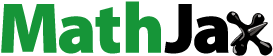
Abstract
Ingestion of L-theanine and L-tyrosine has been shown to reduce salivary stress biomarkers and improve aspects of cognitive performance in response to stress. However, there have been no studies to concurrently examine the impact of both L-theanine and L-tyrosine ingestion during a mental stress challenge (MSC) involving a brief cognitive challenge and a virtual reality based active shooter training drill. Thus, the purpose of this study was to determine the impact of ingestion of L-theanine and L-tyrosine on markers of stress and cognitive performance in response to a virtual reality active shooter drill and cognitive challenge. The cognitive challenge involved a Stroop challenge and mental arithmetic. Eighty subjects (age = 21 ± 2.6 yrs; male = 46; female = 34) were randomly assigned L-tyrosine (n = 28; 2000 mg), L-theanine (n = 25; 200 mg), or placebo (n = 27) prior to MSC exposure. Saliva samples, state-anxiety inventory (SAI) scales, and heart rate (HR) were collected before and after exposure to the MSC. Saliva was analyzed for stress markers α-amylase (sAA) and secretory immunoglobulin A (SIgA). The MSC resulted in significant increases in sAA, SIgA, HR, and SAI. Ingestion of L-theanine and L-tyrosine did not impact markers of stress. However, the L-tyrosine treatment demonstrated significantly lower missed responses compared to the placebo treatment group during the Stroop challenge. These data demonstrate that ingestion of L-theanine or L-tyrosine does not impact markers of stress in response to a MSC but may impact cognitive performance. This study was pre-registered as a clinical trial ("Impact of supplements on stress markers”: NCT05592561).
1. Introduction
Tactical operators (e.g. firefighters, military, law enforcement personnel) are involved in high-stress scenarios that require a high amount of physical strength and cognitive performance. During such scenarios, the sympathoadrenal and hypothalamic pituitary adrenal (HPA) axes are activated (i.e. fight or flight response) resulting in increased circulating concentrations of catecholamines (i.e. epinephrine, norepinephrine) increasing heart rate, respiratory rate, and blood pressure (Herman et al., Citation2016). Among law enforcement personnel, these conditions can result in increased anxiety, urgency, potentially impacting mental and physical performance (Stephenson et al., Citation2022). Chronic exposure to such stressors can adversely impact cardiometabolic health by increasing inflammation, and oxidative stress (McAllister et al., Citation2023; Soteriades et al., Citation2011). As such, more work is needed to examine methods that can be conducted to mitigate the physiological and psychological impact of acute stressors.
Dietary supplement use is common among tactical populations, with the goal typically being to improve vigilance, as well as physical and cognitive performance (Huang et al., Citation2006; Maughan et al., Citation2007). L-tyrosine is an amino acid and acts as a precursor to catecholamines (i.e. norepinephrine, epinephrine) (Stock et al., Citation2018; Wurtman et al., Citation1980) and can prevent a depletion of catecholamine stores resulting from excessive stress (Lieberman et al., Citation2015). L-tyrosine supplementation has been purported to alleviate the effects of stressors (Attipoe et al., Citation2015; Young, Citation2007) and appears to demonstrate favorable effects when exposed to high stress scenarios (Attipoe et al., Citation2015). Findings from a review suggested that L-tyrosine ingestion may produce beneficial effects when exposed to stressors (e.g. reduced blood pressure, improved cognitive performance) (Attipoe et al., Citation2015).
L-theanine supplementation has been shown to reduce the physiological impact of stressors among human participants (Hidese et al., Citation2019; Yamada et al., Citation2005; Yokogoshi et al., Citation1998; Yoto et al., Citation2012). Supplementation with L-theanine (∼200mg/day) has been shown to reduce heart rate and concentrations of salivary secretory immunoglobulin A (SIgA) in response to acute stress (Kimura et al., Citation2007). L-theanine has been shown suppress HPA axis activity, potentially due to its ability to cross the blood brain barrier, reduce glutamate release and increase γ-aminobutyric acid, glycine and dopamine activity in the brain (Levy & Tasker, Citation2012; Unno et al., Citation2013; Wang et al., Citation2022).
While past work has shown that that ingestion of L-tyrosine and L-theanine can reduce the effects of stress, more work is needed to better understand the acute impact of these supplements when exposed to a high stress environment. Based on past findings that L-tyrosine and L-theanine can reduce biomarkers of stress [e.g. SIgA and α-amylase (sAA)] and improve aspects of cognitive performance, we hypothesized that ingestion of these amino acids reduce biomarkers of stress and improve markers of cognitive performance and after participating in a virtual reality based active shooter training drill (VR-ASD). Thus, the purpose of this study was to examine the impact of acute ingestion of L-theanine, L-tyrosine, with respect to salivary and subjective markers of stress. A secondary aim was to investigate the impact of supplementation on markers of cognitive performance.
2. Material and methods
2.1. Subjects and experimental design
This study utilized a randomized, single- blinded, between subjects, parallel experimental design. Subjects were blinded and randomly assigned to ingest either L-tyrosine (n = 28), L-theanine (n = 25) or a flavor matched placebo (n = 27) prior to being exposed to a mental stress challenge (MSC) that included a VR-ASD and a cognitive challenge. The treatment randomization is shown in . Note, since this was part of a larger clinical trial (NCT05592561) the data for the placebo condition were included in a previous study conducted by our team investigating the effects of caffeine ingestion (McAllister et al., Citation2024). The cognitive challenge was conducted for four minutes and occurred both before and after the VR-ASD which lasted approximately 2 minutes. Thus, the total duration for the MSC was ∼10 min. Prior to involvement, subjects provided electronic informed consent and completed a health history questionnaire. Subjects were recruited from university campus in the age range of 18-40 and were required to be free from: 1) any known cardiovascular or metabolic diseases, 2) any significant stressors (divorce, passing in the family or workplace problems) in the past 30 days, 3) history of vertigo, epilepsy, or a brain injury. In addition, subjects were asked to arrive fasted (no food or dietary supplement; caffeine intake) for ≥4 hours and to avoid intense exercise and any drug or alcohol use within 24 hours of testing. Testing occurred between the early and late afternoon (∼12:00-17:30). All procedures were approved by the University’s Institutional Review Board. This study has been pre-registered as a clinical trial (NCT05592561).
2.2. Experimental procedures
Once subjects arrived, body mass and height were measured using a digital scale (Rice Lake Weighing Systems, Rice Lake, WI, USA) and a stadiometer (213; SECA, CA, USA). Subsequently, subjects were given a water bottle and were asked to mouth rinse and rest quietly for 10 min before the first saliva sample. During this time wait period, participants were fitted with a heart rate (HR) monitor (Polar verity sense; Polar Electro Ltd, Kempele, Finland). Upon completion of the 10 min rest period, subjects provided an initial baseline saliva sample via passive drool method (45 min prior to the MSC). Subjects ingested their assigned supplement beverage 40 min prior to the MSC. During this wait time, subjects were asked to rest quietly and were allowed to use their mobile devices or read a book, and were instructed to not speak to each other if more than one person was present in the waiting room. Concurrent with each saliva sample, HR and state-anxiety inventory (SAI) measures were collected. There was a total of 4 timepoints during which a saliva sample, HR and SAI measures were collected: 1) 45 minutes before testing, 2) immediately before the MSC, 3) immediately after the MSC, and 4) 30 minutes after the MSC. An overview of the procedures is shown in .
Figure 2. Overview of experimental procedures. Note, the figure was adopted from our past work (McAllister et al., Citation2024).
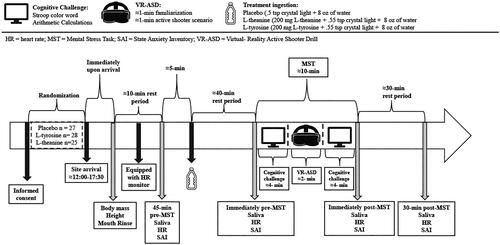
2.2.1. Mental stress challenge: cognitive challenge & VR-ASD
The MSC for this study was previously performed by our team (McAllister et al., Citation2024). The cognitive challenge portion included an altered version of the Stroop challenge and mental arithmetic calculations, which lasted 2 minutes each and were displayed on e-Prime 2 software (Psychology Software Tools, Inc., Pittsburgh, PA, USA). Immediately after, subjects performed the 2 min VR- ASD, followed by the same cognitive challenges with different questions. Thus, the entire MSC lasted ∼10 minutes.
2.2.2. Stroop challenge
For the Stroop challenge, subjects were in front of a computer monitor that displayed a word (yellow, green, red or blue) for .5 seconds. However, those words were presented with a conflicting-color font, which presented a mental challenge (e.g. the word yellow displayed with a blue font). The subjects had to quickly respond by pressing the correct font color on a color-coordinated keypad. Response times and decision accuracy were recorded. Note, the first ∼1 min was set up as a practice trial and not included in statistical analysis in an attempt to minimize learning effects.
2.2.3. Arithmetic calculations
The mathematical problems contained addition and subtraction of either single, double, or triple-digit numbers (e.g. 501 minus 27). The subject was allowed 10 seconds to respond, there was an automated buzzer if the answer was wrong or if the subject took too long. The arithmetic calculations portion of the MSC was performed immediately after the Stroop test.
2.3. Virtual reality active shooter drill
The VR-ASD was the same protocol as mentioned earlier (McAllister et al., Citation2024). In more detail, the subject was the responding officer on the scene of an active shooter. As the law enforcement officer, subjects walked through the initial door and heard screaming and sirens. One victim was on the ground immediately past the initial door, and a second victim ran out of a room on the left. The subject then proceeded to the room where the second victim ran from. Upon turning the corner and entering the room, the subject determined which person was the suspect and “shot” him with a virtual reality training weapon. Once the shooter fell after being shot, the VR-ASD scenario ended. The VR-ASD was presented using HTC VIVE Pro VR equipment (HTC Corp, New Taipei, Taiwan) and was developed by Street Smarts VR (streetsmartsvr.com). The VR laboratory environment was larger than the virtual environment (∼35 ft x 20 ft); making it easier for subjects to move around without disruption of the drill.
2.4. State-anxiety inventory assessment
To assess self-reported stress levels, a validated SAI with six statements was used at four different points of the testing duration. These were given at the same time the saliva test tube was administered along with the recording of HR. The statements included; “I feel uneasy” or “I feel calm” or “I am worried.” Subjects responded on a scale of 1-4 (1 signifying the least agreement with the statement and 4 signifying the greatest agreement). The SAI has been shown to be reliable and accurate (Marteau & Bekker, Citation1992; Tluczek, Henriques, & Brown, Citation2009).
2.5. Supplement ingestion
L-Theanine and L-tyrosine powder was purchased from Bulk Supplements (Hard Eight Nutrition, LLC, dba, Bulk Supplements, Henderson, NV). The L-theanine (200 mg) and L-tyrosine (2000 mg) powders were mixed with crystal light (a zero calorie powdered drink mix that is artificially flavored). Specific quantities used were determined through pilot testing to ensure consistent flavor and sweetness prior to experimental testing. The 200 mg L-theanine was mixed with 0.55 tsp of crystal light and 8 oz of water. The 2000 mg of L-tyrosine was mixed with 0.625 tsp of crystal light and 8 oz of water, and the placebo included 0.5 tsp of crystal light and 8 oz of water. The subjects were required to ingest within a two-minute period. These dosages were based off assessments from previous work showing significant effects among humans (Colzato et al., Citation2013; Deijen et al., Citation1999; Kimura et al., Citation2007; Unno et al., Citation2013). Regarding the supplement ingestion period (40 min prior to the initial exposure to the stressor), it should be noted that plasma concentrations of L-theanine and L-tyrsoine have been shown to peak and/or impact other plasma analytes within 45–90 min after ingestion (Rasmussen et al., Citation1983; Scheid et al., Citation2012; Van de Rest et al., Citation2017).
2.6. Saliva sample collection and analysis
Saliva samples were collected via passive drool technique utilizing a mouthpiece adaptor attached to the collection vial (Salimetrics, PA, USA). Saliva was collected at the following timepoints: 1) 45-minutes before the test, 2) immediately before, 3) right after, and 4) 30-minutes after the MSC. To optimize collection, subjects were told to start ‘pooling’ the saliva at the front of their mouth five minutes prior to saliva collection. This is necessary because participants were asked to tilt the head forward (either sitting or standing) and passively drool inside the test tube, limiting the bubbles in the sample. Participants were encouraged to produce approximately 1.0 mL of saliva per each collection timepoint. The saliva samples were immediately frozen at −80 °C and stored until subsequent analysis. Saliva samples were shipped overnight on dry ice for analysis of concentrations of SIgA and sAA at a commercial laboratory (Salimetrics, PA, USA). Samples were analyzed in duplicate using commercially available kits (Salimetrics, PA, USA). The intra-assay and inter-assay %CV for these assays was < 8.8%.
2.7. Statistical analysis
All statistical data procedures conducted using SAS v 9.4 (Cary, NC, USA). To examine potential changes across time and between treatments, variables sAA, SIgA, HR, and SAI were analyzed via 3 4 (treatment
timepoint) factorial analysis of variance (ANOVA) – these were the primary analyses of this study. A post-hoc power analysis of these data indicated the study’s sample size (n = 80) was sufficient and achieved a statistical power of 0.94 to detect the medium main effects presented in the results (G*Power 3.1.9.7). Regarding mental challenge data, to examine changes across time (pre-and post-MSC) and between treatments, 3
2 (treatment x timepoint) factorial ANOVAs were conducted. These were conducted for both Stroop and mental arithmetic including the following outcome measures: correct responses, missed responses, and response time. In the instance of a significant main effect or interaction (p < 0.05), Fisher’s Least Significant Difference test was conducted to compare means. No corrections were done as related to multiple comparisons. It is worth noting that analyses of the MSC were secondary to the objective and subjective markers of acute stress. As with the stress marker analyses, we utilized the resulting effect sizes to check for power post-hoc. The MSC analyses yielded adequate statistical power (0.82) to detect the medium-sized main effects presented. Effect size was calculated and reported as partial eta squared (ηp2). Statistical significance was set a priori at p < 0.05.
3. Results
A total of 80 subjects (male = 46; female = 34; height: 171.5 ± 9.8 cm; mass: 79.5 ± 18.4 kg; age = 21 ± 2.61 yrs.) finished the testing procedures. Subject demographics by treatment group are demonstrated in . The subjects were asked to self-report activity levels (exercise at least three times per week = 71; No = 9), if they used any nicotine products (No = 73; Yes = 7), and if they ingested caffeine (e.g. coffee, pre-workout, soda) on a regular basis (Yes = 41; No = 39). Data are shown as mean ± standard deviation unless otherwise noted.
Table 1. Subject descriptive characteristics
3.1. Salivary α-amylase
Regarding mean sAA concentrations, no significant treatment time interaction was noted (F = 0.49, p = 0.81, ηp2 = 0.00). However, there was a main effect for treatment (F = 27.57, p < 0.001, ηp2 = 0.19) and time (F = 5.37, p = 0.001, ηp2 = 0.06). Post hoc analysis indicated significantly higher sAA concentrations in the L-tyrosine group compared to L-theanine (p < 0.001) and placebo (p < 0.001) treatment groups. In addition, mean sAA concentrations were also significantly greater in the L-theanine treatment compared to the placebo (p = 0.001) treatment group. With respect to changes across time, there was no change from −45 to immediately pre MSC; however, there was a significant increase from pre to post MSC (p < 0.001). A significant decrease was noted in sAA concentrations from immediately post to 30 min post MSC (p = 0.001). Since there was no treatment
time interaction, results indicate that supplementation did not impact mean sAA responses to the MSC. Mean sAA concentrations are shown in .
Figure 3. Changes in salivary α-amylase (sAA) concentrations across time and between treatments. -45 = 45 min pre MSC, pre = immediately pre MSC, post: immediately post MSC, +30 = 30 min post MSC. θ Indicates higher sAA concentrations in L-tyrosine compared to both L-theanine (p < 0.001) and placebo (p < 0.001), and higher sAA concentrations in the L-theanine group compared to placebo (p < 0.001). *Indicates significantly higher sAA concentrations compared to pre and 30 min post MSC (p < 0.001).
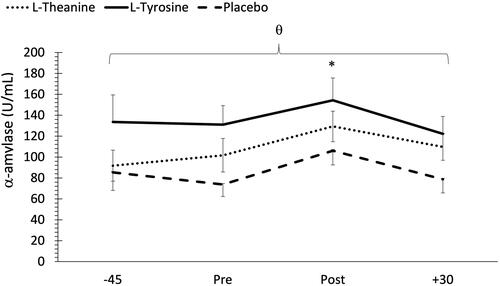
3.2. Secretory immunoglobulin A
No significant treatment time interaction was noted for mean SIgA concentrations (F = 0.24, p = 0.96, ηp2 = 0.00). However, there was a main effect for treatment (F = 5.64, p = 0.004 ηp2 = 0.04) and time (F = 35.59, p < 0.001, ηp2 = 0.32). Post hoc analysis demonstrated significantly lower SIgA concentrations in the L-tyrosine treatment group compared to placebo (p < 0.001). In terms of changes across time, a significant decrease in SIgA concentrations was noted from −45 to immediately pre MSC (p = 0.004). Mean SIgA concentrations increased significantly from pre to post MSC (p < 0.001). In fact, SIgA concentrations immediately post MSC were significantly higher than all other timepoints (p < 0.001). Since there was no treatment
time interaction, results indicate that supplementation did not impact mean SIgA responses to the MSC. Mean SIgA concentrations are shown in .
Figure 4. Changes in secretory immunoglobuin A (SIgA) concentrations across time and between treatments. -45 = 45 min pre MSC, pre: immediately pre MSC, post: immediately post MSC, +30 = 30 min post MSC. θ Indicates significantly lower SIgA concentrations in the L-tyrosine group compared to placebo (p < 0.001). *Indicates a significant increase in SIgA post MSC compared to all other timepoints (p < 0.001).
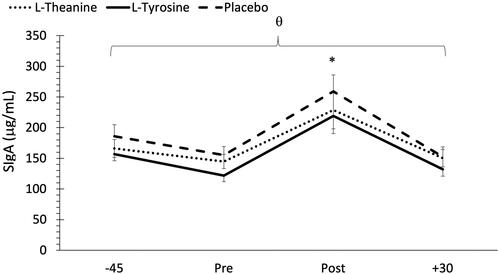
3.3. Heart rate and state anxiety inventory
No significant treatment time interaction was noted for mean HR (F = 1.03, p = 0.40, ηp2 = 0.02). However, a main effect for treatment and time was noted (F = 11.58, p < 0.001, ηp2 = 0.09 &, ηp2 = 0.13 respectively). Mean HR was higher in the L-theanine treatment group compared to placebo (p < 0.001) and L-tyrosine (p = 0.03) groups. In addition, mean HR was higher in the L-tyrosine group compared to placebo (p = 0.006). With respect to changes across time, no change in HR was noted from −45 to pre MSC (p > 0.05). However, a significant increase was found from pre to post MSC (p = 0.004). In fact, HR was significantly higher immediately post-MSC compared to all other timepoints (p < 0.05). Since there was no treatment
time interaction, results indicate that supplementation did not impact mean HR responses to the MSC. Mean HR data are shown in .
Figure 5. a: Changes in heart rate (HR) across time and between treatments. θ Indicates significantly higher HR in the L-theanine group compared to the placebo (p < 0.001) and L-tyrosine (p = 0.03) groups. *Indicates a significantly higher HR post MSC compared to all other timepoints (p < 0.05). : Changes in state anxiety inventory (SAI) across time and between treatments. θ Indicates SAI values were significantly different in all treatment groups, with the lowest levels in the placebo treatment, and the highest levels in the L-tyrosine treatment. *Indicates a significant increase in mean SAI values post MSC compared to all other timepoints (p < 0.05).
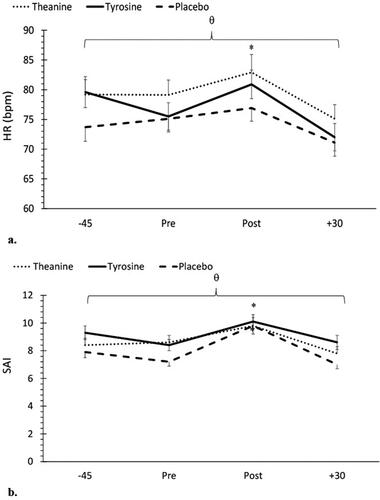
With respect to SAI, no significant treatment time interaction was noted for mean SAI (F = 1.33, p = 0.24, ηp2 = 0.03). However, a main effect for treatment (F = 11.5, p < 0.001, ηp2 = 0.09) and time (F = 24.41., p < 0.001, ηp2 = 0.24) was found. Mean SAI values were significantly different in all treatment groups with the lowest levels in the placebo treatment, and the highest levels in the L-tyrosine treatment. More specifically, mean SAI levels were higher in the L-tyrosine treatment group compared to both L-theanine (p = 0.04) and placebo (p < 0.001) groups. Mean SAI levels were significantly lower in the placebo treatment compared to both L-theanine (p = 0.009) and L-tyrosine (p < 0.001) treatments. In addition, SAI levels in the L-theanine treatment were significantly higher than placebo (p = 0.009) treatment. With respect to changes across time, SAI values immediately post MSC were significantly higher compared to all other timepoints (p < 0.05). SAI values at 30 min post MSC were significantly lower than mean SAI at −45 and immediately post MSC (p < 0.05). Since there was no treatment
time interaction, results indicate that supplementation did not impact mean SAI responses to the MSC. Mean SAI values are shown in .
3.4. Mental stress challenge data: stroop
All mental challenge data are reported in . Regarding Stroop performance, it should be noted that three outliers were removed as their data were extreme outliers (i.e. >3SD from the mean). No treatment time interaction was found for Stroop correct responses (F = 0.30, p = 0.73, ηp2 = 0.00). The main effect for treatment approached significance (F = 3.07, p = 0.05, ηp2 = 0.07) with the highest correct responses in the L-tyrosine treatment. There was a main effect for time (F = 8.9, p = 0.003, ηp2 = 0.11) with significantly higher correct responses noted post-VR-ASD compared to pre-VR-ASD (p = 0.003). With respect to missed responses, no treatment
time interaction was noted (F = 0.77, p = 0.46, ηp2 = 0.02). The main effect for time approached significance (F = 3.28, p = 0.07, ηp2 =0.04). Finally, a main effect for treatment was found (F = 3.75, p = 0.02, ηp2 = 0.09) with significantly lower missed responses in the L-tyrosine condition compared to placebo (p = 0.007). In terms of response time, no treatment x time interaction or main effect for treatment or time was found (p > 0.05); however, the main effect for treatment approached significance (F = 2.51, p = 0.08, ηp2 = 0.06) with the highest overall response time noted in the L-tyrosine condition.
Table 2. Mental challenge data (Stroop plus mental arithmetic challenge) for L-theanine, L-tyrosine and placebo groups presented in mean values ± standard deviation, respectively.
3.5. Mental stress challenge data: mental arithmetic
Regarding mental arithmetic, no treatment time interaction, or main effect for time or treatment was found for any variable (correct, missed responses and response time) (p > 0.05). However, the treatment x time interaction approached significance with correct responses (p = 0.08, ηp2 = 0.06). From pre- to post-VR-ASD, the placebo treatment decreased by 8%, whereas the L-theanine and L-tyrosine groups increased by 7% and 2.5%. However, this change was not significant (p = 0.08).
4. Discussion
The main findings of this study demonstrate that the MSC resulted in significant increases in all stress measures (sAA, SIgA, HR, and SAI). While between-group differences existed for these variables, they were not impacted by ingestion of either L-theanine or L-tyrosine, as no significant treatment time interactions were found. Regarding cognitive performance, the L-tyrosine treatment demonstrated significantly lower missed Stroop responses compared to the placebo treatment. While the L-tyrosine treatment demonstrated higher correct Stroop responses, the difference was not significant (p = 0.05). Since these data were collected ∼40 min after supplement ingestion, it is possible that ingestion of L-tyrosine contributed to improvements to markers of cognitive performance as previously shown in past research (Attipoe et al., Citation2015; Bloemendaal et al., Citation2018; Deijen et al., Citation1999; Deijen & Orlebeke, Citation1994; Wang et al., Citation2022). However, it is also possible that these findings are explained by between group differences that are not attributed to supplement ingestion.
The present data are in line with past work that reported increases salivary stress markers such as sAA and SIgA in response to exposure to high stress tactical scenarios (Groer et al., Citation2010; McAllister et al., Citation2022). In fact, a recent study demonstrated that the physiological stress response to a VR-ASD is the same as the response noted from a real-life active shooter scenario involving professional actors (Martaindale et al., Citation2023). VR based simulated high stress tactical scenarios may be a way to facilitate occupational training to improve performance and decision making while operating in a safe and controlled environment (Greco & Fischetti, Citation2018; Pallavicini et al., Citation2016). A review on stress exposure training suggests that exposing individuals to high stress scenarios may increase stress resilience, potentially attenuating risk for developing stress related psychiatric conditions such as post-traumatic stress disorder and depression (Russo et al., Citation2012). Moreover, past work has shown that VR based stress exposure/inoculation training can be used as a beneficial tool for stress inoculation training to mitigate physiological stress responses and improve performance while under stress (Izard et al., Citation2018; Pallavicini et al., Citation2016; Popović et al., Citation2009). While ingestion of L-theanine and L-tyrosine did not impact the salivary stress response to acute participation in a high stress scenario, more work is needed to better understand the impact of long-term nutritional interventions involving L-theanine and L-tyrsoine.
In terms of the impact of L-theanine and L-tyrosine on biomarkers of stress, the present study demonstrated between group differences for the stress markers sAA, SIgA, HR and SAI. However, it is important to note that regardless of the between group differences, ingestion of the amino acids did not impact the stress response to the MSC. These findings are in contrast with two previous studies that reported reduced sAA and SIgA from supplementation with L-theanine (Kimura et al., Citation2007; Unno et al., Citation2013). There are a few potential explanations for the conflicting results. First, the while the study conducted by Kimura et al. (Citation2007) used a similar dosage (200 mg L-theanine) and age range for participants, the reduced SIgA concentrations were reported during a 20-minute mental stress challenge involving mental arithmetic. There were no differences reported in pre or post stress with respect to SIgA concentrations (Kimura et al., Citation2007). As such, it is possible that the differences in the length of mental stressor, or timing of collection of SIgA samples between the present study and data reported by Kimura et al. (Citation2007) could potentially explain the discrepancy in results. Moreover, data from Unno et al. (Citation2013) demonstrated reduced sAA concentrations among university students during pharmacy practice. Briefly, 200 mg of L-theanine was ingested twice per day for a total of 17 days. Concentrations of sAA were found to be lower in the morning (after waking up) following L-theanine supplementation during pharmacy practice (i.e. a stressful condition) compared to placebo (Unno et al., Citation2013). This effect was likely attributed to suppressed stress-induced HPA axis activation as L-theanine can cross the blood-brain barrier, reduce glutamate release and increase γ-aminobutyric acid, glycine and dopamine release in the brain (Levy & Tasker, Citation2012; Unno et al., Citation2013; Wang et al., Citation2022). Since the subjects in the present study were exposed to an acute high-stress scenario, it is possible that factors such as the timing of the sample collection, duration and/or intensity of the stressor, or duration of the supplement ingestion period could all impact the results. As such, more research is needed to further examine the potential impact of L-theanine ingestion on concentrations of sAA and SIgA in response to acute stress.
In terms of the impact on mental performance, the L-tyrosine group demonstrated significantly less missed responses compared to the placebo treatment for the Stroop challenge. While other aspects of cognitive performance were higher in the L-tyrosine condition (response time and correct responses for Stroop), the findings did not reach statistical significance (p = 0.05–0.08). These findings are similar to past work showing benefits to aspects of cognitive performance associated with L-tyrosine ingestion such as improvements to working memory (Colzato et al., Citation2013; Mahoney et al., Citation2007), decision making (Coull et al., Citation2015), and mathematical processing (addition/subtraction) (Attipoe et al., Citation2015; Magill et al., Citation2003). Supplementation with L-theanine and L-tyrosine have both been shown to improve aspects of working memory or cognitive processing in sleep deprived conditions (Magill et al., Citation2003; Tamano et al., Citation2013). It should be noted that the finding from Tamano et al. (Citation2013) involved rodent models. However, data from Magill et al. (Citation2003) demonstrated favorable effects among healthy young men under sleep deprived conditions. It is possible that the improvements to markers of cognitive performance could be attributed to L-tyrosine being a precursor to catecholamines epinephrine, norepinephrine, and dopamine. More recent data from Bloemendaal et al. (Citation2018) suggest that the cognitive enhancing effects of L-tyrosine are likely attributed to dopamine activity and are perhaps age dependent, however more data are needed to support this hypothesis. Finally, with respect to salivary stress markers, is should be mentioned that the present study incorporated a dosage of 2000 mg L-tyrosine which is in line with previous studies (Colzato et al., Citation2013; Deijen et al., Citation1999). However, the most common dose among literature is typically 150 mg/kg (Attipoe et al., Citation2015; Bloemendaal et al., Citation2018; Coull et al., Citation2015; Magill et al., Citation2003; Mahoney et al., Citation2007). As such, the lack of an interaction effect from supplement ingestion on salivary stress markers could be due to a lower dosage than what is commonly used for L-tyrosine.
The present study does have some limitations that should be acknowledged. First, regarding the mental performance data, a baseline assessment was not conducted prior to supplement ingestion. This was done in attempt to minimize order/learning effects. Therefore, while between group differences were reported for some of the mental performance data, it is not possible to determine whether or not these effects were attributed to supplement ingestion, or due to beween group differences that existed at baseline. While this is a limitation, the statistical analysis was conducted in such a way to test for interactions between the three experimental conditions (i.e., differences in the response across time between the treatments). Moreover, the mental performance assessment presently used (Stroop and mental arithmetic) has not been validated in terms of reliability and validity for a cognitive/executive performance measure. It should also be mentioned that a single blinded study was used and this should also be viewed as a limitation. In addition, the present study used college-aged subjects (mean age = 21 ± 2.61 yrs) which were not law enforcement or military personnel. As such, the responses to the MSC could differ in a tactical population. Finally, we are not aware of whether or not third-party validation testing may have been done on these supplement powders to verify dosing.
5. Conclusions
The findings from the present study indicate that ingestion of L-theanine and L-tyrosine, does not impact markers of stress (HR, SAI, sAA, SIgA) in response to a high-stress scenario but may result in cognitive enhancing effects. This finding was supported by higher markers of Stroop performance in the supplemented groups compared to the placebo group. As such, these findings could provide beneficial insight to individuals working in high-stress occupations such as military or law enforcement personnel. However, more studies are needed to further examine the potential cognitive enhancing effects of these supplements among those personnel.
Authors’ contribution
All authors contributed to conceptualization of study design, data analysis, interpretation, and initial and final manuscript write up.
Data accessibility statement
Data will be made available upon reasonable request by contacting corresponding author. Note, consent was not obtained to make data publicly available.
Acknowledgements
The authors would like to thank the graduate and undergraduate students of Texas State University for their assistance with data collection.
Disclosure statement
No potential conflict of interest was reported by the author(s).
Additional information
Funding
Notes on contributors
Matthew J. McAllister
Matthew J. McAllister is an Associate Professor and the Director of the Metabolic & Applied Physiology Laboratory in the Department of Health and Human Performance at Texas State University. He holds a PhD in Nutrition from Mississippi State University. His research examines the impact of occupational stressors among tactical athletes. His research team studies nutritional & training interventions that can mitigate the physiological impact of these stressors and improve aspects of cardiometabolic health and physical performance.
M. Hunter Martaindale
M. Hunter Martaindale is the Director of Research at the ALERRT Center and an Associate Research Professor within the School of Criminal Justice and Criminology, both at Texas State University. He received a Ph.D. in Criminal Justice and Criminology from Texas State University in 2016. His research interests include improving law enforcement performance/ decision-making, non-law enforcement perceptions of law enforcement functions, and active shooter events.
Courtney C. Dillard
Courtney C. Dillard is a doctoral student at the University of North Alabama, conducting research in the Exercise Biochemistry Laboratory under the supervision of Dr. Hunter Waldman. She completed her Master’s in Exercise Science at Texas State University in 2023. Her research focuses on utilizing exercise and nutrition interventions to alter physiological stress markers and enhance metabolic flexibility, with an emphasis on tactical populations (e.g., law enforcement, firefighters, military) and females.
Rory McCullough
Rory McCullough graduated from Texas State University with a Master’s in Exercise Science in May 2023. His research interests include studying the relationship with stress and performance among law enforcement personnel, and how pH and gut health are impacted by dietary supplement ingestion.
References
- Attipoe, S., Zeno, S. A., Lee, C., Crawford, C., Khorsan, R., Walter, A. R., & Deuster, P. A. (2015). Tyrosine for mitigating stress and enhancing performance in healthy adult humans, a rapid evidence assessment of the literature. Military Medicine, 180(7), 1–10. https://doi.org/10.7205/milmed-d-14-00594
- Bloemendaal, M., Froböse, M. I., Wegman, J., Zandbelt, B. B., van de Rest, O., Cools, R., & Aarts, E. (2018). Neuro-cognitive effects of acute tyrosine administration on reactive and proactive response inhibition in healthy older adults. eNeuro, 5(2), ENEURO.0035-17.2018. https://doi.org/10.1523/ENEURO.0035-17.2018
- Colzato, L. S., Jongkees, B. J., Sellaro, R., & Hommel, B. (2013). Working memory reloaded: tyrosine repletes updating in the N-back task. Frontiers in Behavioral Neuroscience, 7, 200. https://doi.org/10.3389/fnbeh.2013.00200
- Coull, N. A., Watkins, S. L., Aldous, J. W., Warren, L. K., Chrismas, B. C., Dascombe, B., Mauger, A. R., Abt, G., & Taylor, L. (2015). Effect of tyrosine ingestion on cognitive and physical performance utilising an intermittent soccer performance test (iSPT) in a warm environment. European Journal of Applied Physiology, 115(2), 373–386. https://doi.org/10.1007/s00421-014-3022-7
- Deijen, J. B., & Orlebeke, J. F. (1994). Effect of tyrosine on cognitive function and blood pressure under stress. Brain Research Bulletin, 33(3), 319–323. https://doi.org/10.1016/0361-9230(94)90200-3
- Deijen, J. B., Wientjes, C. J., Vullinghs, H. F., Cloin, P. A., & Langefeld, J. J. (1999). Tyrosine improves cognitive performance and reduces blood pressure in cadets after one week of a combat training course. Brain Research Bulletin, 48(2), 203–209. https://doi.org/10.1016/s0361-9230(98)00163-4
- Greco, G., & Fischetti, F. (2018). Physical, technical and tactical training and stress management in law enforcement. Journal of Physical Education and Sport, 18(2), 555–560.
- Groer, M., Murphy, R., Bunnell, W., Salomon, K., Van Eepoel, J., Rankin, B., White, K., & Bykowski, C. (2010). Salivary measures of stress and immunity in police officers engaged in simulated critical incident scenarios. Journal of Occupational and Environmental Medicine, 52(6), 595–602. https://doi.org/10.1097/JOM.0b013e3181e129da
- Herman, J. P., McKlveen, J. M., Ghosal, S., Kopp, B., Wulsin, A., Makinson, R., Scheimann, J., & Myers, B. (2016). Regulation of the hypothalamic-pituitary-adrenocortical stress response. Comprehensive Physiology, 6(2), 603–621. https://doi.org/10.1002/cphy.c150015
- Hidese, S., Ogawa, S., Ota, M., Ishida, I., Yasukawa, Z., Ozeki, M., & Kunugi, H. (2019). Effects of L-theanine administration on stress-related symptoms and cognitive functions in healthy adults: A randomized controlled trial. Nutrients, 11(10), 2362. https://doi.org/10.3390/nu11102362
- Huang, S. H., Johnson, K., & Pipe, A. L. (2006). The use of dietary supplements and medications by Canadian athletes at the Atlanta and Sydney Olympic Games. Clinical Journal of Sport Medicine: official Journal of the Canadian Academy of Sport Medicine, 16(1), 27–33. https://doi.org/10.1097/01.jsm.0000194766.35443.9c
- Izard, S. G., Juanes, J. A., García Peñalvo, F. J., Estella, J. M. G., Ledesma, M. J. S., & Ruisoto, P. (2018). Virtual reality as an educational and training tool for medicine. Journal of Medical Systems, 42(3), 50. https://doi.org/10.1007/s10916-018-0900-2
- Kimura, K., Ozeki, M., Juneja, L. R., & Ohira, H. (2007). L-Theanine reduces psychological and physiological stress responses. Biological Psychology, 74(1), 39–45. https://doi.org/10.1016/j.biopsycho.2006.06.006
- Levy, B. H., & Tasker, J. G. (2012). Synaptic regulation of the hypothalamic-pituitary-adrenal axis and its modulation by glucocorticoids and stress. Frontiers in Cellular Neuroscience, 6, 24. https://doi.org/10.3389/fncel.2012.00024
- Lieberman, H. R., Thompson, L. A., Caruso, C. M., Niro, P. J., Mahoney, C. R., McClung, J. P., & Caron, G. R. (2015). The catecholamine neurotransmitter precursor tyrosine increases anger during exposure to severe psychological stress. Psychopharmacology, 232(5), 943–951. https://doi.org/10.1007/s00213-014-3727-7
- Magill, R. A., Waters, W. F., Bray, G. A., Volaufova, J., Smith, S. R., Lieberman, H. R., McNevin, N., & Ryan, D. H. (2003). Effects of tyrosine, phentermine, caffeine d-amphetamine, and placebo on cognitive and motor performance deficits during sleep deprivation. Nutritional Neuroscience, 6(4), 237–246. https://doi.org/10.1080/1028415031000120552
- Mahoney, C. R., Castellani, J., Kramer, F. M., Young, A., & Lieberman, H. R. (2007). Tyrosine supplementation mitigates working memory decrements during cold exposure. Physiology & Behavior, 92(4), 575–582. https://doi.org/10.1016/j.physbeh.2007.05.003
- Martaindale, M. H., Sandel, W. L., Duron, A., & McAllister, M. J. (2023). Can a virtual reality training scenario elicit similar stress response as a realistic scenario-based training scenario? Police Quarterly, 27(1), 109–129. https://doi.org/10.1177/10986111231182729
- Marteau, T. M., & Bekker, H. (1992). The development of a six-item short-form of the state scale of the Spielberger State-Trait Anxiety Inventory (STAI). British Journal of Clinical Psychology, 31(3), 301–306
- Maughan, R. J., Depiesse, F., & Geyer, H, (2007). The use of dietary supplements by athletes. Journal of Sports Sciences, 25 Suppl 1(sup1), S103–S113. https://doi.org/10.1080/02640410701607395
- McAllister, M. J., Gonzalez, D. E., Leonard, M., Martaindale, M. H., Bloomer, R. J., Pence, J., & Martin, S. E. (2023). Risk factors for cardiometabolic disease in professional firefighters. Journal of Occupational and Environmental Medicine, 65(2), 119–124. https://doi.org/10.1097/JOM.0000000000002743
- McAllister, M. J., Martaindale, M. H., Dillard, C. C., & Gonzalez, D. E. (2024). Stress response to virtual reality based active shooter training: Impact of caffeine consumption. Psychoneuroendocrinology, 161(106923), 106923. https://doi.org/10.1016/j.psyneuen.2023.106923
- McAllister, M. J., Martaindale, M. H., Gonzalez, A. E., & Case, M. J. (2022). Virtual reality based active shooter training drill increases salivary and subjective markers of stress. The Yale Journal of Biology and Medicine, 95(1), 105–113.
- Pallavicini, F., Argenton, L., Toniazzi, N., Aceti, L., & Mantovani, F. (2016). Virtual reality applications for stress management training in the military. Aerospace Medicine and Human Performance, 87(12), 1021–1030. https://doi.org/10.3357/amhp.4596.2016
- Popović, S., Horvat, M., Kukolja, D., Dropuljić, B., & Cosić, K. (2009). Stress inoculation training supported by physiology-driven adaptive virtual reality stimulation. Studies in Health Technology and Informatics, 144, 50–54.
- Rasmussen, D., Ishizuka, B., Quigley, M., & Yen, S. (1983). Effects of tyrosine and tryptophan ingestion on plasma catecholamine and 3, 4-dihydroxyphenylacetic acid concentrations. The Journal of Clinical Endocrinology and Metabolism, 57(4), 760–763. https://doi.org/10.1210/jcem-57-4-760
- Russo, S. J., Murrough, J. W., Han, M.-H., Charney, D. S., & Nestler, E. J. (2012). Neurobiology of resilience. Nature Neuroscience, 15(11), 1475–1484. https://www.nature.com/articles/nn.3234.pdf https://doi.org/10.1038/nn.3234
- Scheid, L., Ellinger, S., Alteheld, B., Herholz, H., Ellinger, J., Henn, T., Helfrich, H.-P., & Stehle, P. (2012). Kinetics of ʟ-theanine uptake and metabolism in healthy participants are comparable after ingestion of ʟ-theanine via capsules and green tea, 4. The Journal of Nutrition, 142(12), 2091–2096. https://doi.org/10.3945/jn.112.166371
- Soteriades, E. S., Smith, D. L., Tsismenakis, A. J., Baur, D. M., & Kales, S. N. (2011). Cardiovascular disease in US firefighters: A systematic review. Cardiology in Review, 19(4), 202–215. https://doi.org/10.1097/CRD.0b013e318215c105
- Stephenson, M. D., Schram, B., Canetti, E. F. D., & Orr, R. (2022). Effects of acute stress on psychophysiology in armed tactical occupations: A narrative review. International Journal of Environmental Research and Public Health, 19(3), 1802. https://doi.org/10.3390/ijerph19031802
- Stock, A. K., Colzato, L., & Beste, C. (2018). On the effects of tyrosine supplementation on interference control in a randomized, double-blind placebo-control trial. European Neuropsychopharmacology: The Journal of the European College of Neuropsychopharmacology, 28(8), 933–944. https://doi.org/10.1016/j.euroneuro.2018.05.010
- Tamano, H., Fukura, K., Suzuki, M., Sakamoto, K., Yokogoshi, H., & Takeda, A. (2013). Preventive effect of theanine intake on stress-induced impairments of hippocamapal long-term potentiation and recognition memory. Brain Research Bulletin, 95, 1–6. https://doi.org/10.1016/j.brainresbull.2013.02.005
- Tluczek, A., Henriques, J. B., & Brown, R. L. (2009). Support for the reliability and validity of a six-item state anxiety scale derived from the State-Trait Anxiety Inventory. Journal of Nursing Measurement, 17(1), 19-28
- Unno, K., Tanida, N., Ishii, N., Yamamoto, H., Iguchi, K., Hoshino, M., Takeda, A., Ozawa, H., Ohkubo, T., Juneja, L. R., & Yamada, H. (2013). Anti-stress effect of theanine on students during pharmacy practice: Positive correlation among salivary α-amylase activity, trait anxiety and subjective stress. Pharmacology, Biochemistry, and Behavior, 111, 128–135. https://doi.org/10.1016/j.pbb.2013.09.004
- Van de Rest, O., Bloemendaal, M., De Heus, R., & Aarts, E. (2017). Dose-dependent effects of oral tyrosine administration on plasma tyrosine levels and cognition in aging. Nutrients, 9(12), 1279. https://doi.org/10.3390/nu9121279
- Wang, L., Brennan, M., Li, S., Zhao, H., Lange, K. W., & Brennan, C. (2022). How does the tea L-theanine buffer stress and anxiety. Food Science and Human Wellness, 11(3), 467–475. https://doi.org/10.1016/j.fshw.2021.12.004
- Wurtman, R. J., Hefti, F., & Melamed, E. (1980). Precursor control of neurotransmitter synthesis. Pharmacological Reviews, 32(4), 315–335.
- Yamada, T., Terashima, T., Okubo, T., Juneja, L. R., & Yokogoshi, H. (2005). Effects of theanine, r-glutamylethylamide, on neurotransmitter release and its relationship with glutamic acid neurotransmission. Nutritional Neuroscience, 8(4), 219–226. https://doi.org/10.1080/10284150500170799
- Yokogoshi, H., Kobayashi, M., Mochizuki, M., & Terashima, T. (1998). Effect of theanine, r-glutamylethylamide, on brain monoamines and striatal dopamine release in conscious rats. Neurochemical Research, 23(5), 667–673. https://doi.org/10.1023/a:1022490806093
- Yoto, A., Motoki, M., Murao, S., & Yokogoshi, H. (2012). Effects of L-theanine or caffeine intake on changes in blood pressure under physical and psychological stresses. Journal of Physiological Anthropology, 31(1), 28. https://doi.org/10.1186/1880-6805-31-28
- Young, S. N. (2007). L-tyrosine to alleviate the effects of stress? Journal of Psychiatry & Neuroscience, 32(3), 224.