Abstract
Exposure to stress leads to adaptive responses including both behavioral and physiological changes. This process is induced by the activation of multiple brain regions. The present study examined the role of the rostral perirhinal cortex (rPRh) in behavioral changes following electrical foot shock-induced stress. The rPRh of rats was lesioned bilaterally by local microinjections of 10 μg N-methyl-d-aspartic acid (NMDA) before foot shocks (0.7 mA, 1 s). The effects of these lesions on foot shock-induced changes in exploratory behaviors were tested in the open field (4 h, 48 h, 72 h, and 14 days after foot shocks) and the light–dark box (7 days after foot shocks). Foot-shocked and sham-lesioned rats showed several well known behavioral changes in the open field (e.g., immobility, reduction of exploratory activity) most marked at 48 h after foot shocks, and the light–dark box (e.g., reduction of time spent and activity in the lit compartment). All these stress-induced behavioral changes were blocked by neurotoxic lesions of the rPRh. Furthermore, rPRh lesions did not affect behavior in the open field and the light–dark box in unstressed rats. Taken together, these data indicate that the rPRh is involved in neurophysiological mechanisms that mediate changes induced by foot-shock stress in exploratory behaviors which indicate unconditioned fear or anxiety.
Introduction
Stress responses are adaptive processes that play a critical role in the life and survival of an animal. Animals are in a continual interchange with the environment, both physically and behaviorally. However, there are differences in how animals interact with their environment as a result of the individual experiences and coping strategies of each animal. Stress is induced when an individual perceives stimuli or situations as potentially threatening without the possibility to gain control, i.e., to cope with the situation.
Exposure to a stressor elicits physiological and behavioral responses. The physiological changes include an increase in blood pressure, and the release of several hormones (adrenaline, noradrenaline, and glucocorticoids), which support protective functions like the fight-or-flight response, and increase the pain threshold. In addition, other functions like reproduction and body growth are adversely affected by stress (Cannon Citation1929; Selye Citation1950; Henry Citation1992). Furthermore, stress affects mood, motivation and emotions, as well as declarative and emotional memory (Henry Citation1992; McEwen and Sapolsky Citation1995; Shors Citation2004).
The behavioral changes induced by stress can be tested in a variety of behavioral paradigms (Blanchard et al. Citation2001; Patchev and Patchev Citation2006). For example, van Dijken et al. (Citation1992a) and colleagues showed long-lasting behavioral changes after exposure to inescapable foot shocks, a physical stressor. Stressed rats show a suppression of locomotion, rearing and sniffing behavior and an increase of immobility measured in the open field. Furthermore, exposure to a predator-associated stimulus potentiates defensive responses, as measured by the acoustic startle response, decreases exploratory behavior and risk assessment behavior in the elevated plus-maze, and exploratory activity in the light–dark box, as well as decreasing the time spent in social interaction (Adamec and Shallow Citation1993; Metz et al. Citation2001; Blundell et al. Citation2005).
To understand the psychological and biological aspects of stress, it is important to investigate the neural circuitry processing the stress-inducing stimuli, the expression of the different stress responses and of the related coping strategies. One brain region that merits attention is the rostral perirhinal cortex (rPRh). This brain area has some characteristics suggesting involvement in the processing of stress. The PRh has strong reciprocal connections with the amygdala, the hippocampus, and the prefrontal cortex (McDonald Citation1998; McDonald et al. Citation1999; Naber et al. Citation1999; Shi and Cassell Citation1999; Pikkarainen and Pitkanen Citation2001; Hoover and Vertes Citation2007), and each of these structures has been shown to be involved in stress or processing of stress reactions (Gray Citation1993; McEwen and Sapolsky Citation1995; Adamec et al. Citation2001; McEwen Citation2007; Sandi and Pinelo-Nava Citation2007). Neuroanatomical and autoradiographic studies have shown a strong noradrenergic innervation of the PRh which has a high density of α2-adrenergic and corticotropin releasing factor (CRF) receptors (Fallon et al. Citation1978; De Souza et al. Citation1985; King et al. Citation1995). Exposure to stress activates the brain noradrenergic and CRF systems leading to an increase of noradrenergic and CRF transmission in several brain regions (Koob Citation1999). In addition, the PRh is critically involved in aversive information processing, expression of unconditioned fear or anxiety and emotional learning (Herzog and Otto Citation1997, Citation1998; Tassoni et al. Citation1999; Otto et al. Citation2000; Schulz et al. Citation2004; Schulz-Klaus et al. Citation2005). Finally, exposure to learned aversive stimuli, visceral pain stimuli and restraint stress leads to an activation of the PRh as measured by the c-fos technique (Cullinan et al. Citation1995; Traub et al. Citation1996; Campeau and Watson Citation1997).
The objective of the present study was to investigate the role of the rPRh in stress-related behavioral changes that indicate unconditioned fear or anxiety. Accordingly, neurotoxic lesions of rPRh were carried out before rats were exposed to foot shock stress. The effects of rPRh lesions on stress-induced behavioral changes were than tested in the open field and the light–dark box, with the hypothesis that the rPRh lesion would alter these behavioral changes.
Materials and methods
Animals
Fifty-four male Sprague-Dawley rats (obtained from Charles River, Sulzfeld, Germany) weighing between 200 and 320 g were used. They were housed in groups of 4–5 rats under a 12 h light/dark cycle (lights on from 7:00 to 19:00 h) with food and water continuously available in a temperature and humidity-controlled animal holding room (21°C, 60%). All experiments were performed between 8:00 and 18:00 h. The experiments were performed in accordance with the ethical guidelines for the care and use of laboratory animals for experiments and were approved by the local animal care committee (Regierungspräsidium Tübingen, ZP 3/06).
Surgery and intracranial infusions
Rats were anesthetized with chloral hydrate (400 mg/kg i.p. in 0.9% saline; Sigma, Steinheim, Germany) and placed into a stereotaxic frame with blunt ear bars. The skull was exposed and small holes were drilled dorsal to the rPRh. Lesions were induced by bilateral infusions of N-methyl-d-aspartic acid (NMDA) (10 μg in 0.5 μl phosphate buffer; Sigma, Steinheim, Germany), using a 1 μl Hamilton syringe. The syringe was fixed in a Kopf microinjection unit, lowered to the rPRh (3.0 mm caudal, ± 6.2 mm lateral, 7.6 mm ventral to Bregma; according to Paxinos and Watson (Citation1997)) and left in place for 4 min before and after infusion which also took 4 min (infusion rate: 0.125 μl/min). Sham surgery involved the same manipulations but phosphate buffer (pH 7.4) was infused instead of NMDA solution. Experiments began 4–5 days after the surgery, when all rats had fully recovered.
Stress exposure—apparatus and procedure
The rats for shock exposure were placed individually in a box (32 cm × 35 cm × 25 cm, Med Associates Inc., St Albans, VT, USA) with a transparent Plexiglas front and a grid floor consisting of stainless steel bars (1 cm apart). After 5 min acclimatization, the rats received 30 foot shocks (constant current: 0.7 mA, 1 s duration, 1 min inter-stimulus interval) generated by a shock scrambler (Med Associates Inc.) and administered via the floor grid. Control rats were placed in the shock box without receiving the foot shocks. After each test, the box was cleaned with water.
Open field—apparatus and procedure
The open field (ActiMot, TSE Systems, Bad Homburg, Germany) was a Plexiglas square arena with transparent walls (92 × 92 × 32 cm). Locomotor activity [movements of the whole body were recorded as distance traveled (m)] of the rat was automatically monitored by infrared detectors (2.5 cm between two detectors) located at a height of 2.5 cm. In addition, behavior was videotaped. The tapes were later analyzed by an observer who was not aware of the rats' test conditions for the following behaviors: duration and frequency of exploratory behavior (exploratory locomotion and sniffing behavior), immobility and rearing behavior.
The rats were tested in the open field four times: 4 h, 48 h, 72 h and 14 days after the stress exposure. For the test, the rats were placed in the arena for 10 min under normal light conditions (250 lux). After each test, the apparatus was cleaned with water.
Light–dark-box—apparatus and procedure
The light–dark box apparatus (ActiMot) consists of a dark compartment (48 × 92 × 32 cm) with dark walls and dark cover and a lit compartment (48 × 92 × 32 cm) with transparent walls. A small door (10 × 11 cm) in the dividing wall allowed the rats to move freely between the compartments. The locomotor activity (total traveled distance [m], distance traveled in the lit compartment) was monitored by infrared detectors (see above) and was automatically calculated by the ActiMot software. Furthermore, the rats were videotaped. Time spent in the lit compartment and the frequency of risk assessment behavior (oriented scanning toward the lit compartment) were analyzed by an observer who was not aware of the rats' test conditions.
Rats were tested in the light–dark paradigm 7 days after stress exposure; these were the same rats used for the open-field tests and therefore had some open field experience (4, 48, and 72 h after stress, see above). The test was performed between 12:00 and 18:00 h. The rats were placed in a corner of the lit compartment facing toward the dark box and allowed to explore the compartments for 10 min. After each session the apparatus was cleaned with water.
Histology
After final testing, all rats were killed with an overdose of Nembutal (i.p.) and their brains were removed. Coronal sections (50 μm) were cut on a freezing microtome and stained with thionine. Only those rats with bilateral lesions restricted to the rPRh were used for analysis. Rats with misplaced infusions sites and lesions mainly including adjacent brain structures were excluded from further analysis.
Statistical analysis
The behavioral data were analyzed by analysis of variance (ANOVA, Software: Systat Software Inc., San Jose, CA, USA) using test session as within subject factors (open field experiment) and lesion (sham vs. rPRh) and stress condition (stressed vs. unstressed) as between subject factors. After statistical detection of effects, single ANOVAs for each test day were performed. Alpha was set at 0.05.
Results
Histology
A photomicrograph of a representative lesion is shown in . Only data from rats with lesions mainly including the rPRh (n = 23) were taken for further analysis. If large parts of the auditory cortex were lesioned (n = 5) or if there was any damage to the amygdala (n = 6) or to the caudate putamen (n = 1), the rats were excluded from further analysis. Nineteen rats received injections of phosphate buffer into the rPRh (sham-lesion rats).
Open field
Exploratory behavior
depicts the exploratory behavior in the open-field during the different experimental sessions. ANOVA including stress condition (stressed vs. unstressed), lesion (sham vs. lesion) and test time (4 h, 48 h, 72 h, and 14 days) revealed highly significant effects of the stress condition (F(1,38) = 10.47, p = 0.003), lesion (F(1,38) = 12.79, p = 0.001) and test time (F(3,114) = 15.37, p < 0.001). Furthermore, all possible interactions reached a significant level, especially test time × lesion × stress condition (F(3,114) = 5.70, p = 0.001; for all other interactions: F>6.46, p < 0.008). Single ANOVAs for each test session detected an effect of the stress condition at 4 and 48 h (all F>5.90, p < 0.02; ) but not in the later test sessions (all F < 3.43, p>0.07). This stress effect was blocked by the rPRh lesion at the 48 h time point (interaction stress condition × lesion: F(1,38) = 16.47, p < 0.001; ), and the lesion effect narrowly failed to reach statistical significance at 4 h (F(1,38) = 3.55, p = 0.07). In summary, foot shock-induced stress decreased exploratory behavior in the open field, tested at 4 and 48 h. Neurotoxic lesions of the rPRh tended to block this effect at 4 h and clearly blocked this stress effect at 48 h.
Figure 2 Effects of bilateral rPRh lesions on the stress-induced behavior changes measured in the open field. (A) exploration behavior, (B) immobility behavior. Rats were tested 4 h, 48 h, 72 h, and 14 days after foot-shock stress in the following four groups of rats: No stress+sham lesioned (n = 10; ♦), no stress+rPRh lesion (n = 12; ◊), stress+sham lesioned (n = 9; •) and stress+rPRh lesion (n = 11; ○). Data are presented as group means ± SEM. *p < 0.05, **p < 0.01, ***p < 0.001 (stress effect),+++p < 0.001 (interaction stress condition × lesion).
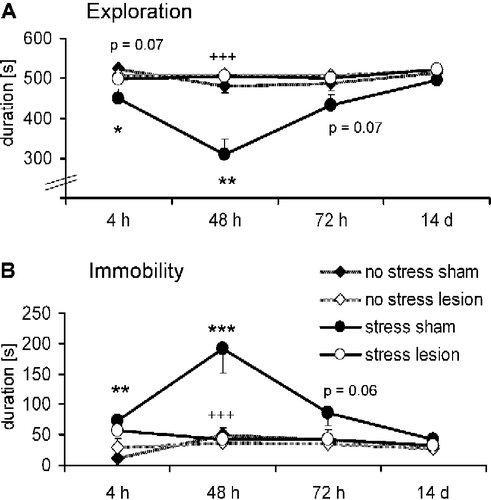
Similar effects of the rPRh lesions were found for the distance traveled in the open field (data not shown; all F>3.97, p < 0.05) tested 48 h post stress, whereas stress only tended to decrease the rearing behavior tested at the same time point (data not shown; all F>2.97, p′ < 0.09).
Immobility
presents the immobility behavior in the open-field during the different experimental sessions. ANOVA revealed significant effects of the stress condition (F(1,38) = 14.00, p = 0.001), lesion (F(1,38) = 7.85, p = 0.008) and test time (F(3,114) = 9.52, p < 0.001). Furthermore, all possible interactions reached a significant level, especially test time × lesion × stress condition (F(3,114) = 4.91, p = 0.003; all other interactions: F>4.70, p < 0.004). Single ANOVAs for each test session revealed a significant effect of the stress condition at 4 and 48 h (all F>9.33, p < 0.004) but not in the later test sessions (all F < 3.87, p>0.06). This stress effect was blocked at the 48 h time point (interaction stress condition × lesion: F(1,38) = 11.12, p = 0.001) but not at 4 h (F(1,38) = 1.44, p = 0.23). In summary, foot shock-induced stress increased immobility in the open field, tested at 4 and 48 h, and neurotoxic lesions of the rPRh blocked this stress effect at 48 h.
Light–dark-box
depicts the time the rats spent in the lit compartment of the light–dark box. Statistical analysis revealed a time-dependency of the effects of foot shock-induced stress seven days previously on this behavior: During the first 5 min of the test (open bars), the time spent in the lit compartment was not affected by stress (F(1,37) = 0.16, p = 0.69) whereas it was significantly reduced in the second 5 min (F(1,37) = 4.20, p = 0.04). Induction of the lesion had no major effect on either stressed or unstressed rats (F(1,37) = 2.29, p = 0.13) but the significant interaction stress condition × lesion (F(1,37) = 6.89, p = 0.01) indicates that the stress effects on the time spent in the lit compartment were blocked by neurotoxic rPRh lesions.
Figure 3 Effects of bilateral rPRh lesions on the stress-induced behavior changes measured in the light–dark box. The bars represent behaviors of sham lesioned and rPRh lesioned rats measured seven days after foot-shock stress in the first (white bars) and second 5 min (dark bars) of the light–dark box test session. (A) time spent in lit compartment, (B) locomotor activity in lit compartment (measured as traveled distance in light), and (C) total locomotor activity (measured as total traveled distance). Four different groups were tested: No stress+sham lesioned (n = 10), no stress+rPRh lesion (n = 12), stress+sham lesioned (n = 9) and stress+rPRh lesion (n = 11). Data are presented as group means ± SEM. *p < 0.05, **p < 0.01 (interaction stress condition × lesion).
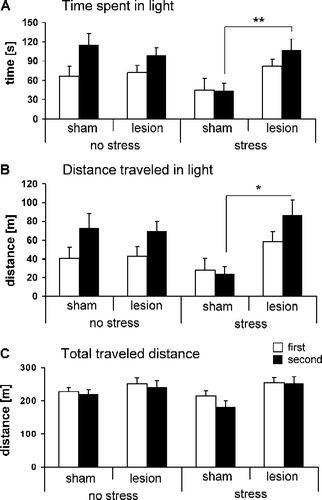
Similar effects of the rPRh lesions were found for the distance traveled in the lit compartment (; interaction stress condition × lesion: F(1,37) = 6.14, p = 0.02), whereas the total distance traveled was not affected by stress (; F(1,37) = 0.387, p = 0.54).
Discussion
The present study investigated the role of the rPRh in inescapable foot shock stress-induced behavioral changes. The results clearly show that neurotoxic lesions of the rPRh prevented all stress-induced behavior changes observed in the present study, in particular a decrease of locomotion, and exploration and an increase in immobility in the open field paradigm, as well as a decrease in exploratory behavior in the light compartment of the light–dark box.
The open field and light–dark test are well established paradigms for the study of stress-induced behavioral changes (Lister Citation1990; Patchev and Patchev Citation2006). In the present study, the exposure to inescapable foot shocks induced a time-dependent inhibition of exploratory behavior in the open field. Specifically, locomotion measured as traveled distance was decreased 48 h after administration of stress stimuli (data not shown), immobility was increased 4 and 48 h post-stress, exploration was decreased 4 and 48 h post-stress and rearing behavior was tended to be decreased 48 h post-stress (data not shown). These behavioral stress effects have been reported by other investigators (van Dijken et al. Citation1992a,Citationb; Van den Berg et al. Citation1998; Pijlman and Van Ree Citation2002). In the present study all of these behavioral changes were normalized again at day 14. This indicates that the effects of the foot shock stress used in the present study were less prolonged compared with other studies in the literature. For example, van Dijken et al. (Citation1992a,Citationb) showed a long-lasting effect of stress on the same behavioral readouts at days 14 and 28 after stress exposure. These differences are probably due to a different “stress protocol”: Van Dijken and colleagues used fewer foot shocks (10 instead of 30) but their foot shocks had a stronger intensity (1 mA instead of 0.7 mA), a much longer duration (6 s instead 1 s), and a variable interstimulus interval (instead of constant). It is well known that some of these variables (e.g., predictability) are crucial for the long-lasting effects of stress (McCarty et al. Citation1988; Tilders et al. Citation1999).
Interestingly, in the light–dark box experiment, stress did not affect the main locomotor activity measured by total distance traveled and total risk assessment (data not shown). Possibly the existence of a withdrawal opportunity (into the dark compartment) could compensate for the reduction in locomotion observed in the open-field. Furthermore, the stressed rats did not differ from unstressed rats in their exploratory behavior of the lit compartment, measured as distance traveled and time spent in the lit compartment, in the first 5 min of the light–dark box test session. However, in the second 5 min of the test, the stress-exposed rats show less exploratory behavior compared with the control rats. A possible explanation for this delayed behavioral effect of stress could be that at the beginning of the test the novelty effect (anxiety) overlays the stress exposure effect. In the second part of the test session, this novelty effect is weaker (Lister Citation1990) and the stress-induced behavioral changes are becoming more evident. Generally it cannot be excluded that the preceding experience in one test paradigm (e.g., open field) has a sustained influence on the tested rats which in turn could impact on their behavioral responses in the subsequent tests (e.g., light–dark box test).
The main finding of the present study is that the behavioral changes induced by stress exposure could be blocked by rPRh lesions, indicating that the rPRh is involved in mediating these stress effects. Thus, rPRh lesioned rats did not show the stress-induced reduction of locomotion, exploration or the stress-induced increase of immobility measured in the open field. In addition, the stress-induced reduction of exploratory behavior in the lit compartment of the light–dark box test session was not observed in the rats with rPRh lesions. It is possible that rPRh lesions may have affected the perception of nociceptive information and consequently prevented the stress-induced behavioral changes. However, in a previous study (Schulz et al. Citation2004) we showed that temporary inactivation of the rPRh by injections of muscimol did not reduced foot shock sensitivity measured by the flinch and shuffle thresholds which is a sensitive procedure for quantifying responses to a foot shock (Evans Citation1961). This indicates that the observed behavioral changes in the present study are not caused by changes in the perception of nociceptive information.
To date few studies have investigated the importance of the PRh in the processing of stressors or in the expression of stress responses (Meunier et al. Citation2006). The majority of studies have examined the role of the PRh in mnemonic function including recognition memory, identification and discrimination functions (Suzuki Citation1996). However, the PRh is also important in associative learning of neutral stimuli (object association; Murray and Richmond Citation2001) as well as of emotional stimuli, as in fear learning (Herzog and Otto Citation1997, Citation1998; Tassoni et al. Citation1999; Otto et al. Citation2000; Schulz et al. Citation2004). Finally, in a previous study (Schulz-Klaus et al. Citation2005), we demonstrated the importance of the rPRh in anxiety. The present results show moreover that the rPRh is also essential for stress-induced inhibition of exploratory behavior, which is assumed to reflect enhanced general anxiety.
The results of this study do not allow dissociation of the role of the rPRh in the different phases of processing of stressful stimuli, i.e., initial stimulus perception and assessment of the information as an aversive event, later acquisition and consolidation of this negative information, and its retrieval, and the expression of the behavioral changes. To investigate the role of the rPRh in these different phases further studies are required, perhaps involving temporary rPRh inactivation during a specific phase. An interesting feature of the results is that the rPRh lesion prevented the stress-induced behavior in the open field most strongly 48 h after stress. This late appearance of the lesion effect indicates that the rPRh may not be involved in the early stressor processing phases, including perception, but rather is involved in the late phases. Future studies could explore this suggestion.
In summary, the present study shows that neurotoxic lesions of the rPRh prevent stress-induced behavioral changes in the open field and in the light–dark box. These results indicate that the rPRh plays an essential role in the mechanism by which stress-inducing stimuli lead to altered expression of exploratory behaviors.
Acknowledgements
This work was supported by the Deutsche Forschungsgemeinschaft (Schu 2338/1-1).
Declaration of interest: The author reports no conflicts of interest. The author alone is responsible for the content and writing of the paper.
References
- Adamec RE, Shallow T. Lasting effects on rodent anxiety of a single exposure to a cat. Physiol Behav 1993; 54: 101–109
- Adamec RE, Blundell J, Collins A. Neural plasticity and stress induced changes in defense in the rat. Neurosci Biobehav Rev 2001; 25: 721–744
- Blanchard RJ, McKittrick CR, Blanchard DC. Animal models of social stress: Effects on behavior and brain neurochemical systems. Physiol Behav 2001; 73: 261–271
- Blundell J, Adamec R, Burton P. Role of NMDA receptors in the syndrome of behavioral changes produced by predator stress. Physiol Behav 2005; 86: 233–243
- Campeau S, Watson SJ. Neuroendocrine and behavioral responses and brain pattern of c-fos induction associated with audiogenic stress. J Neuroendocrinol 1997; 9: 577–588
- Cannon WB. Bodily changes in pain, hunger, fear and rage. Appleton, New York, NY 1929
- Cullinan WE, Herman JP, Battaglia DF, Akil H, Watson SJ. Pattern and time course of immediate early gene expression in rat brain following acute stress. Neuroscience 1995; 64: 477–505
- De Souza EB, Insel TR, Perrin MH, Rivier J, Vale WW, Kuhar MJ. Corticotropin-releasing factor receptors are widely distributed within the rat central nervous system: An autoradiographic study. J Neurosci 1985; 5: 3189–3203
- Evans WO. A new technique for the investigation of some analgesic drugs on reflexive behaviour in the rat. Psychopharmacologia 1961; 2: 318–325
- Fallon JH, Koziell DA, Moore RY. Catecholamine innervation of the basal forebrain II. Amygdala, suprarhinal cortex and entorhinal cortex. J Comp Neurol 1978; 180: 509–532
- Gray TS. Amygdaloid CRF pathways. Role in autonomic, neuroendocrine, and behavioral responses to stress. Ann N Y Acad Sci 1993; 697: 53–60
- Henry JP. Biological basis of the stress response. Integr Physiol Behav Sci 1992; 27: 66–83
- Herzog C, Otto T. Odor-guided fear conditioning in rats: 2. Lesions of the anterior perirhinal cortex disrupt fear conditioned to the explicit conditioned stimulus but not to the training context. Behav Neurosci 1997; 111: 1265–1272
- Herzog C, Otto T. Contributions of anterior perirhinal cortex to olfactory and contextual fear conditioning. Neuroreport 1998; 9: 1855–1859
- Hoover WB, Vertes RP. Anatomical analysis of afferent projections to the medial prefrontal cortex in the rat. Brain Struct Funct 2007; 212: 149–179
- King PR, Gundlach AL, Louis WJ. Quantitative autoradiographic localization in rat brain of alpha 2-adrenergic and non-adrenergic I-receptor binding sites labelled by [3H]rilmenidine. Brain Res 1995; 675: 264–278
- Koob GF. Corticotropin-releasing factor, norepinephrine, and stress. Biol Psychiatry 1999; 46: 1167–1180
- Lister RG. Ethologically-based animal models of anxiety disorders. Pharmacol Ther 1990; 46: 321–340
- McCarty R, Horwatt K, Konarska M. Chronic stress and sympathetic-adrenal medullary responsiveness. Soc Sci Med 1988; 26: 333–341
- McDonald AJ. Cortical pathways to the mammalian amygdala. Prog Neurobiol 1998; 55: 257–332
- McDonald AJ, Shammah-Lagnado SJ, Shi C, Davis M. Cortical afferents to the extended amygdala. Ann N Y Acad Sci 1999; 877: 309–338
- McEwen BS. Physiology and neurobiology of stress and adaptation: Central role of the brain. Physiol Rev 2007; 87: 873–904
- McEwen BS, Sapolsky RM. Stress and cognitive function. Curr Opin Neurobiol 1995; 5: 205–216
- Metz GA, Schwab ME, Welzl H. The effects of acute and chronic stress on motor and sensory performance in male Lewis rats. Physiol Behav 2001; 72: 29–35
- Meunier M, Cirilli L, Bachevalier J. Responses to affective stimuli in monkeys with entorhinal or perirhinal cortex lesions. J Neurosci 2006; 26: 7718–7722
- Murray EA, Richmond BJ. Role of perirhinal cortex in object perception, memory, and associations. Curr Opin Neurobiol 2001; 11: 188–193
- Naber PA, Witter MP, Lopez da Silva FH. Perirhinal cortex input to the hippocampus in the rat: Evidence for parallel pathways, both direct and indirect. A combined physiological and anatomical study. Eur J Neurosci 1999; 11: 4119–4133
- Otto T, Cousens G, Herzog C. Behavioral and neuropsychological foundations of olfactory fear conditioning. Behav Brain Res 2000; 110: 119–128
- Patchev VK, Patchev AV. Experimental models of stress. Dialogues Clin Neurosci 2006; 8: 417–432
- Paxinos G, Watson C. The rat brain in stereotaxic coordinates. Academic Press, San Diego 1997
- Pijlman FT, Van Ree JM. Physical but not emotional stress induces a delay in behavioural coping responses in rats. Behav Brain Res 2002; 136: 365–373
- Pikkarainen M, Pitkanen A. Projections from the lateral, basal and accessory basal nuclei of the amygdala to the perirhinal and postrhinal cortices in rat. Cereb Cortex 2001; 11: 1064–1082
- Sandi C, Pinelo-Nava MT. Stress and memory: Behavioral effects and neurobiological mechanisms. Neural Plast 2007; 2007(78970)1–20
- Schulz B, Fendt M, Richardson R, Schnitzler HU. Temporary inactivation of the perirhinal cortex by muscimol injections block acquisiton and expression of fear-potentiated startle. Eur J Neurosci 2004; 19: 713–720
- Schulz-Klaus B, Fendt M, Schnitzler HU. Temporary inactivation of the rostral perirhinal cortex induces an anxiolytic-like effect on the elevated plus-maze and on the yohimbine-enhanced startle response. Behav Brain Res 2005; 163: 168–173
- Selye H. The physiology and pathology of exposure to stress, Acta, Montreal 1950
- Shi C, Cassell MD. Perirhinal cortex projections to the amygdaloid complex and hippocampal formation in the rat. J Comp Neurol 1999; 406: 299–328
- Shors TJ. Learning during stressful times. Learn Mem 2004; 11: 137–144
- Suzuki WA. The anatomy, physiology and functions of the perirhinal cortex. Curr Opin Neurobiol 1996; 6: 179–186
- Tassoni G, Lorenzini CA, Baldi E, Sacchetti B, Bucherelli C. A peculiar pattern of temporal involvement of rat perirhinal cortex in memory processing. Behav Neurosci 1999; 113: 1161–1169
- Tilders FJ, Schmidt ED, Hoogendijk WJ, Swaab DF. Delayed effects of stress and immune activation. Baillieres Best Pract Res Clin Endocrinol Metab 1999; 13: 523–540
- Traub RJ, Silva E, Gebhart GF, Solodkin A. Noxious colorectal distention induced-c-Fos protein in limbic brain structures in the rat. Neurosci Lett 1996; 215: 165–168
- Van den Berg CL, Lamberts RR, Wolterink G, Wiegant VM, Van Ree JM. Emotional and footshock stimuli induce differential long-lasting behavioural effects in rats; involvement of opioids. Brain Res 1998; 799: 6–15
- van Dijken HH, Mos J, van der Heyden JA, Tilders FJ. Characterization of stress-induced long-term behavioural changes in rats: evidence in favor of anxiety. Physiol Behav 1992a; 52: 945–951
- van Dijken HH, van der Heyden JA, Mos J, Tilders FJ. Inescapable footshocks induce progressive and long-lasting behavioural changes in male rats. Physiol Behav 1992b; 51: 787–794