Abstract
Regulation of the production of hypothalamic-pituitary-adrenal (HPA) axis secretagogues, corticotropin-releasing hormone (CRH) and arginine vasopressin (AVP), may be differentially sensitive to the negative feedback effects of glucocorticoids. We chose to study this phenomenon by examining the ability of dexamethasone to influence CRH and AVP heteronuclear RNA (hnRNA) levels in an escapable/inescapable (ES/IS) foot-shock stress paradigm. On Day 1, adult male rats were subjected to either ES or IS foot-shock; on Day 2, saline or dexamethasone (100 μg/kg) was administered 2 h prior to the stressor. We found that ES/IS foot-shock stimulated similar robust increases in plasma adrenocorticotrophic hormone (ACTH) and corticosterone concentrations, and medial parvocellular division of the paraventricular nucleus (mpPVN) AVP and CRH hnRNA and c-fos mRNA levels in saline-treated ES/IS rats. Dexamethasone pretreatment suppressed ACTH and corticosterone levels similarly in IS and ES animals. Dexamethasone pretreatment also suppressed mpPVN CRH and AVP hnRNA levels at 30 min. However, by 120 min, the mpPVN AVP hnRNA levels in dexamethasone-treated rats were similar to those measured in the saline group. We also found that rats that received the most shocks on Day 1 had greater HPA axis activation on Day 2. We conclude that the magnitude of the foot-shock stressor, determined by learned and immediate cues, is important in determining the magnitude of the HPA response.
Introduction
The hypothalamic-pituitary-adrenal (HPA) axis is a vital neuroendocrine axis whose end-products, glucocorticoids, have wide ranging and diverse actions on multiple organ systems and behavior (Sapolsky et al. Citation2000). Stress, either acute or chronic, activates the HPA axis, which results in an increased secretion of glucocorticoids from the adrenal cortex. Glucocorticoid secretion (cortisol in humans, corticosterone in the rat) from the adrenal gland depends on the release of peptides, primarily corticotropin-releasing hormone (CRH) and arginine vasopressin (AVP), from the medial parvocellular division of the paraventricular nucleus of the hypothalamus (mpPVN). These neuropeptides travel through the portal vasculature to reach the anterior pituitary and stimulate the release of adrenocorticotrophic hormone (ACTH), which in turn stimulates glucocorticoid secretion from the adrenal gland. Negative feedback effects of circulating glucocorticoids, in part, terminate ACTH secretion. Although CRH is considered to be the primary ACTH secretagogue, other peptides, primarily AVP, are colocalized with CRH and can act as ACTH secretagogues via potentiation of the effects of CRH (Antoni Citation1993; Keller-Wood and Dallman Citation1984). While both CRH and AVP act to stimulate ACTH secretion, the negative feedback effects of corticosterone impact them differentially. Previous work has indicated that regulation of the AVP transcriptional response is exquisitely sensitive to stressful situations and changes in circulating corticosterone levels, while CRH transcription appears relatively insensitive to acute changes in circulating corticosterone (Helmreich et al. Citation2001; Kovacs et al. Citation2000; Scott and Dinan Citation1998; Shepard et al. Citation2005).
Acutely, glucocorticoids act at multiple levels throughout the organism to enable successful adaptation to stressful stimuli, but chronic dysregulation of the axis and subsequent prolonged elevations in glucocorticoid levels are proposed to be a causal factor for pathophysiological conditions including hippocampal atrophy, bone mineral loss, and obesity (McEwen Citation2003). Dysregulation of the HPA axis has been classically determined by the use of the dexamethasone suppression test (DST). In this test, the ability of dexamethasone, a synthetic glucocorticoid agonist, to suppress plasma ACTH and corticosteroid levels through negative feedback mechanisms is assessed. Dysregulation of the HPA axis is identified when dexamethasone fails to suppress ACTH and corticosteroid release. The use of the DST has suggested that HPA axis dysregulation is associated with multiple psychiatric conditions, including mood disorders such as depression (APA, Citation1987; Ferrari et al. Citation2000; Matthews et al. Citation1986). However, the usefulness of DST is diminished because the particular defect within the HPA axis being assessed has not been well defined. Simplistically, higher than the expected levels of ACTH and corticosteroids after dexamethasone administration could result from an increase in the stimulatory drive to the HPA axis and/or faulty feedback mechanisms, such as change in signaling by the glucocorticoid receptor.
To further study the DST, animal models have been developed. A decreased sensitivity to the negative feedback effects of dexamethasone occurs in animals subjected to subordinate status (Pohorecky et al. Citation2004), social isolation (Serra et al. Citation2005), and social defeat (Buwalda et al. Citation1999). We have been particularly interested in the differential sensitivity to dexamethasone reported in animals subjected to escapable stress (ES) vs. inescapable stress (IS) (Haracz et al. Citation1988; Zhukov Citation1993). These studies reported that corticosterone secretion in animals subjected to IS, but not to ES, is resistant to the suppressive or inhibitory effects of dexamethasone; there are also contrasting data (O'Connor et al. Citation2003).
One possibility for the mechanism underlying dexamethasone non-suppression is that different categories or types of stressors elicit differential secretagogue release (Ceccatelli and Orazzo Citation1993; Harbuz and Lightman Citation1989; Plotsky et al. Citation1985a,Citationb; Watts Citation1991), specifically differential CRH or AVP release, from the mpPVN, rendering the HPA axis more or less sensitive to negative feedback effects of glucocorticoids. This has been previously examined in an animal model of ES/IS, and despite the reported differential sensitivity to dexamethasone between these two conditions, the HPA axis activation in these two stress conditions is similar, including regulation of secretagogue mRNA within the mpPVN (Helmreich et al. Citation1999).
However, this previous work did not examine the regulation of the HPA axis in these two stress conditions in the presence of dexamethasone. Furthermore, examining the hnRNA or transcriptional responses of secretagogue genes to stressful circumstances, rather than changes in the large mRNA pool, has been a productive tool for gaining insight into the regulation of the HPA axis (Itoi et al. Citation1999; Kovacs and Sawchenko Citation1996; Ma et al. Citation1997b; Watts et al. Citation2004). Therefore, in the current study, we examined the regulation of the transcription of CRH and AVP genes in the PVN, as indicated by the changes in hnRNA levels, in the presence of dexamethasone in animals subjected to IS or ES. We found, as in our previous work (Helmreich et al. Citation1999), that the magnitude or intensity of the stressor, rather than psychological components of the stressor, seems to be an important determinant factor in the regulation of the HPA axis.
Materials and methods
Animals
Ninety-six adult male Sprague–Dawley rats from Charles river, with a body weight of 225–350 g were used for this study. All animals were housed two per cage in 40 × 18 × 20 cm tubs; the room temperature ranged from 22 to 23°C, 45% humidity. Lights were on 0730–1930 h, and food and water were available ad libitum. All rats were acclimated to the housing facility for at least a week before testing. Within each tub, a rat from the pair was randomly assigned to either ES or IS.
Stress paradigm
An escapable/inescapable foot-shock paradigm was used for these studies. A pair of rats were individually placed in side-by-side operant testing chambers (30.5 × 24 × 29 cm) with stainless steel grid floors; a white opaque PVC cylinder (20 cm I.D., 23 cm high) was placed within the chambers on top of the grid floor (Zhukov and Vinogradova Citation2002). Within these cylinders, a lever was 7.6 cm from the floor, and a light was 15 cm from the floor, above the lever. The stainless steel grid floors were connected to scrambled shock generators (Med Associates, St. Albans, VT). Med-PC software (Med-Associates) was used to control the onset of the foot-shock stimulus (0.8 mA) and to record data. One session consisted of 80 trials; the variable inter-trial interval ranged from 5 to 115 s, with a mean duration of 60 s. The shock duration for the pair was determined by one of the rats, which had the ability to terminate shock by pressing the lever located within his cage and therefore experienced ES. The lever press by this animal also terminated the shock for the second rat of the pair in the neighboring stress chamber. This second rat had no control over the onset or duration of the stimulus and therefore received IS. If the first rat failed to make an appropriate lever press, the shock was automatically terminated after 30 s. The stress sessions lasted approximately 80–90 min.
Experimental protocol
All rats were weighed every 3 days after arrival and during the course of the experiment (data not shown). On Day 1, ES and IS rats received one 80 trial stress session as described above. On Day 2, 120 min prior to the onset of the stress session, rats were pretreated with either dexamethasone (dexamethasone sodium phosphate, American Regent Laboratories, Inc., Shirley, NY; 100 μg/kg, s.c. in a volume of 1 ml/kg) or an equivalent volume of saline. This dosage of dexamethasone is similar to that in a previously reported study (Haracz et al. Citation1988). One hundred and twenty minutes after injections, rats experienced another stress session, with the same conditions and partners as on Day 1. The pair of rats was killed via rapid decapitation 0, 30, or 120 min post-stress initiation (0 min rats received no stress). All animals were unanaesthetized for decapitation. After decapitation, brains were rapidly removed, frozen in isopentane, and stored at − 80°C until processing. Trunk blood was collected in chilled EDTA-containing tubes (Vacutainer), spun in a refrigerated centrifuge, and the red blood cells were separated and removed; the plasma samples were stored at − 80°C until analysis. Additionally, a blood sample was collected via tail-nick into a chilled EDTA-treated tube (Microvette CB 300 K2E, Sarstedt) at 90 min post-stress initiation in the rats that were later decapitated at 120 min. After the tail-nick, these rats were returned to their home cage until the 120 min time point.
Stress sessions were initiated at 0930 and 1130 h; on Day 2 sample collection occurred between 0930 and 1330 h. At each time point (0, 30, and 120 min), for each stress (ES/IS) and pretreatment condition (dexamethasone or saline), each group size was n = 8 rats.
The University of Rochester Committee on animals resources approved all experimental protocols, and all animals were treated in accordance with the NIH Guide for care and use of laboratory animals.
Radioimmunoassay
Plasma corticosterone and ACTH concentrations were determined using kits from ICN Diagnostics/MP Pharmaceuticals (Irvine, CA). For each hormone, all samples were run in a single assay. Inter- and intra-assay variabilities are reported from information supplied by MP Pharmaceuticals: ACTH intra-assay variability = 4.1–6.8%, inter-assay variability = 3.9–10.7%, corticosterone intra-assay variability = 4.4–10.3%, and inter-assay variability = 6.5–7.1%.
In situ hybridization
Brains were sectioned at 12 μm in the coronal plane throughout the PVN, using a Leica CM1850 cryostat. Four sections per slide, in a series of six, were collected onto Fisher (Pittsburgh PA) Super-Frost Plus slides and stored at − 80°C until further processing. Fisher Super-Frost Plus slides have a permanent electrostatic charge that binds the section to the slide so no subbing is required. In situ hybridization was conducted by using the techniques described previously (Schafer et al. Citation1993). Briefly, sections were removed from the freezer and placed into cold 4% paraformaldehyde for 60 min. Following washes in 2 × SSC (sodium chloride sodium citrate solution), sections were placed in 0.1 M triethanolamine for 15 min to which 0.25% acetic anhydride had been added. Sections were thoroughly washed with 2 × SSC, and then dehydrated through a series of graded ethanol.
The 35S-labeled cRNA probes for CRH and AVP hnRNA and CRH and c-fos mRNA were generated using standard in vitro transcription methodology. Dr R. C. Thompson generously provided the rat DNA templates for CRH hnRNA and mRNA. Dr T. S. Sherman constructed the template for AVP hnRNA and Dr Tom Curran provided the DNA for the c-fos riboprobe template. Labeled probe was separated from unincorporated nucleotides using a Micro Bio-Spin 6 column (Bio-Rad, Hercules, CA, USA). The probe was diluted in 50% formamide hybridization buffer (Amresco, Solon, OH, USA) to yield approximately 2.5 × 106 dpm/70 μl buffer. Diluted probe (70 μl) was applied to each slide and sections were coverslipped. Slides were placed in sealed plastic boxes lined with filter paper moistened with 50% formamide. These boxes were wrapped with plastic wrap and incubated at 55°C for 16 h. Coverslips were removed, and slides were washed in 2 × SSC. Slides were then incubated in RNase A (200 μg/ml) at 37°C for 60 min, washed successively in decreasing concentrations of SSC (2 × , 1 × , 0.5 × , 0.1 × ) and then washed in 0.1 × SSC at 65°C for 60 min. Slides were subsequently washed in the distilled water and dehydrated through graded ethanol.
Slides were then exposed to BioMax MR film (Eastman Kodak, Rochester, NY, USA): AVP hnRNA for 7 days; c-fos mRNA for 4 days; CRH hnRNA for 14 days; and CRH mRNA for 3 days. For all assays, all sections were processed together.
Data analysis
For in situ hybridization, the relative optical density (grey level) was determined for the area of interest from the X-ray film; positive signal was considered to be grey levels above a threshold level that was 3.5 SD above the mean level of the background signal. Background signal intensity was determined over a region of section, near the PVN, without specific labeling. CRH mRNA, hnRNA, and AVP hnRNA images from the X-ray film were captured using a Northern Light light box (Imaging Research/GE Healthcare), Panasonic CCTV camera, and Image J Software. An Epson 3200 scanner was used to digitize the images for the analysis of c-fos mRNA. Relative grey levels were determined using Scion Image J software (Wright Cell Imaging Facility, Toronto Western Research Institute). Both signal intensity and integrated grey level, which incorporates both the intensity and the area of positive signal values, were determined. For mpPVN AVP hnRNA analysis, to limit and define the medial parvocellular region of the PVN, we used contours generated by outlining positive CRH mRNA signal from adjacent sections to identify this region. This analysis strategy does include scattered magnocellular neurons within the CRH mRNA contours; we also quantified the AVP hnRNA expression within the PVN that was dorsal and lateral to the CRH-defined contours and considered this as the magnocellular region. For each probe, the three sections with the highest levels of expression were averaged together to determine the strength of signal in each animal.
Statistical analysis
Differences between saline- and dexamethasone-treated groups were determined with a two-way analysis of variance (ANOVA), with pretreatment and sample time as factors, followed by post-hoc tests using the Bonferroni method (GraphPad Prism). Significant differences among saline vs. dexamethasone and ES vs. IS groups were determined via three-way ANOVA, with pretreatment, shock condition, and sample time as independent factors, using Statview, version 5.0.1, SAS institute. Correlations between variables were determined using Statview; a significance testing of correlations was performed using a two-tailed t-test, which tests the null hypothesis that the correlation is not significantly different from zero (Howell Citation1982). Significance testing between high and low shock groups was determined using a one-way t-test. The significance was p ≤ 0.05; values shown are mean ± SEM.
Results
Shock duration effects on the HPA axis
For all rats, the average amount of shock received on Day 1 was 839 ± 62.5 s, over the course of 80 trials, and on Day 2 the average amount of shock received for all rats was 411 ± 49 s, including the rats exposed to either 30 min or 80–90 min of the stress session. On Day 2, there was no significant difference in the total shock between those rats pretreated with saline (404 ± 84 s) and those pretreated with dexamethasone (412 ± 113 s).
Dexamethasone effects on ACTH and corticosterone secretion
On Day 2, dexamethasone pretreatment (100 μg/kg s.c.; 2 h prior to start of stress session) significantly inhibited the ACTH and corticosterone response to foot-shock stress, as shown in . A significant interaction between pretreatment and sample time was determined via ANOVA for both ACTH and corticosterone {F(3,112) = 21.79, p < 0.0001}. There were no differences between IS and ES for these or any other variables.
Figure 1 Shown are plasma ACTH and corticosterone concentrations measured on Day 2 of foot-shock stress, in rats subjected to either escapable (executive) or inescapable (yoked) foot-shock stress. Rats were pretreated with either 0.9% saline or dexamethasone (100 μg/kg, s.c.) 2 h prior to stress initiation on Day 2. Both ACTH and corticosterone secretion were significantly inhibited by dexamethasone; * indicates a significant difference, p ≤ 0.05, from the saline groups at the same time point, n = 8 rats for each group. There was no effect of stressor controllability on the suppressive effects of dexamethasone on ACTH and corticosterone secretion.
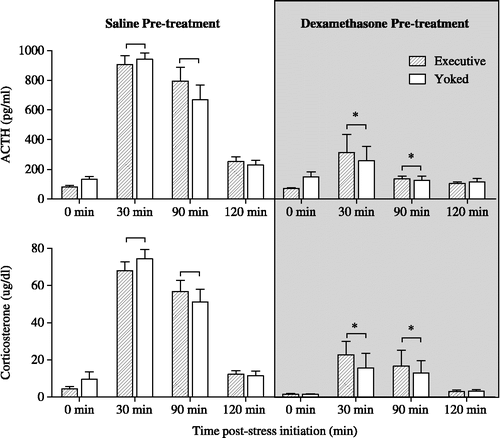
Dexamethasone effects on responses within the mpPVN
The CRH hnRNA levels at 0 min on Day 2 were barely detectable. Foot-shock stress (both conditions) elicited a marked increase at 30 min in mpPVN CRH hnRNA levels, and levels returned to baseline by 120 min ( and ); data between IS/ES groups were collapsed for data analysis and presentation. Dexamethasone pretreatment significantly blunted the CRH hnRNA response to foot-shock stress at the 30 min time point {F(2,86) = 4.93, p < 0.01}.
Figure 2 Shown are photomicrographs of representative images for CRH hnRNA (top panels) and AVP hnRNA (bottom panels) in the PVN from rats killed 0 min (left panels), 30 min (center panels) or 120 min (right panels) post-stress initiation on Day 2. Rats were given either 0.9% saline (left and center panels) or dexamethasone (right panels; 100 μg/kg, s.c.) 120 min before stress initiation. For AVP hnRNA, the medial parvocellular region of the PVN was defined by contours of CRH mRNA expression (white line) obtained from the adjacent section (not shown).
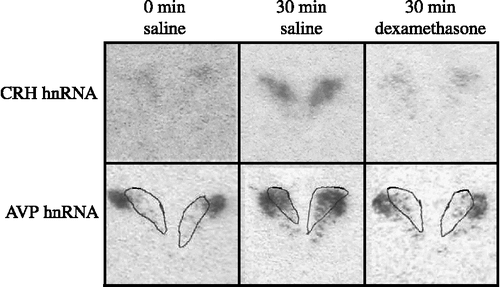
Figure 3 Shown are results from quantification of (A) CRH hnRNA, (B) AVP hnRNA, (C) c-fos mRNA, and (D) CRH mRNA levels within the mpPVN in 0.9% saline and dexamethasone-treated rats. Dexamethasone administration significantly reduced CRH hnRNA level at 30 min, and AVP hnRNA level at 30 min, but not at 120 min post-stress initiation on Day 2. c-fos mRNA level was not significantly altered by dexamethasone pretreatment, ND is non-detectable. CRH mRNA level was significantly decreased by the dexamethasone pretreatment, compared with saline, across both time points. * indicates a significant difference from the saline group at the same time point, p ≤ 0.05; a,b indicate a significant main effect for dexamethasone pretreatment (p ≤ 0.05); groups that share a common letter do not differ. For all analyses, numbers of rats per group are shown in (B).
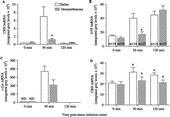
Dexamethasone pretreatment also altered the mpAVP hnRNA response to stress. In the saline-treated groups, foot-shock stress stimulated an increase in the AVP hnRNA level measured at 30 and 120 min. Dexamethasone pretreatment blunted this response at the 30 min time point, but not at the 120 min time point {F(2,86) = 5.05, p < 0.01; and }.
c-fos mRNA was also measured in these rats at 0 and 30 min post-stress, as an indicator of neuronal activation (Ginsberg et al. Citation2003). c-fos mRNA levels within the mpPVN were non-detectable at 0 min post-stress. At 30 min, foot-shock stress stimulated a significant increase in c-fos mRNA levels that was not significantly altered by dexamethasone pretreatment {F(1,28) = 3.41, p = 0.07; }.
CRH mRNA levels within the mpPVN were significantly altered by dexamethasone pretreatment {F(1,88) = 5.4, p < 0.05}. Dexamethasone pretreatment caused a significant reduction in CRH mRNA levels, when compared with saline (). Additionally, there was a trend for CRH mRNA levels at 30 min to be elevated, when compared with 0 and 120 min, irrespective of pretreatment group {F(2,88) = 2.9, p = 0.06}.
Effects of pattern and duration of shock delivery on the HPA axis
The bar press behavior of the rats in the ES condition varied, with some animals learning the required operant response quickly, while others took much longer to acquire the appropriate bar press response. We used a criterion of reaching four consecutive trials of ≤ 4 s as our index of learning. As shown in , this index of shock delivery on Day 1 had higher correlations with activity of components of the HPA axis measured on Day 2 than absolute shock duration on Day 1 or either shock descriptor on Day 2. As this variable had the greatest predictive value for components of the HPA axis, we used it to split all animals into two groups: low shock, pairs of rats in which Day 1 4 ≤ 4 was less than trial 30 and high shock, pairs of rats in which Day 1 4 ≤ 4 was greater to or equal to trial 30. As shown in , we found that rats in the low shock group had significantly lower levels of plasma ACTH {F(1,94) = 8.02, p ≤ 0.01}, corticosterone {F(1,94) = 5.73, p ≤ 0.05}, mpPVN CRH hnRNA {F(1,90) = 4.14, p ≤ 0.05} and mpPVN AVP hnRNA {F(1,90) = 7.42, p ≤ 0.01} than rats in the high shock group.
Table I. Correlation coefficients between plasma hormone levels, mpPVN hnRNA and c-fos mRNA levels measured on Day 2 and shock descriptors for Day 1 and Day 2.
Figure 4 Shown are the stress plasma ACTH and corticosterone concentrations (see ) and mpPVN CRH and AVP hnRNA levels measured on Day 2 in rats exposed to either low or high shock conditions. We used a criterion of reaching four consecutive trials of ≤ 4 s as the index of learning. Low shock: pairs of rats in which Day 1 4 ≤ 4 was less than trial 30. High Shock: pairs of rats in which Day 1 4 ≤ 4 was greater to or equal to trial 30. Rats in high shock group had significantly greater (p ≤ 0.05) plasma ACTH, corticosterone, mpPVN CRH hnRNA and AVP hnRNA levels than rats in low shock group. * indicates significant difference (p < 0.05) from low shock group. Numbers of rats per group for the variables in each row are shown in the left panels.
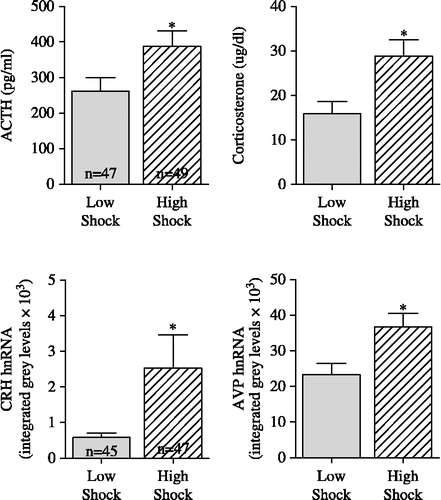
AVP hnRNA expression within magnocellular region
As shown in , AVP hnRNA levels outside of the CRH defined boundaries were not altered by dexamethasone administration {F(1,81) = 1.62, p(0.05}. Foot-shock stress did cause a significant increase in the AVP hnRNA levels within this magnocellular region at 120 min post-stress initiation. There was no significant effect of high vs. low shock on Day 1, as there was for the medial division AVP hnRNA and there were no significant correlations between magnocellular AVP hnRNA levels and corticosterone and ACTH levels, as there were for the mpAVP hnRNA levels.
Discussion
The initial goals of the current experiments were to determine if dissimilar stressors, having similar physical characteristics but diverging in psychological aspects of coping, would vary in their effects with respect to sensitivity to the negative feedback actions of dexamethasone on the HPA axis and, in turn, if this altered sensitivity was mediated by differential secretagogue gene sensitivity to the inhibitory effects of dexamethasone. From the data collected, we found that the HPA axis activity stimulated by different psychological stressors was similarly sensitive to the inhibitory effects of dexamethasone. Also, by using hnRNA levels to measure rapid changes in mpPVN secretagogue transcription caused by stress and dexamethasone, we gained insight into effects of dexamethasone on the HPA axis. Finally, the current results supported our previous hypothesis that the physical intensity of the stressor is important in determining the degree of activation of the HPA axis (Helmreich, Watkins et al. Citation1999), and that the appraised intensity of the stressor was greatly influenced by previous history/experience with the stressor, in addition to the immediate proximate stress stimulus.
We found that dexamethasone pretreatment, 120 min before the onset of the stressor, inhibited both CRH and AVP gene stress-induced transcription, as indicated by decreased hnRNA levels at 30 min post-stress onset within the defined mpPVN. As previous work has demonstrated that mpAVP transcription is especially sensitive to the inhibitory effects of glucocorticoids, particularly corticosterone (Helmreich et al. Citation2001), this was expected. In contrast, glucocorticoid regulation of CRH transcription is not as clearly defined (Aguilera et al. Citation2007). There is evidence suggesting that the stress-induced increases in CRH hnRNA are not sensitive to changes in circulating corticosterone levels (Helmreich et al. Citation2001 Kovacs et al. Citation2000; Shepard et al. Citation2005), but there is also evidence indicating that CRH transcription is indeed sensitive to changes in circulating glucocorticoid levels (Ginsberg et al. Citation2003; Herman et al. Citation1992; Imaki et al. Citation1996; Jiang et al. Citation2004). As proposed by Ginsberg et al. (Citation2003), it seems that the important variable in determining glucocorticoid sensitivity is the timing of the changes in glucocorticoid level relative to the stressor and/or measurement of CRH hnRNA. If the changes in corticosterone occur at the time of the stressor, i.e., stimulus onset in an adrenalectomized animal or corticosterone injection as a stressor mimic (Helmreich et al. Citation2001; Kovacs and Sawchenko Citation1996; Shepard et al. Citation2005), there is not enough time for the changes in glucocorticoid level to impact the rapid increase in CRH hnRNA levels that occurs after stress. However, if changes in glucocorticoid concentration are 1–2 h or longer before the stress {current study (Ginsberg et al. Citation2003; Herman et al. Citation1992; Imaki et al. Citation1996; Jiang et al. Citation2004; Watts et al. Citation2004)}, the CRH hnRNA response will be altered by the change in circulating glucocorticoid concentrations. Notably, the increase in c-fos mRNA level 30 min after foot-shock initiation was not significantly reduced by dexamethasone, indicating that dexamethasone did not prevent trans-synaptic activation of the mpPVN neurons by the stressor (Ginsberg et al. Citation2003).
Stress-induced mpPVN CRH hnRNA levels at the 120 min time point had returned to baseline, while mpPVN AVP hnRNA levels were still elevated. In contrast to the 30 min time point, there was no significant difference in mpAVP hnRNA levels between saline and dexamethasone pretreated groups at the 120 min time point. Dexamethasone pretreatment was without effect on magnocellular AVP hnRNA levels, although foot-shock stress did cause an increase measured at the 120 min time point. Because our anatomical definition of the mpPVN includes scattered magnocellular neurons, it is possible that our observed stress-induced increase at 120 min within the mpPVN may be partly due to magnocellular activity, in addition to parvocellular AVP hnRNA expression. It is important to note that the stress-induced increase at 120 min within the mpPVN is similar to the previous reports examining the time course of stress-induced parvocellular AVP hnRNA levels (Itoi et al. Citation1999; Kovacs et al. Citation2000) and also that the time course of the stress-induced increases and glucocorticoid sensitivity appear to be different between the two regions.
Similar mpPVN AVP hnRNA levels in the dexamethasone-treated and saline-treated rats at the 120 min time point may have resulted from diminution of the pharmacological properties of dexamethasone by this time point. This may not be the primary cause as most DSTs occur over the course of 10–12 h (Lurie et al. Citation1989) and dexamethasone can inhibit median eminence CRH release for at least 6 h post administration (Keller-Wood and Dallman Citation1984; Takebe et al. Citation1971). An alternative hypothesis is that the AVP hnRNA levels at 120 min in the dexamethasone-treated groups are a result of a decrease in circulating corticosterone concentrations or partial “chemical adrenalectomy” (de Kloet Citation1997; Karssen et al. Citation2005) caused by the dexamethasone pretreatment. Previous work has demonstrated that in intact animals, the observed peak increase in mpPVN AVP hnRNA level occurs 120 min post stimulus (Itoi et al. Citation1999; Kovacs et al. Citation2000). Elimination of the stimulus induced increase in corticosterone results in a greater or potentiated increase in AVP hnRNA levels at 120 min (Helmreich et al. Citation2001; Kovacs et al. Citation2000). It is possible that in our current study the inhibition of endogenous stress-induced corticosterone secretion by dexamethasone disinhibited AVP transcription, leading to the observed AVP hnRNA levels at 120 min in the dexamethasone-treated animals. This release from inhibition of AVP transcription occurs even in the presence of exogenous glucocorticoid tone provided by dexamethasone, as CNS penetration of dexamethasone is much less than that of corticosterone and provides a weaker signal to the hypothalamus (de Kloet Citation1997). Finally, the current stress-induced increase of AVP hnRNA at 120 min measured in the mpPVN may be partially due to an increased AVP hnRNA level in the scattered magnocellular neurons, as in the magnocellular part of the PVN at this time.
Secretagogue gene activation in the mpPVN, but not in the magnocellular PVN, was directly correlated with the intensity of the stressor, operationally defined as the duration and pattern of shock delivery. Interestingly, with regard to the factors that are important in determining the “magnitude” of the stressor, the variable that had the greatest predictive value (i.e., the highest correlations, ) for the activation of components of the HPA axis measured on Day 2, was the rate at which the animals learned to press the bar on Day 1. This led to, but was not always associated with, greater absolute duration of shock on Day 1 and on Day 2. Because there was no significant effect of stressor controllability in any variable measured, we do not think that the HPA axis reactivity is a direct corollary of an individual rat's ability to learn, but is indicative of how much shock the animal received on Day 1, and in particular, how much shock the rat received at the beginning of the stress session on Day 1. This suggests that the magnitude of the central drive to the HPA axis is dependent on both learned contextual experiential cues associated with the stressor, in addition to the physical characteristics of the current stressor. This hypothesis requires further direct testing, by a direct manipulation of shock delivery patterns on Day 1 and Day 2. Furthermore, whether this phenomenon extends to additional stressor types will require future investigation. This is particularly relevant in the context of habituation, which occurs when repeated presentation of the same stressor, such as restraint, results in a diminution of the HPA axis response (Grissom et al. Citation2007; Keller-Wood and Dallman Citation1984). Interestingly, foot-shock, the stimulus used in the current studies, has been classified as a non-habituating stressor, in contrast to stressors such as restraint (Helmreich et al. Citation2005; Kant et al. Citation1985). Therefore, the positive relation between stress magnitude on Day 1 and HPA axis activity on Day 2 may be highly dependent on the category of stressor (habituating vs. non-habituating) that is utilized.
Additionally, it is important to note that HPA axis activity was assessed on Day 2 of stress. We utilized 2 days of stress, to try to ensure that rats killed at the 30 min time point would have had enough experience, through stress exposure on Day 1, to learn the appropriate operant response. However, previous work has demonstrated that a previous stress experience can alter the HPA axis response to the current stressor (Ma et al. Citation1997a; Schmidt et al. Citation1996). Therefore, it is probable that the HPA axis activity that we measured on Day 2 was impacted by the stress exposure on Day 1.
As stated previously, the original overall goal of these experiments was to examine differential sensitivity of ES vs. IS to the suppressive effects of dexamethasone. We did not observe this phenomenon in the current studies, even if only the pairs of rats with quick learning executive animals were analyzed. However, the fact that this difference has been previously reported suggests that the observation may be reliable if the correct variables are controlled for. The difficulty lies in identifying all of the relevant variables. One possible factor not previously accounted for is individual differences among animals that influence the reactivity of the HPA axis, such as those between high responders and low responders (Kabbaj et al. Citation2000) or active vs. passive coping style (Bohus et al. Citation1987). Alternatively, we may not have observed any difference between ES/IS because there may not have been any operational differences between them in the current study, despite the experimental setup. Previous studies examining the effects of controllability on behavior, including learned helplessness, and dexamethasone sensitivity (Haracz et al. Citation1988; Maier Citation1984; Maier et al. Citation1995) have utilized one day of stress exposure. However, in the current study, ES animals may not have perceived the stress as controllable, despite the stress exposure on Day 1. Additionally, the IS animals may have performed non-prescribed, superstitious behaviors that helped them cope with the stress resulting in psychologically similar stress conditions in the ES and IS chambers.
Although we did not observe any differences between the rats exposed to ES vs. IS, the use of ES to control the amount of shock delivered allowed for variable amounts of shock on Day 1 and Day 2. This variable amount of shock allowed us to determine how the duration and timing of the stressor impacted the HPA axis. We conclude that the magnitude of the stressor, determined by both learned experiential and immediate cues, is important in determining the magnitude of the HPA response. Additionally, we conclude that a potential event underlying the DST may be the escape of AVP gene transcription in the mpPVN from inhibition by endogenous glucocorticoids.
Acknowledgements
The authors would like to gratefully acknowledge Drs S. J. Watson and H. Akil, in whose laboratory this work was originally undertaken. The authors would also like to acknowledge the significant contributions made by two undergraduate students at Middlebury College—Nate Dorr and Heather Tory. We also appreciate the thoughtful comments from Drs R. Ader, J. Fudge, and J. Moynihan throughout the experiment. We would also like to thank the vivarium staff at the University of Rochester for their animal care. This work was supported by the Department of Psychiatry at the University of Rochester.
References
- Aguilera G, Kiss A, Liu Y, Kamitakahara A. Negative regulation of corticotropin releasing factor expression and limitation of stress response. Stress 2007; 10(2)153–161
- Antoni F. Vasopressinergic control of pituitary adrenocorticotropin secretion comes of age. Front Neuroendocrinol 1993; 14: 76–122
- APA. The dexamethasone suppression test: An overview of its current status in psychiatry. Am J Psychiatry 1987; 144(10)1253–1262
- Bohus B, Benus R, Fokkema D, Koolhaas J, Nyakas C, van Oortmerssen G, Prins A, de Ruiter A, Scheurink A, Steffens A. Neuroendocrine states and behavioral and physiological stress responses. Prog Brain Res 1987; 72: 57–70
- Buwalda B, de Boer S, Schmidt E, Felszeghy K, Nyakas C, Sgoifo A, Vab der Vegt B, Tilders F, Bohus B, Koolhaas J. Long-lasting deficient dexamethasone suppression of hypothalamic-pituitary-adrenocortical activation following peripheral CRF challenge in socially defeated rats. J Neuroendocrinol 1999; 11: 513–520
- Ceccatelli S, Orazzo C. Effect of different types of stressors on peptide messenger ribonucleic acids in the hypothalamic paraventricular nucleus. Acta Endocrinol (Copenh) 1993; 128(6)485–492
- Ferrari E, Fioravanti M, Magri F, Solberte S. Variability of interactions between neuroendocrine and immunological functions in physiological aging and dementia of the Alzheimer's type. Ann NY Acad Sci 2000; 917: 582–596
- Ginsberg A, Campeau S, Day H, Spencer R. Acute glucocorticoid pretreatment suppresses stress-induced hypothalamic-pituitary-adrenal axis hormone secretion and expression of corticotropin-releasing hormone hnRNA but does not affect c-fos mRNA or fos protein expression in the paraventricular nucleus of the hypothalamus. J Neuroendocrinol 2003; 15: 1075–1083
- Grissom N, Iyer V, Vining C, Bhatnagar S. The physical context of previous stress exposure modifies hypothalamic-pituitary-adrenal responses to a subsequent homotypic stress. Horm Behav 2007; 51: 95–103
- Haracz J, Minor T, Wilkins J, Zimmermann E. Learned helplessness: An experimental model of the DST in rats. Biol Psychiatry 1988; 23: 388–396
- Harbuz MS, Lightman SL. Responses of hypothalamic and pituitary mRNA to physical and psychological stress in the rat. J Endocrinol 1989; 122(3)705–711
- Helmreich DL, Watkins LR, Deak T, Maier SF, Akil H, Watson SJ. The effect of stressor controllability on stress-induced neuropeptide mRNA expression within the paraventricular nucleus of the hypothalamus. J Neuroendocrinol 1999; 11(2)121–128
- Helmreich DL, Itoi K, Lopez-Figueroa MO, Akil H, Watson SJ. Norepinephrine-induced CRH and AVP gene transcription within the hypothalamus: Differential regulation by corticosterone. Brain Res Mol Brain Res 2001; 88(1–2)62–73
- Helmreich D, Parfitt D, Lu X-Y, Akil H, Watson S. Relation between the hypothalamic-pituitary-thyroid (HPT) axis and the hypothalamic-pituitary-adrenal (HPA) axis during repeated stress. Neuroendocrinology 2005; 81: 183–192
- Herman J, Schafer M, Thompson R, Watson S. Rapid regulation of corticotropin-releasing hormone gene transcription in vivo. Mol Endocrinol 1992; 1061–1069
- Howell D. Statistical methods for psychology. PWS Publishers, Boston 1982
- Imaki T, Shibasaki T, Chikada N, Harada S, Naruse M, Demura H. Different expression of immediate-early genes in the rat paraventricular nucleus induced by stress: Relation to corticotropin-releasing factor gene transcription. Endocr J 1996; 43: 629–638
- Itoi K, Helmreich DL, Lopez-Figueroa MO, Watson SJ. Differential regulation of corticotropin-releasing hormone and vasopressin gene transcription in the hypothalamus by norepinephrine. J Neurosci 1999; 19(13)5464–5472
- Jiang Y, Kawashima H, Iwasaki Y, Ichida K, Sugimoto K, Itoi K. Differential effects of forced swim-stress on the corticotropin-releasing hormone and vasopressin gene transcription in the parvocellular division of the paraventricular nucleus of rat hypothalamus. Neurosci Lett 2004; 358: 201–204
- Kabbaj M, Devine DP, Savage VR, Akil H. Neurobiological correlates of individual differences in novelty-seeking behavior in the rat: Differential expression of stress-related molecules. J Neurosci 2000; 20(18)6983–6988
- Kant G, Eggleston T, Landman-Roberts L, Kenion C, Driver G, Meyerhoff J. Habituation to repeated stress is stressor specific. Pharmacol Biochem Behav 1985; 22: 631–634
- Karssen AM, Meijer OC, Berry A, Sanjuan Pinol R, de Kloet ER. Low doses of dexamethasone can produce a hypocorticosteroid state in the brain. Endocrinology 2005; 146(12)5587–5595
- Keller-Wood M, Dallman M. Corticosteroid inhibition of ACTH secretion. Endocr Rev 1984; 5(1)1–24
- de Kloet E. Why dexamethasone poorly penetrates in brain. Stress 1997; 2(1)13–20
- Kovacs K, Sawchenko P. Regulation of stress-induced transcriptional changes in the hypothalamic neurosecretory neurons. J Mol Neurosci 1996; 7: 125–133
- Kovacs K, Foides A, Sawchenko P. Glucocorticoid negative feedback selectively target vasopressin transcription in parvocellular neurosecretory neurons. J Neurosci 2000; 20(10)3843–3852
- Lurie S, Kuhn C, Bartolome J, Schanberg S. Differential sensitivity to dexamethasone suppression in an animal model of the DST. Biol Psychiatry 1989; 26: 26–34
- Ma X, Levy A, Lightman S. Emergence of an isolated arginine vasopressin (AVP) response to stress after repeasted restraint: A study of both AVP and corticotropin-releasing hormone messenger ribonucleic acid (RNA) and heteronuclear RNA. Endocrinology 1997a; 138: 4351–4357
- Ma X, Levy A, Lightman S. Rapid changes in heteronuclear RNS for corticitropic-releasing hormone and arginine vasopressin in response to acute stress. J Endocrinol 1997b; 152: 81–89
- Maier S. Learned helplessness and animal models of depression. Prog Neuropsychopharmacol Biol Psychiatry 1984; 8: 435–446
- Maier S, Busch C, Maswood S, Grahn R, Watkins L. The dorsal raphe nucleus is a site of action mediating the behavioral effects of the benzodiazepine receptor inverse agonist DMCM. Behav Neurosci 1995; 109(4)759–766
- Matthews M, Akil H, Greden J, Charney D, Weinberg V, Rosenbaum A, Watson S. Beta-endorphin/beta-lipotropin immunoreactivity in endogenous depression. Effect of dexamethasone. Arch Gen Psychiatry 1986; 43(4)374–381
- McEwen B. Mood disorders and allostatic load. Biol Psychiatry 2003; 54: 200–207
- O'Connor K, Johnson J, Hammack S, Brooks L, Spencer R, Watkins L, Maier S. Inescapabable shock induces resistance to the effects of dexamethasone. Psychoneuroendocrinology 2003; 28: 481–500
- Plotsky PM, Bruhn TO, Vale W. Evidence for multifactor regulation of the adrenocorticotropin secretory response to hemodynamic stimuli. Endocrinology 1985a; 116(2)633–639
- Plotsky PM, Bruhn TO, Vale W. Hypophysiotropic regulation of adrenocorticotropin secretion in response to insulin-induced hypoglycemia. Endocrinology 1985b; 117(1)323–329
- Pohorecky L, Baumann M, Benjamin D. Effects of chronic social stresson on neuroendocrine responsiveness to challenge with ethanol, dexamethasone and corticotropin-releasing hormone. Neuroendocrinology 2004; 80: 332–342
- Sapolsky R, Romero L, Munck A. How do glucocorticoids influence stress responses? Integrating permissive, suppressive, stimulatory, and preparative actions. Endocr Rev 2000; 21(1)55–89
- Schafer K-H, Herman J, Watson S. In situ hybridization histochemistry. Imaging drug action in the brain, E London. CRC Press, Boca Raton, FL 1993; 337–377
- Schmidt ED, Binnekade R, Janszen AW, Tilders FJ. Short stressor induced long-lasting increases of vasopressin stores in hypothalamic corticotropin-releasing hormone (CRH) neurons in adult rats. J Neuroendocrinol 1996; 8(9)703–712
- Scott L, Dinan T. Vasopressin and the regulation of hypothalamic-pituitary-adrenal axis function: Implications for the pathophysiology of depression. Life Sci 1998; 62(22)1985–1998
- Serra M, Pisu M, Floris I, Biggio G. Social isolation-induced changes in the hypothalamic-pituitary-adrenal axis in the rat. Int J Biol Stress 2005; 8: 259–264
- Shepard J, Liu Y, Sassone-Corsi P, Aguilera G. Role of glucocorticoids and cAMP-mediated repression in limiting corticotropin-releasing hormome transcription during stress. J Neurosci 2005; 25: 4073–4081
- Takebe K, Kunita H, Sakakura M, Horiuchi Y, Mashimo K. Suppressive effect of dexamethasone on the rise of CRF activity in the median eminence of the rat. Endocrinology 1971; 89: 1014–1019
- Watts AG. Ether anesthesia differentially affects the content of prepro-corticotropin-releasing hormone, prepro-neurotensin/neuromedin N and prepro-enkephalin mRNAs in the hypothalamic paraventricular nucleus of the rat. Brain Res 1991; 544(2)353–357
- Watts A, Tanimura S, Sanchez-Watts G. Corticotropin-releasing hormone and arginine vasopressin gene transcription in the hypothalamic paraventricular nucleus of the unstressed rat: Daily rhythms and their intereractions with corticosterone. Endocrinology 2004; 145: 529–540
- Zhukov D. The dexamethasone suppression test in genetically different rats exposed to inescapable and escapable electric shocks. Psychoneuroendocrinology 1993; 18(7)467–474
- Zhukov D, Vinogradova K. Learned helplessness or learned inactivity after inescapable stress? Interpretation depends on coping styles. Integr Physiol Behav Sci 2002; 37(1)35–43