REMS (sin-REMS) episodes did not significantly differ between males and females at any time point. Similarly, there were no significant main effects of stress or sex on sequential REMS (seq-REMS) episodes. Thus, 5 days of restraint stress does not significantly impair REM sleep continuity in male and female rats.
Figure 4. (A, B) Continuity of REMS (single versus sequential episodes of REMS) in the light period before, during, and after repeated restraint. Repeated restraint stress did not significantly alter the number of single or sequential REMS episodes in male or female rats.
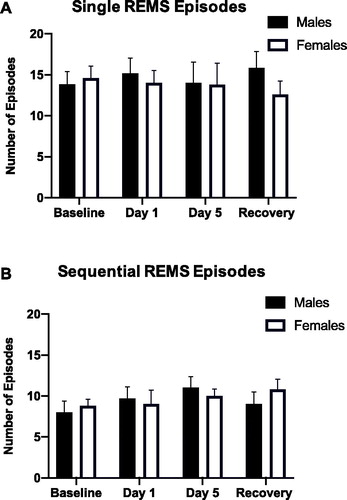
Previous studies have demonstrated that the neuropeptides orexins are important in modulating both stress and sleep (Johnson et al., Citation2012; Winsky-Sommerer et al., Citation2003). Based on our earlier study (Grafe, Cornfeld, et al., Citation2017), we hypothesized that orexins contribute to the stress-induced sleep deficits observed in females. To test this hypothesis, orexin system activity was reduced in females using an inhibitory DREADDs construct (for experimental paradigm and representative images of viral expression, see ). Wake and sleep percentages were analyzed in the light and dark period after orexin neural inhibition by Clozapine-N-Oxide (CNO) administration prior to each restraint (). There were no significant effects of CNO on the percentage of wake, SWS, or REMS in either the light or dark period. Additionally, orexin neural inhibition did not significantly affect sleep to wake transitions. Specifically, CNO administration prior to each restraint did not change the number of exaggerated motor responses to waking in females (data not shown). Thus, inhibiting orexin action during repeated restraint did not significantly change sleep in female rats either before or after stress.
Figure 5. Experimental paradigm and representative viral expression (Experiment: inhibiting orexin neurons throughout repeated restraint in female rats via DREADDs). (A) Experimental paradigm depicting the timeline for DREADDs viral injection, implantation of telemetry devices, continuous wireless EEG/EMG recordings, and 5 days of repeated restraint stress in female rats. The light period (analyzed from 10am to 7pm) is represented by open white boxes, while the dark period (analyzed from 7pm to 6am) is represented by black boxes. 30-min restraint took place during the light period. (B) Representative images displaying viral expression of DREADDs in the LH of female rats at 4 weeks. (C) A composite image displaying the spread of viral expression along the LH in female rats is depicted using rat brain atlas images (Paxinos & Watson, Citation1998). Each red dot represents a cell expressing the viral tag.
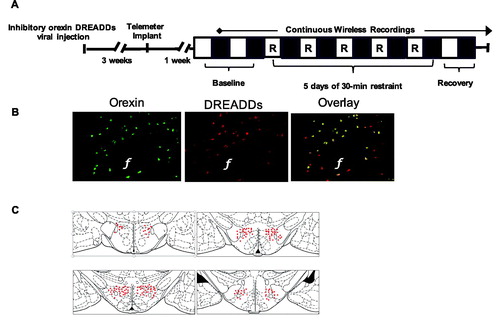
Figure 6. Inhibiting orexin neurons throughout repeated restraint does not change percent wake, SWS, and REMS in the light or dark period in female rats. (A–C) Percent time spent in wake, slow wave sleep (SWS) and REM during the light period in female rats at baseline, restraint, and recovery. Clozapine-N-oxide (CNO)-treated females (orexins inhibited before each restraint) do not show significant changes in wake or sleep compared to vehicle-treated female rats. (D–F) Percent time spent in wake, slow wave sleep (SWS) and REM during the dark period in female rats at baseline, restraint, and recovery. Clozapine-N-oxide (CNO)-treated females (orexins inhibited before each restraint) do not show significant changes in wake or sleep compared to vehicle-treated female rats.
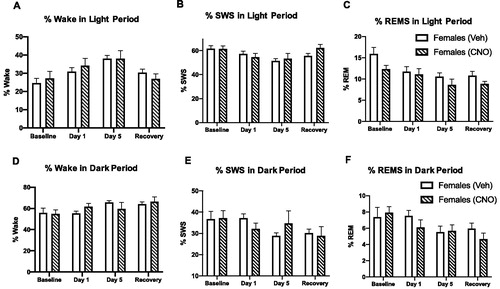
Discussion
Repeated stress increases the risk of developing psychiatric illnesses including MDD and PTSD. Sleep disturbances are common phenotypes in these disorders, often presenting as hyperarousal in the form of insomnia or recurrent nightmares (Ross et al., Citation1989). Interestingly, these stress-related disorders disproportionately affect females. Although previous research has examined the effect of stress on sleep in women, there are limited studies comparing the effects of stress on sleep amounts and architecture in males and females in either humans or preclinical models (Mallampalli & Carter, Citation2014).
To fill this gap in knowledge, our study examined sex differences in the effects of repeated stress on sleep in adult male and female rats. Specifically, we measured sleep quantity and continuity before, during, and after repeated restraint stress. Our data revealed that repeated restraint stress increased the percent of time spent awake, and decreased percentages of SWS and REMS to a greater degree in females compared to males, and these disruptions were still apparent during recovery days in females. Female rats also demonstrated more exaggerated motor responses to waking from REMS compared with male rats. However, restraint stress did not significantly impair REMS continuity in either sex (assessed by single and sequential REM episodes). Altogether, these data indicate that repeated restraint stress leads to persistent disruptions in sleep in female rats.
The neuropeptides orexins are important in regulating both stress and sleep (Johnson et al., Citation2012; Winsky-Sommerer et al., Citation2003; Xu et al., Citation2013), and have been shown to contribute to sex differences in the stress response (Grafe, Cornfeld, et al., Citation2017). Therefore, we tested whether orexins might contribute to these stress-induced sleep deficits observed in females. However, inhibition of orexin neurons with DREADDs prior to each restraint did not cause significant changes in sleep in female rats. These data suggest that orexins released in temporal proximity to the restraint exposure are not important in producing the sleep deficits in females. However, these results do not rule out the possibility that actions of orexins over the remainder of the day are involved in producing the sleep deficits in females.
Stress effects on percent time spent in wake, SWS, and REMS
We found that repeated restraint stress increased the percentage of wake and decreased the percentage of SWS in both sexes during the light period, when sleep should be prominent. Sex differences became even more apparent in the dark period, where females spent more time awake and less time in SWS in the two days following repeated restraint stress. Thus, females were unable to compensate for decreased SWS at any point throughout the day. These data partially support previous clinical findings of lower SWS in women relative to men (Mong & Cusmano, Citation2016; Spoormaker & Montgomery, Citation2008). Moreover, in clinical populations, lower amounts of slow-wave sleep indicates lighter sleep, which increases the likelihood to be awakened (Fuller et al., Citation1994).
While repeated restraint stress did not significantly reduce the percentage of time spent in REMS in the light period, there was a significant effect of stress on percent REMS in the dark period. Specifically, when comparing day 5 of restraint with day 1 of restraint, there was a decrease in percent time in REMS. Data concerning REMS percentages in stress-related disorders such as PTSD are equivocal, with some showing a higher percentage of REMS in PTSD, while others show no changes between clinical and non-clinical populations (Pace-Schott et al., Citation2015). The time after trauma where sleep is examined could be a critical factor in the effects observed (Mellman et al., Citation2014). At this point, the literature provides more evidence that stress may influence the continuity of REMS, rather than the quantity of this type of sleep (Pace-Schott et al., Citation2015).
Taken together, the data indicate that repeated restraint stress decreases percentages of SWS and REMS, with sex differences becoming more apparent by day 5 of restraint and persisting during the recovery period. This is congruent with a previous finding showing that women tend to take longer to recover from sleep deprivation (Corsi-Cabrera et al., Citation2003). Ultimately, our data demonstrate pervasive insomnia-like symptoms in rats following exposure to repeated stress, with significant increases in wake and decreases in REMS and SWS, especially in females. Increases in the wake are clearly related to the maladaptive state of hyperarousal, a key marker of PTSD. Hyperarousal, by causing hypervigilance and restlessness, contributes to perpetuating sleep impairments over time (Mellman et al., Citation2002).
Stress effects on sleep-wake transitions
Recurring nightmares, defined by the DSM-5 as long frightening dreams that cause one to awaken, are a hallmark of PTSD (Campbell & Germain, Citation2016). Treatment focused on alleviating nightmare frequency and intensity significantly improves patients’ quality of life (Spoormaker & Montgomery, Citation2008). Moreover, previous studies have indicated a strong relationship between the experience of distressing dreams following trauma and the development of PTSD (Zadra & Germain, Citation2009). Trauma-related nightmares are associated with exaggerated motor activation and autonomic arousal (Campbell & Germain, Citation2016). In our study and others, exaggerated motor responses to waking from sleep are used to represent stress-related nightmares (Grafe et al., Citation2020; Yu et al., Citation2015).
Our analyses of sleep to wake transitions during the light period showed a main effect of sex on the number of exaggerated motor responses to wake from REM sleep in particular. Specifically, female rats demonstrated significantly more exaggerated motor responses to wake from REMS than male rats during recovery from 5 days of repeated restraint stress. However, neither time nor sex had an effect on exaggerated motor responses from SWS. As nightmares are primarily a phenomenon of REMS (Germain, Citation2013), our study may model these sleep disturbances that appear soon after traumatic stress in humans. Importantly, previous studies have indicated that women report experiencing more nightmares than men (Habukawa et al., Citation2018). Because nightmares reinforce stressful memories, they may contribute to the increased rates of PTSD in women compared to men (Germain, Citation2013; Kobayashi & Mellman, Citation2012).
Stress effects on REMS continuity
As exaggerated motor responses to waking appeared to be more prevalent during REMS, we next examined REMS microarchitecture. Fragmented REMS episodes are predominant in PTSD patients and thus represent a neural marker of the deleterious consequences of psychological trauma on sleep quality (Habukawa et al., Citation2018; Yehuda et al., Citation2015). In our study, REMS continuity was measured by classifying REM episodes as single or sequential as described in previous studies (Amici et al., Citation1994; Grafe et al., Citation2020). Ultimately, more frequent sequential REMS episodes indicate increased REMS fragmentation (DaSilva et al., Citation2011). Our data revealed that repeated restraint stress did not significantly alter the number of single or sequential REMS episodes in male or female rats. In short, 5 days of repeated stress did not produce REM fragmentation. Thus, although females spent more time awake and showed more frequent exaggerated motor activations to waking from REMS, they did not significantly differ from males in terms of REM fragmentation. However, it is possible that other measures of REMS quality not assessed, such as REM density, may show sex differences.
Role of orexins in stress-induced sleep impairments
While the neural substrates underlying stress-induced hyperarousal in females are unknown, the neuropeptides orexins are one likely candidate. First, orexins have a well-established role in promoting arousal and wakefulness (Sakurai, Citation2007; Winsky-Sommerer et al., Citation2003). Moreover, previous studies have indicated that orexins reciprocally interact with the HPA system (Winsky-Sommerer et al., Citation2005) and are important mediators of sex differences in the response to repeated stress (Grafe, Cornfeld, et al., Citation2017). Importantly, female rats demonstrate heightened orexin activity and expression, which is associated with exacerbated behavioral and neuroendocrine responses to stress. Thus, it seemed plausible that orexins might explain increased wake after stress in females.
However, when we inhibited orexin neural activity with DREADDs prior to each restraint stress, we did not observe significant changes in sleep. Previous studies have shown that CNO promotes behavioral effects in the rat within 30 min and effects last up to 4 hours (Alexander et al., Citation2009; Farrell & Roth, Citation2013). Thus, in our study in which DREADDs were activated 60 min prior to the onset of the five daily restraint episodes, orexin release would have been inhibited for the period of time around the stress exposure. The lack of effect of orexin inhibition suggests that the orexins released proximal to restraint exposure are not important in the sleep deficits that occur throughout the day. One explanation for these lack of effects is that orexins acting at other times of the day are involved in producing the sleep deficits. Alternatively, other neural substrates known to promote arousal, including norepinephrine, histamine, and acetylcholine (Cano et al., Citation2008; Mignot et al., Citation2002; Zeitzer, Citation2013), may have compensated in response to reduced orexin action.
Study limitations and future directions
Our study reveals the presence of observed sex differences in insomnia-like and nightmare-like sleep parameters after repeated restraint stress. Indeed, female rats exhibited increased percentages of wakefulness as well as decreased percentages of time spent in REM and SWS. Females further experienced a greater number of exaggerated motor responses to wake from REM. Though orexins are known to contribute to heightened stress in female rats, inhibition of orexins proximal to the time of stress did not ameliorate stress-induced sleep deficits. While we verified sufficient expression of our orexin DREADDs virus, we did not colabel with a neural marker, thus, it is possible that glial inhibition could contribute to the observed lack of effects. Moreover, we did not verify the successful inhibition of orexin neurons. Thus, future studies should incorporate electrophysiological measures or an easily observable behavioral change upon CNO inhibition of orexin neurons to verify its efficacy.
As our rats are singly housed, it is important to consider the effects of single housing on each sex, and how this could have influenced our results. Previous research has indicated that corticosterone levels are elevated in adult females when singly-housed compared to when group-housed; in contrast, males may have lower corticosterone levels when singly-housed compared to when group-housed (Brown & Grunberg, Citation1995). Thus, isolation housing may influence susceptibility to restraint stress (Goel et al., Citation2014). However, housing status does not appear to exacerbate or attenuate sex differences in sleep physiology directly (Ehlers et al., Citation1993). Future research could investigate the potential interaction between housing status and sex differences in stress-related sleep deficits.
Future studies could also examine how other neural substrates of arousal (besides orexins) contribute to these sex-specific stress-induced sleep effects. Moreover, gonadal hormones should be considered as a factor affecting stress-induced sleep changes. Indeed, gonadal hormones are known to affect the stress response and have been reported to affect different measures of sleep (Corsi-Cabrera et al., Citation2000; Paul et al., Citation2009). Ultimately, it is crucial to explore how complex neurotransmitter and hormonal systems interact in the development of sex-specific symptoms in stress-related illnesses.
Disclosure statement
No potential conflict of interest was reported by the author(s).
Additional information
Funding
Notes on contributors
Andrew T. Gargiulo
Andrew Gargiulo (B.S. Millsaps College, 2014; Ph.D. Drexel University College of Medicine, 2019) is a postdoctoral fellow in the lab of Dr. Laura Grafe. Dr. Gargiulo’s postdoctoral work focuses on how stress changes the brain to affect cognition, appetite, and sleep.
Varuna Jasodanand
Varuna Jasodanand was an undergraduate at Bryn Mawr College (graduated in May 2020), double majoring in Psychology and Philosophy. She worked in the lab of Laura Grafe to better understand the effects of stress on sleep. She is now a research technician at Vanderbilt University.
Sandra Luz
Sandra Luz is a research technician at the Children’s Hospital of Philadelphia in the lab of Seema Bhatnagar.
Lauren O’Mara
Lauren O’Mara was an undergraduate at the University of Pennsylvania studying the biological basis of behavior (graduated in May 2019). She is now in medical school at Harvard.
Leszek Kubin
Leszek Kubin (MS Warsaw Polytechnic, 1976; PhD Warsaw School of Medicine, 1983) is a Research Professor of Physiology in the Department of Biomedical Sciences at the University of Pennsylvania. He studies the neurochemical mechanisms of REM sleep and sleep-related respiratory disorders.
Richard J. Ross
Richard J. Ross (BA Columbia University, 1968; MD/PhD Albert Einstein College of Medicine, 1975) is an Emeritus Professor of Psychiatry at the University of Pennsylvania. He is particularly interested in how sleep is disrupted in disorders such as Post-Traumatic Stress Disorder (PTSD).
Seema Bhatnagar
Seema Bhatnagar (BS McGill University, 1984; PhD McGill University, 1994) is a Professor of Anesthesiology and Critical Care at the University of Pennsylvania/Children’s Hospital of Philadelphia. She studies individual differences in resilience and vulnerability to chronic stress.
Laura A. Grafe
Laura Grafe (B.S. University of Maryland, 2008; Ph.D. University of Pennsylvania, 2014) is an Assistant Professor of Psychology at Bryn Mawr College and a behavioral neuroscientist. Her research program focuses on how stress affects the brain and behaviors relevant to mental health.
References
- Abdallah, C. G., Averill, L. A., Akiki, T. J., Raza, M., Averill, C. L., Gomaa, H., Adikey, A., & Krystal, J. H. (2019). The neurobiology and pharmacotherapy of posttraumatic stress disorder. Annual Review of Pharmacology and Toxicology, 59, 171–189. https://doi.org/10.1146/annurev-pharmtox-010818-021701
- Alexander, G. M., Rogan, S. C., Abbas, A. I., Armbruster, B. N., Pei, Y., Allen, J. A., Nonneman, R. J., Hartmann, J., Moy, S. S., Nicolelis, M. A., McNamara, J. O., & Roth, B. L. (2009). Remote control of neuronal activity in transgenic mice expressing evolved G protein-coupled receptors. Neuron, 63(1), 27–39. https://doi.org/10.1016/j.neuron.2009.06.014
- Amici, R., Zamboni, G., Perez, E., Jones, C. A., Toni, I. V. A. N., Culin, F., & Parmeggiani, P. L. (1994). Pattern of desynchronized sleep during deprivation and recovery induced in the rat by changes in ambient temperature. Journal of Sleep Research, 3(4), 250–256. https://doi.org/10.1111/j.1365-2869.1994.tb00139.x
- Bangasser, D. A., & Valentino, R. J. (2014). Sex differences in stress-related psychiatric disorders: Neurobiological perspectives. Frontiers in Neuroendocrinology, 35(3), 303–319. https://doi.org/10.1016/j.yfrne.2014.03.008
- Bangasser, D. A., & Wiersielis, K. R. (2018). Sex differences in stress responses: A critical role for corticotropin-releasing factor. Hormones, 17(1), 5–13. https://doi.org/10.1007/s42000-018-0002-z
- Brown, K. J., & Grunberg, N. E. (1995). Effect, of housing on male and female rats: Crowding stresses males but calms females. Physiology & Behavior, 58(6), 1085–1089. https://doi.org/https://doi.org/10.1016/0031-9384(95)02043-8
- Campbell, R. L., & Germain, A. (2016). Nightmares and posttraumatic stress disorder (PTSD. Current Sleep Medicine Reports, 2(2), 74–80. https://doi.org/10.1007/s40675-016-0037-0
- Cano, G., Mochizuki, T., & Saper, C. B. (2008). Neural circuitry of stress-induced insomnia in rats. The Journal of Neuroscience, 28(40), 10167–10184. https://doi.org/10.1523/JNEUROSCI.1809-08.2008
- Corsi-Cabrera, M., Sánchez, A. I., del-Río-Portilla, Y., Villanueva, Y., & Pérez-Garci, E. (2003). Effect of 38 h of total sleep deprivation on the waking EEG in women: Sex differences. International Journal of Psychophysiology, 50(3), 213–224. https://doi.org/10.1016/S0167-8760(03)00168-5
- Corsi-Cabrera, M., Ugalde, E., Del-Río-Portilla, Y., & Fernández-Guasti, A. (2000). Organizational and activational effects of gonadal steroid hormones on the EEG of male and female rats. Developmental Psychobiology, 37(3), 194–207. https://doi.org/10.1002/1098-2302(200011)37:3 < 194::aid-dev7 > 3.0.co;2-i
- DaSilva, J. K., Lei, Y., Madan, V., Mann, G. L., Ross, R. J., Tejani-Butt, S., & Morrison, A. R. (2011). Fear conditioning fragments REM sleep in stress-sensitive Wistar-Kyoto, but not Wistar, rats. Progress in Neuro-Psychopharmacology & Biological Psychiatry, 35(1), 67–73. https://doi.org/10.1016/j.pnpbp.2010.08.023
- Eacret, D., Grafe, L. A., Dobkin, J., Gotter, A. L., Renger, J. J., Winrow, C. J., & Bhatnagar, S. (2019). Orexin signaling during social defeat stress influences subsequent social interaction behaviour and recognition memory. Behavioural Brain Research, 356, 444–452. https://doi.org/10.1016/j.bbr.2018.05.032
- Ehlers, C. L., Kaneko, W. M., Owens, M. J., & Nemeroff, C. B. (1993). Effects of gender and social isolation on electroencephalogram and neuroendocrine parameters in rats. Biological Psychiatry, 33(5), 358–366. https://doi.org/10.1016/0006-3223(93)90325-8
- Farrell, M. S., & Roth, B. L. (2013). Pharmacosynthetics: Reimagining the pharmacogenetic approach. Brain Research, 1511, 6–20. https://doi.org/10.1016/j.brainres.2012.09.043
- Fuller, K. H., Waters, W. F., & Scott, O. (1994). An investigation of slow-wave sleep processes in chronic PTSD patients. Journal of Anxiety Disorders, 8(3), 227–236. https://doi.org/10.1016/0887-6185(94)90004-3
- Germain, A. (2013). Sleep disturbances as the hallmark of PTSD: Where are we now? The American Journal of Psychiatry, 170(4), 372–382. https://doi.org/10.1176/appi.ajp.2012.12040432
- Goel, N., Workman, J. L., Lee, T. T., Innala, L., & Viau, V. (2014). Sex differences in the HPA axis. Comprehensive Physiology, 4, 36.
- Grafe, L. A., & Bhatnagar, S. (2018). Orexins and stress. Frontiers in Neuroendocrinology, 51, 132–145. https://doi.org/10.1016/j.yfrne.2018.06.003
- Grafe, L. A., Cornfeld, A., Luz, S., Valentino, R., & Bhatnagar, S. (2017). Orexins mediate sex differences in the stress response and in cognitive flexibility. Biological Psychiatry, 81(8), 683–692. https://doi.org/10.1016/j.biopsych.2016.10.013
- Grafe, L. A., Eacret, D., Dobkin, J., & Bhatnagar, S. (2018). Reduced orexin system function contributes to resilience to repeated social stress. Eneuro, 5(2), https://doi.org/10.1523/ENEURO.0273-17.2018
- Grafe, L. A., Eacret, D., Luz, S., Gotter, A. L., Renger, J. J., Winrow, C. J., & Bhatnagar, S. (2017). Orexin 2 receptor regulation of the hypothalamic-pituitary-adrenal (HPA) response to acute and repeated stress. Neuroscience, 348, 313–323. https://doi.org/10.1016/j.neuroscience.2017.02.038
- Grafe, L. A., O’Mara, L., Branch, A., Dobkin, J., Luz, S., & Vigderman, A. (2020). Passive coping strategies during repeated social defeat are associated with long-lasting changes in sleep in rats. Frontiers in Systems Neuroscience, 14, 6. https://doi.org/10.3389/fnsys.2020.00006
- Habukawa, M., Uchimura, N., Maeda, M., Ogi, K., Hiejima, H., & Kakuma, T. (2018). Differences in rapid eye movement (REM) sleep abnormalities between posttraumatic stress disorder (PTSD) and major depressive disorder patients: REM interruption correlated with nightmare complaints in PTSD. Sleep Medicine, 43, 34–39. https://doi.org/10.1016/j.sleep.2017.10.012
- Johnson, P. L., Molosh, A., Fitz, S. D., Truitt, W. A., & Shekhar, A. (2012). Orexin, stress, and anxiety/panic states. Progress in Brain Research, 198, 133–161. https://doi.org/10.1016/B978-0-444-59489-1.00009-4
- Kobayashi, I., & Mellman, T. A. (2012). Gender differences in sleep during the aftermath of trauma and the development of posttraumatic stress disorder. Behavioral Sleep Medicine, 10(3), 180–190. https://doi.org/10.1080/15402002.2011.654296
- Mallampalli, M. P., & Carter, C. L. (2014). Exploring sex and gender differences in sleep health: A society for Women’s Health Research Report. Journal of Women’s Health, 23(7), 553–562. https://doi.org/10.1089/jwh.2014.4816
- Mellman, T. A., Bustamante, V., Fins, A. I., Pigeon, W. R., & Nolan, B. (2002). REM sleep and the early development of posttraumatic stress disorder. The American Journal of Psychiatry, 159(10), 1696–1701. https://doi.org/10.1176/appi.ajp.159.10.1696
- Mellman, T. A., Kobayashi, I., Lavela, J., Wilson, B., & Hall Brown, T. S. (2014). A relationship between REM sleep measures and the duration of posttraumatic stress disorder in a young adult urban minority population. Sleep, 37(8), 1321–1326. https://doi.org/10.5665/sleep.3922
- Mignot, E., Taheri, S., & Nishino, S. (2002). Sleeping with the hypothalamus: Emerging therapeutic targets for sleep disorders. Nature Neuroscience, 5(S11), 1071–1075. https://doi.org/10.1038/nn944
- Mong, J. A., & Cusmano, D. M. (2016). Sex differences in sleep: Impact of biological sex and sex steroids. Philosophical Transactions of the Royal Society of London. Series B, Biological Sciences, 371(1688), 20150110. https://doi.org/10.1098/rstb.2015.0110
- Pace-Schott, E. F., Germain, A., & Milad, M. R. (2015). Sleep and REM sleep disturbance in the pathophysiology of PTSD: The role of extinction memory. Biology of Mood & Anxiety Disorders, 5, 3. https://doi.org/10.1186/s13587-015-0018-9
- Paul, K. N., Losee-Olson, S., Pinckney, L., & Turek, F. W. (2009). The ability of stress to alter sleep in mice is sensitive to reproductive hormones. Brain Research, 1305, 74–85. https://doi.org/10.1016/j.brainres.2009.09.055
- Paxinos, G., & Watson, C. (1998). The rat brain in stereotaxic coordinates. San Diego: Academic Press.
- Ross, R. J., Ball, W. A., Sullivan, K. A., & Caroff, S. N. (1989). Sleep disturbance as the hallmark of posttraumatic stress disorder. American Journal of Psychiatry, 146(6), 697–707. https://doi.org/10.1176/ajp.146.6.697
- Sakurai, T. (2007). The neural circuit of orexin (hypocretin): Maintaining sleep and wakefulness. Nature Reviews. Neuroscience, 8(3), 171–181. https://doi.org/10.1038/nrn2092
- Spoormaker, V. I., & Montgomery, P. (2008). Disturbed sleep in post-traumatic stress disorder: Secondary symptom or core feature? Sleep Medicine Reviews, 12(3), 169–184. https://doi.org/10.1016/j.smrv.2007.08.008
- Whitaker, A. M., Gilpin, N. W., & Edwards, S. (2014). Animal models of post-traumatic stress disorder and recent neurobiological insights. Behavioural Pharmacology, 25(5–6), 398–409. https://doi.org/10.1097/FBP.0000000000000069
- Winsky-Sommerer, R., Boutrel, B., & De Lecea, L. (2003). The role of the hypocretinergic system in the integration of networks that dictate the states of arousal. Drug News & Perspectives, 16(8), 504–512. https://doi.org/10.1358/dnp.2003.16.8.829349
- Winsky-Sommerer, R., Boutrel, B., & de Lecea, L. (2005). Stress and arousal: The corticotrophin-releasing factor/hypocretin circuitry. Molecular Neurobiology, 32(3), 285–294. https://doi.org/10.1385/MN:32:3:285
- Xu, T. R., Yang, Y., Ward, R., Gao, L., & Liu, Y. (2013). Orexin receptors: Multi-functional therapeutic targets for sleeping disorders, eating disorders, drug addiction, cancers and other physiological disorders. Cellular Signalling, 25(12), 2413–2423. https://doi.org/10.1016/j.cellsig.2013.07.0
- Yehuda, R., Hoge, C. W., McFarlane, A. C., Vermetten, E., Lanius, R. A., Nievergelt, C. M., Hobfoll, S. E., Koenen, K. C., Neylan, T. C., & Hyman, S. E. (2015). Post-traumatic stress disorder. Nature Reviews. Disease Primers, 1, 15057. https://doi.org/10.1038/nrdp.2015.57
- Yu, B., Cui, S.-Y., Zhang, X.-Q., Cui, X.-Y., Li, S.-J., & Sheng, Z.-F. (2015). Mechanisms underlying footshock and psychological stress-induced abrupt awakening From posttraumatic “nightmares”. International Journal of Neuropsychopharmacology, 19(4), pyv113. doi:https://doi.org/ https://doi.org/10.1093/ijnp/pyv113
- Zadra, A. & Germain, (2009). Dreams and nightmares in PTSD. Encyclopedia of Neuroscience, 3, 655–661.
- Zeitzer, J. M. (2013). Control of sleep and wakefulness in health and disease. Progress in Molecular Biology and Translational Science, 119, 137–154. https://doi.org/10.1016/B978-0-12-396971-2.00006-3