Abstract
Long-term exposure to stressful situations can affect the immune system. The T-cell response is an important component of anti-tumoral immunity. Hence, impairment of the immune function induced by a chronic stressor has been postulated to alter the immunosurveillance of tumors, thus leading to a worse neoplastic prognosis. Here, we show that chronic restraint stress affects T-cell mediated immunity in mice. This was evidenced by a decrease of mitogen-induced T-cell proliferation, a reduction in CD4+T lymphocyte number and a decrease of tumor necrosis factor-alpha (TNF-α) and Interferon-gamma (IFN-γ) production in stressed mice. Additionally, mice subjected to chronic restraint stress displayed an enhancement of tumor growth in a syngeneic lymphoma model, i.e. an increase of tumor proliferation and a reduction of animal survival. Finally, stressed mice had a reduced specific cytotoxic response against these tumor cells. These results suggest that chronic exposure to stress promotes cancer establishment and subsequent progression, probably by depressing T-cell mediated immunity. The T-cell immunity impairment as well as the tumor progression enhancement emphasize the importance of the therapeutic management of stress to improve the prognosis of cancer patients.
Introduction
In the last decades, stress has become an important aspect of daily life. A growing body of evidence indicates that stress is a key factor in the development of several pathologies, including psychiatric diseases such as anxiety and major depression, disruption of neuroendocrine systems, alterations of the immune response and even cancer (Reiche et al. Citation2004). Several animal models in rodents and primates using physical and/or psychological stressors are useful tools for the study of deleterious effects induced by chronic stress exposure (Willner and Mitchell Citation2002, Van Kampen et al. Citation2002). These models are validated in accordance with typical behavioral alterations, i.e. altered performance in tasks such as the open field, forced swimming and tail suspension tests (Palumbo et al. Citation2007; Morgulis et al. Citation2004). Using such models, it has been reported that chronic stress alters the immune system. This is evidenced by a reduction of T-cytotoxic and natural killer (NK) activities (Nunez et al. Citation2006), alterations of T- and B-lymphocyte proliferative responses to mitogens (Edgar et al. Citation2003; Silberman et al. Citation2002), impairment of antibody production (Silberman et al. Citation2003) and changes in cytokine secretion (Merlot et al. Citation2004). T cells and NK cells are the major components of anti-tumoral immunity. Both CD8+T-cytotoxic and CD4+T-helper lymphocytes are crucial regulators of tumor growth (Foss Citation2002; Ostrand-Rosenberg Citation2005). NK cells are potent effectors against tumor cells by inducing cytotoxicity (O'Hanlon Citation2004; Abbas et al. Citation2000). Cellular cytokines, such as tumor necrosis factor-alpha (TNF-α) and interferon-gamma (IFN-γ) are key mediators in these processes (Knutson and Disis Citation2005; Dredge et al. Citation2002). Taken together, the evidence strongly suggests that chronic stress could enhance tumor growth by suppressing anti-tumoral immunity (Calcagni and Elenkov Citation2006).
This proposal was first made by Vernon Riley (Citation1975, Citation1981) and since then considerable progress in this field has been achieved (Cohen, Rabin Citation1998; Freire-Garabal et al. Citation2004; Saul et al. Citation2005; Thaker et al. Citation2006). However, the influence of chronic stress exposure on the immune response and hence on tumor progression and the mechanisms underlying these processes are still far from being completely understood. In this scenario, the aim of the present work was to evaluate the effects of chronic stress exposure on cellular immunity and on tumor evolution. With this purpose, we first validated our repeated restraint model by performing behavioral measurements in order to confirm that this paradigm induced a state of chronic stress. Next, we evaluated the lymphoproliferative response to T- and B- selective mitogens, as well as the NK cytotoxic activity against YAC-1 cells of splenocytes from normal and stressed mice. In addition, we evaluated balance of the CD4+/CD8+ subsets by dual fluorescence flow cytometry in stressed and normal mice. We also studied the effects of chronic stress on TNF-α and IFN-γ mRNA expression in these animals using real time RT-PCR. Finally, we analyzed the influence of chronic stress on the biological behavior of a T-cell lymphoma (LBC cells) growing in vivo in syngeneic mice, and the specific cytotoxicity against these tumor cells in stressed mice bearing tumors.
Materials and methods
Animals
Inbred female BALB/c (H-2d) mice between 60 and 100 days old were purchased from the Instituto Nacional de Tecnologia Agropecuaria (INTA, Castelar, Buenos Aires, Argentina). Animals were cared for in accordance with the Guide for the Care and Use of Laboratory Animals of the National Institute of Health (NIH, USA); experimental protocols were approved by the Internal Ethics Committee of the School of Medicine of the University of Buenos Aires, i.e. Comité Institucional para Cuidado y Uso de los Animales de Laboratorio (CICUAL).
All the mice were housed in groups of 3–6, depending on the experiment, per cage and maintained on a 12/12 h light/dark cycle with the lights on from 8 AM to 8 PM under controlled temperatures between 18°C and 22°C. Food and water were available ad libitum.
Experimental procedures
Mice were randomly assigned to the stress or control groups (n = 47 mice for each condition in total; numbers per experimental group are in Tables and Figures legends), according to our previous protocol (Alfonso et al. Citation2006). All experiments were repeated at least three times. For the chronic restraint stress model, mice were restrained daily for 6 h (from 11 AM to 5 PM) in well-ventilated polypropylene tubes (2.8 cm diameter × 11.5 cm length) without access to food and water. The mice were not physically compressed and did not experience pain. This protocol was performed for at least three weeks. For immunity studies mice were killed at the third week by cervical dislocation between 9 and 11 AM. For lymphoma evaluation, tumor cells were injected at the third week, and the stress protocol continued until the mice died or were killed; mice were monitored every day, and were killed if a series of observations indicated the need for humane euthanasia following the guidelines for animal care, including hypothermia and slow locomotion among others. The survival time was counted as if euthanased mice had died naturally.
For LBC cytolytic activity assays and for flow cytometric analysis of LBC proliferation assays mice were stressed for three weeks, injected with LBC cells and stressed for two more weeks and then killed. Mice from control groups were housed in normal conditions. Each experiment used control mice of the same weight and age.
Behavioral tests
Open field test
The open field test was performed using a rectangular plastic chamber (42 × 35 × 15 cm), with its floor divided into 30 equal squares. The mice were placed in the center of the open field and allowed to explore freely for 10 min. The parameters registered were: rearing (vertical exploration), crossed lines (horizontal exploration) and immobility time.
Forced swimming test
Mice were placed in a transparent cylinder (17 cm diameter × 25 cm high) half-filled with warm water (30°C) for 6 minutes. Latency to begin floating was scored as the time between introduction of a mouse into the cylinder and the first moment of complete immobility of the entire body, irrespective of the duration of the first floating episode. The total time spent floating was scored during the last 4 min of the test.
Tail suspension test
Mice were suspended by the tail to a plastic string positioned horizontally 75 cm above the table top, using hypoallergenic adhesive tape placed at 1 cm from the tip of the tail. Total duration of immobility was measured over 6 min.
Syngeneic lymphoma model
The LBC cell line is an aggressive T-cell lymphoma derived from an early T-cell lymphocyte precursor in BALB/c mice (H-2d), as described by Mongini et al. (Citation2001). LBC T lymphoma cells were cultured in RPMI 1640 (Invitrogen, Carlsbad, CA, USA) supplemented with 10% fetal bovine serum (Invitrogen), 2 mM glutamine (Invitrogen), 100 μg/ml streptomycin (Invitrogen), and 50 μM 2-β-mercaptoethanol (Sigma–Aldrich, St. Louis, MI, USA). Before implantation, LBC cells were washed with phosphate buffered saline (PBS), counted according to Trypan blue staining (Sigma–Aldrich) and resuspended in sterile PBS. Syngeneic mice from the indicated experimental groups were subcutaneously inoculated with 1 × 106 LBC cells in 100 μl PBS to generate a solid tumor. Tumor length and width were measured every two days using calipers (resolution: 1 mm), and tumor volume was calculated as V = π/6 × length × width2.
Lymph node cell suspensions, culture conditions, and proliferation assays
Lymphocytes from spleens (which are enriched in B-cells and NK cells) and lymph nodes (which are enriched in T-cells) were obtained as previously described (Edgar et al. Citation2003). Briefly, mice were killed by cervical dislocation and spleens and lymph nodes were quickly removed and disrupted through a 1-mm metal mesh. Cell suspensions were centrifuged and washed twice with RPMI 1640 supplemented with 10% fetal bovine serum, 2 mM glutamine and 100 μg/ml streptomycin. Cell viability was estimated according to Trypan blue exclusion criteria and was greater than 90%. Proliferation was determined by culturing 2 × 105 cells per well in 96-well plates in a final volume of 0.2 ml in triplicate aliquots of supplemented medium. The T-cell mitogen Concanavalin A (Con A, Sigma–Aldrich) or the B-cell mitogen lipopolysaccharides from Escherichia coli (LPS, Sigma–Aldrich) were added to the microcultures to yield the optimal mitogen concentrations of 1 and 30 μg/ml, respectively (Mamchak and Hodgkin Citation2000). Cells were cultured at 37°C in a 5% CO2 atmosphere. Mitogenic activity was measured by adding 0.75 μCi [3H]thymidine (Amersham Biosciences, Little Chalfont, Buckinghamshire, UK) per well for the last 24 h of culture. [3H]Thymidine incorporation was measured by scintillation counting after retention over GF/C glass-fiber filters (Whatman, Brentford, Middlesex, UK) of the acid-insoluble macromolecular fraction. Mitogen-stimulated cells displayed the expected proliferation kinetic, with a peak of proliferation at the third day of culture.
Natural killer activity assay
YAC-1 cells were purchased from ATCC (Manassas, VA, USA). Cells were cultured in supplemented medium as described for LBC cells. Specific cytotoxic activity against tumor cells was evaluated according to the JAM method (Ayres et al Citation2003; Wunderlich et al. Citation1997). Briefly, YAC-1 cells were labeled with 5 μCi [3H]thymidine 3 h before the cytotoxicity assays were carried out. Cell suspensions from spleens from normal and stressed mice were obtained as described above. Different ratios of effector and target cells (1:100 to 1:1) were added to microtiter plates (100 μl each) at final volumes of 200 μl, and incubated for 3.5 h at 37°C in a 5% CO2 atmosphere. Plates were harvested as described above. Percentages of cytotoxic activity were calculated as NK = 100 × (SR − ER)/SR, where SR is the spontaneous release and ER is the experimental release.
Lymphocyte subsets determination by flow cytometry
CD4+T-helper/inducer and CD8+T-cytotoxic/suppressor lymphocytes were determined in lymph node cell suspensions by flow cytometry (Silberman et al. Citation2003). Briefly, 1 × 106 cells in 100 μl cytometry buffer (2% bovine fetal serum and 0.01% NaN3 in PBS) were stained with 0.50 μl of fluorescein-conjugated anti-mouse CD4 (CD4-FITC, clone GK1.5) and 0.50 μl of phycoerythrin-conjugated anti-mouse CD8 (CD8-PE, clone 53–6.7) monoclonal antibodies (eBioscience, San Diego, CA, USA), washed twice and fixed with 2% formaldehyde in PBS. Lymphocytes were identified by FACS analysis using a BD FACSCalibur flow cytometer (BD Biosciences, San Jose, CA, USA). Dot plots of two color fluorescence analysis as well as percentages of lymphocytes expressing CD4 and CD8 were determined. Isotype controls (clones MOPC-141 and UPC-10, respectively, Sigma–Aldrich) were used for each assay to determine non-specific staining.
Total RNA and poly(A+) mRNA isolation and cDNA synthesis
Lymph nodes were stored in liquid nitrogen until use. As we described previously (Alfonso et al. Citation2006), tissues were homogenized in Trizol Reagent (Invitrogen) and total RNA was isolated following the manufacturer's instructions. Poly(A+) mRNA was isolated from total RNA using the PolyATract mRNA isolation System (Promega, Madison, WI, USA). Complementary DNA was synthesized by retrotranscription using oligo(dT) and SuperScript II Reverse Transcriptase enzyme (Invitrogen).
Quantitative real time reverse transcription polymerase chain reaction
Real time RT-PCRs were carried out in a GeneAmp 5700 Sequence Detection System (Applied Biosystems, Foster City, CA, USA), as we previously described (Alfonso et al. Citation2006). cDNA amounts present in each sample were determined in duplicates using SYBR Green PCR Core Reagents kit (Applied Biosystems). Primer sequences were designed using Primer Express software (Applied Biosystems). To verify that the SYBR Green dye detected only one PCR product, all the reactions were subjected to a heat dissociation protocol following the final cycle of the PCR. Oligonucleotide sequences used were: TNF-α forward 5′-GCA CCA CCA TCA AGG ACT CAA-3′, TNF-α reverse 5′-TTG CAG AAC TCA GGA ATG GAC A-3′, IFN-γ forward 5′-TGC TGA TGG GAG GAG ATG TCT AC-3′, IFN-γ reverse 5′-ACC TGA CAC ATT CGA GTG CTG T-3′, β-actin forward 5′-CAA CTT GAT GTA TGA AGG CTT TGG T-3′, β-actin reverse 5′-ACT TTT ATT GGT CTC AAG TCA GTG TAC AG-3′. For data normalization, values were referred to β-actin as a housekeeping gene.
Flow cytometric analysis of LBC proliferation assays
LBC cells were cultured as described above. Before tumor implantation, tumor cells were stained with carboxyfluorescein diacetate succinimidyl ester (CFSE) using the Vybrant CFDA SE Cell Tracer Kit (Invitrogen) following the manufacturer's instructions. Briefly, cell suspensions were incubated with 20 μM CFSE for 15 min at 37°C, washed and re-incubated for 30 min. Traced cells were subcutaneously injected as described above. Two weeks later, mice were killed and tumors were quickly removed and disrupted through a 1 mm metal mesh. Cell suspensions were centrifuged and washed twice with PBS and then fixed with 3.7% formaldehyde in PBS. LBC cells were identified by fluorescence analysis at 492 nm using a BD FACSCalibur flow cytometer (Lyons Citation1999).
LBC cytolytic activity assay
Specific cytotoxic activity against tumor cells was evaluated according to the JAM method as described for NK activity. Briefly, LBC were labeled over night with 5 μCi [3H]thymidine and co-cultured with cell suspensions from spleens from normal and stressed mice that were injected with the lymphoma cells two weeks before and hence were bearing solid tumors. Different concentrations of effector and target cells were added to microtiter plates (100 μl each) at final volumes of 200 μl, and incubated for 3.5 h at 37°C in a 5% CO2 atmosphere. Plates were harvested as described above. Percentages of cytotoxic activity were calculated as the relation CTL = 100 × (SR–ER)/SR, where SR is the spontaneous release and ER is the experimental release.
Statistical analysis
Group means were analyzed for statistical significance with the software XLSTAT 2007.8.01 Version (Addinsoft, New York, NY, USA), using unpaired two-tailed Student t tests. For survival analysis, Kaplan–Meier curves were constructed and compared using Log–Rank tests. Differences between means were considered significant if p < 0.05. Results are expressed as groups mean ± standard error of the mean, except in the case of animal survival data, which are expressed as mean ± standard deviation.
Results
To investigate the effects of chronic stress on immunity and cancer prognosis, we used the well known model of repeated restraint stress in mice. Stressed mice displayed a significant reduction in body weight gain after three weeks of repeated restraint (as a percentage of initial body weight, Control = 108.44 ± 2.44% vs. Stress = 95.84 ± 1.78%, p < 0.01), as we have previously shown (Alfonso et al. Citation2006). Additionally, chronically restrained mice displayed altered locomotor activity in the open field test, i.e. an increase of crossed lines and rearings and a reduction of the immobility time (; p < 0.05 and p < 0.01). As expected, these mice also showed a reduction in the latency to escape as well as an increase of the immobility time in the forced swimming test and in the tail suspension test (; p < 0.05).
Table I. Behavioral alterations induced by chronic restraint stress.
Chronic stress impairs the T-cell lymphoproliferative response
In order to evaluate the status of the cellular and humoral immune response in normal and stressed mice, lymphocyte proliferation to both T- and B- selective mitogens was evaluated by [3H]thymidine incorporation. Cell suspensions from lymph nodes and spleens were stimulated with Con A (T-cell specific) and LPS, respectively (B-cell specific). A significant reduction in T-cell proliferation was observed in cells from stressed mice (; p < 0.01), whereas B-cell proliferation was unchanged ().
Figure 1 Effects of chronic stress on lymphoproliferative response to selective T- and B- mitogens. Cells from lymph nodes and spleens of stressed mice and untreated controls were stimulated with Con A and LPS respectively, labeled with [3H]thymidine for 72 h and harvested. Representative results from three independent experiments are shown. Values are expressed as group means ± SEM. of the stimulated/basal ratio (proliferation index). Statistical significance was determined using unpaired t-test (n = 6 mice per group, **p < 0.01).
![Figure 1 Effects of chronic stress on lymphoproliferative response to selective T- and B- mitogens. Cells from lymph nodes and spleens of stressed mice and untreated controls were stimulated with Con A and LPS respectively, labeled with [3H]thymidine for 72 h and harvested. Representative results from three independent experiments are shown. Values are expressed as group means ± SEM. of the stimulated/basal ratio (proliferation index). Statistical significance was determined using unpaired t-test (n = 6 mice per group, **p < 0.01).](/cms/asset/de9c8118-c09e-4726-bcf7-9d8fdf58507f/ists_a_313909_f0001_b.gif)
Chronic stress does not alter natural killer activity
We also studied whether chronic stress affects NK cell activity. Cytotoxicity was evaluated using [3H]thymidine, previously incorporated into the DNA (Ayres et al Citation2003; Wunderlich et al. Citation1997). DNA fragments are retained in filters when harvested, and their radioactivity is inversely related to cytolytic activity of effector cells. We found no differences in NK specific cytotoxicity against YAC-1 cells between mice control and stressed mice in the target:effector ratios ().
Figure 2 Effects of chronic stress on NK activity. Cells suspensions from spleens of stressed mice and untreated controls were co-incubated with YAC-1 cells labeled with [3H]-thymidine at different target:effector ratios, cultured for 3.5 h and harvested. Representative results from three independent experiments are shown. Values are expressed as group means ± SEM. of NK specific cytolysis, calculated as 100 × (SR-ER)/SR, where SR is the spontaneous release and ER is the experimental release of [3H]thymidine. Statistical significance was determined using unpaired t-test (n = 4 mice per group, no significant difference).
![Figure 2 Effects of chronic stress on NK activity. Cells suspensions from spleens of stressed mice and untreated controls were co-incubated with YAC-1 cells labeled with [3H]-thymidine at different target:effector ratios, cultured for 3.5 h and harvested. Representative results from three independent experiments are shown. Values are expressed as group means ± SEM. of NK specific cytolysis, calculated as 100 × (SR-ER)/SR, where SR is the spontaneous release and ER is the experimental release of [3H]thymidine. Statistical significance was determined using unpaired t-test (n = 4 mice per group, no significant difference).](/cms/asset/66bdae8c-f959-4a20-8b66-e21a8ca8cb63/ists_a_313909_f0002_b.gif)
Chronic stress alters T-lymphocyte subsets distribution
We also evaluated whether chronic stress induces any modification in CD4+T-helper/inducer and CD8+T-cytotoxic/suppressor lymphocytes. These populations were determined in lymph node cell suspensions by dual fluorescence flow cytometry using specific α-CD4-FITC and α-CD8-PE antibodies. Chronic stress resulted in a significant reduction of CD4+ lymphocytes but did not affect CD8+ lymphocytes (; p < 0.05). As expected, the CD4/CD8 ratio was also reduced in stressed mice (Control = 2.99 ± 0.31; Stress = 1.80 ± 0.17; p < 0.05).
Table II. T lymphocyte subsets distribution in stressed and control mice.
Chronic stress reduces cytokine expression
We next tested if chronic stress affects the production of cytokines involved in T-cell mediated anti-tumoral immunity. We measured TNF-α and IFN-γ mRNA expression in lymph nodes of normal and stressed mice using real time RT-PCR according to the SYBR Green method. Real time PCRs revealed a reduction of TNF-α and IFN-γ mRNA expression levels relative to β-actin in lymphocytes of stressed mice compared with controls ( and ; p < 0.01).
Figure 3 Effects of chronic stress on TNF-α and IFN-γ mRNA expression in lymphocytes. Total RNA and mRNA were isolated from lymph nodes of stressed mice, and untreated mice, and used for cDNA synthesis. Cytokine expression was evaluated by Real Time RT-PCR using SYBR Green dye. Representative results from three independent experiments are shown. Values are expressed as group means ± SEM. normalized with β-actin as housekeeping gene. Statistical significance was determined using unpaired t-test (n = 5 mice per group, **p < 0.01).
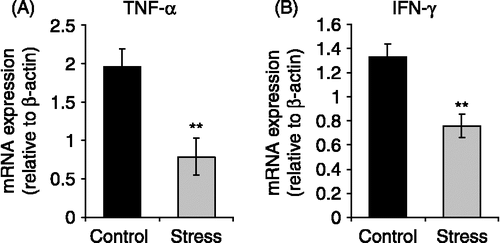
Chronic stress promotes LBC lymphoma growth
We also asked whether immune system depression induced by chronic stress exposure could promote tumor growth. With this purpose, stressed and normal syngeneic mice were subcutaneously injected with 1 × 106 LBC T-lymphoma cells to generate a solid tumor. Measures of tumor volume indicated that growth was increased in chronically stressed mice as compared to control animals at several time points tested (; p < 0.01). To evaluate whether tumors growing in stressed mice proliferated faster than those growing in control animals, LBC cells were stained with CFSE prior to injection. CFSE is a colorant that binds spontaneously and irreversibly to intracellular proteins. As cells proliferate, CFSE distributes equally between daughter cells, thus the amount of dye present in the cytosol is reduced by half with each division (Lyons Citation1999). After two weeks, cells were recovered and analyzed by flow cytometry. LBC cells growing in stressed mice had a smaller mean fluorescence intensity (Gmean) than those grown in control mice (; Control = 32.33 ± 1.40 arbitrary units of CFSE fluorescence at 492 nm (AU); Stress = 12.59 ± 1.58 AU; p < 0.01), indicating that tumor cells had an accelerated proliferation rate in stressed mice. Additionally, Kaplan–Meier plots of animal survival were compared with the Log–Rank analysis. Stressed mice displayed a significant reduction of survival, indicating a worse prognosis of the neoplastic pathology (, Control = 26.80 ± 0.70 days; Stress = 22.10 ± 1.40 days; p < 0.01).
Figure 4 Effect of chronic stress on LBC lymphoma cell proliferation and prognosis. Mice were subjected to chronic restraint stress, or control conditions, for three weeks, and then were inoculated subcutaneously with 1 × 106 LBC cells to generate a solid tumor. Stress procedures continued until the mice died. Representative results from three independent experiments are shown. (A) Tumor progression after appearance. Tumor length and width were measured and tumor volume was calculated as V = π/6 × L × W2. Values are expressed as group means ± SEM. for each day post-tumor injection (p.t.i.). Statistical significance was determined using unpaired t-test (n = 10 mice per group, **p < 0.01). (B) CFSE fluorescence intensity of LBC cells growing in stressed and control mice. The graph shows the number of events against the mean fluorescence intensity for each treatment, for a representative experiment of one stressed and one control mouse. Statistical significance was determined using unpaired t-test (n = 3 mice per group, p < 0.01). (C) Kaplan-Meier plot of survival of stressed and control mice (n = 10 mice per group, p < 0.01, Log-Rank analysis).
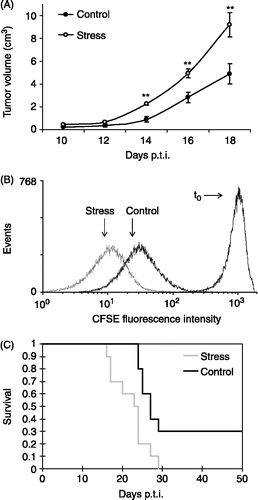
Chronic stress reduces specific cytotoxicity against LBC cells
We finally tested whether cytotoxic activity against LBC cells was altered in stressed mice bearing tumors. Control and stressed mice were injected with LBC cells, and two weeks later spleen cells were co-incubated with LBC cells. Stressed mice showed a reduced cytotoxicity against LBC cells in comparison to control mice (), supporting a worse tumor prognosis induced by immune system depression associated to chronic stress.
Figure 5 Effects of chronic stress on specific cytotoxic activity against LBC cells. Cells from spleens of stressed mice and untreated controls were co-cultured with LBC cells labeled with [3H]thymidine, and its release was evaluated as an indicator of cytolysis. Representative results from three independent experiments are shown. Values are expressed as group means ± SEM. of the percentage of cytotoxic activity at different effector:target ratios. Statistical significance was determined using unpaired t-test (n = 4 mice per group, *p < 0.05, **p < 0.01).
![Figure 5 Effects of chronic stress on specific cytotoxic activity against LBC cells. Cells from spleens of stressed mice and untreated controls were co-cultured with LBC cells labeled with [3H]thymidine, and its release was evaluated as an indicator of cytolysis. Representative results from three independent experiments are shown. Values are expressed as group means ± SEM. of the percentage of cytotoxic activity at different effector:target ratios. Statistical significance was determined using unpaired t-test (n = 4 mice per group, *p < 0.05, **p < 0.01).](/cms/asset/6d943518-2f94-4026-8d4d-0b407eacbe8e/ists_a_313909_f0005_b.gif)
Discussion
To investigate the effects of chronic stress on immunity and cancer prognosis, we used the well known model of repeated restraint stress in mice (Alfonso et al. Citation2006). Stressed mice showed the common behavioral changes observed in this and other models of chronic stress (Palumbo et al. Citation2007; Morgulis et al. Citation2004). Briefly, here we showed that chronic stress impaired T-cell mediated immunity and enhanced syngeneic lymphoma progression. With respect to the effects of stress on cognate immunity, we found that chronic stress significantly impaired T-cell proliferation, without affecting B-cell proliferation. Reduction of T-cell proliferation has been reported in other models of chronic stress. For example, chronic mild stress in mice and chronic social confrontation in rats results in a reduced T-cell response to Con A (Edgar et al. Citation2003; Silberman et al. Citation2002; Stefanski and Engler Citation1999). Instead, mice subjected to chronic mild stress display an increased B-cell proliferation (Edgar et al. Citation2003; Silberman et al. Citation2002). Here, we found no changes on B-cell reactivity to LPS in mice subjected to restraint stress. The main effector components of anti-tumoral immunity are T and NK cells (Abbas et al. Citation2000). Our results are in accordance with these functions, given that we found a reduction of T -but not B-cell proliferation. However, the role of antibodies in anti-tumor immunity can not be ruled out, because they participate in the elimination of tumors (Paschen et al. Citation2004). Indeed, we have found that T-cell depression induced by chronic unpredictable stress results in an impairment of the T-cell dependent humoral response (Silberman et al. Citation2004). Therefore, in our restraint stress model, a reduced T-lymphocyte response could result in a decrease of T-cell dependent antibody production directed to tumor antigens.
With regard to T-lymphocyte subset distribution, here we demonstrated that chronic stress resulted in a reduction of CD4+, but not CD8+, T-lymphocytes in the lymph nodes, suggesting that stress mainly affects T-helper immunity. This is in agreement with a report by Saul et al. (Citation2005), who found a reduction of tumor infiltrating CD4+ but not CD8+- T cells in restraint-stressed mice bearing squamous cell carcinoma tumors. Stefanski and Engler (Citation1999) found a reduction of both CD4+ and CD8+T-lymphocytes in blood from socially stressed rats. However, we found no changes in CD4+ and CD8+T-lymphocyte subsets in lymph nodes from mice exposed to chronic mild stress (Silberman et al. Citation2002). Differences observed between chronic mild and chronic restraint models could be due to the type of stressor applied in each case. The chronic mild stress model is a heterotrophic model that includes different stressors of moderate intensity applied randomly. Instead, chronic restraint stress and social stress are homotrophic models that involve one type of strong stressor. However, it is known that stress affects the redistribution of immune cells among the different lymphoid organs (Dhabhar Citation2003). Therefore, the discrepancies between these studies could also be due to the fact that CD4/CD8 subsets were measured in different immune compartments. It is important to note that even though we did not find any difference in CD8+T-cell number, the participation of T-cytotoxic activity can not be ruled out. In this respect, CD4+T-helper cells modulate cytotoxic activity of CD8+T cells (Dredge et al. Citation2002). Thus, the reduction of CD4+T-lymphocytes observed in stressed mice could at least impair CD8+T-cell activity. This is in accordance with a report indicating that chronic stress reduces T-cytotoxic activity (Nunez et al. Citation2006). Nevertheless, it is important to highlight that it was recently demonstrated that CD4+T-helper cells are more efficient in the elimination of tumors compared to CD8+T-cytotoxic cells (Perez-Diez et al. Citation2007).
However, we found no changes in cytotoxic activity against YAC-1 cells between normal and chronically stressed mice. Although some reports have shown a reduction of NK activity induced by stress (Nuñez et al. Citation2006; Stefanski and Engler Citation1999), other reports indicate that NK activity can remain unaltered after stress exposure depending on the stressor applied and its duration (Oishi et al. Citation2003). In particular, it was demonstrated that chronic restraint stress did not modify NK activity in rats (Steplewski and Vogel Citation1986). Although the participation of NK cells in these processes can not be ruled out, NK activity has classically been related to the inhibition of metastasis (Christianson et al. Citation1996) rather than to the control of tumor cell proliferation, which is undoubtedly mediated by T cells (Turner et al. Citation1990), in accordance with the present results.
Changes in the patterns of cytokine production have been reported in stress models. Here, we observed an important decrease in TNF-α and IFN-γ, two key cytokines involved in T-cell mediated anti-tumoral immunity, in lymphocytes from chronically restrained mice compared to controls. It was demonstrated that chronic restraint stress exposure reduces IFN-γ production (Saul et al. Citation2005). In the case of TNF-α, it was also shown that combined acoustic and restraint stress decreases this proinflammatory cytokine (Kiank et al. Citation2006), in agreement with the present results. Furthermore, stress hormones have been reported to inhibit systemically TNF-α and IFN-γ (Calcagni and Elenkov Citation2006). The present results indicating a reduction of TNF-α and IFN-γ mRNA expression are consistent with the promotion of tumor growth observed in restrained mice, and could be associated with a subsequent impairment of T-cell proliferation, NK activity and specific cytotoxicity against tumor cells.
We also asked whether this impairment of immunity induced by chronic stress could be associated with an alteration of tumor evolution. We observed an enhanced syngeneic T-lymphoma growth in chronically restrained mice. Stressed mice had an increase of tumor volume and of tumor cell proliferation, and also a reduced survival. Moreover, specific cytolytic activity against this lymphoma cell line was reduced in stressed mice bearing a tumor. Recent findings indicate that chronically restrained stressed mice have a higher susceptibility to skin cancer (Saul et al. Citation2005) and that behavioral stress results in the promotion of ovarian carcinoma growth and angiogenesis (Thaker et al. Citation2006). In addition, Freire-Garabal et al. (Citation2004) found that chronic auditory stress results in a modification of the incidence of breast cancer in mice and development of lung metastases in rats. It was also shown that prolonged psychosocial stress decreases resistance to B16F10 melanoma (Sá-Rocha et al. Citation2006) and Ehrlich tumor (Morgulis et al. Citation2004). Our results also contribute to the hypothesis of coexistence between stress and cancer (Reiche et al. Citation2004; Calcagni and Elenkov Citation2006; Glaser and Kiecolt-Glaser Citation2005), and point to a key role of T-cell mediated immunity in these processes. Indeed, the importance of T-cell response impairment in pathological challenges during exposure to stressful situations has already been demonstrated (Bonneau et al. Citation1997; Citation1998; Sheridan et al. Citation1991; Citation1998). Moreover, pioneer work in this field suggested that stress compromise immunological function, predisposing the individual to an increased risk of cancer (Riley Citation1975; Citation1981). Th1 CD4+ cells are well known to modulate the activities of cytotoxic lymphocytes, natural killer cells and macrophages, and these are mediated by the production of IFN-γ, TNF-α and IL-2 (Dredge et al. Citation2002). Thus, chronic stress could be affecting anti-tumoral immunity by diminishing the production of these cytokines as observed here, hence impairing the cellular immune response against tumor cells. In particular, T-cell mediated immunity is essential in the control of T-lymphomas, and TNF-α and IFN-γ are involved in these processes (Rook et al. Citation1999; Krawczyk et al. Citation1995), further supporting the results of the present work. It is important to mention that although other mechanisms can be involved, during the exposure to a stressful situation undoubtedly there is a co-existence of T-cell immunity impairment and tumor growth enhancement. Finally, taking into account that stress is a widespread aspect of modern life, the results emerging from this study highlight the importance of the therapeutic control of stress to improve cancer prognosis.
Acknowledgements
This work was supported by grants from CONICET, UBA, ANPCyT, and HHMI. LRF, MLBA, and MPZ are research fellows from CONICET, MR is a research fellow from ANPCyT and MB, CM, AMG, and GAC are researchers from CONICET. We wish to thank Dr. Alberto C. Frasch for his invaluable help with real-time RT-PCRs.
Declaration of interest: The authors report no conflicts of interest. The authors alone are responsible for the content and writing of the paper.
References
- Abbas AK, Lichtman AH, Pober JS. Cellular and Molecular Immunology4th ed. McGraw-Hill, Philadelphia, PA 2000; 235–269
- Alfonso J, Frick LR, Silberman DM, Palumbo ML, Genaro AM, Frasch AC. Regulation of hippocampal gene expression is conserved in two species subjected to different stressors and antidepressant treatments. Biol Psychiatry 2006; 59: 244–251
- Ayres FM, Narita M, Takahashi M, Alldawi L, Liu A, Osman Y, Abe T, Yano T, Sakaue M, Toba K, Furukawa T, Aizawa Y. A comparative study of the JAM test and 51Cr-release assay to assess the cytotoxicity of dendritic cells on hematopoietic tumor cells. Immunol Invest 2003; 32: 219–227
- Bonneau RH, Brehm MA, Kern AM. The impact of psychological stress on the efficacy of anti-viral adoptive immunotherapy in an immunocompromised host. J Neuroimmunol 1997; 78: 19–33
- Bonneau RH, Zimmerman KM, Ikeda SC, Jones BC. Differential effects of stress-induced adrenal function on components of the herpes simplex virus-specific memory cytotoxic T-lymphocyte response. J Neuroimmunol 1998; 82: 191–199
- Calcagni E, Elenkov I. Stress system activity, innate and T helper cytokines, and susceptibility to immune-related diseases. Ann N Y Acad Sci 2006; 1069: 62–76
- Christianson SW, Greiner DL, Schweitzer IB, Gott B, Beamer GL, Schweitzer PA, Hesselton RM, Shultz LD. Role of natural killer cells on engraftment of human lymphoid cells and on metastasis of human T-lymphoblastoid leukemia cells in C57BL/6J-scid mice and in C57BL/6J-scid bg mice. Cell Immunol 1996; 171: 186–199
- Cohen S, Rabin BS. Psychologic stress, immunity, and cancer. J Natl Cancer Inst 1998; 90: 3–4
- Dhabhar FS. Stress, leukocyte trafficking, and the augmentation of skin immune function. Ann N Y Acad Sci 2003; 992: 205–217
- Dredge K, Marriott JB, Todryk SM, Dalgleish AG. Adjuvants and the promotion of Th1-type cytokines in tumor immunotherapy. Cancer Immunol Immunother 2002; 51: 521–531
- Edgar VA, Silberman DM, Cremaschi GA, Zieher LM, Genaro AM. Altered lymphocyte catecholamine reactivity in mice subjected to chronic mild stress. Biochem Pharmacol 2003; 65: 15–23
- Foss FM. Immunologic mechanisms of antitumor activity. Semin Oncol 2002; 29: 5–11
- Freire-Garabal M, Rey-Méndez M, García-Vallejo LA, Balboa J, Suárez JM, Rodrigo E, Brenlla J, Núñez MJ. Effects of nefazodone on the development of experimentally induced tumors in stressed rodents. Psychopharmacology 2004; 176: 233–238
- Glaser R, Kiecolt-Glaser JK. Stress-induced immune dysfunction: Implications for health. Nat Rev Immunol 2005; 5: 243–251
- Kiank C, Holtfreter B, Starke A, Mundt A, Wilke C, Schütt C. Stress susceptibility predicts the severity of immune depression and the failure to combat bacterial infections in chronically stressed mice. Brain Behav Immun 2006; 20: 359–368
- Knutson KL, Disis ML. Tumor antigen-specific T helper cells in cancer immunity and immunotherapy. Cancer Immunol Immunother 2005; 54: 721–728
- Krawczyk CM, Verstovsek S, Ujházy P, Maccubbin D, Ehrke MJ. Protective specific immunity induced by cyclophosphamide plus tumor necrosis factor alpha combination treatment of EL4-lymphoma-bearing C57BL/6 mice. Cancer Immunol Immunother 1995; 40: 347–357
- Lyons AB. Divided we stand: Tracking cell proliferation with carboxyfluorescein diacetate succinimidyl ester. Immunol Cell Biol 1999; 77: 509–515
- Mamchak AA, Hodgkin PD. Regulation of lipopolysaccharide-induced B-cell activation: Evidence that surface immunoglobulin mediates two independently regulated signals. Immunol Cell Biol 2000; 78: 142–148
- Merlot E, Moze E, Dantzer R, Neveu PJ. Cytokine production by spleen cells after social defeat in mice: Activation of T cells and reduced inhibition by glucocorticoids. Stress 2004; 7: 55–61
- Mongini C, Ruybal P, Gravisaco MJ, Croci M, Sánchez Lockhart M, Fabris V, Waldner AC. Characterization of the immunophenotype and the metastatic properties of a murine T-lymphoma cell line. Unexpected expression of cytoplasmatic CD4. In Vitro Cell Dev Biol Anim 2001; 37: 499–504
- Morgulis MS, Stankevicius D, Sá-Rocha LC, Palermo-Neto J. Cohabitation with a sick cage mate: Consequences on behavior and on ehrlich tumor growth. Neuroimmunomodulation 2004; 11: 49–57
- Nunez MJ, Balboa J, Rodrigo E, Breñilla J, Gonzalez-Peteiro M, Freire-Garabal M. Effects of fluoxetine on cellular immune response in stressed mice. Neurosci Lett 2006; 396: 247–251
- O'Hanlon LH. Natural born killers: NK cells drafted into the cancer fight. J Natl Cancer Inst 2004; 96: 651–653
- Oishi K, Nishio N, Konishi K, Shimokawa M, Okuda T, Kuriyama T, Machida K. Differential effects of physical and psychological stressors on immune functions of rats. Stress 2003; 6: 33–40
- Ostrand-Rosenberg S. CD4+T lymphocytes: A critical component of antitumor immunity. Cancer Invest. 2005; 23: 413–419
- Palumbo ML, Fosser NS, Rios H, Zorrilla Zubilete MA, Guelman LR, Cremaschi GA, Genaro AM. Loss of hippocampal neuronal nitric oxide synthase contributes to the stress-related deficit in learning and memory. J Neurochem 2007; 102: 261–274
- Paschen A, Eichmuller S, Schadendorf D. Identification of tumor antigens and T-cell epitopes, and its clinical application. Cancer Immunol Immunother 2004; 53: 196–203
- Perez-Diez A, Joncker NT, Choi K, Chan WF, Anderson CC, Lantz O, Matzinger P. CD4 cells can be more efficient at tumor rejection than CD8 cells. Blood 2007; 109: 5346–5354
- Reiche EM, Nunes SO, Morimoto HK. Stress, depression, the immune system, and cancer. Lancet Oncol 2004; 5: 617–625
- Riley V. Mouse mammary tumors: Alteration of incidence as apparent function of stress. Science. 1975; 189: 465–467
- Riley V. Psychoneuroendocrine influences on immunocompetence and neoplasia. Science 1981; 212: 1100–1109
- Rook AH, Wood GS, Yoo EK, Elenitsas R, Kao DM, Sherman ML, Witmer WK, Rockwell KA, Shane RB, Lessin SR, Vonderheid EC. Interleukin-12 therapy of cutaneous T-cell lymphoma induces lesion regression and cytotoxic T-cell responses. Blood 1999; 94: 902–908
- Sá-Rocha VM, Sá-Rocha LC, Palermo-Neto J. Variations in behavior, innate immunity and host resistance to B16F10 melanoma growth in mice that present social stable hierarchical ranks. Physiol Behav 2006; 88: 108–115
- Saul AN, Oberyszyn TM, Daugherty C, Kusewitt D, Jones S, Jewell S, Malarkey WB, Lehman A, Lemeshow S, Dhabhar FS. Chronic stress and susceptibility to skin cancer. J Natl Cancer Inst 2005; 97: 1760–1767
- Sheridan JF, Feng NG, Bonneau RH, Allen CM, Huneycutt BS, Glaser R. Restraint stress differentially affects anti-viral cellular and humoral immune responses in mice. J Neuroimmunol 1991; 31: 245–255
- Sheridan JF, Dobbs C, Jung J, Chu X, Konstantinos A, Padgett D, Glaser R. Stress-induced neuroendocrine modulation of viral pathogenesis and immunity. Ann N Y Acad Sci 1998; 840: 803–808
- Silberman DM, Wald M, Genaro AM. Effects of chronic mild stress on lymphocyte proliferative response. Participation of serum thyroid hormones and corticosterone. Int Immunopharmacol 2002; 2: 487–497
- Silberman DM, Wald MR, Genaro AM. Acute and chronic stress exert opposing effects on antibody responses associated with changes in stress hormone regulation of T-lymphocyte reactivity. J Neuroimmunol 2003; 144: 53–60
- Silberman DM, Ayelli-Edgar V, Zorrilla-Zubilete M, Zieher LM, Genaro AM. Impaired T-cell dependent humoral response and its relationship with T lymphocyte sensitivity to stress hormones in a chronic mild stress model of depression. Brain Behav Immun 2004; 18: 81–90
- Stefanski V, Engler H. Social stress, dominance and blood cellular immunity. J Neuroimmunol 1999; 94: 144–152
- Steplewski Z, Vogel WH. Total leukocytes, T-cell subpopulation and natural killer (NK) cell activity in rats exposed to restraint stress. Life Sci 1986; 38: 2419–2427
- Thaker PH, Han LY, Kamat AA, Arevalo JM, Takahashi R, Lu C, Jennings NB, Armaiz-Pena G, Bankson JA, Ravoori M, Merritt WM, Lin YG, Mangala LS, Kim TJ, Coleman RL, Landen CN, Li Y, Felix E, Sanguino AM, Newman RA, Lloyd M, Gershenson DM, Kundra V, Lopez-Berestein G, Lutgendorf SK, Cole SW, Sood AK. Chronic stress promotes tumor growth and angiogenesis in a mouse model of ovarian carcinoma. Nat Med 2006; 12: 939–944
- Turner WJ, Chatten J, Lampson LA. Human neuroblastoma cell growth in xenogeneic hosts: Comparison of T cell-deficient and NK-deficient hosts, and subcutaneous or intravenous injection routes. J Neurooncol 1990; 8: 121–132
- Van Kampen M, Kramer M, Hiemke C, Flugge G, Fuchs E. The chronic psychosocial stress paradigm in male tree shrews: Evaluation of a novel animal model for depressive disorders. Stress 2002; 5: 37–46
- Willner P, Mitchell PJ. The validity of animal models of predisposition to depression. Behav Pharmacol 2002; 13: 169–188
- Wunderlich J, Shearer G, Livingstone A. Current Protocols in Immunology. John Wiley:, New York 1997; Vol 1:, 3.11.1-3.11.20