Abstract
The brain is the key organ that orchestrates the stress response which translates to the retina. The retina is an extension of the brain and retinal symptoms in subjects with neurodegenerative diseases substantiated the eye as a window to the brain. The retina is used in this study to determine whether chronic stress reflects neurodegenerative signs indicative of neurodegenerative conditions. A three-year prospective cohort (n = 333; aged 46 ± 9 years) was stratified into stress-phenotype cases (n = 212) and controls (n = 121) by applying the Malan stress-phenotype index. Neurodegenerative risk markers included ischemia (astrocytic S100 calcium-binding protein B/S100B); 24-h blood pressure, proteomics; inflammation (tumor-necrosis-factor-α/TNF-α); neuronal damage (neuron-specific-enolase); anti-apoptosis of retinal-ganglion-cells (beta-nerve-growth-factor), astrocytic activity (glial-fibrillary-acidic-protein); hematocrit (viscosity) and retinal follow-up data [vessels; stress-optic-neuropathy]. Stress-optic-neuropathy risk was calculated from two indices: a newly derived diastolic-ocular-perfusion-pressure cut-point ≥68 mmHg relating to the stress-phenotype; combined with an established cup-to-disk ratio cut-point ≥0.3. Higher stress-optic-neuropathy (39% vs. 17%) and hypertension (73% vs. 16%) prevalence was observed in the stress-phenotype cases vs. controls. Elevated diastolic-ocular-perfusion-pressure, indicating hypoperfusion, was related to arterial narrowing and trend for ischemia increases in the stress-phenotype. Ischemia in the stress-phenotype at baseline, follow-up and three-year changes was related to consistent inflammation (TNF-α and cytokine-interleukin-17-receptor-A), neuron-specific-enolase increases, consistent apoptosis (chitinase-3-like protein 1, low beta-nerve-growth-factor), glial-fibrillary-acidic-protein decreases, elevated viscosity, vein widening as risk marker of endothelial dysfunction in the blood-retinal barrier, lower vein count, and elevated stress-optic-neuropathy. The stress-phenotype and related neurodegenerative signs of ongoing brain ischemia, apoptosis and endothelial dysfunction compromised blood–retinal barrier permeability and optic nerve integrity. In fact, the stress-phenotype could identify persons at high risk of neurodegeneration to indicate a neurodegenerative condition.
1. Introduction
Chronic stress was shown to alter brain activity and reduce neurovascular coupling in rodents (Berndt, Citation2014). The process of neurovascular coupling involves adjustment of blood flow in neural tissue in response to functional activity to meet increased metabolic demand (Berndt, Citation2014). Reduced neurovascular coupling was observed in the Malan chronic stress and stroke risk phenotype (hereafter stress-phenotype) (Malan & Malan, Citation2021). Moreover, the stress-phenotype reflected delayed retinal vaso-activity and -tone in response to low norepinephrine and hypothalamic–pituitary–adrenal axis (HPAA) functioning (Malan et al., Citation2021). Chen et al. (Citation2020) and Wei et al. (Citation2019) also demonstrated HPAA dysregulation in relation to neuro-ischemia, neuro-inflammation, and neurodegeneration. Clearly, humans with chronic stress-related disorders have a higher risk of developing vascular and structural neurodegenerative changes (Song et al., Citation2020). Several well-defined neurodegenerative conditions that affect the brain manifested in the eye, and ocular symptoms often preceded the clinical manifestation of a central nervous system disorder (London et al., Citation2013). Hence the retina, due to its shared origin and drainage with the cerebrovascular circulation, is of particular interest as a measure of cerebral vascular disease and neurodegeneration (Moss, Citation2015).
As the retina allows noninvasive assessment of the vasculature, it provides a window to the brain through which some mechanisms of neurodegeneration can be visualized. The human retina is the tissue with the highest metabolic demand (8%) in the body and an ischemic insult such as brain ischemia (Seidenfaden et al., Citation2021), or an inadequate blood supply, resulted in lower vessel count or rarefaction as observed in hypertensives (Bosch et al., Citation2017) and retinal hypoperfusion (Patel & Chih, Citation2017).
Currently, one of the most widely accepted clinical risk markers to identify brain or retinal ischemia is serum S100 calcium-binding protein B (S100B), a molecule found primarily in the cytoplasm of glial astrocytes (Seidenfaden et al., Citation2021). Nash et al. (Citation2008) suggested S100B as a significantly useful marker to assess acute ongoing brain ischemia and long-term clinical outcome. In eighteen studies with a total of 1,643 patients, S100B was also applied as a marker to monitor mild ongoing brain injury (Shulman, Citation2020). Cerebral ischemia was furthermore accompanied by a marked inflammatory reaction rendering the vascular blood-brain-barrier more permeable to solutes (Galea, Citation2021). Vascular leakage occurs earlier in retinal than in cortical tissues (Hui et al., Citation2017), and retinal vein widening as a sign of leakage was observed in blood-retinal barrier (BRB) breakdown (Gu et al., Citation2014) and ischemic stroke (Moss Citation2015). The BRB is composed of tight junctions between vascular endothelial cells (the inner BRB) forming the highly selective semi-permeable border or neurovascular-unit (Diaz-Coránguez et al., Citation2017). The defense strategy of the BRB is to maintain an immune-privileged site as it insulates the retina from circulating immune cells within the capillaries (Yang et al., Citation2020).
For the current investigation, the validated Malan stress-phenotype index (Malan & Malan, Citation2021) was applied, and the retina was used as a noninvasive measure to substantiate neurodegenerative risk. Furthermore, peripheral risk markers in the systemic circulation reflective of cerebral tissue damage, included proteomic analyses (Querol-Vilaseca et al., Citation2017); as well as three-year trajectories of ischemia (S100B) (Nash et al., Citation2008; Seidenfaden et al., Citation2021); neuron-specific-enolase (NSE) (Dichev et al., Citation2020); astrocytic activity or glial-fibrillary-acidic-protein expression (GFAP) (Sorci et al., Citation2010); beta-nerve-growth-factor (ß-NGF) as a protector of the retinal endothelium by inhibiting apoptosis (Troullinaki et al., Citation2019); glial-cell-line-derived-neurotrophic-factor (GDNF) regulating permeability of the BRB (Igarashi et al., Citation2000); and retinal data at 3-yr follow-up (Vilser, Citation2019). Our objectives were to 1) use the retina as noninvasive measure to substantiate neurodegenerative risk; and to 2) determine whether the stress-phenotype will reflect ocular and neurodegenerative signs to indicate a neurodegenerative condition.
2. Materials and methods
2.1. Study sample
A sub-sample of the Sympathetic activity and Ambulatory Blood Pressure in Africans (SABPA) prospective cohort study (Malan et al., Citation2015) was used for this investigation. The prospective cohort, aged 20–63 years, participated at baseline and three-year follow-up (2008–2012) ().
Figure 1. Participant selection criteria.
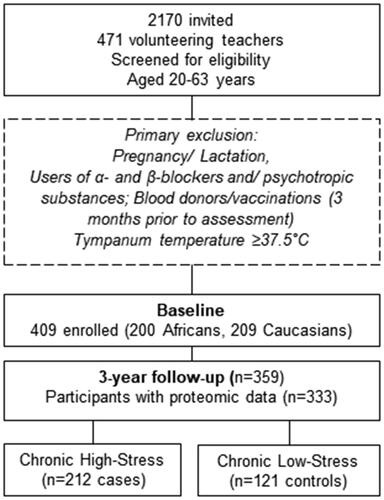
Ethical approval was obtained from the Health Research Ethics Committee (HREC) of the North-West University, Potchefstroom Campus (NWU-00036-07-S6) and complied with the Helsinki Declaration. Informed consent was obtained from the all the participants before the study began. The participant sample included 333 individuals having proteomic data points. The exclusion criteria for the SABPA study were pregnancy, lactation, users of α- and β-blockers or psychotropic substance usage, blood donors or vaccinations within 3 months prior to clinical assessment and a tympanum temperature ≥ 37.5 °C. Clinical data included cardiac risk marker measures namely ambulatory blood pressure assessments (Cardiotens CE120®. Meditech, Budapest Hungary); Cornell product left ventricular hypertrophy via a 12-lead resting ECG [(RaVL + SV3) *QRS duration (NORAV Medical Ltd PC 1200, Israel, Software version 5.030)]. Atherosclerotic burden (Supplemental text) was determined by carotid intima media screening and retinal risk data assessed by using the validated retinal vessel analyzer (Imedos Systems GmbH, Jena, Germany) (Vilser, Citation2019).
Assessments took place over two consecutive days at the overnight facilities of the Metabolic Unit Research Facility at North-West University. Each participant completed demographic and medical history questionnaires; received 24-h standardized diets and fasted from 22:00 (Malan et al., Citation2015). The next morning at 07:30, ambulatory apparatuses were disconnected and hereafter participants were in a semi-recumbent position for approximately 30–45 minutes before resting blood sampling commenced.
2.2. The Malan chronic stress and stroke risk phenotype (PCT/IB2020/057269)
Disturbed neural and stress hormone activity was related to oxygen perfusion deficits in the brain-heart axis (Malan & Malan, Citation2021; Malan et al., Citation1996, Citation2017, Citation2021). From this, the challenge emerged to identify an individual’s observable traits or phenotype to reflect a chronic stress and stroke risk index (hereafter stress-phenotype). This was achieved by construing a stress-phenotype that contains four biological markers, namely adrenocorticotrophic hormone, high-density lipoprotein, high-sensitivity cardiac troponin T, and diastolic blood pressure (DBP). The stress-phenotype is independent of age, ethnicity, or sex and demonstrated discriminatory ability for the positive prediction of stroke risk in two modules:
Module 1, chronic stress and ischemic heart disease-related stroke risk [AUC 0.77 (95% confidence interval: 0.72–0.82); 85% sensitivity/48% specificity]; and
Module 2, chronic stress and diabetes-related stroke risk [0.82 (95% confidence interval: 0.75–0.85); 85% sensitivity/58% specificity].
An algorithm was developed for web and mobile application to be utilized as a stress-phenotype screening tool in medical practice. Module 1 was applied for the current investigation and the four markers were determined with standardized methods (Malan et al., Citation2015), and not included in any statistical regression analysis models of the current investigation.
2.3. Retinal vessel assessment
Retinal vessel analyses were done at follow-up mostly in the right eye (Malan et al., Citation2015). Participants abstained from food or caffeine containing beverages, alcohol, smoking or exercise one hour prior to measurements. On arrival, they were introduced to the procedure by a trained registered nurse and screened for acute anterior angle chamber glaucoma risk with a small light source. Mydriasis was induced in the right eye of the participant by means of a drop containing tropicamide, 1% and benzalkonium chloride 0.01% (m/v). Fundus imaging was performed in a well-controlled light and temperature regulated room with the retinal vessel analyzer based on Zeiss fundus camera FF450+ with retinal vessel analysis Software VesselMap 2 Version 3.02 (Imedos Systems GmbH, Jena, Germany).
Retinal vessel diameter (arterioles and venules, hereafter arteries and veins) and count (rarefaction) data were determined by using monochrome images as well as the original color photographs for reference. First order vessels in the measuring zone located between 0.5 and 1.0 optic disk diameters from the margin or the optic disk were selected. Trunks in this area were counted and if not clear, the branches were counted. Branch measurements were declined if either of the branches could not be measured accurately. On average, between 7 and 17 arteries and an equal number of veins were measured. The Knudtson-Parr-Hubbard formula was used to standardize individual vessel calibers. Large vessels in the measuring area usually belong to the small arteries and veins of the microcirculation with vessel diameters between 40 and 300 µm (central artery or vein are the largest retinal vessels with diameters between 200 and 300 µm). Automated software calculations determined estimates from the 6 largest arteries and veins. As the image scale of each eye was unknown, the values of the retinal arteries and veins were expressed as measuring units (MU). 1 MU is equivalent to 1 micrometer when the dimensions of the eye being examined correspond to those of the normal Gullstrand eye. Reproducibility was computed for a randomly selected cohort with a correlation coefficient of 0.84.
2.4. Diastolic-ocular-perfusion-pressure as an indicator of hypoperfusion
Diastolic-ocular-perfusion-pressure (Supplemental text) as an indicator of perfusion changes (blood flow changes) reflects changes in vascular resistance and autoregulatory capacity (Vilser, Citation2019), and which could induce ischemia and/or hypoxia in the optic nerve head (Moore et al., Citation2008). Intra-ocular pressure was measured after a local anesthetic drop (Novasine Wander 0.4% Novartis) was instilled (Tono-Pen Avia Applanation Tonometer (Reichert 7-0908, ISO 9001, NY, USA). Diastolic-ocular perfusion-pressure (mmHg) was calculated and hypertensive/diabetic retinopathy was diagnosed by a registered ophthalmologist.
2.5. Biochemical analyses
Registered nurses obtained fasting blood samples from the ante-brachial vein with a sterile winged infusion set. Samples were handled according to standardized protocol and stored at −80 °C until analyses. Serum C-reactive protein (CRP) was measured with the ultra-high sensitivity turbidimetric method (Unicel DXC 800, Beckman and Coulter, Germany). Whole blood count analysis determined viscosity (hematocrit) with the Coulter AcT 5 diff Analyzer (Beckman Coulter, South Kraemer Boulevard Brea, CA, USA). Whole blood EDTA glycated hemoglobin (HbA1C) was determined with turbidimetric inhibition immunoassays (Cobas Integra 400 Plus, ROCHE Basel, Switzerland). Chronic stress was related to suppression of testosterone synthesis in rodent Leydig cells through mitochondrial damage (Xiong et al., Citation2022). Here, electrochemiluminescence immunoassays were used to analyze hair testosterone (Supplemental text) (ECLIA IBL-Hamburg, Germany), serum S100B and NSE (e411, ROCHE, Basel, Switzerland) with intra- and inter-assay coefficients of variation of less than 5%. Ongoing brain ischemia was determined at the acceptable cut point of S100B ≥ 0.1 μg/L (Seidenfaden et al., Citation2021). DuoSet ELISA kits from R&D Systems Inc. (Minneapolis, USA) were used for analyses of serum GFAP: catalogue #DY2594-05; intra- (3.96%) and inter-assay (1.63%); serum ß-NGF with a normal mean level of 194 ± 25 pg/ml (Serrano et al., Citation1996) catalogue #DY256; intra- (3.13%) and inter-assay (1.50%); and serum GDNF: catalogue #DY2594-05; intra- (3.96%) and inter-assay (1.63%). Serum tumor-necrosis-factor-α (TNF-α) values were determined with a quantikine high sensitivity human TNF-α enzyme-linked immunosorbent assay (HS ELISA; R&D Systems, Minneapolis, MN, USA). The inter- and intra-assay variability for TNF-α was 15% and 17.8%, respectively.
Proteomic Profiling: Plasma levels of proteins were analyzed by the Proximity Extension Assay (PEA) technique, PCR-based affinity proteomics technology, using the Proseek Multiplex CVD III 96 × 96 reagent kits (Olink Bioscience, Uppsala, Sweden) (Supplemental text). Mean intra-assay and inter-assay variations were 7% and 14% respectively.
2.6. Statistical analysis
Statistica version 13.3 (TIBCO Software Inc., Palo Alto, USA, 2021) and IBM® SPSS® statistics version 27 (2020) were used for data analyses. Proximity Extension Assay (PEA) proteins were log transformed whereas neurodegenerative risk markers S100B, NSE, GFAP, ß-NGF, GDNF and TNF-α were Box-Cox transformed before analyses.
Independent t-tests for continuous variables or Chi-square tests for binary variables were used to compare differences between baseline means an percentages in the stress-phenotype and controls. Dependent samples t-test were used to determine neurodegenerative risk marker changes over 3-years in the stress-phenotype and controls.
Logistic regression analyses were performed for each of the 89 proteins to identify which were significantly related to the stress-phenotype, adjusting for neurodegenerative risk markers (S100B, NSE, GFAP, ß-NGF, GDNF) at baseline. Univariate significance levels were Bonferroni-corrected (p < 0.05/94 = 5.5 × 10−4) for each set of logistic regression analyses. Subsequent logistic regression analysis to determine risk for prevalent and incident stress-phenotype related ischemia included the identified stress-phenotype related proteins as potentially confounding variables. Stress-phenotype related ischemic injury or risk was defined at the clinically accepted S100B ≥0.1 μg/L cut point (Seidenfaden et al., 2019). Additional potential confounders included neurodegenerative risk markers at baseline and 3-yr percentage changes in NSE, GFAP, ß-NGF, GDNF.
Decreased neurovascular coupling in the stress-phenotype (Malan et al., Citation2021) motivated determination of stress-ON risk (neuro = optic nerve integrity/cup-to-disk ratio, vascular = hypoperfusion). A hypoperfusion cut point, using a receiver-operating-characteristic (ROC) area-under-the-curve analysis, combined with the established cup-to-disk ratio cut-point ≥0.3 (Tsai & Forbes, Citation2004) will form an alternative way to screen for potential neurodegeneration related to stress.
Retinal vein widening has been considered as an endothelial dysfunction indicator in assessment of blood-brain-barrier hyperpermeability risk (Dumitrascu et al., Citation2018). Pearson pairwise correlation analyses were used to determine unadjusted correlations between baseline, follow-up ischemia (S100B) and retinal vein widening at baseline and follow-up in the stress-phenotype and controls.
Thereafter forward stepwise linear regression analyses were used to determine continuous neurodegenerative risk associations in several models at baseline, follow-up and 3-year changes (3-yr Δ%) in cases and controls. Percentage changes were determined as ((follow-up – baseline)/baseline) X 100. Dependent variables were 1) diastolic-ocular-perfusion-pressure at follow-up and 2) ischemia at baseline, follow-up and lastly 3-yr Δ%. Independent neurodegenerative risk markers included retinal vessels, vessel count, NSE, GFAP, ß-NGF, GDNF, and hematocrit, the respective retinal artery/vein caliber, identified dysregulated proteins and stress-ON. Stress-ON was omitted from hypoperfusion models to avoid collinearity. Fundus assessment was graphically presented and applied in a stress-phenotype case with ongoing ischemia. Forward stepwise regression analyses were repeated by adding age and sex as independent confounders in all regression models as it may influence the optic cup-to-disk ratio (Tsai & Forbes, Citation2004). Statistical significance was set at p ≤ 0.05 (two-tailed).
3. Results
In , the stress-phenotype showed significantly higher cardiac risk with medium to large effects in men (phi = 0.4), hair testosterone in men (d = 0.6), increased central obesity (phi = 0.3), low grade inflammation and prediabetes (phi = 0.3), atherosclerotic burden, ECG-LVH (phi = 0.5), cardiac myocyte injury (phi = 0.3), elevated hematocrit (phi = 0.4) and hypertension (phi = 0.5) (p ≤ 0.05) compared to controls. Regarding neurodegenerative risk (), TNF-α and retinal arterial narrowing (d = 0.3) and lower retinal arterial and vein count (d = 0.5) showed small to medium effects in the stress-phenotype compared to the controls.
Table 1. Comparing baseline clinical characteristics of the stress-phenotype vs. controls.
In , statistically significant neurodegenerative risk marker changes over 3-years with small effects were evident for the stress-phenotype. Increases were observed in S100B and NSE levels and decreases in GFAP and GDNF; whilst no changes were apparent in TNF-α and ß-NGF levels. TNF-α levels increased in the controls.
Table 2. Changes in neurodegenerative riskmarkers in the stress-phenotype and controls over three years.
By using logistic regression analysis, potential dysregulation of proteins was identified in the stress-phenotype at baseline (Tables S1–S4). Ongoing brain ischemia was defined as S100B ≥0.1 μg/L (Nash et al., Citation2008; Seidenfaden et al., Citation2021). In , proteins indicating prevalent (Model 1) and incident (Model 2) stress-related ongoing ischemia, are presented. Pro-inflammatory receptor IL-17RA showed consistently higher prevalence (OR = 2.6) and incidence (OR = 2.3) ongoing ischemia risk (Models 1 and 2). Three-year increases (3-yr Δ%) in NSE (OR = 1.4); IL-17RA (OR = 2.3) and CHI3L1 (OR = 1.7) were associated with incident ongoing ischemia risk (p < 0.05) in the stress-phenotype (Model 2). Model 2 showed good fit (p ≤ 1.00) with Hosmer Lemeshow at p = 0.91. Only IL-17RA and apoptotic CHI3L1 proteins indicated higher risk for dysregulation, which defined a stress-phenotype proteome.
Table 3. Multivariate logistic regression analysis was used to explore proteins significantly related to prevalent and incident risk of ongoing brain ischemia (S100B) in the stress-phenotype.
Table 4. Forward stepwise linear regression analyses depicting associations between *retinal hypoperfusion and neurodegenerative risk markers at follow-up considering 3-year changes (3-yr Δ %) in stress-phenotype cases.
illustrates a receiver-operating-characteristic (ROC) curve with an area-under-the-curve (AUC) [0.72 (0.67, 0.78), p ≤ 0.001] for a newly derived hypo-perfusion cut point of 68 mmHg in relation to the stress-phenotype (sensitivity 71%/specificity 64%).
Figure 2. Presenting a novel hypoperfusion cut point of 68 mmHg in relation to the stress-phenotype by using a receiver-operating-characteristic curve with AUC [0.72 (0.67, 0.78), p ≤ 0.001] (sensitivity 71%/specificity 64%).
![Figure 2. Presenting a novel hypoperfusion cut point of 68 mmHg in relation to the stress-phenotype by using a receiver-operating-characteristic curve with AUC [0.72 (0.67, 0.78), p ≤ 0.001] (sensitivity 71%/specificity 64%).](/cms/asset/c8ef543d-e5e0-4cee-83dc-880883865916/ists_a_2210687_f0002_c.jpg)
In , retinal hypoperfusion was related to arterial narrowing and trend for increasing ischemia over time (p ≤ 0.078).
Vein widening was correlated with both baseline S100B (r = 0.22; p = 0.003) and three-year follow-up S100B (p = 0.37, p ≤ 0.001) (data not shown).
In , established higher/out of range neurodegenerative risk signs in a stress-phenotype male case with ongoing ischemia, included 24h SBP 158/DBP 93 mmHg (24h hypertension 130 and/or 80 mmHg); less retinal veins (n = 7; range 7–17); S100B = 0.11 µg/l (brain injury S100B ≥ 0.1 µg/l); low ß-NGF = 16.7 pg/ml (healthy mean 194 ± 25pg/ml); NSE = 11.4 ng/ml (moderate cognitive impairment 11.4 ng/ml); stress-optic neuropathy risk, and stroke risk (arteriovenous nicking).
Figure 3. Illustrating assessment of retinal vessel diameter and count in a control eye (); and retinal neurodegenerative risk signs in a stress-phenotype case with ongoing ischemia (S100B ≥0.1μg/L) (). The blue V, indicates veins and the red A, indicates arteries.
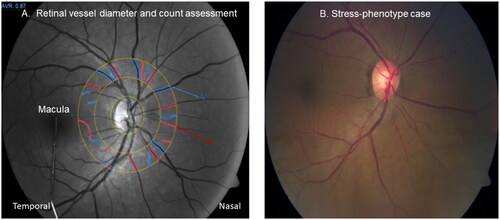
In , S100B levels at baseline, follow-up and 3-yr Δ% increases in the stress-phenotype were related to neurodegenerative risk signs (p ≤ 0.05). The stress-phenotype indicated ongoing ischemia as S100B levels increased from baseline to follow-up. Ongoing ischemia further reflected pro-inflammatory IL-17RA, or consistent TNF-α, increases in neuronal damage, decreases in astrocyte activity, consistent apoptosis (CHI3LI, low ß-NGF), elevated hematocrit, vein widening, low vein count, and stress-ON risk. Repeating regression analyses by using a cup-to-disk ratio cut point ≥4 index (Turgut, Citation2017) for determination of stress-ON, did not change the outcome. All three ischemic models in the controls were non-significant (Adjusted R2 values <0.10).
Table 5. Forward stepwise linear regression analyses depicting associations between S100B and neurodegenerative risk markers including retinal data, at baseline, follow-up and 3-year percentage changes (3-yr Δ (%) in the stress-phenotype (n = 160).
4. Discussion
The retina was used in this investigation to determine whether chronic stress will reflect eye and neurodegenerative signs to indicate a neurodegenerative condition. Several neurodegenerative signs in relation to the stress-phenotype were observed, including ongoing brain ischemia, apoptosis and endothelial dysfunction, which compromised blood-retinal barrier permeability and optic nerve integrity.
4.1. The stress-phenotype, hypoperfusion and ongoing brain ischemia
The stress-phenotype reflected hypoperfusion and elevated viscosity, which could alter blood flow and endothelial cell function through wall shear-stress (Gaehtgens, Citation1988). Shear-induced red blood cell release of ATP did increase endothelial cell intracellular Ca2+ activity, resulting in endothelial cell gap formation (Xu et al., Citation2017). Hypoperfusion may also induce ischemia and/or hypoxia via higher blood pressure and/or lower intraocular pressure to enhance arterial vascular resistance, and to reduce ocular blood flow to the optic nerve head (Hansen et al., Citation2012). Hence the higher blood pressure and diastolic-ocular-perfusion-pressure in the stress-phenotype supported the notion of ischemia and/or hypoxia effects, particularly in men with low testosterone (Malan et al., Citation2015). In support, abnormal intraocular pressure reflected abnormal intracranial pressure, which impeded cerebral blood flow to cause secondary ischemic insult (Marshall et al., Citation2015).
S100B is primarily synthesized by astrocyte end-feet processes, and ongoing brain ischemia will induce quick release of S100B in the circulation (Mori et al., Citation2008). S100B exerts regulatory effects on a relatively large number of cell types in an autocrine, paracrine and, possibly, endocrine manner. At micromolecular concentrations, circulating S100B may become pro-apoptotic and pro-inflammatory in the nervous and cardiovascular systems (Donato et al., Citation2009). Increased S100B concentrations also compromised brain tissue integrity and BRB permeability in the retinal vascular endothelium (Alexander et al., Citation2013). Seidenfaden et al. (Citation2021) used the S100B cut point of 0.1 μg/L as a marker of ongoing brain ischemia and mild traumatic brain injury. In our stress-phenotype group, a S100B mean of 0.1 μg/L resembled stress-related ongoing brain ischemia and non-traumatic brain injury as a secondary ischemic insult.
We could replicate findings from Seidenfaden et al. (Citation2021) as we demonstrated higher apoptotic (CHI3L1) odds for ongoing brain ischemia and non-traumatic brain injury incidence (OR = 1.7) in the novel stress-ischemia proteome. Our findings were further supported by another meta-analysis (Unden and Romner Citation2010) in pre-hospital and in-hospital patients (Seidenfaden et al., Citation2021) with the S100B ≥ 0.10 μg/L cut point showing sensitivity of 97% and 89–100%, respectively with CT-visual brain pathology. The stress-phenotype reflecting ongoing brain ischemia over 3-years infers ischemia in damaged astrocytes due to decreased GFAP expression (Zhang et al., Citation2017), implying its benefit as neurodegenerative marker. In another study, Wallensten et al. (Citation2022) could replicate raised S100B levels in relation to astrocytic apoptosis but not to self-reported chronic stress-induced disorders. Hence, our stress-phenotype and ongoing ischemia might rather explain the inflammatory homeostatic reflex responses.
4.2. Stress-phenotype and inflammation
Ongoing brain ischemia in the stress-phenotype was related to consistent inflammation with raised TNF-α and IL-17RA incidence risk. According to Zhong and Sun (Citation2022), central nervous system including retinal damage, induced pro-inflammatory gene activation with increased IL-17RA expression, heightened microglial activity and simultaneous higher IL-17 levels. Downstream IL-17 signaling activated production of the pro-inflammatory cytokine TNF-α (Kebir et al., Citation2007). In our stress-phenotype group, pro-inflammatory and apoptotic proteins of the chronic stress proteome were identified as neurodegenerative risk markers. Chronic stress might thus act as a trigger or ischemic insult to induce inflammation and central nervous system damage or apoptosis.
4.3. Stress-phenotype and apoptotic risk
Stress as an ischemic insult in the retina emerged, as ongoing brain ischemia and related apoptotic risk altered BRB permeability (Yang et al., Citation2020). In our stress-phenotype, ongoing brain ischemia reflected persistent inflammation and consistent apoptosis (low ß-NGF and CHI3L1) in relation to brain-ischemia incidence risk (Capsoni et al., Citation2010; Xiao et al., Citation2014; Zhao et al., Citation2020). The low ß-NGF mean of 114 pg/ml in the stress-phenotype considering the mean 194 pg/ml in normal healthy subjects (Serrano et al., Citation1996) exemplified higher stress-induced apoptotic risk. Beta-NGF, which possesses neurotrophic effects critical for neurite outgrowth, survival, and maintenance of neuronal-glia health, was not protective. Beta-NGF has two receptors, namely low affinity neurotrophic receptor (p75NTR) in glia cells and high affinity tyrosine kinase receptor (TrkA) in retinal-ganglion-cells (Rocco et al., Citation2018). In our stress-phenotype, ongoing ischemia and related stress-ON reflected less neurotrophic effects and apoptotic risk in retinal-ganglion-cells and the optic nerve. As consistent inflammation is apparent, increased vascular permeability in the BRB is suggested (Yang et al., Citation2020). Apoptotic risk with lower ß-NGF has previously been implicated in progressive neurodegeneration in a hypoxic retinal microvascular endothelium (Troullinaki et al., Citation2019) and ß-NGF was considered as therapeutic intervention. However, ongoing clinical trials for topical application of ß-NGF were hampered by side-effects linked to sensitization in the sensory and sympathetic systems (Rocco et al., Citation2018). Considering chronic stress in association with low ß-NGF is reasonable as similar findings were observed in major depressive disorder cases (Chen et al., Citation2015).
In support, non-enzymatic CHI3L1 was related to ongoing ischemia incidence risk in the stress-phenotype. As an acute phase reactant, it is secreted by immune and endothelial cells in response to consistent inflammation (Cekić et al., Citation2014). Chitinase 3-like-1 belongs to the glycoside hydrolase family 18 and was overexpressed in malignant gliomas cultured under stressed conditions, including hypoxia (Cekić et al., Citation2014). Ongoing brain ischemia in the stress-phenotype reflected increases in neuronal damage (NSE levels) with concomitant apoptotic risk, i.e., low ß-NGF and CHI3L1. This might underscore a stress-phenotype profile of structural neuronal-glia and vascular damage (Andrés-Benito et al., Citation2018; Troullinaki et al., Citation2019) revealing lower retinal artery and vein counts. Indeed, apoptotic CHI3L1 has previously been associated with retinal vessel rarefaction in diabetic retinopathy patients (Cekić et al., Citation2014) and in amyotrophic lateral sclerosis, a neurodegenerative disorder (Andrés-Benito et al., Citation2018).
Neuro-specific-enolase leakage from the neuronal cytoplasm (Haque et al., Citation2018) during structural damage of neuronal cells, will occur into the extracellular compartment and circulation, to be detected in serum after neuronal death secondary to brain injury (Dichev et al., Citation2020). Ongoing brain ischemia, or insult was related to neuronal damage in the stress-phenotype. This reflects clinical progression of neurodegeneration risk in the retina as an extension of the brain. An optimal NSE cut point of 11.4 ng/ml diagnosed moderate cognitive impairment (sensitivity: 92.9%, specificity: 47.1%) (Yu et al., Citation2020), which corresponds with the follow-up mean NSE levels of 11.4 ng/ml in the stress-phenotype. Concomitant 3-yr decreases in stress-related astrocyte activity (decreases in GFAP) and the neurotrophin GDNF with consistent inflammatory cytokine activity were observed. It is therefore viable that stress-related NSE may impair tissue restoration and disrupt BRB integrity (Michinaga & Koyama, Citation2019). As a biomarker of neuronal stress, NSE levels may therefore have prognostic potential for a variety of neurological disorders including stress-induced ongoing brain injury and retinal ischemia.
4.4. The stress-phenotype mirrored in the retina as a neurodegenerative condition
We propose a central neural mechanism to describe the stress-phenotype as a neurodegenerative condition: Elevated blood pressure and diastolic-ocular-perfusion-pressure in the stress-phenotype altered blood flow and narrowing of retinal arteries. Elevated viscosity and stress-induced hypoperfusion induced shear stress and resistance in retinal arteries (Gaehtgens, Citation1988). Shear stress augmented mitochondrial ATP generation and release, with Ca2+ signaling in vascular endothelial cells (Yamamoto et al., Citation2018). S100B as a calcium-binding protein is essential for the structural maintenance of tight junctions in BRB vascular endothelial cells (Krishnan et al., Citation2020). Stress-induced ongoing ischemia, inflammation and neuronal damage with increasing metabolic demands could alter BRB permeability via disruption of tight junctions (Michetti et al., Citation2019). Indeed, elevated S100B concentrations in the BRB of the stress-phenotype functionally enforced ischemic insult or injury to reflect neurodegenerative signs of apoptosis and endothelial dysfunction. Retinal vein branch widening, as hotspots of leakage in the superficial retina, were identified as signs of BRB breakdown (Gu et al., Citation2014). Stress-induced vein widening hereby reflected inability of endothelial cell mechanoreceptors (caveolae) to cope with mechanical stress or vascular loading. Pooling of blood resulted from continuous venous pulsations (Castillo et al., Citation2019). This was evidenced in the stress-phenotype midst HPA-axis hypoactivity, where retinal vein recovery responses were delayed upon photic stimulation (Malan et al., Citation2021). Whether stress-induced ischemia inferred both vein widening and/or collapse of veins and damage (lower vein count) near the optic nerve head, is unclear. If this holds true it might explain the higher prevalence of stress-ON (39% vs. 17% in controls) and potential glaucoma risk. A ticking-clock prognosis of the stress-phenotype as a progressive neurodegenerative condition was therefore reflected in the retina over a period of 3 years ().
Figure 4. Ticking-clock prognosis of the stress-phenotype as a progressive neurodegenerative condition. Abbreviation: BRB, blood-retinal barrier.
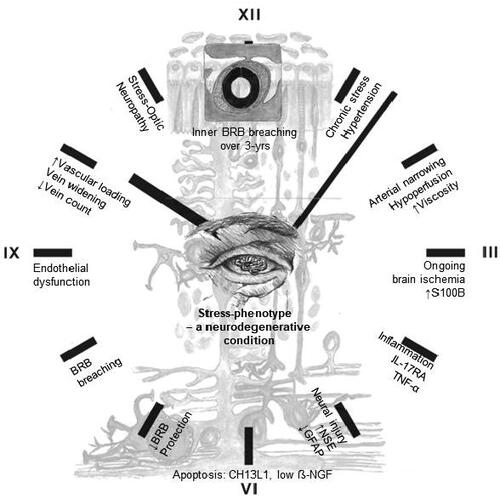
Our study findings should be expanded to larger prospective cohorts to evaluate BRB breaching. Homeostatic protective reflexes for retinal neuronal-glial cells have not been observed as decreases/downregulation in growth factor (GDNF) and astrocyte activity (GFAP) occurred. We suggest that retinal-glial stress hormones and growth factor thresholds might reset due to chronic stress prevalence, thereby debilitating protective reflexes.
To conclude, the stress-phenotype reflected ongoing ischemia, apoptosis and endothelial cell dysfunction, which compromised integrity of the BRB and optic nerve. In fact, the stress-phenotype may indicate a neurodegenerative condition. Future directions include a) optical coherence tomography assessment of rarefaction in the inner retina; and b) screening everyone to identify stress-phenotypes who are at a substantial risk of neurodegeneration, as neurodegenerative diseases are escalating (Castillo et al., Citation2019). Clinician and patient inertia could be overcome when recognizing the combination of chronic stress and stroke as potential risk for progressive neurodegeneration. This notion will support the proclamation issued by the World Stroke Organization focused on global brain and cardiovascular health, calling for the joint prevention of stroke and dementia (Hachinski et al., Citation2019).
Supplemental Material
Download MS Word (49.1 KB)Acknowledgements
This study would also not have been possible without all the individuals who voluntarily participated; and the collaborators sponsoring in-kind analyses.
Disclosure statement
No potential conflict of interest was reported by the author(s).
Correction Statement
This article has been corrected with minor changes. These changes do not impact the academic content of the article.
Additional information
Funding
Notes on contributors
Leoné Malan
Leoné Malan raised a family before she commenced with research in 2007. She aimed to describe a neurophysiological pathway linking emotional stress to microvascular dysregulation in the brain-heart axis. With this focus in mind, she designed the first psychophysiological prospective cohort study in Sub-Saharan Africa [Sympathetic activity and Ambulatory Blood pressure in Africans/SABPA], which received an international award for project design excellence. She and her husband, Nico Malan, developed a preventive screening tool to determine the risk for chronic stress and stroke to combat neurodegeneration. She delivered 41 Master’s and PhD students and co-authored >180 scientific publications.
Roelof van Wyk
Roelof van Wyk is a clinical ophthalmologist in private practice, located in Potchefstroom in the North-West Province, South Africa. His research interests are the retina and glaucoma. He is an anterior segment and posterior segment Ophthalmic surgeon. He is updated with the latest research and has membership with the European VitreoRetinal Society, the European Society of Retina Specialists and the Ophthalmological Society of South Africa.
Roland von Känel
Roland von Känel is a specialist in psychiatry and psychotherapy and in general internal medicine. Since 2018, he is Director of the Division of Consultant Liaison Psychiatry and Psychosomatic Medicine at the University Hospital Zurich and Chair of Consultant Liaison Psychiatry and Psychosomatic Medicine at the University of Zurich, Switzerland. His clinical focus is on behavioural cardiology and stress-related disorders. His research examines the psychobiological mechanisms linking psychological processes and physical illness, with a particular focus on cardiovascular disease. He has co-authored over 500 scientific articles and book chapters and co-edited two textbooks on psychosomatic medicine.
Tjalf Ziemssen
Tjalf Ziemssen is the Director of the Center of Clinical Neuroscience, and Head of the Autonomic Neuroendocrinological lab (ANF) and multiple sclerosis center. He has been involved with more than >140 interventional and non-interventional studies and delivered > 60 PhD and MD students. He received substantial funds for third-party projects and is the recipient of several awards. He has co-authored over 400 scientific articles.
Walthard Vilser
Dr-Ing Walthard Vilser, is an engineer and specialist in biomedical engineering. He patented, researched and developed with his team the Dynamic Retinal Vessel Analysis. He is the main founder of Imedos and was the managing director until he left the company two years ago. Today he is involved in research work of different international research groups on retinal vessel analysis and is lecturer at the Technical University of Ilmenau on the topic of retinal vessel analysis.
Peter M. Nilsson
Peter M. Nilsson is a Senior Professor of Clinical Cardiovascular Research and was previously the head of a research group in epidemiology and, for the past 25 years, former head of the Department for History of Medicine, Lund University, Sweden. He has been the acting Director for the Strategic Research Area (SRA) Epidemiolgy for Health (EpiHealth) between 2010 and 2019, linking many experts in epidemiology at the Lund and Uppsala universities in Sweden. He has expertise in diabetes, hypertension, insulin resistance, microbiota, epidemiology, and vascular dysfunction. He co-authored >700 scientific articles. In addition, he is a part-time senior consultant at a Clinical Research Department of Internal Medicine, Skåne University Hospital, Malmö, Sweden.
Martin Magnusson
Martin Magnusson is a professor and senior consultant in cardiology. Magnusson is PI of the Heart and Brain Failure Investigation study group. Magnusson is a clinically active researcher with special interest in heart failure research, cardiovascular complications to diabetes and predictors of cardiovascular and metabolic disease, e.g., genetic variants, (common and rare), metabolomics, proteomics and targeted biomarkers. He co-authored >92 scientific articles.
Amra Jujic
Amra Jujic is a postdoctoral fellow with her research focusing mainly on heart failure and cardiometabolic disease, with special interest in neuroendocrinology biomarkers.
Daniel W. Mak
Daniel W. Mak completed his PhD in 2019 in Health Sciences at the University of Witwatersrand, South Africa, which focused on hepatitis B virus (HBV), inflammation, hepatocelluar carcinoma and epidemiology in the sub-Saharan African setting. In 2021, he worked as a Postdoctoral Fellow at the University of Hong Kong wherein his research involved understanding induced pluripotent stem cell reprogramming using proteomics and bioinformatic tools.
Faans Steyn
Faans Steyn was a statistical consultant at the Statistical Consultation Services of the North-West University from 1974 to 2008. After retirement, he was appointed by the university in a part-time capacity for another 10 years. During that time, he was involved in epidemiological research and the validation process of the chronic stress and stroke risk phenotype. In his role as statistician, he has co-authored several publications in the field of stress research till date.
Nico T. Malan
Nico T. Malan was Director of the School for Physiology, Nutrition and Consumer Sciences (1991–2010) and in this time he was also Vice-Dean Faculty Health Sciences for 3 years at the North-West University, Potchefstroom South Africa. He became interested in the effect of stress on the health of especially African people in South Africa in 1988. In this regard, he was involved in several studies where the effect of urbanization on African people was studied. This evolved in the study of stress on the retinal and cardiac microvasculature and the role of stress hormones (catecholamines and cortisol) as well as sex hormones (testosterone and estradiol). He has >160 scientific publications.
References
- Alexander, J. S., Prouty, L., Tsunoda, I., Ganta, C. V., & Minagar, A. (2013). Venous endothelial injury in central nervous system diseases. BMC Medicine, 11, 1. https://doi.org/10.1186/1741-7015-11-219
- Andrés-Benito, P., Domínguez, R., Colomina, M. J., Llorens, F., Povedano, M., & Ferrer, I. (2018). YKL40 in sporadic amyotrophic lateral sclerosis: cerebrospinal fluid levels as a prognosis marker of disease progression. Aging, 10(9), 2367–13. https://doi.org/10.18632/aging.101551
- Berndt, J. D. (2014). Stress stops the blood from flowing. Science Signaling, 7(327), ec140. https://doi.org/10.1126/scisignal.2005510
- Bosch, A. J., Harazny, J. M., Kistner, I., Friedrich, S., Wojtkiewicz, J., & Schmieder, R. E. (2017). Retinal capillary rarefaction in patients with untreated mild-moderate hypertension. BMC Cardiovascular Disorders, 17(1), 300. https://doi.org/10.1186/s12872-017-0732-x
- Capsoni, S., Tiveron, C., Vignone, D., Amato, G., & Cattaneo, A. (2010). Dissecting the involvement of tropomyosin-related kinase A and p75 neurotrophin receptor signaling in NGF deficit-induced neurodegeneration. Proceedings of the National Academy of Sciences of the United States of America, 107(27), 12299–12304. https://doi.org/10.1073/pnas.1007181107
- Castillo, X., Castro-Obregón, S., Gutiérrez-Becker, B., Gutiérrez-Ospina, G., Karalis, N., Khalil, A. A., Lopez-Noguerola, J. S., Rodríguez, L. L., Martínez-Martínez, E., Perez-Cruz, C., Pérez-Velázquez, J., Piña, A. L., Rubio, K., García, H. P. S., Syeda, T., Vanoye-Carlo, A., Villringer, A., Winek, K., & Zille, M. (2019). Re-thinking the etiological framework of neurodegeneration. Frontiers in Neuroscience, 13, 728. https://doi.org/10.3389/fnins.2019.00728
- Cekić, S., Cvetković, T., Jovanović, I., Jovanović, P., Pesić, M., Stanković-Babić, G., Milenković, S., & Risimić, D. (2014). C-reactive protein and chitinase 3-like protein 1 as biomarkers of spatial redistribution of retinal blood vessels on digital retinal photography in patients with diabetic retinopathy. Bosnian Journal Basic Medical Science, 14(3), 177–184.
- Chen, J., Liu, X., & Zhong, Y. (2020). Interleukin-17A: the key cytokine in neurodegenerative diseases. Frontiers in Aging Neuroscience, 12, 566922. https://doi.org/10.3389/fnagi.2020.566922
- Chen, Y. W., Lin, P. Y., Tu, K. Y., Cheng, Y. S., Wu, C. K., & Tseng, P. T. (2015). Significantly lower nerve growth factor levels in patients with major depressive disorder than in healthy subjects: a meta-analysis and systematic review. Neuropsychiatric Disease and Treatment, 11, 925–933. https://doi.org/10.2147/NDT.S81432
- Diaz-Coránguez, M., Ramos, C., & Antonetti, D. A. (2017). The inner blood-retinal barrier: Cellular basis and development. Vision Research, 139, 123–137. https://doi.org/10.1016/j.visres.2017.05.009
- Dichev, V., Kazakova, M., & Sarafian, V. (2020). YKL-40 and neuron-specific enolase in neurodegeneration and neuroinflammation. Reviews in the Neurosciences, 31(5), 539–553. https://doi.org/10.1515/revneuro-2019-0100
- Donato, R., Sorci, G., Riuzzi, F., Arcuri, C., Bianchi, R., Brozzi, F., Tubaro, C., & Giambanco, I. (2009). S100B’s double life: Intracellular regulator and extracellular signal. Biochimica et Biophysica Acta, 1793(6), 1008–1022. https://doi.org/10.1016/j.bbamcr.2008.11.009
- Dumitrascu, O. M., Demaerschalk, B. M., Sanchez, C. V., Almader-Douglas, D., O’Carroll, C. B., Aguilar, M. I., Lyden, P. D., & Kumar, G. (2018). Retinal microvascular abnormalities as surrogate markers of cerebrovascular ischemic disease: A meta-analysis. Journal of Stroke and Cerebrovascular Diseases : The Official Journal of National Stroke Association, 27(7), 1960–1968. https://doi.org/10.1016/j.jstrokecerebrovasdis.2018.02.041
- Flammer, J., Konieczka, K., & Flammer, A. J. (2013). The primary vascular dysregulation syndrome: implications for eye diseases. EPMA Journal, 4(1), 14. https://doi.org/10.1186/1878-5085-4-14
- Gaehtgens, P. (1988). Microvascular flow disturbances: Rheological aspects. In: Manabe, H., Zweifach, B.W., Messmer, K. (eds) Microcirculation in Circulatory Disorders. Springer. 11–18. https://doi.org/10.1007/978-4-431-68078-9
- Galea, I. (2021). The blood–brain barrier in systemic infection and inflammation. Cellular & Molecular Immunology, 18(11), 2489–2501. https://doi.org/10.1038/s41423-021-00757-x
- Gu, X., Fliesler, S. J., Zhao, Y. Y., Stallcup, W. B., Cohen, A. W., & Elliott, M. H. (2014). Loss of caveolin-1 causes blood-retinal barrier breakdown, venous enlargement, and mural cell alteration. The American Journal of Pathology, 184(2), 541–555. https://doi.org/10.1016/j.ajpath.2013.10.022
- Hachinski, V., Einhäupl, K., Ganten, D., Alladi, S., Brayne, C., Stephan, B. C. M., Sweeney, M. D., Zlokovic, B., Iturria-Medina, Y., Iadecola, C., Nishimura, N., Schaffer, C. B., Whitehead, S. N., Black, S. E., Østergaard, L., Wardlaw, J., Greenberg, S., Friberg, L., Norrving, B., … Khachaturian, Z. S. (2019). Preventing dementia by preventing stroke: The Berlin Manifesto. Alzheimer’s & Dementia : The Journal of the Alzheimer’s Association, 15(7), 961–984. https://doi.org/10.1016/j.jalz.2019.06.001
- Hansen, G. L., Kofoed, P. K., Munch, I. C., Sillesen, H., Jensen, L. P., Iversen, H. K., & Larsen, M. (2012). Retinal angiographic blood flow us reduced in the ocular ischaemic syndrome. Danish Medicine, 60(10), A4716.
- Haque, A., Polcyn, R., Matzelle, D., & Banik, N. L. (2018). New insights into the role of neuron-specific enolase in neuro-inflammation, neurodegeneration, and neuroprotection. Brain Sciences, 8(2), 33. https://doi.org/10.3390/brainsci8020033
- Hui, F., Nguyen, C. T. O., He, Z., Vingrys, A. J., Gurrell, R., Fish, R. L., & Bui, B. V. (2017). Retinal and cortical blood flow dynamics following systemic blood-neural barrier disruption. Frontiers in Neuroscience, 11, 568. https://doi.org/10.3389/fnins.2017.00568
- Igarashi, Y., Chiba, H., Utsumi, H., Miyajima, H., Ishizaki, T., Gotoh, T., Kuwahara, K., Tobioka, H., Satoh, M., Mori, M., & Sawada, N. (2000). Expression of receptors for glial cell line-derived neurotrophic factor (GDNF) and neurturin in the inner blood-retinal barrier of rats. Cell Structure and Function, 25(4), 237–241. https://doi.org/10.1247/csf.25.237
- Kebir, H., Kreymborg, K., Ifergan, I., Dodelet-Devillers, A., Cayrol, R., Bernard, M., Giuliani, F., Arbour, N., Becher, B., & Prat, A. (2007). Human TH17 lymphocytes promote blood-brain barrier disruption and central nervous system inflammation. Nature Medicine, 13(10), 1173–1175. https://doi.org/10.1038/nm1651
- Krishnan, A., Wu, H., Venkataraman, V. (2020). Astrocytic S100B, blood-brain barrier and neurodegenerative diseases. In (Ed.), Glia in Health and Disease. Intech Open. 1–19.
- London, A., Benhar, I., & Schwartz, M. (2013). The retina as a window to the brain-from eye research to CNS disorders. Nature Reviews. Neurology, 9(1), 44–53. https://doi.org/10.1038/nrneurol.2012.227
- Malan, L., Hamer, M., Frasure-Smith, N., Steyn, H. S., & Malan, N. T. (2015). COHORT PROFILE: Sympathetic activity and ambulatory blood pressure in Africans (SABPA) prospective cohort study. International Journal of Epidemiology, 44(6), 1814–1822. https://doi.org/10.1093/ije/dyu199
- Malan, L., Hamer, M., von Känel, R., Kotliar, K., van Wyk, R. D., Lambert, G. W., Vilser, W., Ziemssen, T., Schlaich, M. P., Smith, W., Magnusson, M., Wentzel, A., Myburgh, C. E., Steyn, H. S., & Malan, N. T. (2021). Delayed retinal vein recovery responses reflect non-adaptation to Stress; and an increased stroke risk: the SABPA study. Cardiovascular Journal of Africa, 32(1), 5–16. https://doi.org/10.5830/CVJA-2020-031
- Malan, L., Hamer, M., von Känel, R., Lambert, G. W., Delport, R., Steyn, H. S., & Malan, N. T. (2017). Chronic defensiveness and neuroendocrine dysfunction reflect a novel cardiac troponin T cut point: the SABPA study. Psychoneuroendocrinology, 85, 20–27. https://doi.org/10.1016/j.psyneuen.2017.07.492
- Malan, L., Hamer, M., von Känel, R., van Wyk, R. D., Wentzel, A., Steyn, H. S., van Vuuren, P., & Malan, N. T. (2020). Retinal-glia ischemia and inflammation induced by chronic Stress: the SABPA study. Brain, Behavior Immunity - Health, 2, 100027. https://doi.org/10.1016/j.bbih.2019.100027
- Malan, L., & Malan, N. T. (2021). Inventors, north-west university, applicant: method of determining risk for chronic stress and stroke. World Patent Application WO 2021/019511 A1. https://patentscope.wipo.int/search/en/detail.jsf?docId=WO2021019511&_cid=P20-KYIGCT-90262-115
- Malan, N. T., Smith, W., von Känel, R., Hamer, M., Schutte, A. E., & Malan, L. (2015). Low serum testosterone and increased diastolic ocular perfusion pressure: a risk for retinal microvasculature. Vasa, 44(6), 435–443. https://doi.org/10.1024/0301-1526/a000466
- Malan, N. T., Brits, J. S., Eloff, F. C., Huisman, H. W., Kruger, A., Laubscher, P. J., Pretorius, P. J., & Steyn, H. S. (1996). The influence of acculturation on endocrine reactivity during acute stress in urban black males. Stress Medicine, 12(1), 55–63. https://doi.org/10.1002/(SICI)1099-1700(199601)12:1 < 55::AID-SMI683 >3.0.CO;2-8
- Marshall, S., Bayley, M., McCullagh, S., Velikonja, D., Berrigan, L., Ouchterlony, D., & Weegar, K,.; mTBI Expert Consensus Group. (2015). Updated clinical practice guidelines for concussion/mild traumatic brain injury and persistent symptoms. Brain Injury, 29(6), 688–700. https://doi.org/10.3109/02699052.2015.1004755
- Michetti, F., D’Ambrosi, N., Toesca, A., Puglisi, M. A., Serrano, A., Marchese, E., Corvino, V., & Geloso, M. C. (2019). The S100B story: from biomarker to active factor in neural injury. Journal of Neurochemistry, 148(2), 168–187. https://doi.org/10.1111/jnc.14574
- Michinaga, S., & Koyama, Y. (2019). Dual roles of astrocyte-derived factors in regulation of blood-brain barrier function after brain damage. International Journal of Molecular Sciences, 20(3), 571. https://doi.org/10.3390/ijms20030571
- Moore, D., Harris, A., Wudunn, D., Kheradiya, N., & Siesky, B. (2008). Dysfunctional regulation of ocular blood flow: A risk factor for glaucoma? Clinical Ophthalmology (Auckland, N.Z.), 2(4), 849–861. https://doi.org/10.2147/opth.s2774
- Mori, T., Tan, J., Arendash, G. W., Koyama, N., Nojima, Y., & Town, T. (2008). Overexpression of human S100B exacerbates brain damage and peri-infarct gliosis after permanent focal ischemia. Stroke, 39(7), 2114–2121. https://doi.org/10.1161/STROKEAHA.107.503821
- Moss, H. E. (2015). Retinal vascular changes are a marker for cerebral vascular diseases. Current Neurology and Neuroscience Reports, 15(7), 40. https://doi.org/10.1007/s11910-015-0561-1
- Nash, D. L., Bellolio, M. F., & Stead, L. G. (2008). S100 as a marker of acute brain ischemia: a systematic review. Neurocritical Care, 8(2), 301–307. https://doi.org/10.1007/s12028-007-9019-x
- Patel, G., & Chih, A. (2017). Hypoperfusion retinopathy. Federal Practioner, 2, 34(4), 42–45.
- Querol-Vilaseca, M., Colom-Cadena, M., Pegueroles, J., Martín-Paniello, C. S., Clarimon, J., Belbin, O., Fortea, J., & Lleó, A. (2017). YKL-40 (Chitinase 3-like I) is expressed in a subset of astrocytes in Alzheimer’s disease and other tauopathies. Journal Neuroinflammation, 14, 118. https://doi.org/10.1186/s12974-017-0893-7
- Rocco, M. L., Soligo, M., Manni, L., & Aloe, L. (2018). Nerve growth factor: Early studies and recent clinical trials. Current Neuropharmacology, 16(10), 1455–1465. https://doi.org/10.2174/1570159X16666180412092859
- Seidenfaden, S. C., Kjerulff, J. L., Juul, N., Kirkegaard, H., Møller, M. F., Münster, A. B., & Bøtker, M. T. (2021). Diagnostic accuracy of prehospital serum S100B and GFAP in patients with mild traumatic brain injury: a prospective observational multicenter cohort study – the PreTBI I study. Scandinavian Journal Trauma Resuscitation Emergency Medicine, 29, 75. https://doi.org/10.1186/s13049-021-00891-5
- Serrano, T., Lorigados, L. C., & Armenteros, S. (1996). Nerve growth factor levels in normal human sera. Neuroreport, 8(1), 179–181. https://doi.org/10.1097/00001756-199612200-00036
- Shulman, L. M. (2020). Emotional traumatic brain injury. Cognitive and Behavioral Neurology : official Journal of the Society for Behavioral and Cognitive Neurology, 33(4), 301–303. https://doi.org/10.1097/WNN.0000000000000243
- Song, H., Sieurin, J., Wirdefeldt, K., Pedersen, N. L., Almqvist, C., Larsson, H., Valdimarsdóttir, U. A., & Fang, F. (2020). Association of stress-related disorders with subsequent neurodegenerative diseases. JAMA Neurology, 77(6), 700–709. https://doi.org/10.1001/jamaneurol.2020.0117
- Sorci, G., Bianchi, R., Riuzzi, F., Tubaro, C., Arcuri, C., Giambanco, I., & Donato, R. (2010). S100B protein, a damage-associated molecular pattern protein in the brain and heart, and beyond. Cardiovascular Psychiatry Neurology, 2010, 1–13. https://doi.org/10.1155/2010/656481
- Tan, E. S., Chan, S. P., Xu, C. F., Yap, J., Richards, A. M., Ling, L. H., Sim, D., Jaufeerally, F., Yeo, D., Loh, S. Y., Ong, H. Y., Leong, K. T. G., Ng, T. P., Nyunt, S. Z., Feng, L., Okin, P., Lam, C. S., & Lim, T. W. (2019). Cornell product is an ECG marker of heart failure with preserved ejection fraction. Heart Asia, 11(1), e011108. https://doi.org/10.1136/heartasia-2018-011108
- Troullinaki, M., Alexaki, V. I., Mitroulis, I., Witt, A., Klotzsche-von Ameln, A., Chung, K. J., Chavakis, T., & Economopoulou, M. (2019). Nerve growth factor regulates endothelial cell survival and pathological retinal angiogenesis. Journal of Cellular and Molecular Medicine, 23(4), 2362–2371. https://doi.org/10.1111/jcmm.14002
- Tsai, J. C., & Forbes, M. (2004). Medical management of glaucoma. 2nd ed. Profess Communication. 111, 1508–1514.
- Turgut, B, Department of Ophthalmology, Faculty of Medicine, Yuksek Ihtisas University, Ankara, Turkey. (2017). Pearls for correct assessment of optic disc at glaucoma diagnosis. US Ophthalmic Review, 10(02), 104–110. https://doi.org/10.17925/USOR.2017.10.02.104
- Unden, J., & Romner, B. (2010). Can low serum levels of S100B predict normal CT findings after minor head injury in adults? An evidence-based review and meta-analysis. The Journal of Head Trauma Rehabilitation, 25(4), 228–240. https://doi.org/10.1097/HTR.0b013e3181e57e22
- Vilser, W. (2019). Method of Retinal Vessel Analysis in Retinal vessel analysis - a new method of diagnostics and risk prediction. (Ed Hanssen H, Vilser W) UNI-MED Verlag AG. 1st ed 2019, Ch 2:17–29; ISBN 978-3-8374-5576-2; Pp 64.
- Wallensten, J., Mobarrez, F., Åsberg, M., Borg, K., Beser, A., Wilczek, A., & Nager, A. (2022). Plasma levels of S100B and neurofilament light chain protein in stress-related mental disorders. Scientific Reports, 12(1), 8339. https://doi.org/10.1038/s41598-022-12287-1
- Wei, F., Song, J., Zhang, C., Lin, J., Xue, R., Shan, L. D., Gong, S., Zhang, G. X., Qin, Z. H., Xu, G. Y., & Wang, L. H. (2019). Chronic stress impairs the aquaporin-4-mediated glymphatic transport through glucocorticoid signaling. Psychopharmacology, 236(4), 1367–1384. https://doi.org/10.1007/s00213-018-5147-6
- Xiao, J., Wang, H., Wang, R., Thrimawithana, T., Little, P. J., Xu, J., Feng, Z.-P., & Zheng, W. (2014). The nerve growth factor signaling and its potential as therapeutic target for glaucoma. BioMed Research International, 2014, 759473. https://doi.org/10.1155/2014/759473
- Xiong, X., Wu, Q., Zhang, L., Gao, S., Li, R., Han, L., Fan, M., Wang, M., Liu, L., Wang, X., Zhang, C., Xin, Y., Li, Z., Huang, C., & Yang, Y. (2022). Chronic stress inhibits testosterone synthesis in Leydig cells through mitochondrial damage via Atp5a1. Journal of Cellular and Molecular Medicine, 26(2), 354–363. https://doi.org/10.1111/jcmm.17085
- Xu, S., Li, X., LaPenna, K. B., Yokota, S. D., Huke, S., & He, P. (2017). New insights into shear stress-induced endothelial signalling and barrier function: cell-free fluid versus blood flow. Cardiovascular Research, 113(5), 508–518. https://doi.org/10.1093/cvr/cvx021
- Yamamoto, K., Imamura, H., & Ando, J. (2018). Shear stress augments mitochondrial ATP generation that triggers ATP release and Ca2+ signaling in vascular endothelial cells. American Journal of Physiology. Heart and Circulatory Physiology, 315(5), H1477–H1485. https://doi.org/10.1152/ajpheart.00204.2018
- Yang, X., Yu, X. W., Zhang, D. D., & Fan, Z. G. (2020). Blood-retinal barrier as a converging pivot in understanding the initiation and development of retinal diseases. Chinese Medical Journal, 133(21), 2586–2594. https://doi.org/10.1097/CM9.0000000000001015
- Yu, Z. W., Liu, R., Li, X., Wang, Y., Fu, Y. H., Li, H. Y., Yuan, Y., & Gao, X. Y. (2020). High serum neuron-specific enolase level is associated with mild cognitive impairment in patients with diabetic retinopathy. Diabetes, Metabolic Syndrome and Obesity : targets and Therapy, 13, 1359–1365. https://doi.org/10.2147/DMSO.S249126
- Zhang, S., Wu, M., Peng, C., Zhao, G., & Gu, R. (2017). GFAP expression in injured astrocytes in rats. Experimental and Therapeutic Medicine, 14(3), 1905–1908. https://doi.org/10.3892/etm.2017.4760
- Zhao, T., Su, Z., Li, Y., Zhang, X., & You, Q. (2020). Chitinase-3 like-protein-1 function and its role in diseases. Signal Transduction and Targeted Therapy, 5(1), 201. https://doi.org/10.1038/s41392-020-00303-7
- Zhong, H., & Sun, X. (2022). Contribution of Interleukin-17A to Retinal Degenerative Diseases. Frontiers in Immunology, 13, 847937. https://doi.org/10.3389/fimmu.2022.847937