Abstract
Previous findings of decreased mental and perceptual motor performance during parabolic flights have been attributed mainly to the primary effects of weightlessness rather than the accompanying effects of stress and altered mood. Although recent studies have alluded to the possible negative effects of stress on performance, there has been no attempt to investigate this during parabolic flights. Over a period of 3 years, 27 human participants (male n = 18, mean age ± SD 34.67 ± 7.59 years; female n = 9, 36.22 ± 9.92 years) were recruited with the aim to evaluate if, and to what extent, parabolic flights are accompanied by changes in mood. Furthermore, the relationships between mood and physiological markers of stress and arousal, namely circulating stress hormones (ACTH, cortisol, epinephrine, norepinephrine, prolactin and brain activity (EEG)) were investigated. A strong and significant correlation was found between circulating stress hormone concentrations and perceived physical state, motivational state (MOT) and psychological strain (PSYCHO), whereas no interaction between mood and EEG or EEG and stress hormone concentrations was observed. Therefore, two different stress responses appear to be present during parabolic flight. The first seems to be characterised by general cortical arousal, whereas the second seems to evolve from the adrenomedullary system. It is likely that both these mechanisms have different effects on mental and perceptual motor performance, which require further investigation and should to be taken into account when interpreting previous weightlessness research.
Introduction
Microgravity induces a range of complex biological changes that affect various systems and processes of the body. There is evidence that central and peripheral neural processes, e.g. mental and perceptual motor performance, are negatively affected by weightlessness during space missions (Fowler et al. Citation2000a; Bock et al. Citation2001). Opportunities to study the body's response to weightlessness during space shuttle and space station missions are limited and alternative methods of inducing microgravity, such as parabolic flights, are commonly used for weightlessness experiments. While the reductions in mental and perceptual motor performance seen during space flight have also been consistently observed during weightlessness in parabolic flight experiments (Bock Citation1996; Bock et al. Citation2003; Augurelle et al. Citation2003; Girgenrath et al. Citation2004), it remains unclear whether these effects are the direct result of weightlessness (zero gravity), or rather the psycho-physiological effects of stress and/or arousal during the flight. It is conceivable that factors such as noise, confined space, and a negative perception of the situation all contribute to stress during parabolic flight experiments. For this reason, a more complete understanding of the stress response during parabolic flights is required.
During a parabolic flight manoeuvre, there is approximately 20 s of free flight, depending upon the type of aircraft, during which weightlessness is achieved. This period of weightlessness is preceded and followed by equal periods of hyper-gravity as the aircraft enters and exits the parabolic manoeuvre, respectively. Typically, multiple parabolic manoeuvres are performed consecutively during a flight resulting in repeated cycles of normal gravity (1 G), microgravity (∼0 G) and hyper-gravity (∼2 G). We recently demonstrated an acute stress response in parabolic flight participants that was characterised by alterations in circulating stress hormone concentrations (Schneider et al. Citation2007b) and higher frequency electroencephalography (EEG) spectra (Schneider et al. Citation2008a).
Besides, the physical responses associated with these fluctuations in gravity, which may include motion sickness, parabolic flight subjects must also deal with a wide range of emotions that are also likely to alter motor and cognitive functions. The present study aimed to assess the effect of parabolic flight on mood by assessing the subject's motivation and their perceived physical and psychological states before, during and after a parabolic flight. Additionally, the relationships between these psychological markers and physiological parameters, namely circulating stress hormone concentrations and EEG activity, were assessed. We hypothesised that mood, measured as self-perceived physical, motivational and psychological state, would be reduced during the course of the parabolic flight. Given that frontal areas of the cortex are most affected by emotions (Coan and Allen Citation2004), we hypothesised that these changes in mood would be related to changes in EEG activity, and we anticipated that changes in mood would also be correlated with alterations in stress hormone concentrations.
Materials and methods
With approval from the University ethics committee, 27 participants aged (mean ± SD) 35.19 ± 8.28 years (male n = 18, age 34.67 ± 7.59 years; female n = 9, age 36.22 ± 9.92 years) participated in one out of four parabolic flight campaigns. Parabolic flights were conducted by NOVESPACE in an Airbus 300 (A300 Zero-G) from September 2005 to September 2007. During each campaign we tested six to eight participants, depending on the number of flight days (three or four). All flights commenced at 9.30 am and followed the flight protocol described below. Three of the participants had previous experience with parabolic flights. Out of 27 participants, 6 suffered from severe motion sickness. As a result, not all the data could be obtained from those subjects (please refer to the specific methods section for further information). The aircraft A300 Zero-G is based at Mérignac International Airport in Bordeaux, France and flights are operated under the authority of the Centre d'Essais en Vole (CEV). The parabolic flight protocol is illustrated in . Each flight commenced with a test-parabola (parabola zero, 0), which was then followed by 30 experimental parabolas (parabolas 1–30), all within a time period of 90 min. Each parabola consisted of a characteristic set of changes in gravity (1.8 G–0 G–1.8 G), with each gravity phase lasting approximately 20–25 s and, therefore, each parabola lasting approximately 70 s. Parabolas were separated by 90 s of level light at normal gravity (1 G) and after each series of five parabolas there was a short 4–8 min break.
Figure 1 Flight procedure. After take off, prior to parabola zero, EEG recording, blood collection and assessment of mood state took place. EEG recording was repeated after parabolas 10, 20 and 30. Blood collection and mood assessment were repeated after parabolas 5, 15 and 25. Thirty minutes post-flight another data set of EEG, blood and mood data were collected. Each of the 31 parabolas is marked by two hyper-gravity phases (dark grey) and a zero-gravity phase (light grey).
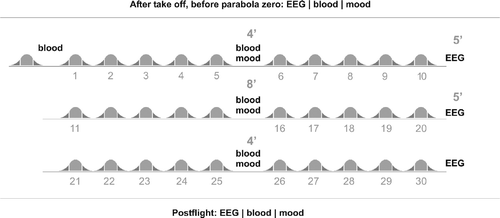
All participants underwent a thorough clinical examination prior to participation and gave written informed consent. Scopolamine, which is normally administered before parabolic flights to prevent motion sickness, was not dispensed to the participants; and none of them reported taking any other medication during the study. During the flight, each participant was seated in a standard flight seat and was secured with a safety belt for the first 25 parabolas. Participants were free to leave their seat and move about the cabin during the last five parabolas after the last period of in-flight data collection.
Mood assessment
Mood assessments were made using the MoodMeter®, a hand-held computer program that consists of two modules, Bodyfinder and Feelfinder. The Bodyfinder module assesses the current perceived physical state (PEPS) such as that traditionally incorporated into clinical or behavioural orientated research and is very sensitive to short-term alterations in mood. The Feelfinder module includes a short 16-item form of the “Eigenzustandsskala” (EZ-scale, (Nitsch Citation1976) and assesses psychological strain (PSYCHO) and motivational state (MOT). The MoodMeter® was validated over a 5-year period (2001–2005) on a total of 645 participants (Cronbach alpha intraclass correlation coefficient between 0.82 and 0.92) (Kleinert Citation2006).
For this study, the MoodMeter® was configured with 32 adjectives (16 Bodyfinder and 16 Feelfinder), that were stored and presented to participants on a touch screen handheld PC in a quasi-random sequence. Upon the presentation of each adjective, participants were asked to indicate how well that adjective described their current physical or mental state by selecting one out of six options from 0 (not at all) to 5 (totally). The response time for each adjective was limited to 5 s so as to discourage rational deliberation, and this time was shown with a progress bar at the bottom of the screen. On each occasion, completion of the mood assessment (all 32 adjectives) took the participants approximately 2 min. In the days prior to the experiment, all participants were familiarised with the MoodMeter® using a small catalogue of adjectives that were not included in the experiment. MoodMeter® instructions and adjectives were presented in German and the English equivalents have been used in the text. The mood assessment was completed on five occasions; during level flight before parabola 0 ( < 0), immediately after parabolas 5 (>5), 15 (>15), and 25 (>25) and 30 min after the completion of the flight (post-flight) while still on the aircraft.
From the 32 MoodMeter® adjectives, three different dimensions were formed for assessment: (PEPS), (PSYCHO) and (MOT). Each of these three dimensions was further categorised into four sub-dimensions. The first of the four (PEPS) sub-dimensions was physical energy, which includes adjectives associated with lethargy and tiredness (drained, weak, weary, shiftless). The other PEPS sub-dimensions were physical fitness (well-trained, vigorous, fit, strong), physical health (healthy, groggy, battered, sick) and physical flexibility (agile, flexible, immobile, stiff). PSYCHO was broken down into the following four sub-dimensions: positive mood (positive mood, cheerful), calmness (relaxed, calm), recovery (recuperated, well-rested) and relaxation (feeble, drowsy). The remaining four sub-dimensions characterised the (MOT): willingness to seek contact (open for contact, communicative), social acceptance (accepted, popular), readiness to strain (energetic, powerful) and self-confidence (experienced, self-confident).
Blood collection and analysis
Circulating hormone concentrations were measured in blood samples taken through an indwelling venous catheter that had been inserted into each participant's left arm by a physician 2 h prior to take off and was flushed with 0.9% saline. Blood was collected prior to parabola 0 ( < 0), and immediately following parabolas 5 (>5), 15 (>15) and 25 (>25), as per our preliminary investigation of nine subjects (Schneider et al. Citation2007b). For the present study, we included two additional blood collections, the first 30 min after the flight (post-flight) and the second (for the assessment of catecholamines only) immediately after parabola 0 (>0). At each collection the following samples were drawn into pre-chilled vacutainers: 3 ml EDTA blood for ACTH analysis, 10 ml for cortisol and prolactin analysis and 10 ml EGTA–GSH blood for catecholamine analysis. Blood samples were immediately cooled in flight and stored for up to 4 h at 8°C and then centrifuged at 5000 rpm for 10 min after the flight in a refrigerated centrifuge. Plasma was divided into aliquots to allow triplicate analyses, then stored at − 20°C for 7 days and then at − 80°C for 4 months prior to analysis. We have previously demonstrated that these blood sample preparation methods have little or no effect on the hormones of interest (Schneider et al. Citation2007a). To control for effects of time, several baseline measurements were conducted hourly starting at 9 am and ending at 1 pm the day before the flight for each participant (baseline).
Serum prolactin/cortisol and EDTA–plasma ACTH concentrations were detected by an immunoassay for the in vitro quantitative determination of hormone concentrations (prolactin and ACTH: sandwich principle [ACTH sensitivity: 1.0–2000 pg/ml or 0.220–440 pmol/l, inter-assay variability 2.5%, intra-assay variability 2.1%; PRL sensitivity: 1–10,000 μIU/ml or 0.0470–470 ng/ml, inter-assay variability 1.6%; intra-assay variability 1.2%], cortisol: competition principle [sensitivity: 0.5–1750 nmol/l or 0.018–63 μg/dl, inter-assay variability 1.5%, intra-assay variability 1.2%). The electrochemiluminescence immunoassays (ECLIA®) for prolactin, cortisol and ACTH were provided by ROCHE (Mannheim, Germany) and processed on an immunoassay analyser (Elecsys 2010, ROCHE). Catecholamines were analysed by high pressure liquid chromatography using electrochemical detection (HPLC reagents: CHROMSYSTEMS, Muenchen Germany [epinephrine sensitivity: 15–10,000 ng/l, inter-assay variability 6.5%, intra-assay variability 7.9%; norepinephrine sensitivity: 15–10,000 ng/l, inter-assay variability 5.9%; intra-assay variability 3.9%]; hardware: SHIMADZU, Langenfeld, Germany).
EEG recording and analysis
An EEG-cap (Electro-Cap International, Inc., USA) with 19 Ag–AgCl-electrodes arranged in the international 10–20 system (Jasper Citation1958) was mounted on each participant's head. Each electrode was referenced to a reference electrode that was mounted in the triangle of FP1, FP2 and FZ. Electrodes were filled with Electro-GelTM (Electro-Cap International, Inc., Eaton, USA) to optimise signal transduction. If the impedance of an electrode exceeded 10 kΩ, then the electrode was excluded from further analysis. The analogue signal of the EEG was amplified and converted to digital signals using Braintronics Iso 1064 CE Box (Braintronics B. V., Hl Almere, The Netherlands) and stored with a frequency of 256 Hz on a hard disk of the Neurofile XP EEG-System (IT-Med, Usingen, Germany). Simultaneously, participants were monitored by video that was triggered by the EEG-system to synchronise each individual's activities and EEG-signals during analysis. During the EEG recordings, participants had their eyes closed and were asked not to move or speak. Recordings lasted for 2 min and were supervised by an investigator. When there was no EEG recording, participants were free to look around but were required to stay seated. EEG data were collected after take off prior to parabola 0 ( < 0), then after parabolas 10 (>10), 20 (>20) and 30 (>30), and again 30 min after landing (post-flight) ().
EEG analysis was performed offline with the Brain Vision Analyzer (Brain Products, München, Germany) software. High- and low-pass filters were applied so that a frequency range from 0.5 to 128 Hz remained for analysis (time constant 0.3183 s; 24 dB/octave, notch filter at 50 Hz).
A systematic protocol for excluding artefacts followed that included careful visual inspection of all EEG data, which was time aligned with video data, and automated exclusion procedures which were set to gradient threshold < 35 μV; maximum and minimum amplitude between − 100 and 100 μV. After FFT analysis (see below), data were inspected manually for artefacts on each channel and frequency domain. This was done as it is difficult to recognise artefacts (e.g. vibrations of the aeroplane) in raw signals at, or above, 5 Hz. If there was a dominant frequency it would have been present in all channels and therefore made visible by FFT analysis, which was not the case. Prior to standardised low-resolution brain electromagnetic tomography (sLORETA analysis (see below), data were referenced using an average reference; again this would have eliminated distracting frequencies. Following this, the data were segmented into 4 s sections, where an overlap of 10% was accepted. Segmented data were then baseline corrected, and analysed by spectral analysis (FFT) for α1 [7.5–10.0 Hz], α2 (10.0–12.5 Hz), β1 (12.5–18.0 Hz) and β2 (18.0–35.0 Hz) activity, and averaged over all remaining segments. δ (0.5–3.5 Hz) and θ (3.5–7.5 Hz) were disregarded from analysis as those frequencies tended to be influenced by aeroplane movements. For correlation of EEG activity with stress hormone concentrations and perceived mood, an average value (MEAN) was calculated from all electrodes yielding the overall EEG-activity within each frequency range.
For localisation of EEG activity, sLORETA was used, which enables the spatial identification and analysis of brain cortical activity via traditional EEG recordings (Pascual-Marqui et al. Citation2002; Pascual-Marqui Citation2002b; Fuchs et al. Citation2002; Jurcak et al. Citation2007). Given that extracranial EEG activity is evoked by synchronised postsynaptic potentials (PSPs), which are located in clusters of pyramidal cells (Martin Citation1991), sLORETA makes it possible to determine the three-dimensional orientation of these highly synchronised PSPs. Cortical regions are created by allocating the raw sLORETA values of individual voxels to their corresponding Broadmann areas (BA) or cerebral gyri on the basis of the coordinates of the digitised Talairach Atlas (Talairach and Tournoux Citation1988). First, the coordinates of the 19 electrode positions were applied to a digitised MRI version of the Talairach Atlas (McConnell Brain Imaging Centre, Montréal Neurological Institute, McGill University). These Talairach coordinates were then used to compute the sLORETA transformation matrix. Following transformation to an average reference, EEG activity of a minimum of 25 four second epochs of artefact-free resting EEG were averaged to calculate cross spectra in sLORETA for α1 (7.5–10 Hz), α2 (10–12.5 Hz), β1 (12.5–18 Hz) and β2 (18–35 Hz) bands for each subject. Using the sLORETA transformation matrix, cross spectra of each subject and for each frequency band were then transformed to sLORETA files. This resulted in the corresponding three-dimensional cortical distribution of the electrical neuronal generators for each subject. The computed sLORETA image displayed the cortical neuronal oscillators in 6430 voxels, with a spatial resolution of 5 mm (Pascual-Marqui Citation2002a). Δ and θ frequencies again were not analysed.
Statistics
Mood assessment
For each dimension, changes across time were determined using Friedman's repeated measures analysis of variance (ANOVA). Wilcoxon-paired samples test was used as post hoc test, wherever a significant measurement effect was detected so as to determine the exact location of differences. Due to motion sickness, six of the 27 participants were not able to proceed with the data acquisition and were therefore excluded from analysis. Gender specific effects were calculated on 7 females and 14 males.
Blood hormone concentrations
Blood variables were analysed using repeated measures ANOVA (single factor: MEASUREMENT) and Fisher's Least Significant Difference (LSD) test was used for post hoc testing as required. Due to motion sickness and/or problems with blood collection, datasets of only 15 (epinephrine/norepinephrine) and 19 (cortisol, prolactin, ACTH) participants were available for blood hormone analysis. To distinguish between stress responses in the hypothalamus-pituitary-adrenal axis and the sympathetic nervous system (SNS) and adrenal medulla, the norepinephrine/epinephrine ratio (NOR/EPI ratio) was calculated and analysed (Schofl et al. Citation1997). Norepinephrine concentrations have frequently been considered to primarily reflect physical influences such as exercise, while an increase in epinephrine has been shown to result also from mental stress. Hence, the NOR/EPI ratio is an indication of the different response of individuals to stressors and allows an estimation of relative sympathetic (NOR)/adrenomedullary (EPI) activation (Peaston and Weinkove Citation2004). As the Kolmogorov–Smirnov test revealed a non-normal distribution (p < 0.1) for the NOR/EPI ratio, we conducted a log-transform of these values using V ′ = ln(V) before analysis. Gender-specific effects were calculated using gender as an additional factor in the statistical analysis.
Sloreta
To display differences in EEG spectral activity between pre-, in- and post-flight, paired sampled t-tests were computed for log of the ratio of averages (log of F-ratio) of sLORETA power at each voxel. Statistical significance was assessed using a non-parametric randomisation test (Nichols and Holmes Citation2002). Results were corrected for multiple comparisons on the cluster level. The output file contained the exact randomisation probabilities (corrected for multiple comparisons) for testing cluster size (Nichols and Holmes Citation2002). These voxel-by-voxel t-values are displayed as statistical parametric maps (SPMs).
Correlations
For the assessment of relationships, between 78 and 99 data sets from the 27 participants were available for analysis. Correlations between EEG activity, hormone concentrations and PEPS, MOT and PSYCH were analysed using Spearmans' Rank Correlation (Spearman R). The relationship between EEG activity and hormonal concentrations was analysed by linear regression. Due to the limited time available between parabolic manoeuvres during flight, it was not possible to make all measurements concurrently (see ). Therefore, the relationships with EEG were established using hormone concentration and psychological data that were collected five parabolas beforehand.
For all statistical analyses two-tailed level of significance was set at *p < 0.05, **p < 0.01, ***p < 0.001. Data in the text are presented as means ± standard deviation. All statistical analyses were performed using Statistica version 7.1 (STATSOFT, Tulsa, USA).
Results
Mood assessment
PEPS tended to decrease throughout the flight. Although there was a tendency for PEPS to be reduced after parabolas 5 (p = 0.10) and 10 (p = 0.07) compared to the first in-flight measurement, post hoc analysis revealed that this trend only became significant following parabola 25 (p < 0.05, ). PEPS increased again at the post-flight measurement (p < 0.05). Of the sub-dimensions of PEPS, physical energy and physical health decreased early during the flight and recuperated post-flight (Figur and E), whereas neither physical fitness nor physical flexibility showed any significant changes throughout the flight.
Figure 2 Perceived physical state (A), motivational state (B), psychological strain (C), physical energy (D), physical health (E), self-confidence (F), calmness (G), willingness to seek contact (H) and relaxation (I) for measurements nos. 1–4 in-flight and measurement no. 5 30 min post-flight. Data show means (small box), standard error (large box) and standard deviation (bar). Stars indicate significant changes (*p < 0.05; **p < 0.01) followed by: 1 different to 1st measurement, 2 different to 2nd measurement, 3 different to 3rd measurement, 4 different to fourth measurement, 5 different to fifth measurement.
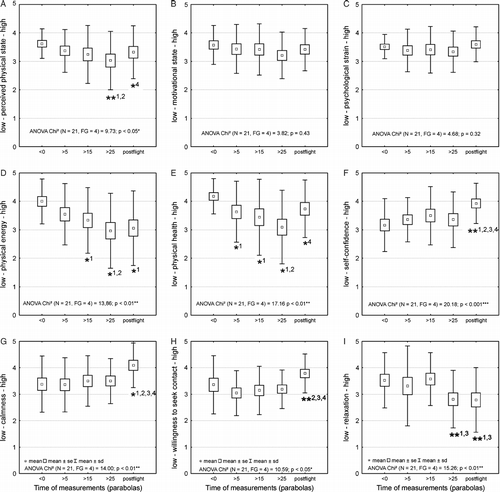
(MOT) and (PSYCHO) did not change throughout the flight period ( and C). Of the sub-dimensions, an increase in self-confidence was observed post-flight (p < 0.01) (). A similar effect was observed for calmness (p < 0.05) () and willingness to seek contact (p < 0.01) (). Towards the end of the flight after parabola 25, as well during post-flight, relaxation was significantly decreased compared to the prior in-flight measurements (p < 0.01) (). All other sub-dimensions of (MOT) and (PSYCHO) remained relatively stable throughout the flight.
Interestingly, a gender specific analysis of mood data showed that the decrease in (PEPS) was only prominent for male participants (p < 0.01) whereas female participants did not show any significant changes (p = 0.62). There were no gender differences for (PSYCHO) (male p = 0.23, female p = 0.75) or (MOT) (male p = 0.11, female p = 0.85).
Blood hormone concentrations
Plasma concentrations of prolactin, ACTH, epinephrine and cortisol significantly increased (two-fold increase for cortisol and prolactin, four-fold increase for ACTH and epinephrine) during the 90 min flight period (, B, C and D). Compared with a pre-flight measurement, appropriately chronologically adjusted, made the day before the flight, no changes were observed in the first in-flight measurement prior to parabola zero in any of the hormone concentrations. The increase in plasma cortisol concentration reached significance after the first set of five parabolas (p < 0.05) and then further increased during the flight. At 30 min post-flight, which was approximately 70 min after the final parabola was flown, cortisol values remained elevated compared to the baseline measurement (p < 0.001). Plasma concentrations of prolactin (p < 0.001), ACTH (p < 0.01) and epinephrine (p < 0.001) all increased significantly by the third flight measurement, after parabola 15, and then returned to baseline values during the post-flight period. Plasma norepinephrine concentration was reduced (p < 0.01) after parabola 15 (). Accordingly, the NOR/EPI ratio showed a significant decrease in measurements after parabola 15 and 25 (p < 0.001) (). Although no significant changes were observed in plasma norepinephrine or epinephrine concentrations from pre-flight ( < 0) to flight (>0), the NOR/EPI ratio was significantly reduced after the first parabola (>0) compared with the pre-flight ( < 0) value (p < 0.05). None of the hormone concentrations showed any gender-specific effect (GENDER*MEASUREMENT: cortisol p = 0.75; prolactin p = 0.97; ACTH p = 0.79; epinephrine p = 0.26; norepinephrine p = 0.37).
Figure 3 Progression of plasma hormone concentrations from baseline through parabolic flight to post-flight measurements (first to sixth for (A) ACTH, (B) COR, (C) prolactin; and from first to seventh samples for (D) epinephrine, (E) norepinephrine, and (F) NOR/EP ratiosI). Data show means ± 95% confidence intervals. Asterisks indicate significant changes (*p < 0.05; **p < 0.01) followed by: 1 versus first measurement, 2 versus second measurement, 3 versus third measurement, 4 versus fourth measurement, 5 versus fifth measurement, 6 versus sixth measurement.
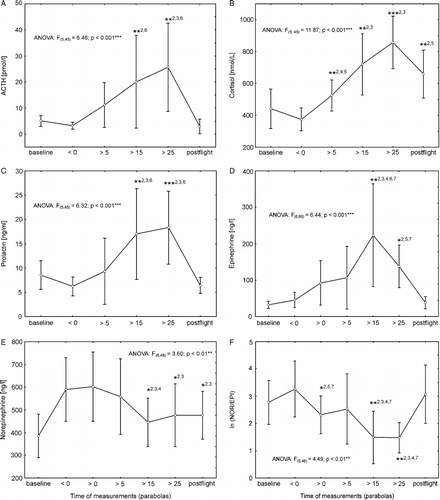
EEG activity
Comparisons of EEG activity from pre-flight to in-flight revealed a distinct increase in β1 activity in the frontal lobe and medial frontal gyrus (p < 0.01, ). No significant changes were observed within the β2, α1 and α2 frequency ranges. Within the flight, there were no further changes in any of the frequency ranges. However, there was a significant decrease in β1 activity in the post-flight period that was localised to the frontal lobe and medial frontal gyrus (p < 0.05, ).
Figure 4 Standardised low resolution brain electromagnetic tomography (sLORETA) differences in the EEG β1 frequency band during parabolic flight. Comparisons of (A) pre-flight activity and in-flight activity, and (B) in-flight activity and post-flight activity (B), respectively. Images depicting SPMs seen from different perspectives are based on voxel-by-voxel t-values of differences. Red colours indicate increased activity in the in-flight condition (A). Blue colours indicate decreased activity in the post-flight condition. Changes were found to be localised in the frontal lobe and medial frontal gyrus. Structural anatomy is shown in grey scale (L left, R right, A anterior, P posterior).
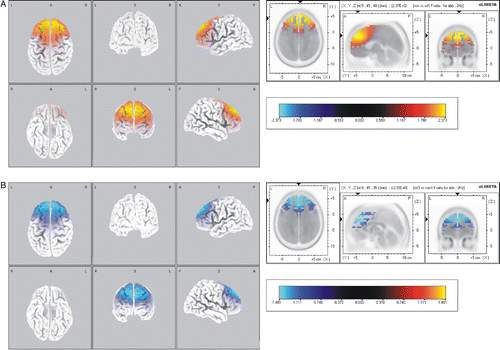
Correlations
Stress hormone concentrations were significantly correlated with the various psychological parameters (). Increases in plasma cortisol, epinephrine, ACTH and prolactin concentrations were associated with reductions in perceived physical state, (PSYCHO) and (MOT); whereas decreases in plasma norepinephrine concentration were significantly correlated with negative changes in motivation and (PSYCHO).
Figure 5 Correlations between subjective mood assessment and plasma hormone concentrations during parabolic flight. (A) cortisol (COR [μg/dl]); (B) ACTH [pmol/l]; (C) prolactin (PRL [ng/ml]); (D) epinephrine (EPI [ng/l]); (E) norepinephrine (NOR [ng/l]), and perceived physical state (PEPS, left column), psychological strain (PSYCHO, middle column) and motivational state (MOT, right column). Solid line represents linear regression, dotted lines 95% confidence intervals. All correlations, except those with NOR/PEPS (D, column 1; p = 0.08), were significant (p < 0.05).
![Figure 5 Correlations between subjective mood assessment and plasma hormone concentrations during parabolic flight. (A) cortisol (COR [μg/dl]); (B) ACTH [pmol/l]; (C) prolactin (PRL [ng/ml]); (D) epinephrine (EPI [ng/l]); (E) norepinephrine (NOR [ng/l]), and perceived physical state (PEPS, left column), psychological strain (PSYCHO, middle column) and motivational state (MOT, right column). Solid line represents linear regression, dotted lines 95% confidence intervals. All correlations, except those with NOR/PEPS (D, column 1; p = 0.08), were significant (p < 0.05).](/cms/asset/9ef9d3b5-4125-43b0-9d60-80aa786955f7/ists_a_350085_f0005_b.gif)
Regression analysis between EEG activity and blood hormone concentrations as well as psychological parameters revealed few clear relationships. Plasma ACTH concentration and α1 activity were weakly correlated. Similarly, there was a weak but significant association between plasma norepinephrine concentration and α1-frequency power. There were no significant relationships between EEG activity and any of the psychological parameters.
Discussion
Research conducted over the last two decades has concluded that reduced gravity during parabolic flights has a significant impact on mental and perceptual motor performance. Besides the primary influences of weightlessness, so called “non-specific stressors” (Fowler et al. Citation2000a, Heuer et al. Citation2003) or “environmental stressors” (Fowler and Manzey Citation2000) are also considered to have an impact on performance. To better understand the stress response during parabolic flight we assessed changes in mood by examining perceived physical state, (MOT) and (PSYCHO). We hypothesised that alterations in mood would be related to physiological markers of stress, namely circulating hormone concentrations and EEG activity. Parabolic flight had a significant negative impact on (PEPS). A clear relationship between circulating stress hormone concentrations and perceived changes in mood was detected, whereas no clear relationships between EEG activity and perceived changes in mood, or between EEG activity and stress hormone concentrations, were observed.
Mood assessment
The primary aim of the present study was to assess the effect of parabolic flight on mood. Previous studies so far have only assessed mood to picture mental and emotional state during long-term space flight (Manzey et al. Citation1998; Lorenz et al. Citation1996; Rosnet et al. Citation1998). The conditions during parabolic flight are different in that the periods of weightlessness are interspersed with periods of normal gravity (1 G) and hyper-gravity (∼2 G). Therefore, it cannot be assumed that the mood response seen during long-term space flight will be the same as that during a parabolic flight.
The present study is novel in that it represents the first time that changes in mood during parabolic flight have been assessed. As hypothesised, a general decrease in (PEPS) was observed during the flight, especially in the perceived physical energy and relaxation sub-dimensions, which reached significance mid-flight and persisted until the final post-flight measurement. While we did not include measures of physical performance, the reduction in PEPS was accompanied by anecdotal reports from participants of being physically exhausted at the end of the flight. The reduction in PEPS was most pronounced in male subjects. Whether this reflects a true gender effect on mood, or whether the assessment of mood needs to be gender specific requires further investigation.
During the post-flight period there was a trend towards the recovery of PEPS. Perceived physical health showed a similar trend in that is was significantly reduced throughout the flight before an acute and significant recovery in the post-flight period. The recovery of (PEPS) and physical health was accompanied, and possibly explained by, an increase of self-confidence, calmness and willingness to seek contact in the post-flight period. Therefore, despite their physical exhaustion, participants demonstrated a recovery in mood that possibly reflects their sense of achievement or relief immediately after the flight. We have demonstrated a similar, although, less pronounced effect, after short-term exposure to hyper-gravity (Schneider et al. Citation2008b). From our findings, it appears that participation in a parabolic flight has a pronounced effect on mood, particularly the perception of physical state. This alteration in mood is transient and recovers relatively quickly during the post-flight period. Similar transient changes of emotional states were reported for the transition phases (i.e. the last days on earth and the first 3 weeks in space as well as the first 2 weeks after return to earth) of long-term space flight (Manzey et al. Citation1998; Lorenz et al. Citation1996). We conclude that neither weightlessness nor hyper-gravity per se affects changes in mood, but it is more likely that these changes are caused by non-specific effects of multiple stressors (Fowler et al. Citation2000b) (e.g. workload, environmental conditions, recurring alterations of gravity (Manzey et al. Citation2000)).
Blood hormone concentrations
We aimed to clarify the effect of parabolic flight on circulating stress hormone concentrations, and the present data extend our earlier preliminary findings that included only a limited number of participants (Schneider et al. Citation2007b). The recurring changes in gravitational forces during parabolic flights led to a significant increase in circulating stress hormone levels. While the first measurement in-flight, prior to parabola zero, revealed no significant difference compared to a baseline measurements made the previous day (), hormone levels progressively increased throughout the course of the 90 min flight. Whereas our earlier studies connected this increase in stress hormone concentration to motion sickness, it is interesting to note the rapid decrease in the NOR/EPI ratio after the first parabolic manoeuvre (), which is indicative of an increase in the adrenomedullary secretory activity (Schofl et al. Citation1997). This might be evoked by the altered vestibular inputs which provide the overwhelming sensation of weightlessness that most participants had never experienced before. The decrease in the NOR/EPI ratio may best be explained as a response to fear, anxiety and perhaps joy. It is possible that alterations in venous return, baroreceptor activity and cardiovascular dynamics also contributed to the NOR/EPI response, but this is most likely to be the case in subjects who are prone to syncope, of which there were none in the present study (Kamiya et al. Citation1999). Changes in epinephrine and norepinephrine levels have been observed during long-term space flight, although these changes have been attributed to physical and metabolic loads rather than weightlessness per se (Macho, Koska et al. Citation2003; Stowe et al. Citation2003). We conclude that processes governed and controlled by the central and peripheral nervous systems might potentially be affected by this psychological stress reaction at an early stage of a parabolic flight.
In addition to the psychological impact discussed above, the gradual increase in circulating stress hormone concentrations during the in-flight period may be a reflection of the physical burden of the flight that is reflected in the mood data. While we do not have direct measures of physical capacity and fatigue throughout the flight, there was a clear and significant reduction in perceived physical state. Previous studies have linked the development of nausea or motion sickness during parabolic flight to the elevation in stress hormone concentrations (Schneider et al. Citation2007b; Otto et al. Citation2006). It is interesting to note that even experienced flight participants (e.g. investigators) often report motion sickness during the last five to ten parabolas of a flight, and this physical burden may, independent of any psychological burden, contribute to the observed stress hormone response. The recovery of the NOR/EPI ratio, as well as the overall decrease in stress hormone concentrations during the post-flight period, supports the notion that the increase in stress measures is tightly connected to the flight itself (Schneider et al. Citation2007b). The finding that norepinephrine levels at the first in-flight measurement before parabola zero were greatly increased might be due to the volunteers' preparation for the flights, activating a sympathetic response.
EEG
Changes in EEG activity during the present study concur with earlier findings that there is an increased β1 activity (averaged across the whole cortex) during a parabolic flight (Schneider et al. Citation2008a). It is widely agreed that β EEG activity represents a form of cortical arousal, whereas increased α activity reflects a relaxed state (Miller Citation2007). Therefore, the increase in β activity is likely to represent an increase in cortical arousal during parabolic flight. It is now important to consider the localisation of this increased activity. A key finding of this study was that the increase in β1 activity during the flight was localised within the frontal lobe. Recent evidence indicates that the frontal cortex mediates or regulates amygdala activity via strong projections (Mcdonald et al. Citation1996; Sesack et al. Citation1989), especially in the regulation of emotion. Thus, the frontal cortex is viewed as an extension of different sub- and posterior-cortical systems. Faw (Citation2003) classifies those systems as Perceiver, Verbaliser, Motivator, Attender and Coordinator. Based on our finding of an increased β1 activity in the frontal cortex in-flight, particular attention should be paid to the emotional (Coan and Allen Citation2004) and attentional subsystems (Niedermeyer Citation2003; Neuper and Pfurtscheller Citation2001). There is little doubt that participation in a parabolic flight is strongly associated with increases in emotion and attention. This is characterised by an increase in the activity of the frontal lobe and medial frontal gyrus immediately after take-off, followed by a decrease in the same areas post-flight. Interestingly, there was no further change during the flight beyond the initial increase in EEG activity. Thus, the recurring changes in gravitational forces seem to have no further impact on brain cortical activity because a high degree of cortical activation is reached early during the flight.
Overall discussion: The relationships between perceived mood, circulating stress hormones and EEG activity
We hypothesised that changes in mood throughout the parabolic flight would be related to changes in EEG activity, and we anticipated that changes in mood would also be correlated with alterations in stress hormone concentrations. Our data show that there is a decrease in (PEPS) during the course of a parabolic flight, which corresponds with an increase in circulating stress hormone concentrations. Although neither motivational state nor (PSYCHO) showed any changes during the flight, there was a significant correlation between these dimensions and stress hormone concentrations. This suggests that changes in an individual's perception of stress during parabolic flight are closely coupled with changes in stress hormone concentrations. These physiological and psychological manifestations of stress may contribute to the previously observed declines in performance during parabolic flights (Mierau, Girgenrath et al. Citation2008; Girgenrath et al. Citation2004; Bock et al. Citation2003).
Interestingly, no significant correlations were observed between psychological data and EEG activity. This was the case for all EEG frequency ranges, including the higher frequency areas >13 Hz that are well known to be affected by stress and arousal (Guger et al. Citation2005). Furthermore, there was no clear correlation between EEG activity and stress hormone concentrations in the present study. The finding that plasma ACTH and norepinephrine concentrations showed a weak correlation with α1 activity whereas no other correlations between hormone concentrations and EEG were found probably indicates that this was an incidental finding. This is despite previous reports that link an increase in central neural activity, especially in the β–frequency range, with ongoing release of stress hormones (Stock et al. Citation1996; Buckley and Schatzberg Citation2005). The lack of significant relationships with EEG activity in the present study might be because EEG activity was already significantly elevated at the start of the flight. Conversely, we have previously demonstrated that stress hormone concentrations at the start of the in-flight period, prior to parabola zero, are not different from those taken several days before (Schneider et al. Citation2007b). No further changes in EEG activity were observed during the course of the flight, whereas stress hormone concentrations increased, and perception of mood decreased, throughout the flight. This indicates that there are two different, and potentially unrelated, responses. First, there seems to be a general cortical arousal which is characterised by an increased EEG activity, especially in the β1–frequency range. Secondly, our data provide evidence of a connection between stress hormone concentrations and mood. As the term ‘stress’ is related to a state in which a real or perceived threat to homeostasis has been detected or perceived, it might be helpful to distinguish a general effect of cortical arousal (i.e. that measurable by an increase in EEG activity), from a stress response involving the adrenomedullary system. Indeed, this differentiation is the subject of ongoing debate (Day and Walker Citation2007; Pfaff et al. Citation2007). Establishing relationships between the responses is complex and requires further investigation. It is plausible that the perception and processing of these stress responses will vary between individuals, and what may be interpreted as an arousal response (e.g. altered EEG activity before flight) may later be interpreted as a stress response (e.g. altered EEG activity during flight).
Identification of these stress and arousal responses support the notion that, apart from the effects of weightlessness, one must also consider possible effects of stress and/or arousal on the reductions in mental and perceptual motor performance that have previously been observed during parabolic flights (Fowler and Manzey Citation2000; Fowler et al. Citation2000b). It remains to be determined whether these processes enhance or impair performance. Increased arousal is known to affect central processes (Simpson et al. Citation2001a; Simpson et al. Citation2001b; Jones and Hardy Citation1988) as well as peripheral processes (Brunton et al. Citation2006). While alterations in arousal or stress might enhance attentional processes, peripheral implementation and control might be negatively affected. For example, the elevated epinephrine concentrations observed during parabolic flight in the present study might induce muscle tremor (Brunton et al. Citation2006), and this may contribute to the impaired fine motor performance previously observed during parabolic flight (Bock et al. Citation2003). Conversely, elevated hormone concentrations may have a positive effect; the intravenous administration of norepinephrine (Cahill and Alkire Citation2003) and cortisol (Buchanan and Lovallo Citation2001) lead to increases in arousal and improvements in memory consolidation. In addition, an association between increases in blood cortisol concentration and improvements in cognitive task performance has previously been demonstrated (Wolf et al. Citation2001).
This study is limited by the small number of electrodes used for sLORETA. This was dictated by the organisational limits of parabolic flight experimentation in that there was not time prior to each flight to prepare more electrodes than this. Increasing the number of electrodes, located not only on the scalp but on the whole head, may give a much more detailed analysis of deeper brain regions (e.g. brainstem and cerebellum activity).
We aimed to investigate the stress response during parabolic flight. During the flight, prior to the first parabola, there was no significant change in circulating hormone concentrations compared with pre-flight. While this suggests that the observed changes in hormone concentrations are related to the parabolic flight procedure (especially the transitions to 1.8 G–0 G–1.8 G), we cannot exclude the possibility that similar results would be obtained during a standard 90 min flight. Future research should aim to differentiate the effects of the parabolic flight procedure from that of regular flying conditions.
This study demonstrates that parabolic flight is associated with a stress response that is characterised by alterations in perceived physical, psychological and motivational state, and these changes in mood are related to elevated stress hormone concentrations. There is also a change in cortical excitability during parabolic flight, which is likely to be a manifestation of an arousal response, as there was no clear relationship between EEG activity and the other markers of stress. In light of this study, previous findings that mental and perceptual motor performance is reduced because of weightlessness need to be reconsidered. It is likely that the stress response during parabolic flight, in addition to the physiological effects of weightlessness, influence performance. For this reason, the use of parabolic flights to test the effects of weightlessness on human performance may be limited.
Acknowledgements
We would like to thank all our subjects for taking part in this study. A special thank goes to the team at NOVESPACE for giving us all the support we needed and to the analytical team of the Institute of Cardiology and Sports Medicine, particularly Anke Manderfeld and Astrid Hofrichter, for their outstanding expertise and help. Thanks to Anja Steinbacher for her help during data analysis. The comments and suggestions of three unknown reviewers as well as the Editor, Prof. J.A. Russell, on previous versions of this manuscript were very much appreciated. This study was made possible by a grant from the German Space Agency (DLR) 50WB0519.
Declaration of interest: The authors report no conflicts of interest. The authors alone are responsible for the content and writing of the paper.
References
- Augurelle AS, Penta M, White O, Thonnard JL. The effects of a change in gravity on the dynamics of prehension. Exp Brain Res 2003; 148: 533–540
- Bock O. Grasping of virtual objects in changed gravity. Aviat Space Environ Med 1996; 67: 1185–1189
- Bock O, Fowler B, Comfort D. Human sensorimotor coordination during spaceflight: an analysis of pointing and tracking responses during the “Neurolab” Space Shuttle mission. Aviat Space Environ Med 2001; 72: 877–883
- Bock O, Abeele S, Eversheim U. Sensorimotor performance and computational demand during short-term exposure to microgravity. Aviat Space Environ Med 2003; 74: 1256–1262
- Brunton L, Lazo J, Parker K. Goodman and Gilman's the pharmacological basis of therapeutics. McGraw-Hill, New York, NY 2006
- Buchanan TW, Lovallo WR. Enhanced memory for emotional material following stress-level cortisol treatment in humans. Psychoneuroendocrinology 2001; 26: 307–317
- Buckley TM, Schatzberg AF. On the interactions of the hypothalamic-pituitary-adrenal (HPA) axis and sleep: Normal HPA axis activity and circadian rhythm, exemplary sleep disorders. J Clin Endocrinol Metab 2005; 90: 3106–3114
- Cahill L, Alkire MT. Epinephrine enhancement of human memory consolidation: Interaction with arousal at encoding. Neurobiol Learn Mem 2003; 79: 194–198
- Coan JA, Allen JJ. Frontal EEG asymmetry as a moderator and mediator of emotion. Biol Psychol 2004; 67: 7–49
- Day TA, Walker FR. More appraisal please: A commentary on Pfaff et al. (2007) “Relations between mechanisms of CNS arousal and mechanisms of stress”. Stress 2007; 10: 311–313, discussion 314-5
- Faw B. Pre-frontal executive committee for perception, working memory, attention, long-term memory, motor control, and thinking: A tutorial review. Conscious Cogn 2003; 12: 83–139
- Fowler B, Manzey D. Summary of research issues in monitoring of mental and perceptual-motor performance and stress in space. Aviat Space Environ Med 2000; 71: A76–A77
- Fowler B, Bock O, Comfort D. Is dual-task performance necessarily impaired in space?. Hum Factors 2000a; 42: 318–326
- Fowler B, Comfort D, Bock O. A review of cognitive and perceptual-motor performance in space. Aviat Space Environ Med 2000b; 71: A66–A68
- Fuchs M, Kastner J, Wagner M, Hawes S, Ebersole JS. A standardized boundary element method volume conductor model. Clin Neurophysiol 2002; 113: 702–712
- Girgenrath M, Bock O, Jungling S. Validity of the speed-accuracy tradeoff for prehension movements. Exp Brain Res 2004; 158: 415–420
- Guger C, Domej W, Lindner G, Edlinger G. Effects of cable car ascent to 2700 meters on mean EEG frequency and event-related desynchronization (ERD). Wien Med Wochenschr 2005; 155: 143–148
- Heuer H, Manzey D, Lorenz B, Sangals J. Impairments of manual tracking performance during spaceflight are associated with specific effects of microgravity on visuomotor transformations. Ergonomics 2003; 46: 920–934
- Jasper HH. The ten-twenty electrode system of the international Federation. Electroencephalogr Clin Neurophysiol Suppl 1958; 35: 371–375
- Jones JG, Hardy L. The effect of anxiety upon psychomotor performance. J Sport Sci 1988; 6: 59–67
- Jurcak V, Tsuzuki D, Dan I. 10/20, 10/10, and 10/5 systems revisited: their validity as relative head-surface-based positioning systems. Neuroimage 2007; 34: 1600–1611
- Kamiya A, Michikami D, Fu Q, Iwase S, Mano T. Sympathetic vasoconstriction and orthostatic intolerance after simulated microgravity. J Gravit Physiol 1999; 6: P101–P102
- Kleinert J. Adjektivliste zur Erfassung der wahrgenommenen körperlichen Verfassung (WKV): Skalenkonstruktion und erste psychometrische Befunde [Adjective list for assessing Perceived Physical State (PEPS). Scale construction and psychometric results]. Zeitschrift für Sportpsychologie 2006; 13: 156–164
- Lorenz B, Lorenz J, Manzey D. Performance and brain electrical activity during prolonged confinement. Adv Space Biol Med 1996; 5: 157–181
- Macho L, Koska J, Ksinantova L, Pacak K, Hoff T, Noskov VB, Grigoriev AI, Vigas M, Kvetnansky R. The response of endocrine system to stress loads during space flight in human subject. Adv Space Res 2003; 31: 1605–1610
- Manzey D, Lorenz B, Poljakov V. Mental performance in extreme environments: Results from a performance monitoring study during a 438-day spaceflight. Ergonomics 1998; 41: 537–559
- Manzey D, Lorenz TB, Heuers H, Sangals J. Impairments of manual tracking performance during spaceflight: More converging evidence from a 20-day space mission. Ergonomics 2000; 43: 589–609
- Martin JH. The collective electrical behavior of cortical neurons: The electroencephalogram and the mechanisms of epilepsy. Principles of neuroscience, ER Kandel JHSTMJ. Prentice-Hall, London 1991; 777–791
- Mcdonald AJ, Mascagni F, Guo L. Projections of the medial and lateral prefrontal cortices to the amygdala: A Phaseolus vulgaris leucoagglutinin study in the rat. Neuroscience 1996; 71: 55–75
- Miller R. Theory of the normal waking EEG: from single neurones to waveforms in the alpha, beta and gamma frequency ranges. Int J Psychophysiol 2007; 64: 18–23
- Mierau A, Girgenrath M, Bock O. Isometric force production during changed-Gz episodes of parabolic flight. Eur J Appl Physiol 2008; 102: 313–318
- Neuper C, Pfurtscheller G. Event-related dynamics of cortical rhythms: frequency-specific features and functional correlates. Int J Psychophysiol 2001; 43: 41–58
- Nichols TE, Holmes AP. Nonparametric permutation tests for functional neuroimaging: a primer with examples. Hum Brain Mapp 2002; 15: 1–25
- Niedermeyer E. Electrophysiology of the frontal lobe. Clin Electroencephalogr 2003; 34: 5–12
- Nitsch JR. Die Eigenzustandsskala (EZ-Skala) – Ein Versuch zur hierarchisch-mehrdimensionalen Befindlichkeitsskalierung [The Eigenszustandsskala (EZ-scale) – an approach to a ierarchical multidimensional scale of psychological feeling]. Beanspruchung im Sport: Beiträge zur psychologischen analyse sportlicher leistungssituation, J Nitsch, I Udris. Limpert, Bad Homburg 1976; 81–102
- Otto B, Riepl RL, Klosterhalfen S, Enck P. Endocrine correlates of acute nausea and vomiting. Auton Neurosci 2006; 129: 17–21
- Pascual-Marqui RD. Standardized low-resolution brain electromagnetic tomography (sLORETA): technical details. Methods Find Exp Clin Pharmacol 2002a; 24: 5–12
- Pascual-Marqui RD. Standardized low-resolution brain electromagnetic tomography (sLORETA): technical details. Methods Find Exp Clin Pharmacol 2002b; 24: 5–12
- Pascual-Marqui RD, Esslen M, Kochi K, Lehmann D. Functional imaging with low-resolution brain electromagnetic tomography (LORETA): A review. Methods Find Exp Clin Pharmacol 2002; 24: 91–95
- Peaston RT, Weinkove C. Measurement of catecholamines and their metabolites. Ann Clin Biochem 2004; 41: 17–38
- Pfaff DW, Martin EM, Ribeiro AC. Relations between mechanisms of CNS arousal and mechanisms of stress. Stress 2007; 10: 316–325
- Rosnet E, Cazes G, Vinokhodova A. Study of the psychological adaptation of the crew during a 135 days space simulation. Acta Astronaut 1998; 42: 265–272
- Schneider S, Brummer V, Carnahan H, Dubrowski A, Askew CD, Struder HK. Stress hormone stability: Processing of blood samples collected during parabolic flight A pre-flight comparison of different protocols. Clin Biochem 2007a; 40: 1332–1335
- Schneider S, Brummer V, Gobel S, Carnahan H, Dubrowski A, Struder HK. Parabolic flight experience is related to increased release of stress hormones. Eur J Appl Physiol 2007b; 100: 301–308
- Schneider S, Brummer V, Mierau A, Carnahan H, Dubrowski A, Struder HK. Increased brain cortical activity during parabolic flights has no influence on a motor tracking task. Exp Brain Res 2008a; 185: 571–579
- Schneider S, Göbel S, Kleinert J, Steinbacher A, Strüder HK. Centrifugal Acceleration to +3Gz is related to increased release of stress hormones and decreased mood in men and women. Stress 2008b, 11: 339–437
- Schofl C, Becker C, Prank K, von zur Muhlen A, Brabant G. Twenty-four-hour rhythms of plasma catecholamines and their relation to cardiovascular parameters in healthy young men. Eur J Endocrinol 1997; 137: 675–683
- Sesack SR, Deutch AY, Roth RH, Bunney BS. Topographical organization of the efferent projections of the medial prefrontal cortex in the rat: an anterograde tract-tracing study with Phaseolus vulgaris leucoagglutinin. J Comp Neurol 1989; 290: 213–242
- Simpson JR, Jr, Drevets WC, Snyder AZ, Gusnard DA, Raichle ME. Emotion-induced changes in human medial prefrontal cortex: II. During anticipatory anxiety. Proc Natl Acad Sci USA 2001a; 98: 688–693
- Simpson JR, Jr., Snyder AZ, Gusnard DA, Raichle ME. Emotion-induced changes in human medial prefrontal cortex: I. During cognitive task performance. Proc Natl Acad Sci USA 2001b; 98: 683–687
- Stock C, Baum M, Rosskopf P, Schober F, Weiss M, Liesen H. Electroencephalogram activity, catecholamines, and lymphocyte subpopulations after resistance exercise and during regeneration. Eur J Appl Physiol Occup Physiol 1996; 72: 235–241
- Stowe RP, Sams CF, Pierson DL. Effects of mission duration on neuroimmune responses in astronauts. Aviat Space Environ Med 2003; 74: 1281–1284
- Talairach J, Tournoux P. Co-planar stereotaxic atlas of the human brain. Thieme, Stuttgart 1988
- Wolf OT, Schommer NC, Hellhammer DH, McEwen BS, Kirschbaum C. The relationship between stress induced cortisol levels and memory differs between men and women. Psychoneuroendocrinology 2001; 26: 711–720